Abstract
Pluripotent stem cells are defined by their unlimited self-renewal capacities and potential to differentiate into any cell lineage. Many crucial determinants for the induction and maintenance of this pluripotent state have been identified. Long non-coding RNAs have recently emerged as key regulators of pluripotent stem cells and have enhanced our understanding of their potential functions in tissue regeneration. This review provides an overview of recent important insights into the roles of long non-coding RNAs as regulators and markers of pluripotency.
1. Introduction
Pluripotent stem cells (PSCs) are immortal cells capable of differentiating into any adult lineage-specific cells (Evans & Kaufman Citation1981; Martin Citation1981; Thomson et al. Citation1998). These characteristics are of high value in a clinical context, the unlimited repair and replacement of abnormal, damaged, or absent cells types and tissues. However, ethical issues associated with the need to isolate PS cells from blastocysts has limited their use in therapeutic applications (Thomson et al. Citation1998). The conversion of induced PSCs (iPSCs) back into the pluripotent state, using the expression of reprogramming factors, may bypass the ethical and technical controversies associated with deriving embryonic stem cells (ESCs) from embryos (Robinton & Daley Citation2012). Many recent investigations have described transcriptional and epigenetic processes that are fundamental to the maintenance and differentiation of iPS cells, yet a comprehensive molecular strategy outlining their therapeutic applications is lacking (Kim et al. Citation2008; Kim et al. Citation2010; Fisher & Fisher Citation2011; Onder et al. Citation2012).
Recent research has shown that apart from transcription in protein-coding genes, much of the genome is transcribed in non-coding RNA (ncRNA). Several classes of ncRNA have been identified according to their length and/or function, among which are ribosomal and transfer RNAs, and microRNAs. MicroRNAs are a group of 21 base pair (bp) length RNAs that regulate protein levels through the degradation or the translational inhibition of complementary target mRNAs (Lee et al. Citation1993; Kloosterman & Plasterk Citation2006). Their roles in stem cell development and maintenance are well established, and outside of this review's scope (Houbaviy et al. Citation2003; Choi et al. Citation2011; Li et al. Citation2011; Miyoshi et al. Citation2011).
Long ncRNAs (lncRNAs), which are RNAs of more than 200 nucleotides in size, are less well characterized, yet some are vital for a variety of biological processes, including the epigenetic control of chromatin (Rinn et al. Citation2007), promoter-specific gene regulation (Feng et al. Citation2006; Martianov et al. Citation2007), X-chromosome inactivation (Lee Citation2009; Tian et al. Citation2010), imprinting (Bartolomei et al. Citation1991; Lyle et al. Citation2000), nuclear import (Willingham et al. Citation2005), and the maintenance of nuclear body structure (Mao et al. Citation2011). Genomic studies have revealed that the human genome encodes thousands of lncRNAs, which is comparable to the number of coding RNAs. However, putative non-coding transcripts can also encode small peptides and discoveries in D. melanogaster have demonstrated that the gene polar granule component (Pgc) may encode a small protein and that the polycistronic RNA polished rice (Pri) may encode several small peptides that control the activity of the transcription factor Shavenbaby (Kondo et al. Citation2007; Kondo et al. Citation2010).
lncRNAs can be further subdivided into intronic sense and anti-sense lncRNAs (transcribed from within introns located within protein-coding genes) (Kampa et al. Citation2004), anti-sense lncRNAs that overlap with protein-coding genes (Katayama et al. Citation2005; He et al. Citation2008), and lncRNAs that are found between protein-coding genes (Guttman et al. Citation2009; Khalil et al. Citation2009; Jia et al. Citation2010) (). A common feature of lncRNAs is the low coding potential, a feature that can be calculated through the online tool LNCipedia (www.lncipedia.org). The exact role of the vast majority of lncRNAs remains unclear, often due to technical difficulties associated with the manipulation of lncRNAs.
Figure 1. Classification of lncRNAs. Antisense lncRNAs are transcribed in the opposite orientation to a coding gene, and overlap with one or several exons. Intronic lncRNAs are located within an intron of a coding gene. Intergenic lncRNAs do not overlap with any coding gene and thus are located between coding genes.
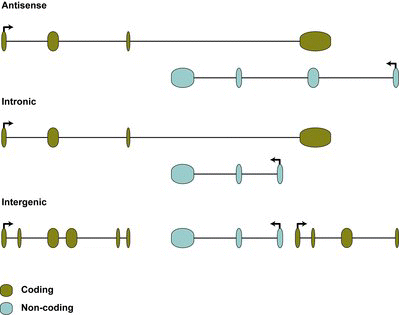
In this review, we highlight the recent advances in our understanding of the action mechanism and function of lncRNAs, with a special focus on lncRNA-mediated regulation of PSC maintenance and differentiation.
2. lncRNAs: action and function
Despite our limited knowledge, arising from just a handful of well-characterized lncRNAs, several mechanistic themes of lncRNAs’ functions have emerged (). Of these, the best known are their scaffolding and guidance functions ((A) and 2(B)). In the former, lncRNA serves to bring two or more proteins together into a complex, which then exert a specific cellular function, such as the assembly of the telomerase complexes TERC and HOTAIR or ANRIL, both of which play roles in generating complexes essential to maintaining a cells chromatin state (Zappulla & Cech Citation2006; Khalil et al. Citation2009; Kotake et al. Citation2010; Tsai et al. Citation2010).
Figure 2. Different modes of action of lncRNAs. (A) lncRNAs can act as miRNA sponges, diminishing functional miRNAs. (B) lncRNAs can bind splice sites, thus interfering with normal splicing. (C) lncRNAs can act as scavengers of proteins responsible for regulating their transport between the cytoplasm and the nucleus. (D) lncRNAs can promote or inhibit gene transcription by providing essential transcription factors or inhibiting their binding to promoter regions. (E) lncRNAs bind chromatin remodeling factors.
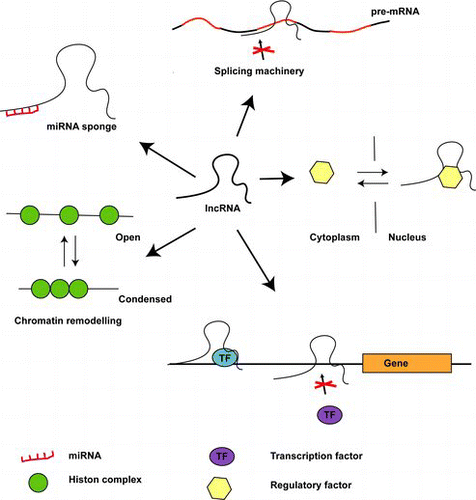
In its guiding function, lncRNAs are essential for the intracellular positioning of protein complexes ((B)). A classical example is the XIST lncRNA, which mediates X-chromosomal dosage compensation (Brown et al. Citation1991). More recently, the lncRNA p21 was shown to be induced by p53, upon DNA damage, and subsequently binds to the nuclear factor hnRNP-K, thereby guiding it to specific promoters (Huarte et al. Citation2010).
Another function is in decoy, in which lncRNAs prevent regulatory proteins accessing DNA. The GAS5 lncRNA is a decoy lncRNA (Kino et al. Citation2010) that possesses a DNA binding motif which resembles the DNA binding motif of the glucocorticoid receptor. During starvation conditions, GAS5 lncRNA is induced releasing the glucocorticoid receptor from the DNA and preventing the transcription of metabolic genes (Kino et al. Citation2010). Furthermore, several recent reports have suggested that lncRNAs may function as competing endogenous RNAs by modulating the concentrations and biological functions of miRNAs (Cesana et al. Citation2011; Tay et al. Citation2011); as such lncRNAs are often denominated ‘miRNA-sponges’. Tay et al. Citation(2011) demonstrated that miRNA response elements present within the PTENP1 lncRNA prevent the phosphatase and tensin homolog (PTEN) mRNA from miRNA-mediated degradation (Tay et al. Citation2011). Similarly, Cesana et al. observed that the Linc-MD1 lncRNA prevents the MAML1 and MEF2CmRNAs from miRNA-mediated degradation (Cesana et al. Citation2011).
Several other mechanisms of action have been reported, including the inhibition of splicing, thereby adding transcriptional complexity, and the regulation of nuclear-cytoplasmic trafficking (Willingham et al. Citation2005; Beltran et al. Citation2008).
3. lncRNAs in pluripotent stem cell functioning
With the advent of genome-wide platforms for studying lncRNA expression, several recent investigations have also demonstrated an involvement for lncRNAs in maintaining the pluripotent states of human and mouse ESCs (Ng & Stanton Citation2013).
Dinger et al. Citation(2008) identified 12 lncRNAs that showed a similar expression profile to Pou5f1, Nanog, and Sox2 and which were essential transcription factors in maintaining the pluripotent state of mouse ESCs during a 16-day differentiation experiment. Chromatin immunoprecipitation (ChIP) with antibodies against Pou5f1 and Nanog further revealed that most of these lncRNAs were bound by Pou5f1 and Nanog in ESCs (Boyer et al. Citation2005; Dinger et al. Citation2008). Ng et al. Citation(2012) also identified 36 lncRNAs with a similar expression pattern to that of Oct4 and Nanog, which were highly expressed in human ESCs and weakly expressed upon differentiation (Ng et al. Citation2012). Both reports identify the telomerase RNA component TERC, which was previously suggested to be a lncRNA associated with pluripotency (Dinger et al. Citation2008; Agarwal et al. Citation2010; Ng et al Citation2012). Furthermore, siRNA-mediated silencing of three lncRNAs exclusively expressed in human ESCs resulted in the up regulation of several lineage differentiation markers and down regulation of pluripotency genes (Ng et al. Citation2012). Moreover, integration with ChIP-Sequencing data revealed that Oct4 and Nanog binding occurs near the transcription start site of these three lncRNAs, suggesting that they are regulated by the pluripotency identifiers Oct4 and Nanog (Ng et al. Citation2012). Sheik Mohamed et al. Citation(2010) also identified a group of lncRNAs bound by Oct4 and Nanog, through immunoprecipitation with Oct4- and Nanog-specific antibodies, and the subsequent sequencing of bound RNAs. This was confirmed by silencing the lncRNAs and analyzing subsequent effects on ESC differentiation, which showed that several lncRNAs bound by Oct4 and Nanog, regulate the expression of these transcription factors.
In addition, recent loss-of-function experiments involving 226 intergenic lncRNAs expressed in mouse ESCs identified at least 26 lncRNAs whose loss resulted in the reduced expression of the pluripotency markers Nanog and Oct4 (Guttman et al. Citation2009). In addition, the induction of expression patterns which corresponded to differentiation was observed (Guttman et al. Citation2009). Taken together these reports demonstrate that lncRNAs are essential components in the regulatory circuitry regulating the maintenance of pluripotency of ESCs.
Similar data have also been shown in human model systems. Loewer et al. Citation(2010) compared lncRNA expression in iPSCs to expression patterns in ESCs, reasoning that their higher expression may have conferred a selective advantage on emerging iPSCs. They identified 28 lncRNAs fulfilling this criterion. Considering that their promoters should be occupied by stem cell factors such as Nanog, Oct4, and/or Sox2, they identified three lncRNAs which fulfilled their definition, i.e. lncRNA-SFMBT2, lncRNA-VLDLR, and lncRNA-RoR. In addition, lncRNA-RoR was shown to repress the p53 response, indicating a role for lncRNAs in promoting pluripotency by providing a survival advantage to iPSCs (Loewer et al. Citation2010).
Using bioinformatics, Wang et al. Citation(2013) also revealed that lncRoR was important in maintaining the pluripotency state. Moreover, as lncRNAs may function as miRNA sponges, thereby controlling the content of regulatory miRNAs and thus their respective targets, these investigators determined if lncRoRs could act as miRNA sponges, thereby regulating Oct4 and Nanog expression (Wang et al. Citation2013). As lncRoR shares binding sites for miR-145, miR-181, and miR-99, with Oct4, Sox2, and Nanog, these miRNAs were obvious candidates. Transfection of the miR-145 binding site mutant lncRoR with miR-mimics, showed that lncRoR serves as a miR-sponge, reducing the concentration of miR-145 in vivo, and protecting Oct4, Sox2, and Nanog from miR-145 dependent suppression, in both HEK293 and hESC cells (Wang et al. Citation2013).
4. lncRNAs and the quality of iPSCs
The use of PSCs for generating therapeutically useful cell lineages must be a clinical goal. However, it has become increasingly clear that both ESCs and iPSCs vary considerably in the quality and quantity of their differentiation potentials (Chang et al. Citation2008; Osafune et al. Citation2008). Efforts to characterize the molecular mechanisms responsible for such variability between human ESC and iPSC lines have initially focused on the variations between expression levels of protein-coding genes (Bock et al. Citation2011). Nonetheless, considering the research outlined in the present review, varying lncRNAs expression patterns should provide insight into the differentiation potentials of ESC and iPSC lines.
5. Conclusions
Recent genome-wide and single lncRNA studies have provided evidence that lncRNA are key regulators in the maintenance of the stem cell pluripotent state. Some lncRNAs function by establishing and/or maintaining chromatin markers, or via interactions with essential transcription factors responsible for regulating pluripotency. Despite this, further molecular functional studies are needed. To date, publications addressing the functional role of lncRNAs in pluripotency maintenance are rare, and even more so in a human model. Considering that many ESC specific lncRNAs are not well conserved between human and mouse, revealing their functional roles in a human developmental context will pose a challenge.
Acknowledgements
This work was supported by a grant from the Foundation Against Cancer (Stichting Tegen Kanker, Belgium, 2010-187) and Kinderkankerfonds vzw (Belgium).
References
- Agarwal S, Loh YH, McLoughlin EM, Huang J, Park IH, Miller JD, Huo H, Okuka M, Dos Reis RM, Loewer S, et al. 2010. Telomere elongation in induced pluripotent stem cells from dyskeratosis congenita patients. Nature. 464:292–296.
- Bartolomei MS, Zemel S, Tilghman SM. 1991. Parental imprinting of the mouse H19 gene. Nature. 351:153–155.
- Beltran M, Puig I, Peña C, García JM, Alvarez AB, Peña R, Bonilla F, de Herreros AG. 2008. A natural antisense transcript regulates Zeb2/Sip1 gene expression during Snail1-induced epithelial-mesenchymal transition. Genes Dev. 22:756–769.
- Bock C, Kiskinis E, Verstappen G, Gu H, Boulting G, Smith ZD, Ziller M, Croft GF, Amoroso MW, Oakley DH, et al. 2011. Reference maps of human ES and iPS cell variation enable high-throughput characterization of pluripotent cell lines. Cell. 144:439–452.
- Boyer LA, Lee TI, Cole MF, Johnstone SE, Levine SS, Zucker JP, Guenther MG, Kumar RM, Murray HL, Jenner RG, et al. 2005. Core transcriptional regulatory circuitry in human embryonic stem cells. Cell. 122:947–956.
- Brown CJ, Ballabio A, Rupert JL, Lafreniere RG, Grompe M, Tonlorenzi R, Willard HF. 1991. A gene from the region of the human X inactivation centre is expressed exclusively from the inactive X chromosome. Nature. 349:38–44.
- Cesana M, Cacchiarelli D, Legnini I, Santini T, Sthandier O, Chinappi M, Tramontano A, Bozzoni I. 2011. A long noncoding RNA controls muscle differentiation by functioning as a competing endogenous RNA. Cell. 147:358–369.
- Chang KH, Nelson AM, Fields PA, Hesson JL, Ulyanova T, Cao H, Nakamoto B, Ware CB, Papayannopoulou T. 2008. Diverse hematopoietic potentials of five human embryonic stem cell lines. Exp Cell Res. 314:2930–2940.
- Choi J, Lin CP, Ho JJ, He X, Okada N, Bu P, Zhong Y, Kim SY, Bennett MJ, Chen C, et al. 2011. miR-34 miRNAs provide a barrier for somatic cell reprogramming. Nat Cell Biol. 13:1353–1360.
- Dinger ME, Amaral PP, Mercer TR, Pang KC, Bruce SJ, Gardiner BB, Askarian-Amiri ME, Ru K, Soldà G, Simons C, et al. 2008. Long noncoding RNAs in mouse embryonic stem cell pluripotency and differentiation. Genome Res. 18:1433–1445.
- Evans MJ, Kaufman MH. 1981. Establishment in culture of pluripotential cells from mouse embryos. Nature. 292:154–156.
- Feng J, Bi C, Clark BS, Mady R, Shah P, Kohtz JD. 2006. The Evf-2 noncoding RNA is transcribed from the Dlx-5/6 ultraconserved region and functions as a Dlx-2 transcriptional coactivator. Genes Dev. 20:1470–1484.
- Fisher CL, Fisher AG. 2011. Chromatin states in pluripotent, differentiated, and reprogrammed cells. Curr Opin Genet Dev. 21:140–146.
- Guttman M, Amit I, Garber M, French C, Lin MF, Feldser D, Huarte M, Zuk O, Carey BW, Cassady JP, et al. 2009. Chromatin signature reveals over a thousand highly conserved large non-coding RNAs in mammals. Nature. 458:223–227.
- He Y, Vogelstein B, Velculescu VE, Papadopoulos N, Kinzler KW. 2008. The antisense transcriptomes of human cells. Science. 322:1855–1857.
- Houbaviy HB, Murray MF, Sharp PA. 2003. Embryonic stem cell-specific microRNAs. Dev Cell. 5:351–358.
- Huarte M, Guttman M, Feldser D, Garber M, Koziol MJ, Kenzelmann-Broz D, Khalil AM, Zuk O, Amit I, Rabani M, et al. 2010. A large intergenic noncoding RNA induced by p53 mediates global gene repression in the p53 response. Cell. 142:409–419.
- Jia H, Osak M, Bogu GK, Stanton LW, Johnson R, Lipovich L. 2010. Genome-wide computational identification and manual annotation of human long noncoding RNA genes. RNA. 16:1478–1487.
- Kampa D, Cheng J, Kapranov P, Yamanaka M, Brubaker S, Cawley S, Drenkow J, Piccolboni A, Bekiranov S, Helt G, et al. 2004. Novel RNAs identified from an in-depth analysis of the transcriptome of human chromosomes 21 and 22. Genome Res. 14:331–342.
- Katayama S, Tomaru Y, Kasukawa T, Waki K, Nakanishi M, Nakamura M, Nishida H, Yap CC, Suzuki M, Kawai J, et al. 2005. Antisense transcription in the mammalian transcriptome. Science. 309:1564–1566.
- Khalil AM, Guttman M, Huarte M, Garber M, Raj A, RiveaMorales D, Thomas K, Presser A, Bernstein BE, van Oudenaarden A, et al. 2009. Many human large intergenic noncoding RNAs associate with chromatin-modifying complexes and affect gene expression. Proc Natl Acad Sci USA. 106:11667–11672.
- Kim J, Chu J, Shen X, Wang J, Orkin SH. 2008. An extended transcriptional network for pluripotency of embryonic stem cells. Cell. 132:1049–1061.
- Kim J, Woo AJ, Chu J, Snow JW, Fujiwara Y, Kim CG, Cantor AB, Orkin SH. 2010. A Myc network accounts for similarities between embryonic stem and cancer cell transcription programs. Cell. 143:313–324.
- Kino T, Hurt DE, Ichijo T, Nader N, Chrousos GP. 2010. Noncoding RNA Gas5 is a growth arrest and starvation-associated repressor of the glucocorticoid receptor. Sci Signal. 3:ra8.
- Kloosterman WP, Plasterk RH. 2006. The diverse functions of microRNAs in animal development and disease. Dev Cell. 11:441–450.
- Kondo T, Hashimoto Y, Kato K, Inagaki S, Hayashi S, Kageyama Y. 2007. Small peptide regulators of actin-based cell morphogenesis encoded by a polycistronic mRNA. Nat Cell Biol. 9:660–665.
- Kondo T, Plaza S, Zanet J, Benrabah E, Valenti P, Hashimoto Y, Kobayashi S, Payre F, Kageyama Y. 2010. Small peptides switch the transcriptional activity of shavenbaby during Drosophila embryogenesis. Science. 329:336–339.
- Kotake Y, Nakagawa T, Kitagawa K, Suzuki S, Liu N, Kitagawa M, Xiong Y. 2010. Long non-coding RNA ANRIL is required for the PRC2 recruitment to and silencing of p15INK4B tumor suppressor gene. Oncogene. 30:1956–1962.
- Lee JT. 2009. Lessons from X-chromosome inactivation: long ncRNA as guides and tethers to the epigenome. Genes Dev. 23:1831–1842.
- Lee RC, Feinbaum RL, Ambros V. 1993. The C. elegans heterochronic gene lin-4 encodes small RNAs with antisense complementarity to lin-14. Cell. 75:843–854.
- Li Z, Yang CS, Nakashima K, Rana TM. 2011. Small RNA-mediated regulation of iPS cell generation. EMBO J. 30:823–834.
- Loewer S, Cabili MN, Guttman M, Loh YH, Thomas K, Park IH, Garber M, Curran M, Onder T, Agarwal S, et al. 2010. Large intergenic non-coding RNA-RoR modulates reprogramming of human induced pluripotent stem cells. Nat Genet. 42:1113–1117.
- Lyle R, Watanabe D, te Vruchte D, Lerchner W, Smrzka OW, Wutz A, Schageman J, Hahner L, Davies C, Barlow DP. 2000. The imprinted antisense RNA at the Igf2r locus overlaps but does not imprint Mas1. Nat Genet. 25:19–21.
- Mao YS, Sunwoo H, Zhang B, Spector DL. 2011. Direct visualization of the co-transcriptional assembly of a nuclear body by noncoding RNAs. Nat Cell Biol. 13:95–101.
- Martianov I, Ramadass A, Serra Barros A, Chow N, Akoulitchev A. 2007. Repression of the human dihydrofolate reductase gene by a noncoding interfering transcript. Nature. 445:666–670.
- Martin GR. 1981. Isolation of a pluripotent cell line from early mouse embryos cultured in medium conditioned by teratocarcinoma stemcells. Proc Natl Acad Sci USA. 78:7634–7638.
- Miyoshi N, Ishii H, Nagano H, Haraguchi N, Dewi DL, Kano Y, Nishikawa S, Tanemura M, Mimori K, Tanaka F, et al. 2011. Reprogramming of mouse and human cells to pluripotency using mature microRNAs. Cell Stem Cell. 8:633–638.
- Ng SY, Johnson R, Stanton LW. 2012. Human long non-coding RNAs promote pluripotency and neuronal differentiation by association with chromatin modifiers and transcription factors. EMBO J. 31:522–533.
- Ng SY, Stanton LW. 2013. Long non-coding RNAs in stem cell pluripotency. Wiley Interdiscip Rev RNA. 4:121–128.
- Onder TT, Kara N, Cherry A, Sinha AU, Zhu N, Bernt KM, Cahan P, Mancarci OB, Unternaehrer J, Gupta PB, et al. 2012. Chromatin-modifying enzymes as modulators of reprogramming. Nature. 483:598–602.
- Osafune K, Caron L, Borowiak M, Martinez RJ, Fitz-Gerald CS, Sato Y, Cowan CA, Chien KR, Melton DA. 2008. Marked differences in differentiation propensity among human embryonic stem cell lines. Nat Biotechnol. 26:313–315.
- Rinn JL, Kertesz M, Wang JK, Squazzo SL, Xu X, Brugmann SA, Goodnough LH, Helms JA, Farnham PJ, Segal E, Chang HY. 2007. Functional demarcation of active and silent chromatin domains in human HOX loci by noncoding RNAs. Cell. 129:1311–1323.
- Robinton DA, Daley GQ. 2012. The promise of induced pluripotent stem cells in research and therapy. Nature. 481:295–305.
- Sheik Mohamed J, Gaughwin PM, Lim B, Robson P, Lipovich L. 2010. Conserved long noncoding RNAs transcriptionally regulated by Oct4 and Nanog modulate pluripotency in mouse embryonic stem cells. RNA. 16:324–337.
- Tay Y, Kats L, Salmena L, Weiss D, Tan SM, Ala U, Karreth F, Poliseno L, Provero P, Di Cunto F, et al. 2011. Coding-independent regulation of the tumor suppressor PTEN by competing endogenous mRNAs. Cell. 147:344–357.
- Thomson JA, Itskovitz-Eldor J, Shapiro SS, Waknitz MA, Swiergiel JJ, Marshall VS, Jones JM. 1998. Embryonic stem cell lines derived from human blastocysts. Science. 282:1145–1147.
- Tian D, Sun S, Lee JT. 2010. The long noncoding RNA, Jpx, is a molecular switch for X chromosome inactivation. Cell. 143:390–403.
- Tsai MC, Manor O, Wan Y, Mosammaparast N, Wang JK, Lan F, Shi Y, Segal E, Chang HY. 2010. Long noncoding RNA as modular scaffold of histone modification complexes. Science. 329:689–693.
- Wang Y, Xu Z, Jiang J, Xu C, Kang J, Xiao L, Wu M, Xiong J, Guo X, Liu H. 2013. Endogenous miRNA sponge lincRNA-RoR regulates Oct4, Nanog, and Sox2 in human embryonic stem cell self-renewal. Dev Cell. 25:69–80.
- Willingham AT, Orth AP, Batalov S, Peters EC, Wen BG, Aza-Blanc P, Hogenesch JB, Schultz PG. 2005. A strategy for probing the function of noncoding RNAs finds a repressor of NFAT. Science. 309:1570–1573.
- Zappulla DC, Cech TR. 2006. RNA as a flexible scaffold for proteins: yeast telomerase and beyond. Cold Spring Harbor Symp Quantum Biol. 71:217–224.