Abstract
The immune system is important for protection against pathogens and malignant cells. However, malfunction of the immune system can also result in detrimental auto-immune diseases, inflammatory diseases, cancers and allergies. The aryl hydrocarbon receptor (AhR), present in numerous tissues and cell subsets, including cells of the immune system, plays an important role in the functioning of the immune system. Activation of the AhR is for example associated with various effects on dendritic cells (DCs), regulatory T cells and the Th1/Th2 cell balance. These cells play a major role in the development of food allergy. Food allergy is an increasing health problem in both humans and animals. Despite the knowledge in risk factors and cellular mechanisms for food allergy, no approved treatments are available yet. Recently, it has been shown that activation of the AhR by dioxin-like compounds suppresses allergic sensitization by suppressing the absolute number of precursor and effector T cells, by preserving CD4+CD25+Foxp3+ Treg cells and by affecting DCs and their interaction with effector T cells. Future research should elucidate whether and how AhR activation can be used to interfere in food allergic responses in humans and in animals. This may lead to new prevention strategies and therapeutic possibilities for food allergy.
1. The aryl hydrocarbon receptor
1.1. The aryl hydrocarbon receptor and toxicity
The AhR, a cytosolic ligand-activated transcription factor, is a member of the eukaryotic Per-ARNT-Sim (PAS) domain protein family that was discovered in the 1970s and is present in numerous tissues and cell subsets throughout the body (Gu et al. Citation2000; Frericks et al. Citation2007). It mediates the toxic responses of a variety of chemical pollutants, including polyhalogenated aromatic hydrocarbons (PHAHs) and polycyclic aromatic hydrocarbons (PAHs). The persistent PHAH compound 2,3,7,8-tetrachlorodibenzo-[p]-dioxin (TCDD) is one of the most potent AhR ligands known (Denison & Nagy Citation2003). Exposure to TCDD has been shown to induce a wide variety of toxic effects in various species as shown in Table 1 (Denison & Nagy Citation2003).
1.2. Endogenous functions of the aryl hydrocarbon receptor
Next to mediating the toxicity of xenobiotics, the AhR plays a major role in developmental processes and physiology, including hematopoiesis, circadian rhythm, and in cell processes such as cell growth and differentiation (Shimba & Watabe Citation2009; Casado et al. Citation2010; Abel & Haarmann-Stemmann Citation2010; Denison et al. Citation2011). This is illustrated by AhR knockout mice, which have hypertrophy and hyperplasia of the heart, liver and gastrointestinal tract, vascular lesions in the uterus, small spleens and skin lesions (Fernandez-Salguero et al. Citation1997; Gonzalez & Fernandez-Salguero Citation1998). Furthermore, the AhR may be relevant in immunology for the differentiation and balance of T cell subsets in ongoing immune responses and the decision for active immunity or the induction of tolerance (Esser Citation2009). Dietary and endogenous AhR ligands, for example indoles, tetrapyroles and arachidonic acid metabolites appear to play a role in these different processes (Denison & Nagy Citation2003; Abel & Haarmann-Stemmann Citation2010).
1.3. Aryl hydrocarbon receptor dependent gene transcription
The AhR is associated with the chaperone proteins hsp90, hsp23 and the X-associated protein 2. Upon ligation, the AhR complex translocates to the nucleus, where the ligand:AhR dimerizes with AhR nuclear translocator (ARNT) and dissociates from the other subunits of the complex (Figure ). This will transform the ligand:AhR:ARNT complex into a high affinity DNA-binding form, which subsequently binds to a specific site on the DNA, the dioxin response element (DRE). There exist multiple DREs and these DREs are present upstream of AhR responsive genes such as CYP1A1 or AhR repressor protein (AhRR) (Denison & Nagy Citation2003; Mitchell & Elferink Citation2009; Denison et al. Citation2011). Prolonged AhR activation can be suppressed by dissociation of the AhR complex from the DRE, followed by export of the AhR into the cytosol and ubiquitin-mediated AhR proteosomal degradation. Furthermore, the AhR-mediated induction of CYP450 enzymes facilitates a negative feedback loop, resulting in the metabolic degradation of AhR agonists. However, in contrast to for instance PAHs, PHAHs like TCDD are resistant to metabolic degradation because of their halogenation. AhR-dependent induction of AhRR can reduce the formation of the ligand:AhR:ARNT complex (Figure ) (Mitchell & Elferink Citation2009; Denison et al. Citation2011).
Figure 1. Schematic overview of mechanisms involved in AhR activation. Scheme 1: After ligation of the AhR with an agonist, the ligand:AhR complex translocates to the nucleus where it dimerizes with ARNT. Then, hsp90, hsp23 and the X-associated protein 2 dissociate from the complex and the ligand:AhR:ARNT complex binds to DREs, resulting in the transcription of genes. Scheme 2: Proteolytic degradation of the AhR by the 25S proteosome. Schemes 3 and 4: AhR-mediated induction of the AhRR reduces the formation of the ligand:AhR:ARNT complex. Scheme 5: AhR ligands are metabolically depleted by CYP450 enzymes. Adapted from Mitchell and Elferink (Citation2009).
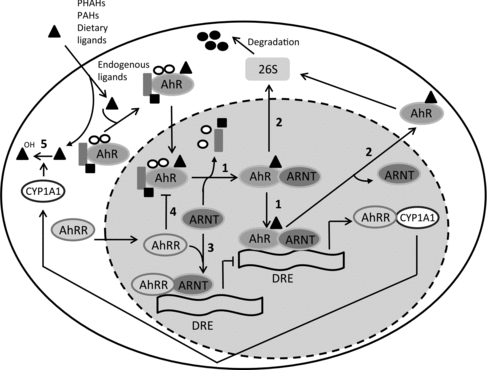
Next to this, AhR activation can interact and crosstalk with other nuclear proteins and signaling factors. For example, the AhR has been shown to cross-talk with estrogen receptor signaling pathways (Safe et al. Citation2000) and to interact with the NF-κβ subunit RelB (Vogel & Matsumura Citation2009). Some of these interactions with other genes and pathways are ligand specific. For instance, the AhR ligands TCDD and 3,3′-diindolylmethane (DIM) have different ability to activate distinct AhR-controlled pathways, including degree of activation of CYP450 enzymes and activation of the estrogen receptor degradation pathway (Okino et al. Citation2009). In summary, AhR activation results in the transcription of various genes and different pathways essential for numerous physiological functions, thereby exerting very diverse biological effects, including toxicity.
1.4. Ligands for the aryl hydrocarbon receptor
There are a wide variety of AhR ligands, which differ in structure, binding affinity and metabolic stability. Ligands of the AhR can be divided into two major categories, synthetic AhR ligands (including both halogenated and non-halogenated) and dietary/endogenous AhR ligands. Structures of various AhR ligands are shown in Figure .
1.4.1. Synthetic aryl hydrocarbon receptor ligands
Synthetic AhR ligands, e.g. PHAHs, PAHs and PAH-like chemicals, are in general associated with toxic effects, mediated through the AhR. Polychlorinated and polybrominated dibenzo-p-dioxins (e.g. TCDD), dibenzofurans and biphenyls (all PHAHs) have the highest binding affinity for the AhR (Denison & Heath-Pagliuso Citation1998). These compounds are environmental contaminants and their extensive halogenation makes these compounds environmentally and metabolically stable (Denison & Heath-Pagliuso Citation1998; Nguyen & Bradfield Citation2008). In addition, the presence of halogens at the lateral positions of the coplanar rings is associated with strong AhR binding potency (Nguyen & Bradfield Citation2008). PAHs, for example β-naphthoflavone, have reduced AhR binding affinity and are less metabolically stable when compared to PHAHs. Furthermore, the electronic and thermodynamic properties of these compounds are important in determining their AhR binding affinity (Kafafi et al. Citation1993). Three-dimensional quantitative structure-activity relationship studies revealed that planar, aromatic and hydrophobic ligands with a maximal dimension of 14Å × 12Å × 5Å can fit into the ligand binding pocket of the AhR (Waller & McKinney Citation1995).
1.4.2. Dietary and endogenous aryl hydrocarbon receptor ligands
The structure and physiochemical properties of endogenous and dietary AhR ligands differ markedly from synthetic AhR ligands (Denison & Heath-Pagliuso Citation1998). In general, most endogenous and dietary AhR ligands have low affinity for the AhR and only induce modest AhR-dependent gene transcription when compared to TCDD (Denison & Nagy Citation2003; Nguyen & Bradfield Citation2008). 2-(1′H-Indole-3′-carbonyl)-thiazole-4-carboxylic acid methyl ester (ITE), UV-products of tryptophan (such as 6-formylindolo[3,2-b]carbazole [FICZ]), metabolites from arachidonic acid and haeme degradation products are examples of endogenous AhR ligands. Furthermore, cruciferous vegetables like broccoli and cabbages contain indole-3-carbinol (I3C), which is condensed into the high affinity AhR ligands DIM and indolocarbazole (ICZ) by stomach acids (Denison & Nagy Citation2003). The AhR-dependent induction of CYP450 enzymes (e.g. CYP1A1) makes that these endogenous and dietary AhR ligands facilitate their own metabolism (Nguyen & Bradfield Citation2008), thereby greatly affecting their bio-availability.
2. Aryl hydrocarbon receptor and the adaptive immune system
As mentioned previously, the AhR could play an important functional role in the immune system, such as in food allergic responses. The AhR is present in a variety of cells of the immune system, including dendritic cells, T cells and B cells.
2.1. Aryl hydrocarbon receptor and dendritic cells
Dendritic cells (DCs) are professional antigen-presenting cells (APCs) linking innate immune responses with adaptive immune responses mediated by T and B lymphocytes. Immature DCs continuously sample foreign antigens. Encounter of a foreign antigen could induce DCs to mature and to migrate to the lymph node. Here, DCs present the foreign antigen as peptides on major histocompatibility complex (MHC) molecules to matching antigen-specific T cells. These maturated DCs also upregulate co-stimulatory molecules that are required for interaction with and activation of antigen-specific T cells. In the absence of inflammation, factors released by epithelial and mesenchymal cells (prostaglanding E2, transforming growth factor [TGF]-β, IL-10) induce only partial maturation of the DCs. This results in the induction of tolerance via induction of regulatory T cells. However, in the presence of pro-inflammatory factors (IL-1, IL-6, IL-8), induced by interaction of pathogens with toll-like receptors present on epithelial cells, mesenchymal cells and macrophages, maturated DCs induce active immunity by activating effector T cells (Mowat Citation2003; Coombes & Powrie Citation2008).
In the mouse, five major subsets of DCs have been defined so far and each subset may have a specific role in the immune system (Guilliams et al. Citation2010). In food allergy, the balance between tolerogenic CD103+ DCs and inflammatory CD11b+ DCs is important, because oral sensitization to peanut is accompanied by an increase of inflammatory CD11b+ DCs and a decrease of CD103+ DCs in the intestine (Smit et al. Citation2011). Moreover, mucosal CD103+ DCs are important in the induction of oral tolerance, since they have been shown to induce Foxp3+ regulatory T (Treg) cells in a TGF-β and retinoic acid dependent manner (Coombes et al. Citation2007; Sun et al. Citation2007; Coombes & Powrie Citation2008). These Treg cells regulate the intensity of immune responses and play an important role in regulating allergic sensitization (Van Wijk et al. Citation2007).
Little is known about AhR gene expression in DCs subsets. However, DCs in the spleen have been shown to express the AhR (Bankoti et al. Citation2010). Moreover, CD103+ DCs in the mesenteric lymph nodes (MLNs) have been shown to increase AhRR upon in vivo AhR activation by TCDD (Chmill et al. Citation2010).
In vitro AhR activation affects maturation and activation of bone marrow cultured DCs by increasing expression of MHC class II, CD80 and CD86 (Lee et al. Citation2007; Simones & Shepherd Citation2011; Benson & Shepherd Citation2011a). Moreover, in vitro AhR activation of DCs decreases the production of pro-inflammatory cytokines, for example IL-1β, IL-6, TNF-α and IL-12, increases the gene expression of regulatory factors, including TGF-β and indoleamine 2,3-dioxygenase (IDO), and increases the frequency of Foxp3+ Treg cells (Simones & Shepherd Citation2011; Benson & Shepherd Citation2011a).
Effects of in vivo AhR activation on DCs resemble the effects of in vitro AhR activation on DCs. In vivo TCDD treatment reduces the amount of splenic DCs and induces activation-like changes in splenic DCs (increase of MHCII, CD86, CD40, CD24, CD54), which may enhance their ability to provide activation signals to T cells resulting in dysregulation of immune responses (Vorderstrasse & Kerkvliet Citation2001; Vorderstrasse et al. Citation2003). Furthermore, DCs isolated from the lung draining lymph nodes of TCDD-treated mice reduce T cell activation (Jin et al. Citation2010). Next, in vivo AhR activation is associated with the induction of tolerogenic splenic CD103+ DCs and the subsequent induction of CD4+CD25+Foxp3+ Treg cells. An increase of retinoic acid production by DCs and/or an increase of the immunosuppressive IDO gene expression induced by AhR activation are proposed mechanisms for the increase of Treg cells and the suppression of the immune response (Vogel et al. Citation2008; Quintana et al. Citation2010; Mezrich et al. Citation2010; Nguyen et al. Citation2010).
2.2. Aryl hydrocarbon receptor and T cells
CD8+ and CD4+ T cells develop in the thymus and after migration to periphery they may become activated by APC presenting antigen on MHC class I or MHC class II, respectively, in the presence of co-stimulatory signals. Activated CD8+ T cells can be cytotoxic T cells, which are important for destroying tumor cells and virus infected cells. Activated CD4+ T cells can develop into T helper 1 (Th1), T helper 2 (Th2), T helper 17 (Th17) or Treg cells, which direct and exert different types of immune responses by the secretion of specific cytokines (Figure ). AhR expression is higher in CD8+ T cells than in CD4+ T cells (Chmill et al. Citation2010). Within the CD4+ T cell population, Th17 cells have the highest AhR expression (Veldhoen et al. Citation2008; Quintana et al. Citation2008).
Figure 3. Differentiation of CD4+ cells into different effector T cells; T helper 1 (Th1), T helper 2 (Th2), T helper 17 (Th17) or regulatory T (Treg) cells. Differentiation of these T cell subsets is mediated by the interaction of dendritic cells with naïve CD4+ T cells, the presence of specific cytokines and mediators and cell-type specific transcription factors. By secreting specific cytokines, effector T cells mediate their regulatory or activating properties as shown on the right. Adapted from Jetten (Citation2009).
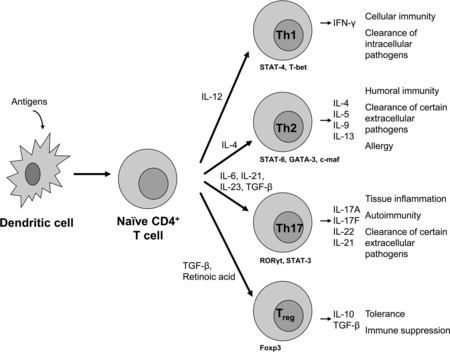
2.2.1. Aryl hydrocarbon receptor and thymic T cells
In the thymus, T cells develop from CD4−CD8− (double-negative) cells to CD4+CD8+ (double-positive) cells before differentiation into either CD4+, including CD4+CD25+Foxp3+ Treg cells, or CD8+ single-positive cells. For decades, it is known that AhR activation by TCDD results in thymus atrophy (Camacho et al. Citation2005; Simanainen et al. Citation2002). AhR activation by TCDD alters thymic selection by affecting thymic precursor T cells, e.g. by increasing percentages of CD4−CD8−, CD4+CD8− and CD4−CD8+ cells and by decreasing percentages of CD4+CD8+ cells (Temchura et al. Citation2005; Nohara et al. Citation2005). Apoptosis of precursor T cells via binding of FasL to Fas is proposed as one of the underlying mechanisms for TCDD-induced thymic atrophy (Camacho et al. Citation2005). AhR activation by TCDD induces up regulation of FasL via NF-кB on stromal cells, resulting in increased apoptosis of thymic T cells (Camacho et al. Citation2005). The epithelium of the thymic stroma is involved in negative and positive selection of thymocytes. Conversely, epithelial cells need the presence of thymocytes to maintain their integrity. TCDD exposure disturbs the cortical and the medullary epithelium, thereby contributing to thymic atrophy and disturbing thymocyte differentiation (De Waal et al. Citation1997). Next to apoptosis of precursor T cells, reduced thymic seeding could be another explanation for TCDD-induced thymic atrophy, because TCDD treatment has been shown to affect thymocyte precursors from the bone marrow (Fine et al. Citation1990). Remarkably, effects of AhR activation on thymic CD4+CD25+Foxp3+ Treg cells have not been studied yet.
2.2.2. General effects of aryl hydrocarbon receptor activation on peripheral CD4+ T cells
Besides suppressing T cell development in the thymus, AhR activation by TCDD can also affect mature T cells in the periphery resulting in suppression of immune responses. In vivo TCDD treatment suppresses survival, activation, differentiation, proliferation and cytokine production of CD4+ T cells (Shepherd et al. Citation2000; Dearstyne & Kerkvliet Citation2002; Nohara et al. Citation2002; Ito et al. Citation2002; Funatake et al. Citation2004). Similar to thymic precursor T cells, there is evidence that TCDD suppresses CD4+ T cell mediated immune responses via Fas/FasL. AhR activation induces Fas via activation of the NF-кB pathway and down regulates the cellular FLICE inhibitory protein (c-FLIP), an inhibitor of apoptosis (Dearstyne & Kerkvliet Citation2002; Funatake et al. Citation2004; Singh et al. Citation2008). Next to these general suppressive effects of AhR activation on T cells, AhR activation can skew T cell responses by affecting the balance between Th1 and Th2 cells, and Th17 and Treg cells.
2.2.3. Aryl hydrocarbon receptor and Th1/Th2 cell balance
Th1 cells secrete IFN-γ and are important for mediating cellular immunity and the clearance of intracellular pathogens. Th2 cells secrete IL-4, IL-5, IL-9 and IL-13 and are involved in humoral immunity, allergy and the clearance of extracellular pathogens (Figure ) (Romagnani Citation2006; Jetten Citation2009).
At subcellular level, development of Th cells is regulated by transcription factors, for Th1 cells signal transducer and activator of transcription-4 (STAT-4) and T-bet. These transcription factors are usually activated when IL-12 is produced by DCs. In contrast, Th2 cell development is regulated by STAT-6, GATA-3 and c-maf and these transcription factors are usually activated in the presence of IL-4.
The transcription factors controlling Th1 or Th2 cell differentiation are antagonistic and conceivably Th1 and Th2 cell development are mutually regulated. Furthermore, the presence of thymic stromal lymphopoietin (TSLP), IL-25 and IL-33 from non-lymphoid sources (e.g. epithelial cells, mast cells, basophils and eosinophils) are important for Th2 cell differentiation (Oliphant et al. Citation2011). Manipulation of the Th1/Th2 balance can be beneficial for food allergic responses, because an enhanced Th1 response may suppress food allergic responses (Lee et al. Citation2001).
AhR activation impairs Th2-mediated immune responses by suppressing Th2-related cytokines and antigen-specific antibody production by B cells (Funatake et al. Citation2004). Concomitantly, AhR activation increases antigen-induced IFN-γ production (Nohara et al. Citation2002; Fujimaki et al. Citation2002; Negishi et al. Citation2005). Interestingly, M50354, a transient but full AhR agonist, skews the differentiation of naïve T cells towards Th1 cells by inhibiting GATA-3 expression in T cells (Kato et al. Citation2003; Negishi et al. Citation2005; Morales et al. Citation2008). This indicates that the AhR may play a significant role in suppressing food allergic responses by affecting the balance of Th1 and Th2 cells.
2.2.4. Aryl hydrocarbon receptor and Treg/Th17 cell balance
Next to Th3 regulatory T cells and T regulatory 1 cells, there exist CD4+CD25+Foxp3+ Treg cells. There are two types of CD4+CD25+Foxp3+ Treg cells, natural and inducible CD4+CD25+Foxp3+ Treg cells. Foxp3 expression is essential for Treg cell differentiation in the thymus and periphery and their suppressor function (Josefowicz et al. Citation2012). Natural CD4+CD25+Foxp3+Treg cells develop in the thymus during the course of positive and negative selection like other T cells. Their development is influenced by co-stimulatory molecules, cytokines, T cell receptor (TCR) and antigen affinity, and the location and context of encountering self-antigen within the thymus (Workman et al. Citation2009; Bettini & Vignali Citation2010; Wirnsberger et al. Citation2011). Inducible CD4+CD25+Foxp3+ Treg cells develop from naïve CD4+ T cells in the periphery upon strong TCR stimulation in the presence of high amounts of TGF-β, IL-2 and/or retinoic acid (Josefowicz et al. Citation2012). Foxp3+ Treg cells secrete IL-10 and TGF-β and these cells are important for the induction of oral tolerance and the suppression of immune-mediated disorders, including food allergy (Figure ) (Van Wijk et al. Citation2007; Josefowicz et al. Citation2012).
Th17 cells develop in the periphery in the presence of low levels of TGF-β, IL-23 and IL-21 or IL-6 (Chen et al. Citation2011). Th17 cells are characterized by retinoic acid-related orphan receptor (ROR)γt and STAT-3 and secrete mainly IL-17A, IL-17F, IL-21 and IL-22. Th17 cells are involved in autoimmunity and tissue inflammation by expanding and recruiting innate immune cells such as neutrophils (Yu & Gaffen Citation2008; Chen et al. Citation2011). Th17 cells may also play a role in the pathogenesis of various allergic diseases, including allergic dermatitis and asthma by recruiting neutrophils. However, whether IL-17 or Th17 cells play a role in food allergy is not clear yet (Oboki et al. Citation2008) (Figure ).
Development of Foxp3+ Treg cells and Th17 cells can be reciprocally regulated, e.g. low concentrations of TGF-β together with IL-6 and IL-21 favors Th17 differentiation, whereas high concentrations of TGF-β induces Foxp3+ Treg cell differentiation (Jetten Citation2009; Chen et al. Citation2011). However, development of Th17 cells in the presence of IL-6 and IL-23, but in the absence of TGF-β, has also been reported (Ghoreschi et al. Citation2010).
Interestingly, the AhR is involved in peripheral differentiation of both Th17 and Treg cells. In various disease models, e.g. colitis (Singh et al. Citation2011; Benson & Shepherd Citation2011b), various autoimmune-mediated diseases (Quintana et al. Citation2008; Zhang et al. Citation2010; Kerkvliet et al. Citation2009; Quintana et al. Citation2010), graft versus host disease (Hauben et al. Citation2008) and viral immunopathology (Veiga-Parga et al. Citation2011) AhR activation by TCDD, the water-soluble derivate of VAF347 (VAG539) or ITE suppresses these immune-mediated disorders by increasing the percentage of Foxp3+ Treg cells (Table ). In contrast, AhR activation by FICZ enhances disease by increasing the percentage of Th17 cells in an experimental autoimmune encephalomyelitis (EAE) model (Veldhoen et al. Citation2008; Quintana et al. Citation2008) (Table ). These divergent effects of AhR activation on Foxp3+ Treg and Th17 cell development may result from the presence or absence of IL-6 and TGF-β during AhR activation, or from effects of the AhR ligand itself (Quintana et al. Citation2008; Jetten Citation2009; Chen et al. Citation2011).
Table 2. Effects of AhR activation on Th17 and Treg cells in various disease models as described in text.
Furthermore, it appears that the AhR has an important regulatory function in the commitment of Th17 cells by negatively regulating STAT-1 activation in vitro. STAT1 activation, induced by IFN-γ, inhibits Th17 cell development (Kimura et al. Citation2008). In addition, induction of human Foxp3+ Treg cells in vitro is coordinated by AhR-mediated up regulation of the transcription factors Smad1 and Aiolos, which are important for the induction of Foxp3+ Treg cells (Gandhi et al. Citation2010). However, so far only relative effects of AhR activation on Treg cells and Th17 cells have been shown. Therefore, it is not clear whether their increased presence is a consequence of a decrease of other cells resulting in an increased percentage of Th17 and Treg cells. The finding by Veiga-Parga et al. that TCDD does not increase the actual numbers of Treg cells suggest the latter (Veiga-Parga et al. Citation2011) (Table ). In conclusion, AhR activation can suppress or enhance disease in various immune-mediated disease models via Treg and Th17 cells, probably dependent on the AhR ligand and the presence or absence of certain cytokines during AhR activation.
2.3. Aryl hydrocarbon receptor and B cells
Interaction of antigen with a mature naïve B cell, as well as interactions with T cells, induces activation and differentiation of B-cell clones for the specific antigen. This occurs through several stages, each stage representing a change in the genome content at the antibody loci, ultimately resulting in the generation of plasma effector cells, memory B cells and generation of antibodies (humoral immunity).
TCDD suppresses humoral immune responses, characterized by suppression of IgM and IgG secretion and decreased B cell differentiation into Ig-secreting cells (Kerkvliet Citation2002). Sulentic et al. recently reviewed the direct effects of TCDD on B cells (Sulentic & Kaminski Citation2011). Briefly, TCDD affects B cells at multiple stages of maturation and differentiation and impairs B cell proliferation and antibody production. B cell activation induces marked up regulation of AhR and B cells are most susceptible to TCDD during activation and less susceptible as they progress towards terminal differentiation. Furthermore, TCDD probably affects AhR-dependent gene transcription in B cells and induces biochemical changes independent of AhR-mediated gene transcription, thereby affecting regulators important for the B cell differentiation program (Sulentic & Kaminski Citation2011). Next to these direct suppressive effects on B cells, AhR activation can also indirectly suppress B cell function and activation by suppressing Th cells and Th-related cytokines as mentioned before.
2.4. Aryl hydrocarbon receptor and the mucosal immune system
The intestine is the largest contact surface between the body and the external environment, which is not only rich in commensals, nutrients and fluids, but also in pathogens. Proper functioning of the intestinal immune system requires a coordinated balance of tolerance and immunity and is crucial for protection against infections and intestinal diseases, for instance inflammatory bowel disease and food allergies (MacDonald & Monteleone Citation2005; Karin et al. Citation2006; Izcue et al. Citation2009).
Recently, it was proposed that AhR activation by dietary ligands plays a major role in the postnatal expansion of RORγt innate lymphoid cells (ILCs) and is necessary to maintain intraepithelial lymphocytes (IELs) in the intestine (Li et al. Citation2011; Kiss et al. Citation2011). By producing IL-22, RORγt ILC maintains the epithelial barrier, which is required for protection against intestinal infections and food allergy (Chahine & Bahna Citation2010; Kiss et al. Citation2011). IELs are important as a first line of defense, epithelial barrier organization, wound repair and food allergic sensitization (Li et al. Citation2011; Bol-Schoenmakers et al. Citation2011b). The importance of the AhR in the intestinal immune system is illustrated by the fact that AhR activation by FICZ or β-NF ameliorates TNBS- and DSS-induced colitis by suppressing inflammatory cytokines (Furumatsu et al. Citation2011) and by enhancing production of IL-22 (Furumatsu et al. Citation2011; Monteleone et al. Citation2011). In addition, TCDD treatment of mice before induction of TNBS-induced colitis suppresses colonic inflammation and this was accompanied by an increase of Foxp3+ Treg cells (Benson & Shepherd Citation2011b).
Furthermore, as mentioned before, mucosal CD103+ DCs can also induce Foxp3+ Treg cells in a TGF-β and retinoic acid dependent manner. These inducible Foxp3+ Treg cells are thought to play a major role in the induction of tolerance (Coombes et al. Citation2007; Sun et al. Citation2007; Peron et al. Citation2009). However, it is not clear yet whether there is a role for the AhR in the induction of Foxp3+ Treg cells by CD103+ DCs in the intestine.
3. Food allergy
Food allergy is defined as an ‘adverse health effect arising from a specific immune response that occurs reproducibly on exposure to a given food’ (Boyce et al. Citation2010). Currently, food allergy in humans is a major health problem, with an estimated prevalence of about 5% in young children and 3–4% in adults and the prevalence is increasing (Sicherer & Sampson Citation2010; Burks et al. Citation2012). The major food allergens in humans are milk, egg, peanut, three nuts, shellfish, fish, wheat and soy (Sicherer & Sampson Citation2010). Exposure to these food allergens causes only mild allergic reactions in most cases. However, in severe cases anaphylaxis can be induced, mainly in people allergic to peanut and tree nuts (Koplin et al. Citation2011).
Adverse reactions to food can also occur in dogs and cats. However, it is not clear whether in dogs and cats adverse reactions to food antigens are caused by an immunological mechanism (e.g. food allergy) or by a non-immunological mechanism (e.g. intolerance) (Mandigers & German Citation2010). Therefore, it is difficult to estimate the prevalence of food allergy in these species, but it has been suggested that approximately 5% of all skin diseases in dogs is caused by a food allergic reaction (Carlotti Citation1990). Beef, lamb, diary, chicken, pork, wheat, soybean and eggs are the major allergens causing adverse reactions to food in dogs and cats (Verlinden et al. Citation2006).
3.1. Risk factors
Allergies are the result of a complex interplay between genetic predisposition and environmental factors. The development of food allergy is determined by genetic predisposition to allergies, but the specific genetic loci that may modulate individual risk of food allergy remain to be identified (Hong et al. Citation2009). Furthermore, reported effects of breastfeeding in humans on food allergy are contradicting, as some report no protective effects and others do (Muraro et al. Citation2004; Kramer Citation2011). However, delayed introduction of solid foods in infants is associated with higher risk of food allergic diseases (Cochrane et al. Citation2009). In addition, early colonization of the intestine by appropriate microbiotica is important for the development of a healthy immune system, including the development of oral tolerance to food antigens (Cochrane et al. Citation2009). Other risk factors for the development of food allergy are the dose, the route of exposure (e.g. allergic sensitization might occur via inflamed skin), concomitant exposure to drugs and the biochemical nature of a food allergen (Cochrane et al. Citation2009; Bol-Schoenmakers et al. Citation2011a). Furthermore, the acid content of the stomach plays a role in allergic sensitization, because an increased pH in the stomach is associated with increased allergic sensitization (Untersmayr et al. Citation2003; Schöll et al. Citation2005). Nevertheless, it is not yet elucidated how all these factors exactly play a role in the development of food allergy (Cochrane et al. Citation2009).
3.2. Allergic sensitization versus oral tolerance: mechanisms
After ingestion, most food proteins are to a large extent digested by gastric acids and enzymes in the stomach and intestine. The remaining food proteins and peptides are subsequently transferred from the lumen to the mucosa via gut epithelial cells, by specialized M cells present in Peyer's Patches or by direct sampling of mucosal dendritic cells. In the mucosa, DCs process these proteins and peptides, move to T cell areas and present them on major histocompatibility complex (MHC) II where they can interact with naïve T cells (Mowat Citation2003). The presence of costimulatory molecules is important in determining the subsequent immune response: interaction of CD28 present on T cells with CD80 and CD86 present on DCs induces T cell activation, whereas interaction of CD80 and CD86 with CTLA-4 present on T cells down regulates T cell activation (Krummel & Allison Citation1995; Vandenborre et al. Citation1999). CTLA-4 signaling has been shown to be important for the regulation of the intensity of food allergic responses, because blocking of CTLA-4 during the induction of food allergy increases food allergic responses (Van Wijk et al. Citation2005).
In normal individuals, presentation of processed proteins and peptides by DCs to naïve T cells will lead to the induction of oral tolerance, a state of active inhibition of immune responses to antigens encountered in the intestine. Two types of oral tolerance are known: high-dose tolerance and low-dose tolerance. A high dose of protein results in tolerance induced by T cell clonal anergy and apoptosis, whereas a low dose of protein results in active suppression of the immune reaction via induction of regulatory T (Treg) cells (Th3, Tr1, CD4+CD25+, CD4+CD25+Foxp3+) (Figure ).
Figure 4. Schematic representation of an IgE-mediated allergic reaction to food allergens. In normal individuals, presentation of processed proteins and peptides by DCs to naïve T cells will lead to the induction of high-dose tolerance (induced by T cell clonal anergy and apoptosis) or low-dose tolerance (active suppression of the immune reaction via induction of Treg cells). However, in certain individuals, presentation of a food protein by DCs on MHCII may result in the induction and activation of Th2 cells. By secreting cytokines (e.g. IL-4, IL-5, IL-13), these Th2 cells stimulate B cells to produce allergen-specific IgE which is distributed systemically. This allergen-specific IgE binds to high affinity IgE receptors (FcϵRI) present primarily on mast cells and basophils. This process is known as allergic sensitization. Re-exposure (challenge) to the food antigen results in crosslinking of the IgE bound on FcϵRI, which provokes degranulation of mast cells and basophils and the release of mediators (such as histamines, cytokines, leukotrines, prostaglandins and platelet-activating factor). This leads to a variety of clinical effects.
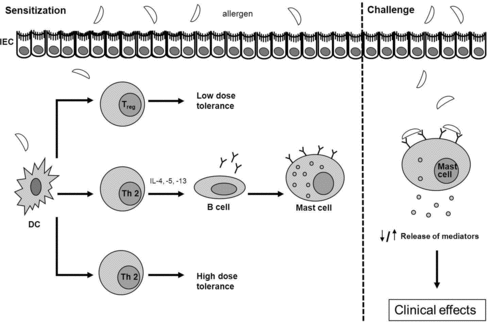
However, in certain cases, caused by factors mentioned before, presentation of a food protein by DCs on MHCII may result in the induction and activation of Th2 cells. By secreting cytokines (e.g. interleukin [IL]-4, IL-5, IL-13), these Th2 cells stimulate B cells to produce allergen-specific IgE which is distributed systemically. This allergen-specific IgE binds to high affinity IgE receptors (FcϵRI) present primarily on mast cells and basophils. This process is known as allergic sensitization. Re-exposure (challenge) to the food antigen results in crosslinking of the IgE bound on FcϵRI, which provokes degranulation of mast cells and basophils and the release of mediators (such as histamines, cytokines, leukotrines, prostaglandins and platelet-activating factor). The release of mediators may lead to a variety of symptoms occurring within minutes to hours affecting the skin (eczema, angioedema and urticaria), gastrointestinal tract (nausea, vomiting and diarrhea) and airways (cough and wheeze). In severe cases, anaphylaxis is induced. Anaphylaxis can include any of these symptoms and additionally may include cardiovascular symptoms (Figure ) (Burks Citation2008; Boyce et al. Citation2010; Perrier & Corthésy Citation2011; Pabst & Mowat Citation2012).
3.3. Foxp3+ regulatory T cells and food allergy
The importance of Foxp3+ Treg cells in food allergic responses is demonstrated in several ways. Individuals that suffer from the immune dysregulation polyendocrinopathy enteropathy X-linked (IPEX) syndrome do not have functional Foxp3+ Treg cells and some of these people suffer from severe food allergy (Torgerson & Ochs Citation2007). Furthermore, Foxp3 and IL-10 gene expression (measured in nucleated cells from peripheral blood) in children with IgE mediated food allergy is lower compared to healthy children and children that acquired tolerance to food express more Foxp3 than children with food allergy (Krogulska et al. Citation2011). Thus, Foxp3+ Treg cells appear very important for the induction of active immune suppression, thereby controlling food allergic responses. As in humans, CD4+CD25+Foxp3+ Treg cells play also an important regulatory role in the functioning of the immune system in dogs and cats including the control of food allergic responses (Garden et al. Citation2011).
3.4. Therapy
Currently, the primary recommendation for food allergy is strict avoidance of the causal food. Antihistamines and corticosteroids are used to treat acute allergic symptoms and in anaphylaxis epinephrine is used as first line treatment. However, these drugs only ameliorate symptoms and do not stop or cure progression of disease (Sicherer & Sampson Citation2010; Burks et al. Citation2012). A potentially curative treatment for (food) allergy is allergen-specific immunotherapy (SIT). SIT is already used for more than 100 years as a desensitizing therapy (Noon Citation1911) and is presently successfully used to treat allergic rhinitis, asthma and wasp and bee venom hypersensitivity (Fitzhugh & Lockey Citation2011). The aim of SIT is to induce peripheral T cell tolerance and to increase the thresholds for IgE mediated mast cell and basophil activation (Fujita et al. Citation2012). The high success rate of SIT (varying from 80 to 90%, depending on the antigen) and the interruption of allergic sensitization is promising. However, disadvantages are the long duration of the treatment (3–5 years), the amount of allergen injections needed (about 80) and allergic side effects (Kündig & Bachmann Citation2010). Key mechanisms of successful SIT are the induction of regulatory T cells (CD4+CD25+Foxp3+ and Tr1 cells) and an increase of IgG4 (in humans) that may capture the allergen before reaching effector cell-bound IgE (Fujita et al. Citation2012). Subcutanous (SCIT) or sublingual (SLIT) immunotherapy are the most widely accepted routes of immunotherapy administration. The efficacy of oral immunotherapy (OIT) on long-term tolerance is not clear yet (Cox et al. Citation2013).
In food allergy, especially peanut allergy, immunotherapy has not been successfully applied yet, because of the unacceptable high risk for side effects, including anaphylactic reactions (Scurlock & Jones Citation2010). Therefore, immune modulators are used to lower these side effects and to improve safety and efficacy of immunotherapy. For example, Nigella sativa seed supplementation may improve immunotherapy by increasing the activity of polymorphonuclear leukocytes (Işik et al. Citation2010). In addition, probiotics, oligomannose-coated liposomes and vitamin D3 have been shown to improve immunotherapy by increasing Th1 and Treg cells and decreasing Th2 cells (Ma et al. Citation2010; Moussu et al. Citation2012; Kawakita et al. Citation2012; Grundström et al. Citation2012). Moreover, activation of the innate immune system by toll-like-receptor (TLR) agonists, including TLR-4 agonist MPL and a phosphorothioate oligodeoxyribonucleotide immunostimulatory sequence of DNA containing a CpG motif, appear to improve immunological and clinical responses in allergic patients (Drachenberg et al. Citation2001; Creticos et al. Citation2006). Furthermore, omalizumab, a humanized, monoclonal anti-IgE antibody that binds to circulating IgE molecules, improves safety and efficacy of immunotherapy in men by interrupting the allergic cascade downstream the IgE production of B cells (Kopp Citation2011). Dogs with atopic dermatitis receiving allergen-specific immunotherapy have decreased levels of IgE and increased levels of CD4+Foxp3+ Treg and IL-10 in their blood (Keppel et al. Citation2008). This indicates that the immunological mechanism of immunotherapy is quite similar in dogs and in men, suggesting that immunotherapy could be used to treat dogs suffering from food allergy.
Next to SIT, treatments involving activation of the intestinal innate immune system via TLRs might be effective in suppressing food allergy by skewing immune responses from Th2 towards Th1 (Vollmer & Krieg Citation2009). For instance, a specific herbal formula reduces peanut-induced anaphylaxis via IFN-γ producing CD8+ T cells (Srivastava et al. Citation2009). In addition, dietary intervention by the use of short-chain galacto-oligosaccharides and long-chain fructo-oligosaccharides (GOS/FOS) in dietary products suppresses food allergic responses via CD25+ Treg cells and an increase of Th1 responses (Vos et al. Citation2006; Schouten et al. Citation2010). In infants, this formula decreases plasma levels of total IgE, IgG1, IgG2 and IgG3, but not IgG4 (Van Hoffen et al. Citation2009).
Furthermore, retinoic acid might represent a potential new target to treat food allergy, because retinoic acid imprints T and B cells with gut-homing signatures and induces, in the presence of TGF-β, Foxp3+ Treg cells via CD103+ DCs (Iwata Citation2009; Mucida et al. Citation2009). In addition, high levels of retinoic acid in the intestine can promote class switching of B cells to IgA (Mora & Von Andrian Citation2009). IgA binds and neutralizes food proteins that retained their immunogenic epitopes after digestion (Kim & Sampson Citation2012).
Finally, vitamin D deficiency has been epidemiologically linked to an increase of food allergy (Vassallo & Camargo Citation2010). Vitamin D acquired through skin exposure to sunlight or via the diet, plays an important role in the immune system. The vitamin D receptor is expressed by T cells, activated B cells, monocytes, DCs and epithelial cells. Activation of this receptor results in regulation of both innate and adaptive immune responses. Therefore, it has been proposed that early correction of vitamin D deficiency might decrease food allergy by enhancing proper functioning of the (intestinal) immune system (Vassallo & Camargo Citation2010).
However, all these suggested treatments or targets of treatments are still in the experimental or preclinical phase of research.
4. The aryl hydrocarbon receptor: a target to interfere in food allergic responses?
Activation of the AhR by dioxins has been shown to suppress a variety of immune responses by affecting various cells of the immune system, including dendritic cells, regulatory T cells and the Th1/Th2 cell balance. As discussed before these cells play a major role in food allergy, because they are involved in the induction of either tolerance or allergic sensitization. Recently, it has been shown that activation of the AhR by dioxin-like compounds suppresses allergic sensitization by suppressing the absolute number of precursor and effector T cells, by preserving CD4+CD25+Foxp3+ Treg cells and by affecting DCs and their interaction with effector T cells (Schulz et al. Citation2012a; Schulz et al. Citation2012b; Schulz et al. Citation2013).
Importantly, the greatest source of exposure to AhR ligands comes from our diet (Denison & Nagy Citation2003). Daily products, like potatoes, cruciferous vegetables, bread, hamburgers and grape fruit induce AhR activation (de Waard et al. Citation2008). Because most natural AhR ligands are metabolized relatively fast after ingestion and have various degrees of AhR binding affinity, it is difficult to estimate the exposure of individuals to AhR ligands. Nevertheless, these dietary AhR ligands might influence the onset of intestinal diseases such as food allergy.
To conclude, no approved curative treatments for food allergy are currently available. Future research should elucidate whether and how AhR activation can be used to interfere in food allergic responses in humans and in animals. This may lead to new prevention strategies and therapeutic possibilities for food allergy.
References
- Abel J, Haarmann-Stemmann T. 2010. An introduction to the molecular basics of aryl hydrocarbon receptor biology. Biol Chem. 391(11): 1235–1248.
- Bankoti J, Burnett A, Navarro S, Miller AK, Rase B, Shepherd DM. 2010. Effects of TCDD on the fate of naïve dendritic cells. Toxicol Sci. 115(2): 422–434.
- Benson JM, Shepherd DM. 2011a. Dietary ligands of the aryl hydrocarbon receptor induce anti-inflammatory and immunoregulatory effects on murine dendritic cells. Toxicol Sci. 124(2): 327–338.
- Benson JM, Shepherd DM. 2011b. Aryl hydrocarbon receptor activation by TCDD reduces inflammation associated with Crohn's disease. Toxicol Sci. 120(1): 68–78.
- Bettini ML, Vignali DAA. 2010. Development of thymically derived natural regulatory T cells. Ann N Y Acad Sci. 1183: 1–12.
- Bol-Schoenmakers M, Bleumink R, Marcondes Rezende M, Mouser E, Hassing I, Ludwig I, Smit JJ, Pieters RHH. 2011a. Diclofenac enhances allergic responses in a mouse peanut allergy model. Clin Exp Allergy. 41(3): 424–433.
- Bol-Schoenmakers M, Marcondes Rezende M, Bleumink R, Boon L, Man S, Hassing I, Fiechter D, Pieters RHH, Smit JJ. 2011b. Regulation by intestinal γδ T cells during establishment of food allergic sensitization in mice. Allergy. 66(3): 331–340.
- Boyce JA, Assa'ad A, Burks AW, Jones SM, Sampson HA, Wood RA, Plaut M, Cooper SF, Fenton MJ, Arshad SH, et al. 2010. Guidelines for the diagnosis and management of food allergy in the United States: summary of the NIAID-sponsored expert panel report. J Allergy Clin Immunol. 126(6): 1105–1118.
- Burks AW. 2008. Peanut allergy. Lancet. 371(9623): 1538–1546.
- Burks AW, Tang M, Sicherer S, Muraro A, Eigenmann PA, Ebisawa M, Fiocchi A, Chiang W, Beyer K, Wood R, et al. 2012. ICON: food allergy. J Allergy Clin Immunol. 129(4): 906–920.
- Camacho IA, Singh N, Hegde VL, Nagarkatti M, Nagarkatti PS. 2005. Treatment of mice with 2,3,7,8-tetrachlorodibenzo-p-dioxin leads to aryl hydrocarbon receptor-dependent nuclear translocation of NF-κB and expression of Fas ligand in thymic stromal cells and consequent apoptosis in T cells. J Immunol. 175(1): 90–103.
- Carlotti DN. 1990. Food allergy in dogs and cats: a review and report of 43 cases. Vet Dermatol. 1(2): 55–62.
- Casado FL, Singh KP, Gasiewicz TA. 2010. The aryl hydrocarbon receptor: regulation of hematopoiesis and involvement in the progression of blood diseases. Blood Cells Mol Dis. 44(4): 199–206.
- Chahine BG, Bahna SL. 2010. The role of the gut mucosal immunity in the development of tolerance versus development of allergy to food. Curr Opin Allergy Clin Immunol. 10(4): 394–399.
- Chen Z, Lin F, Gao Y, Li Z, Zhang J, Xing Y, Deng Z, Yao Z, Tsun A, Li B. 2011. FOXP3 and RORγt: transcriptional regulation of Treg and Th17. Int Immunopharmacol. 11(5): 536–542.
- Chmill S, Kadow S, Winter M, Weighardt H, Esser C. 2010. 2,3,7,8-Tetrachlorodibenzo-p-dioxin impairs stable establishment of oral tolerance in mice. Toxicol Sci. 118(1): 98–107.
- Cochrane S, Beyer K, Clausen M, Wjst M, Hiller R, Nicoletti C, Szepfalusi Z, Savelkoul H, Breiteneder H, Manios Y, et al. 2009. Factors influencing the incidence and prevalence of food allergy. Allergy. 64(9): 1246–1255.
- Coombes JL, Powrie F. 2008. Dendritic cells in intestinal immune regulation. Nat Rev Immunol. 8(6): 435–446.
- Coombes JL, Siddiqui KRR, Arancibia-Cárcamo CV, Hall J, Sun C-M, Belkaid Y, Powrie F. 2007. A functionally specialized population of mucosal CD103+ DCs induces Foxp3+ regulatory T cells via a TGF-β -and retinoic acid-dependent mechanism. J Exp Med. 204(8): 1757–1764.
- Cox L, Compalati E, Kundig T, Larche M. 2013. New directions in immunotherapy. Curr Allergy Asthma Rep. 13(2) 178–195.
- Creticos PS, Schroeder JT, Hamilton RG, Balcer-Whaley SL, Khattignavong AP, Lindblad R, Li H, Coffman R, Seyfert V, Eiden JJ, Broide D. 2006. Immunotherapy with a ragweed-toll-like receptor 9 agonist vaccine for allergic rhinitis. N Engl J Med. 355(14): 1445–1455.
- Dearstyne EA, Kerkvliet NI. 2002. Mechanism of 2,3,7,8-tetrachlorodibenzo-p-dioxin (TCDD)-induced decrease in anti-CD3-activated CD4+ T cells: the roles of apoptosis, Fas, and TNF. Toxicology. 170(1): 139–151.
- Denison MS, Heath-Pagliuso S. 1998. The Ah receptor: a regulator of the biochemical and toxicological actions of structurally diverse chemicals. Bull Environ Contam Toxicol. 61(5): 557–568.
- Denison MS, Nagy SR. 2003. Activation of the aryl hydrocarbon receptor by structurally diverse exogenous and endogenous chemicals. Annu Rev Pharmacol Toxicol. 43: 309–334.
- Denison MS, Soshilov AA, He G, Degroot DE, Zhao B. 2011. Exactly the same but different: promiscuity and diversity in the molecular mechanisms of action of the aryl hydrocarbon (dioxin) receptor. Toxicol Sci. 124(1): 1–22.
- De Waal EJ, Schuurman H-J, Van Loveren H, Vos JG. 1997. Differential effects of 2,3,7,8-tetrachlorodibenzo-p-dioxin, bis(tri-n-butyltin)oxide and cyclosporine on thymus histophysiology. Crit Rev Toxicol. 27(4): 381–430.
- De Waard WJ, Aarts JM, Peijnenburg AC, De Kok TM, Van Schooten FJ, Hoogenboom LA. 2008. Ah receptor agonist activity in frequently consumed food items. Food Addit Contam Part A Chem Anal Control Expo Risk Assess. 25(6): 779–787.
- Drachenberg KJ, Wheeler AW, Stuebner P, Horak F. 2001. A well-tolerated grass pollen-specific allergy vaccine containing a novel adjuvant, monophosphoryl lipid A, reduces allergic symptoms after only four preseasonal injections. Allergy. 56(6): 498–505.
- Esser C. 2009. The immune phenotype of AhR null mouse mutants: not a simple mirror of xenobiotic receptor over-activation. Biochem Pharmacol. 77(4): 597–607.
- Fernandez-Salguero PM, Ward JM, Sundberg JP, Gonzalez FJ. 1997. Lesions of aryl-hydrocarbon receptor-deficient mice. Vet Pathol. 34(6): 605–614.
- Fine JS, Silverstone AE, Gasiewicz TA. 1990. Impairment of prothymocyte activity by 2,3,7,8-tetrachlorodibenzo-p- dioxin. J Immunol. 144(4): 1169–1176.
- Fitzhugh DJ, Lockey RF. 2011. Allergen immunotherapy: a history of the first 100 years. Curr Opin Allergy Clin Immunol. 11(6): 554–559.
- Frericks M, Meissner M, Esser C. 2007. Microarray analysis of the AHR system: tissue-specific flexibility in signal and target genes. Toxicol Appl Pharmacol. 220(3): 320–332.
- Fujimaki H, Nohara K, Kobayashi T, Suzuki K, Eguchi-Kasai K, Tsukumo S, Kijima M, Tohyama C. 2002. Effect of a single oral dose of 2,3,7,8-tetrachlorodibenzo-p-dioxin on immune function in male NC/Nga mice. Toxicol Sci. 66(1): 117–124.
- Fujita H, Soyka MB, Akdis M, Akdis CA. 2012. Mechanisms of allergen-specific immunotherapy. Clin Transl Allergy. 2(1): 1–8.
- Funatake CJ, Dearstyne EA, Steppan LB, Shepherd DM, Spanjaard ES, Marshak-Rothstein A, Kerkvliet NI. 2004. Early consequences of 2,3,7,8-Tetrachlorodibenzo-p-dioxin exposure on the activation and survival of antigen-specific T cells. Toxicol Sci. 82(1): 129–142.
- Furumatsu K, Nishiumi S, Kawano Y, Ooi M, Yoshie T, Shiomi Y, Kutsumi H, Ashida H, Fujii-Kuriyama Y, Azuma T, Yoshida M. 2011. A role of the aryl hydrocarbon receptor in attenuation of colitis. Dig Dis Sci. 56(9): 2532–2544.
- Gandhi R, Kumar D, Burns EJ, Nadeau M, Dake B, Laroni A, Kozoriz D, Weiner HL, Quintana FJ. 2010. Activation of the aryl hydrocarbon receptor induces human type 1 regulatory T cell-like and Foxp3+ regulatory T cells. Nat Immunol. 11(9): 846–853.
- Garden OA, Pinheiro D, Cunningham F. 2011. All creatures great and small: regulatory T cells in mice, humans, dogs and other domestic species. Int Immunopharmacol. 11(5): 576–588.
- Ghoreschi K, Laurence A, Yang X-P, Tato CM, McGeachy MJ, Konkel JE, Ramos HL, Wei L, Davidson TS, Bouladoux N, et al. 2010. Generation of pathogenic TH17 cells in the absence of TGF-β 2 signalling. Nature. 467(7318): 967–971.
- Gonzalez FJ, Fernandez-Salguero P. 1998. The aryl hydrocarbon receptor: studies using the AHR-null mice. Drug Metab Dispos. 26(12): 1194–1198.
- Grundström J, Neimert-Andersson T, Kemi C, Nilsson OB, Saarne T, Andersson M, Van Hage M, Gafvelin G. 2012. Covalent coupling of vitamin D3 to the major cat allergen Fel d 1 improves the effects of allergen-specific immunotherapy in a mouse model for cat allergy. Int Arch Allergy Immunol. 157(2): 136–146.
- Gu Y-Z, Hogenesch JB, Bradfield CA. 2000. The PAS superfamily: sensors of environmental and developmental signals. Annu Rev Pharmacol Toxicol. 40: 519–561.
- Guilliams M, Henri S, Tamoutounour S, Ardouin L, Schwartz-Cornil I, Dalod M, Malissen B. 2010. From skin dendritic cells to a simplified classification of human and mouse dendritic cell subsets. Eur J Immunol. 40(8): 2089–2094.
- Hauben E, Gregori S, Draghici E, Migliavacca B, Olivieri S, Woisetschlager M, Roncarolo MG. 2008. Activation of the aryl hydrocarbon receptor promotes allograft-specific tolerance through direct and dendritic cell-mediated effects on regulatory T cells. Blood. 112(4): 1214–1222.
- Hong X, Tsai H-J, Wang X. 2009. Genetics of food allergy. Curr Opin Pediatr. 21(6): 770–776.
- Işik H, Çevikbaş A, Gürer ÜS, Kiran B, Üresin Y, Rayaman P, Rayaman E, Gürbüz B, Büyüköztürk S. 2010. Potential adjuvant effects of Nigella sativa seeds to improve specific immunotherapy in allergic rhinitis patients. Med Princ Pract. 19(3): 206–211.
- Ito T, Inouye K, Fujimaki H, Tohyama C, Nohara K. 2002. Mechanism of TCDD-induced suppression of antibody production: effect of T cell-derived cytokine production in the primary immune reaction of mice. Toxicol Sci. 70(1): 46–54.
- Iwata M. 2009. Retinoic acid production by intestinal dendritic cells and its role in T-cell trafficking. Semin Immunol. 21(1): 8–13.
- Izcue A, Coombes JL, Powrie F. 2009. Regulatory lymphocytes and intestinal inflammation. Annu Rev Immunol. 27: 313–338.
- Jetten AM. 2009. Retinoid-related orphan receptors (RORs): critical roles in development, immunity, circadian rhythm, and cellular metabolism. Nucl Recept Signa. 7: 1–32.
- Jin G-B, Moore AJ, Head JL, Neumiller JJ, Lawrence BP. 2010. Aryl hydrocarbon receptor activation reduces dendritic cell function during influenza virus infection. Toxicol Sci. 116(2): 514–522.
- Josefowicz SZ, Lu L-F, Rudensky AY. 2012. Regulatory T cells: mechanisms of differentiation and function. Annu Rev Immunol. 30: 531–564.
- Kafafi SA, Afeefy HY, Ali AH, Said HK, Abd-Elazem IS, Kafafi AG. 1993. Affinities for the aryl hydrocarbon receptor, potencies as aryl hydrocarbon hydroxylase inducers and relative toxicities of polychlorinated biphenyls: a congener specific approach. Carcinogenesis. 14(10): 2063–2071.
- Karin M, Lawrence T, Nizet V. 2006. Innate immunity gone awry: linking microbial infections to chronic inflammation and cancer. Cell. 124(4): 823–835.
- Kato Y, Negishi T, Furusako S, Mizuguchi K, Mochizuki H. 2003. An orally active Th1/Th2 balance modulator, M50367, suppresses Th2 differentiation of naïve Th cell in vitro. Cell Immunol. 224(1): 29–37.
- Kawakita A, Shirasaki H, Yasutomi M, Tokuriki S, Mayumi M, Naiki H, Ohshima Y. 2012. Immunotherapy with oligomannose-coated liposomes ameliorates allergic symptoms in a murine food allergy model. Allergy. 67(3): 371–379.
- Keppel KE, Campbell KL, Zuckermann FA, Greeley EA, Schaeffer DJ, Husmann RJ. 2008. Quantitation of canine regulatory T cell populations, serum interleukin-10 and allergen-specific IgE concentrations in healthy control dogs and canine atopic dermatitis patients receiving allergen-specific immunotherapy. Vet Immunol Immunopathol. 123(3–4): 337–344.
- Kerkvliet NI. 2002. Recent advances in understanding the mechanisms of TCDD immunotoxicity. Int Immunopharmacol. 2(2–3): 277–291.
- Kerkvliet NI, Steppan LB, Vorachek W, Oda S, Farrer D, Wong CP, Pham D, Mourich DV. 2009. Activation of aryl hydrocarbon receptor by TCDD prevents diabetes in NOD mice and increases Foxp3+ T cells in pancreatic lymph nodes. Immunotherapy. 1(4): 539–547.
- Kim JS, Sampson HA. 2012. Food allergy: a glimpse into the inner workings of gut immunology. Curr Opin Gastroenterol. 28(2): 99–103.
- Kimura A, Naka T, Nohara K, Fujii-Kuriyama Y, Kishimoto T. 2008. Aryl hydrocarbon receptor regulates Stat1 activation and participates in the development of Th17 cells. Proc Natl Acad Sci. USA. 105(28): 9721–9726.
- Kiss EA, Vonarbourg C, Kopfmann S, Hobeika E, Finke D, Esser C, Diefenbach A. 2011. Natural aryl hydrocarbon receptor ligands control organogenesis of intestinal lymphoid follicles. Science. 334(6062): 1561–1565.
- Koplin JJ, Martin PE, Allen KJ. 2011. An update on epidemiology of anaphylaxis in children and adults. Curr Opin Allergy Clin Immunol. 11(5): 492–496.
- Kopp MV. 2011. Role of immunmodulators in allergen-specific immunotherapy. Allergy. 66(6): 792–797.
- Kramer MS. 2011. Breastfeeding and allergy: the evidence. Ann Nutr Metab. 59(1): 20–26.
- Krogulska A, Borowiec M, Polakowska E, Dynowski J, Młynarski W, Wasowska-Królikowska K. 2011. FOXP3, IL-10, and TGF-β genes expression in children with IgE-dependent food allergy. J Clin Immunol. 31(2): 205–215.
- Krummel MF, Allison JP. 1995. CD28 and CTLA-4 have opposing effects on the response of T cells to stimulation. J Exp Med. 182(2): 459–465.
- Kündig TM, Bachmann MF. 2010. Allergen-specific immunotherapy: regulatory T cells or allergen-specific IgG. Hum Vaccin. 6(8): 673–675.
- Lee JA, Hwang JA, Sung HN, Jeon CH, Gill BC, Youn HJ, Park JH. 2007. 2,3,7,8-Tetrachlorodibenzo-p-dioxin modulates functional differentiation of mouse bone marrow-derived dendritic cells: downregulation of RelB by 2,3,7,8-tetrachlorodibenzo-p-dioxin. Toxicol Lett. 173(1): 31–40.
- Lee S-Y, Huang C-K, Zhang T-F, Schofield BH, Burks AW, Bannon GA, Sampson HA, Li X-M. 2001. Oral administration of IL-12 suppresses anaphylactic reactions in a murine model of peanut hypersensitivity. Clin Immunol. 101(2): 220–228.
- Li Y, Innocentin S, Withers DR, Roberts NA, Gallagher AR, Grigorieva EF, Wilhelm C, Veldhoen M. 2011. Exogenous stimuli maintain intraepithelial lymphocytes via aryl hydrocarbon receptor activation. Cell. 147(3): 629–640.
- Ma, J.X., Xia JB, Cheng XM, Wang CZ. 2010. 1,25-dihydroxyvitamin D3 pretreatment enhances the efficacy of allergen immunotherapy in a mouse allergic asthma model. Chin Med J. 123(24): 3591–3596.
- MacDonald TT, Monteleone G. 2005. Immunity, inflammation, and allergy in the gut. Science. 307(5717): 1920–1925.
- Mandigers P, German AJ. 2010. Dietary hypersensitivity in cats and dogs. Tijdschr Diergeneesk. 135(19): 706–710.
- Mezrich JD, Fechner JH, Zhang X, Johnson BP, Burlingham WJ, Bradfield CA. 2010. An interaction between kynurenine and the aryl hydrocarbon receptor can generate regulatory T cells. J Immunol. 185(6): 3190–3198.
- Mitchell KA, Elferink CJ. 2009. Timing is everything: consequences of transient and sustained AhR activity. Biochem Pharmacol. 77(6): 947–956.
- Monteleone I, Rizzo A, Sarra M, Sica G, Sileri P, Biancone L, MacDonald TT, Pallone F, Monteleone G. 2011. Aryl hydrocarbon receptor-induced signals up-regulate IL-22 production and inhibit inflammation in the gastrointestinal tract. Gastroenterology. 141(1): 237–248.
- Mora JR, von Andrian UH. 2009. Role of retinoic acid in the imprinting of gut-homing IgA-secreting cells. Semin Immunol. 21(1): 28–35.
- Morales JL, Krzeminski J, Amin S, Perdew GH. 2008. Characterization of the antiallergic drugs 3-[2-(2-phenylethyl) benzoimidazole-4-yl]-3-hydroxypropanoic acid and ethyl 3-hydroxy-3-[2-(2-phenylethyl)benzoimidazol-4-yl]propanoate as full aryl hydrocarbon receptor agonists. Chem Res Toxicol. 21(2): 472–482.
- Moussu H, Van Overtvelt L, Horiot S, Tourdot S, Airouche S, Zuercher A, Holvoet S, Prioult G, Nutten S, Mercenier A, Mascarell L, et al. 2012. Bifidobacterium bifidum NCC 453 promotes tolerance induction in murine models of sublingual immunotherapy. Int Arch Allergy Immunol. 158(1): 35–42.
- Mowat AM. 2003. Anatomical basis of tolerance and immunity to intestinal antigens. Nat Rev Immun. 3(4): 331–341.
- Mucida D, Park Y, Cheroutre H. 2009. From the diet to the nucleus: vitamin A and TGF-β join efforts at the mucosal interface of the intestine. Semin Immunol. 21(1): 14–21.
- Muraro A, Dreborg S, Halken S, Høst A, Niggemann B, Aalberse R, Arshad SH, Von Berg A, Carlsen K-H, Duschén K, et al. 2004. Dietary prevention of allergic diseases in infants and small children. Part III: critical review of published peer-reviewed observational and interventional studies and final recommendations. Pediatr Allergy Immunol. 15(3): 291–307.
- Negishi T, Kato Y, Ooneda O, Mimura J, Takada T, Mochizuki H, Yamamoto M, Fujii-Kuriyama Y, Furusako S. 2005. Effects of aryl hydrocarbon receptor signaling on the modulation of Th1/Th2 balance. J Immunol. 175(11): 7348–7356.
- Nguyen LP, Bradfield CA. 2008. The search for endogenous activators of the aryl hydrocarbon receptor. Chem Res Toxicol. 21(1): 102–116.
- Nguyen NT, Kimura A, Nakahama T, Chinen I, Masuda K, Nohara K, Fujii-Kuriyama Y, Kishimoto T. 2010. Aryl hydrocarbon receptor negatively regulates dendritic cell immunogenicity via a kynurenine-dependent mechanism. Proc Natl Acad Sci USA. 107(46): 19961–19966.
- Nohara K, Fujimaki H, Tsukumo SI, Inouye K, Sone H, Tohyama C. 2002. Effects of 2,3,7,8-tetrachlorodibenzo-p- dioxin (TCDD) on T cell-derived cytokine production in ovalbumin (OVA)-immunized C57Bl/6 mice. Toxicology. 172(1): 49–58.
- Nohara K, Pan X, Tsukumo SI, Hida A, Ito T, Nagai H, Inouye K, Motohashi H, Yamamoto M, Fujii-Kuriyama Y, Tohyama C. 2005. Constitutively active aryl hydrocarbon receptor expressed specifically in T-lineage cells causes thymus involution and suppresses the immunization-induced increase in splenocytes. J Immunol. 174(5): 2770–2777.
- Noon L. 1911. Prophylactic inoculation against hay fever. Lancet. 177(4580): 1572–1573.
- Oboki K, Ohno T, Saito H, Nakae S. 2008. Th17 and allergy. Allergol Int. 57(2): 121–134.
- Okino ST, Pookot D, Basak S, Dahiya R. 2009. Toxic and chemopreventive ligands preferentially activate distinct aryl hydrocarbon receptor pathways: implications for cancer prevention. Cancer Prev Res. 2(3): 251–256.
- Oliphant CJ, Barlow JL, Mckenzie ANJ. 2011. Insights into the initiation of type 2 immune responses. Immunology. 134(4): 378–385.
- Pabst O, Mowat AM. 2012. Oral tolerance to food protein. Mucosal Immunol. 5(3): 232–239.
- Peron JPS, Ligeiro de Oliveira AP, Rizzo LV. 2009. It takes guts for tolerance: the phenomenon of oral tolerance and the regulation of autoimmune response. Autoimmun Rev. 9(1): 1–4.
- Perrier C, Corthésy B. 2011. Gut permeability and food allergies. Clin Exp Allergy. 41(1): 20–28.
- Quintana FJ, Basso AS, Iglesias AH, Korn T, Farez MF, Bettelli E, Caccamo M, Oukka M, Weiner HL. 2008. Control of Treg and TH17 cell differentiation by the aryl hydrocarbon receptor. Nature. 453(7191): 65–71.
- Quintana FJ, Murugaiyan G, Farez MF, Mitsdoerffer M, Tukpah A, Burns EJ, Weiner HL. 2010. An endogenous aryl hydrocarbon receptor ligand acts on dendritic cells and T cells to suppress experimental autoimmune encephalomyelitis. Proc Natl Acad Sci USA. 107(48): 20768–20773.
- Romagnani S. 2006. Regulation of the T cell response. Clin Exp Allergy. 36(11): 1357–1366.
- Safe S, Wormke M, Samudio I. 2000. Mechanisms of inhibitory aryl hydrocarbon receptor-estrogen receptor crosstalk in human breast cancer cells. J Mammary Gland Biol Neoplasia. 5(3): 295–306.
- Schöll I, Untersmayr E, Bakos N, Roth-Walter F, Gleiss A, Boltz-Nitulescu G, Scheiner O, Jensen-Jarolim E. 2005. Antiulcer drugs promote oral sensitization and hypersensitivity to hazelnut allergens in BALB/c mice and humans. Am J Clin Nutr. 81(1): 154–160.
- Schouten B, Van Esch BCAM, Hofman GA, Boon L, Knippels LMJ, Willemsen LEM, Garssen J. 2010. Oligosaccharide-induced whey-specific CD25+ regulatory T-cells are involved in the suppression of cow milk allergy in mice. J Nutr. 140(4): 835–841.
- Schulz VJ, Smit JJ, Bol-Schoenmakers M, van Duursen MB, van den Berg M, Pieters RH. 2012a. Activation of the aryl hydrocarbon receptor reduces the number of precursor and effector T cells but preserves CD4+CD25+Foxp3+ Treg cells. Toxicol Lett. 215(2): 100–109.
- Schulz VJ, Smit JJ, Willemsen KJ, Fiechter D, Hassing I, Bleumink R, Boon L, van den Berg M, van Duursen MB, Pieters RH. 2012b. Activation of the aryl hydrocarbon receptor suppresses sensitization in a mouse peanut allergy model. Toxicol Sci. 123(2): 491–500.
- Schulz VJ, van Roest M, Bol-Schoenmakers M, van Duursen MB, van den Berg M, Pieters RH, Smit JJ. 2013. Aryl hydrocarbon receptor activation affects the dendritic cell phenotype and function during allergic sensitization. Immunobiology. S0171-2985(13)00026-0, doi:10.1016/j.imbio.2013.01.004.
- Scurlock AM, Jones SM. 2010. An update on immunotherapy for food allergy. Curr Opin Allergy Clin Immunol. 10(6): 587–593.
- Shepherd DM, Dearstyne EA, Kerkvliet NI. 2000. The effects of TCDD on the activation of ovalbumin (OVA)-specific DO11.10 transgenic CD4+ T cells in adoptively transferred mice. Toxicol Sci. 56(2): 340–350.
- Shimba S, Watabe Y. 2009. Crosstalk between the AHR signaling pathway and circadian rhythm. Biochem Pharmacol. 77(4): 560–565.
- Sicherer SH, Sampson HA. 2010. Food allergy. J Allergy Clin Immunol. 125(2 suppl 2): 116–125.
- Simanainen U, Tuomisto JT, Tuomisto J, Viluksela M. 2002. Structure–activity relationships and dose responses of polychlorinated dibenzo-p-dioxins for short-term effects in 2,3,7,8-tetrachlorodibenzo-p-dioxin-resistant and -sensitive rat strains. Toxicol Appl Pharmacol. 181(1): 38–47.
- Simones T, Shepherd DM. 2011. Consequences of AhR activation in steady-state dendritic cells. Toxicol Sci. 119(2): 293–307.
- Singh NP, Nagarkatti M, Nagarkatti PS. 2008. Primary peripheral T cells become susceptible to 2,3,7,8- tetrachlorodibenzo-p-dioxin-mediated apoptosis in vitro upon activation and in the presence of dendritic cells. Mol Pharmacol. 73(6): 1722–1735.
- Singh NP, Singh UP, Singh B, Price RL, Nagarkatti M, Nagarkatti PS. 2011. Activation of Aryl hydrocarbon receptor (AhR) leads to reciprocal epigenetic regulation of Foxp3 and IL-17 expression and amelioration of experimental colitis. PLoS ONE. 6(8): e25322.
- Smit JJ, Bol-Schoenmakers M, Hassing I, Fiechter D, Boon L, Bleumink R, Pieters RHH. 2011. The role of intestinal dendritic cells subsets in the establishment of food allergy. Clin Exp Allergy. 41(6): 890–898.
- Srivastava KD, Qu C, Zhang T, Goldfarb J, Sampson HA, Li X-M. 2009. Food allergy herbal formula-2 silences peanut-induced anaphylaxis for a prolonged posttreatment period via IFN-γ-producing CD8+ T cells. J Allergy Clin Immunol. 123(2): 443–451.
- Sulentic CE, Kaminski NE. 2011. The long winding road toward understanding the molecular mechanisms for B-cell suppression by 2,3,7,8-tetrachlorodibenzo-p-dioxin. Toxicol Sci. 120(1): S171–S191.
- Sun C-M, Hall JA, Blank RB, Bouladoux N, Oukka M, Mora JR, Belkaid Y. 2007. Small intestine lamina propria dendritic cells promote de novo generation of Foxp3 T reg cells via retinoic acid. J Exp Med. 204(8): 1775–1785.
- Temchura VV, Frericks M, Nacken W, Esser C. 2005. Role of the aryl hydrocarbon receptor in thymocyte emigration in vivo. Eur J Immunol. 35(9): 2738–2747.
- Torgerson TR, Ochs HD. 2007. Immune dysregulation, polyendocrinopathy, enteropathy, X-linked: forkhead box protein 3 mutations and lack of regulatory T cells. J Allergy Clin Immunol. 120(4): 744–750.
- Untersmayr E, Schöll I, Swoboda I, Beil WJ, Förster-Waldl E, Walter F, Riemer A, Kraml G, Kinaciyan T, Spitzauer S, et al. 2003. Antacid medication inhibits digestion of dietary proteins and causes food allergy: a fish allergy model in Balb/c mice. J Allergy Clin Immunol. 112(3): 616–623.
- Vandenborre K, Van Gool SW, Kasran A, Ceuppens JL, Boogaerts MA, Vandenberghe P. 1999. Interaction of CTLA-4 (CD152) with CD80 or CD86 inhibits human T-cell activation. Immunology. 98(3): 413–421.
- Van Hoffen E, Ruiter B, Faber J, M’Rabet L, Knol EF, Stahl B, Arslanoglu S, Moro G, Boehm G, Garssen J. 2009. A specific mixture of short-chain galacto-oligosaccharides and long-chain fructo-oligosaccharides induces a beneficial immunoglobulin profile in infants at high risk for allergy. Allergy. 64(3): 484–487.
- Van Wijk F, Hoeks S, Nierkens S, Koppelman SJ, van Kooten P, Boon L, Knippels LMJ, Pieters R. 2005. CTLA-4 signaling regulates the intensity of hypersensitivity responses to food antigens, but is not decisive in the induction of sensitization. J Immunol. 174(1): 174–179.
- Van Wijk F, Wehrens EJM, Nierkens S, Boon L, Kasran A, Pieters R, Knippels LMJ. 2007. CD4+CD25+ T cells regulate the intensity of hypersensitivity responses to peanut, but are not decisive in the induction of oral sensitization. Clin Exp Allergy. 37(4): 572–581.
- Vassallo MF, Camargo Jr CA. 2010. Potential mechanisms for the hypothesized link between sunshine, vitamin D, and food allergy in children. J Allergy Clin Immunol. 126(2): 217–222.
- Veiga-Parga T, Suryawanshi A, Rouse BT. 2011. Controlling viral immuno-inflammatory lesions by modulating aryl hydrocarbon receptor signaling. PLoS Pathog. 7(12): e1002427.
- Veldhoen M, Hirota K, Westendorf AM, Buer J, Dumoutier L, Renauld J-C, Stockinger B. 2008. The aryl hydrocarbon receptor links TH17-cell-mediated autoimmunity to environmental toxins. Nature. 453(7191): 106–109.
- Verlinden A, Hesta M, Millet S, Janssens GPJ. 2006. Food allergy in dogs and cats: a review. Crit Rev Food Sci Nutr. 46(3): 259–273.
- Vogel CF, Goth SR, Dong B, Pessah IN, Matsumura F. 2008. Aryl hydrocarbon receptor signaling mediates expression of indoleamine 2,3-dioxygenase. Biochem Biophys Res Commun. 375(3): 331–335.
- Vogel CF, Matsumura F. 2009. A new cross-talk between the aryl hydrocarbon receptor and RelB, a member of the NF-kappaB family. Biochem Pharmacol. 77(4): 734–745.
- Vollmer J, Krieg AM. 2009. Immunotherapeutic applications of CpG oligodeoxynucleotide TLR9 agonists. Adv Drug Deliv Rev. 61(3): 195–204.
- Vorderstrasse BA, Dearstyne EA, Kerkvliet NI. 2003. Influence of 2,3,7,8-tetrachlorodibenzo-p-dioxin on the antigen-presenting activity of dendritic cells. Toxicol Sci. 72(1): 103–112.
- Vorderstrasse BA, Kerkvliet NI. 2001. 2,3,7,8-Tetrachlorodibenzo-p-dioxin affects the number and function of murine splenic dendritic cells and their expression of accessory molecules. Toxicol Appl Pharmacol. 171(2): 117–125.
- Vos AP, Haarman M, Buco A, Govers M, Knol J, Garssen J, Stahl B, Boehm G, M’Rabet L. 2006. A specific prebiotic oligosaccharide mixture stimulates delayed-type hypersensitivity in a murine influenza vaccination model. Int Immunopharmacol. 6(8): 1277–1286.
- Waller CL, McKinney JD. 1995. Three-dimensional quantitative structure-activity relationships of dioxins and dioxin-like compounds: model validation and Ah receptor characterization. Chem Res Toxicol. 8(6): 847–858.
- Wirnsberger G, Hinterberger M, Klein L. 2011. Regulatory T-cell differentiation versus clonal deletion of autoreactive thymocytes. Immunol Cell Biol. 89(1): 45–53.
- Workman CJ, Szymczak-Workman AL, Collison LW, Pillai MR, Vignali DAA. 2009. The development and function of regulatory T cells. Cell Mol Life Sci. 66(16): 2603–2622.
- Yu JJ, Gaffen SL. 2008. Interleukin-17: a novel inflammatory cytokine that bridges innate and adaptive immunity. Front Biosci. 13: 170–177.
- Zhang L, Ma J, Takeuchi M, Usui Y, Hattori T, Okunuki Y, Yamakawa N, Kezuka T, Kuroda M, Goto H. 2010. Suppression of experimental autoimmune uveoretinitis by inducing differentiation of regulatory T cells via activation of aryl hydrocarbon receptor. Investig Ophthalmol Visual Sci. 51(4): 2109–2117.