Abstract
Cushing's disease (CD) or pituitary-dependent hypercortisolism is a common endocrinopathy in dogs, with an estimated prevalence of 1 or 2 in 1000 dogs per year. It is caused by an adrenocorticotropic hormone secreting adenoma in the pars distalis or pars intermedia of the pituitary gland. The pituitary gland is a small endocrine gland located in the pituitary fossa. In the postnatal individual, the hypothalamus–pituitary axis plays a central role in maintaining homeostatic functions, like control of metabolism, reproduction, and growth. Stem cells are suggested to play a role in the homeostatic adaptations of the adult pituitary gland, such as the rapid specific cell-type expansion in response to pregnancy or lactation. Several cell populations have been suggested as pituitary stem cells, such as Side Population cells and cells expressing Sox2 or Nestin. These cell populations are discussed in this review. Also, stem and progenitor cells are thought to play a role in pituitary tumorigenesis, such as the development of pituitary adenomas in dogs. There are limited reports on the role of stem cells in pituitary adenomas, especially in dogs. Further studies are needed to identify and characterize this cell population and to develop specific cell targeting therapeutic strategies as a new way of treating canine CD.
1. Introduction on pituitary corticotroph adenomas in dogs
Cushing's disease (CD) or pituitary-dependent hypercortisolism (PDH) is a common endocrinopathy in dogs, with an estimated prevalence of 1 or 2 in 1000 dogs per year (Willeberg & Priester Citation1982). It is caused by an adrenocorticotropic hormone (ACTH) secreting adenoma in the pars distalis or pars intermedia of the pituitary gland. Clinical signs are caused by ACTH-induced hypercortisolism and include polyuria-polydipsia, polyphagia, panting, heat intolerance, enlargement of the abdomen caused by centripetal fat storage, skin atrophy, alopecia, muscle weakness, and lethargy (Rijnberk & Kooistra Citation2010). The diagnosis of hypercortisolism is based on history, clinical signs, and elevated levels of urinary corticoid-to-creatinine ratios (Rijnberk et al. Citation1988; Galac et al. Citation1997). PDH and pituitary-independent hypercortisolism are distinguished with a urinary high-dose dexamethasone suppression test and measurement of basal plasma ACTH concentration (Galac et al. Citation1997). Moreover, imaging of the pituitary gland and adrenals with computed tomography or magnetic resonance imaging contributes to the diagnosis (van der Vlugt-Meijer et al. Citation2002).
PDH in dogs is medically treated with o,p’-DDD (Mitotane®), which selectively destroys the cortex of the adrenal gland, or with trilostane (Vetoryl®), which inhibits corticoid secretion (Kooistra et al. Citation2009; Rijnberk & Kooistra Citation2010). Treatment directed at the cause of the disease, i.e., the adenoma in the pituitary gland, includes radiotherapy and pituitary surgery. There is limited experience with the use of radiotherapy in dogs with PDH (de Fornel et al. Citation2007; Mayer & Treuil Citation2007). In pituitary surgery, the entire pituitary gland is removed via a microsurgical transsphenoidal approach (Meij Citation2001; Meij et al. Citation2002). Postoperatively dogs need lifelong hormone substitution (Meij Citation2001; Hanson et al. Citation2005).
Recently, 3D mice pituitary cultures based on differentiation of embryonic stem cells have been produced. The embryonic stem cell derived pituitary tissue responds as an endocrine tissue in vitro and in vivo; when grafted in hypophysectomized mice plasma, ACTH and glucocorticoid levels increased. However, much further investigation is needed before clinical implementation is possible (Suga et al. Citation2011).
2. Morphology and function of the pituitary gland
The pituitary gland is a small endocrine gland located in the pituitary fossa, a depression in the basisphenoid bone ((a)). It is rostrocaudally placed with its long axis parallel to the ventral surface of the brain. Rostrally, the fossa is lined with the tuberculum sellae; the dorsum sellae forms its caudal lining (Meij Citation2001). A cylindrical stalk, the infundibulum or pars proximalis neurohypophysis, connects the pituitary gland with the hypothalamus. The third ventricle forms an invagination in the pituitary stalk, the infundibular recess. The pars proximalis neurohypophysis continues into the largest part of the neurohypophysis, the pars distalis neurohypophysis or lobus nervosus. The largest part of the pars distalis adenohypophysis is placed ventrorostrally of the pars distalis neurohypophysis and surrounds it almost totally. The inner part of the adenohypophysis, the pars intermedia adenohypophysis, contacts the pars distalis neurohypophysis directly. Together they form the neurointermediate lobe. Rathke's cleft divides the pars distalis adenohypophysis and the pars intermedia. The pars infundibularis adenohypophysis surrounds the pars proximalis neurohypophysis and covers part of the median eminence. The pituitary gland consists of three functional units: the anterior pituitary, formed by the pars infundibularis and pars distalis of the adenohypophysis; the intermediate lobe (pars intermedia) and the posterior pituitary (neurohypophysis) ((b)) (Hullinger Citation1993; Meij Citation2001).
Figure 1. Anatomy of the canine pituitary gland. (a) Schematic lateral view of a canine skull. The pituitary gland (red) is positioned in the cranial cavity underneath the brain. (b) The pituitary gland is lying in the pituitary fossa, which is a shallow hollow in the sphenoid bone. The schematic drawing shows the two parts of the pituitary gland; the adenohypophysis (gray and black) and the neurohypophysis (white) and their respective subregions (reproduced with permission, Hanson 2007).
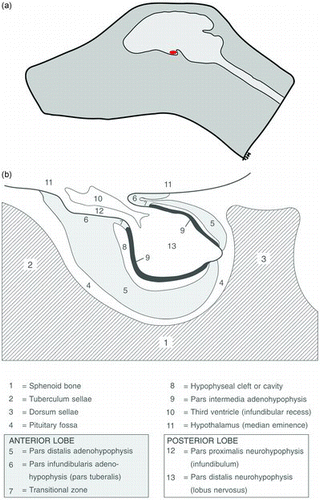
The hypothalamus–pituitary axis plays a central role in maintaining homeostatic functions, like control of metabolism, reproduction, and growth (Rijnberk & Kooistra Citation2010). The anterior pituitary contains five distinct types of endocrine cells (); the corticotroph cells that produce ACTH and β-lipoprotein (β-LPH) account for 10–20% of the total cell population, the thyrotroph cells that produce thyroid stimulating hormone (TSH) comprise 10%, the gonadotroph cells that produce luteinizing hormone (LH) and follicle stimulating hormone (FSH) also represent 10%. Around fifty percent of the cells are somatotroph cells that produce growth hormone (GH). The lactotroph cells that produce prolactin (PRL) make up for 10–20% of the cell population. A small population of cells produces GH and PRL, the mammosomatotroph cells. The anterior pituitary also contains a nonhormone producing population, the folliculostellate cells (Yeung et al. Citation2006).
Figure 2. Hypothalamo-pituitary axis. Simplified diagram of hypophysiotrophic regulation of the secretion of hormones in the adenohypophysis. pd: pars distalis adenohypophysis; pi: pars intermedia adenohypophysis; AVP: arginine vasopressine; CRH corticotropin releasing hormone; GnRH: gonadotropin releasing hormone; GHRH: growth hormone releasing hormone; SRIF: somatostatin; TRH: thyrotrophin releasing factor; PRF: prolactin releasing factor; PIF: prolactin inhibiting factor; DA: dopamine; ACTH: adrenocorticotropic hormone; LH: luteinizing hormone; FSH: follicle-stimulating hormone; GH: growth hormone; TSH: thyroid-stimulating hormone; PRL: prolactin; α-MSH: α-melanocyte-stimulating hormone; IGF-1: insulin growth-factor 1 (reproduced with permission, Hanson 2007).
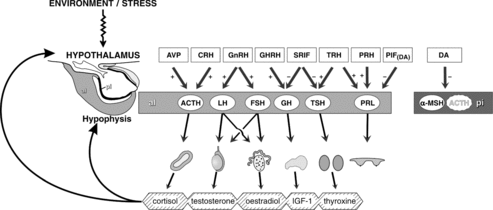
The pars intermedia contains corticotrophs and melanotrophs that produce ACTH and α-melanocyte stimulating hormone (α-MSH), respectively (Halmi et al. Citation1981). The neurohypophysis is the storage place for vasopressin and oxytocin that are produced in hypothalamic nuclei (Hullinger Citation1993).
3. Development of the pituitary gland
The embryological development of the pituitary is more or less similar for all mammals ( (a)). During pituitary organogenesis, the adenohypophysis and neurohypophysis develop from an intimate interaction between the ectoderm of the dorsal aspect of the primitive mouth and the ventral neuroectoderm of the diencephalon, the primordium of the ventral hypothalamus (Daikoku Citation1983; Treier et al. Citation1998; Kouki Citation2001). At the site of apposition of these two ectodermal layers, there is a thickening of the ectoderm of the primitive mouth, which then evaginates and forms Rathke's pouch. At the same time, the diencephalon thickens and evaginates to form the infundibulum, which gradually expands and develops into the neurohypophysis. The pars intermedia develops from the point of fusion of the two ectodermal layers; the bulk of the adenohypophysis develops through proliferation of the anterior wall of Rathke's pouch. During organogenesis, progenitor cells are present along the wall of Rathke's pouch that differentiate into the various pituitary cell lineages (Scully & Rosenfeld Citation2002). With the growth of the embryo Rathke's pouch is closed, the ectodermal stalk regresses and the separation between the adenohypophysis and the oronasal cavity is completed by the formation of the basisphenoid bone (Sasaki & Nishioka Citation1998). In the adult canine pituitary, Rathke's pouch only persists as Rathke's cleft (Sasaki & Nishioka Citation1998).
Figure 3. The development of the pituitary gland. (a) Pituitary ontogeny of the canine pituitary and the corticotroph cells (black spots in the pars distalis adenohypophysis and darkening of the pars intermedia adenohypophysis) (adapted from Sasaki and Nishioka Citation1998). r: Rathke's pouch (the proposed location of stem cells in the postnatal pituitary gland); o: oral cavity; t: tongue; n: neurohypophysis; pi: pars intermedia adenohypophysis; pd: pars distalis adenohypophysis. Right side: Time bar showing embryonic (e) days of development in the dog and mouse. (b) Expression of transcription factors and cellular differentiation during the development of the pituitary gland. Top diagram shows early placode formation and regions of different expression of transcription factors. The middle diagram shows dorsal and ventral transcription factors that build up concentration gradients in which the cells of Rathke's pouch further differentiate. Lower diagram shows differentiation of the hormone-producing cells of the adenohypophysis in relation to embryonic development. Important transcription factors for the respective cell lineages are indicated beside the arrow (adapted from Asteria Citation2002; Treier et al. Citation2001). Reproduced with permission, Hanson 2007.
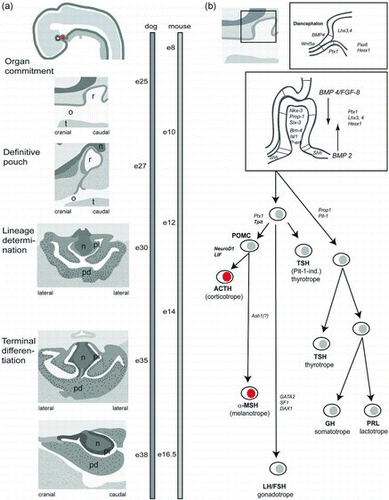
There are three main pathways of differentiation for the hormone producing cells in the anterior hypophysis ((b)) (Sasaki & Nishioka Citation1998; Wagner & Thomas Citation2007). Expression of T-pit that binds with corticotropin upstream transcription-binding element (CUTE) proteins determines the differentiation of the pro-opiomelanocortin cell lineage, which consists of corticotroph and melanotroph cells (Drouin et al. Citation1998; Lamolet et al. Citation2001; Pulichino et al. Citation2003). Gonadotroph cells need expression of steroidogenic factor 1 (SF-1), GATA-2, and LIM homeobox 4 (Lhx4) (Dasen et al. Citation1999; Mullis Citation2001; Zhao et al. Citation2001). Expression of the pituitary transcription factor 1 (Pit-1) and the Prophet of Pit-1 (Prop-1) leads to the development of somatotroph, mammosomatotroph, lactotroph, and thyrotroph cells (Simmons et al. Citation1990; Mullis Citation2001).
It is shown that in the rat pituitary gland, approximately 30% of cells arise from mitosis of already differentiated cells, whereas the others are produced from undifferentiated cells or possible stem cells (Melmed Citation2003). There is increasing interest in the identification of the stem cell population in the pituitary gland from a developmental point of view. Furthermore, stem cells have been implicated to be related to pituitary tumorigenesis (Melmed Citation2011) and are being further explored as a possible anticancer therapeutic approach.
4. Stem cells in the pituitary gland
The main characteristics of stem cells are ability for self-renewal and capacity to differentiate into multiple lineages. Therefore, stem cells are suggested to play a role in the homeostatic adaptations of the adult pituitary gland, such as the rapid specific cell-type expansion in response to pregnancy or lactation (Taniguchi et al. Citation2002; Chen et al. Citation2005; Vankelecom Citation2007a, Citation2010; de Almeida et al. Citation2010; Vankelecom & Gremeaux Citation2010; Nassiri et al. Citation2013).
In the past, various cell types have been suggested to act as stem cells in the adult pituitary, such as folliculo-stellate cells, marginal cells, Side Population (SP) cells, or cells expressing Nestin or Sox2. Most of these studies have been executed in murine models and all proposed stem cell populations possess several characteristics, such as their location along the Rathke's cleft, their ability to form spheres in culture and to differentiate into the five hormonal cell lineages (Vankelecom Citation2007a, Citation2007b; de Almeida et al. Citation2010; Florio Citation2011; Nassiri et al. Citation2013). A summary of stem cell characteristics of the different cell types described is given in .
Table 1. Summary of stem cell characteristics of the different cell types described in the review.
4.1. SP cells
SP isolation by fluorescence-activated cell sorting (FACS) has proven successful to identify and isolate stem and progenitor cells from multiple tissue types (Wolf et al. Citation1993; Goodell et al. Citation1996; Challen & Little Citation2006; Hadnagy et al. Citation2006). This SP phenotype is mediated by membrane efflux pumps of the ATP-binding cassette (ABC) transporter family; the activity of these pumps is inhibited by ABC-inhibitors such as verapamil, resulting in the disappearance of the SP streak from the FACS plot (Chen et al. Citation2005; Challen & Little Citation2006; Hadnagy et al. Citation2006). The SP was first isolated in mouse bone marrow and was enriched with hematopoietic stem cells (Goodell et al. Citation1996). Since then, SP cells have been shown in numerous other normal tissues, cell lines, and tumors (Challen & Little Citation2006; Hadnagy et al. Citation2006). A stem-cell enriched SP was also shown in the murine (Chen et al. Citation2005; Chen et al. Citation2009) and canine pituitary gland (van Rijn et al. Citation2012). The pituitary SP represents 1–5% of total cells in the anterior pituitary. It is mainly localized along the Rathke's cleft and has been shown to express many stem cell and progenitor cell markers. Initially, it was shown that in the murine pituitary gland, SP cells expressed the stem cell marker Stem cell antigen 1 (Sca1) (Chen et al. Citation2005). The Sca1 marker is not expressed in canine cells, so other stem cell markers, such as CD133, were used in canine SP characterization (van Rijn et al. Citation2012). Notably, further dissection of the murine SP revealed that the subpopulation not expressing high levels of Sca1 appeared to have the capacity to generate spheres and to differentiate into multiple hormone lineages (Chen et al. Citation2009; Rizzoti Citation2010). Hence, further dissection of the heterozygous SP with concomitant functional characterization is needed in order to identify which subpopulation is the pituitary progenitor cell population (Chen et al. Citation2009; Nassiri et al. Citation2013; Rizzoti Citation2010).
4.2. Sox2 expressing cells
Sox2 is a member of the SOXB1 group; SOXB1 proteins are widely expressed in the developing neural system. SOXB1 proteins are expressed in pluripotent or undifferentiated cells and their expression is downregulated when the cells are committed to their cell fate (Alatzoglou et al. Citation2009). Together with other transcription factors it maintains the pluripotent capacity of embryonic stem cells (Baltus et al. Citation2009). Sox2 is a crucial transcription factor necessary for the function of multiple stem cell populations, especially in the developing central nervous system (Alatzoglou et al. Citation2009; Nassiri et al. Citation2013). Sox 2 positive cells are also found in craniopharyngiomas, a tumor type arising from the embryonic Rathke's pouch (Garcia-Lavandeira et al. Citation2012).
Sox2 positive cells reside within Rathke's pouch during organogenesis, thereafter Sox2 expression is downregulated. However, a residual level of Sox2 positivity persists in the adult pituitary (Fauquier et al. Citation2008). These cells show several stem cell characteristics, such as sphere formation in culture and ability to differentiate into all different hormonal lineages (Fauquier et al. Citation2008; Chen et al. Citation2009). Recent studies showed that Sox2 positive cells are highly upregulated/increased in numbers during pituitary regeneration after partial destruction of pituitary PRL cells, suggesting a role for Sox2 positive cells as stem cells (Fu & Vankelecom Citation2012; Fu et al. Citation2012). However, also the Sox2 positive cell population is still heterozygous, and will need further analysis to identify stem cells within this population (Rizzoti Citation2010). Within the population of Sox2 expressing pituitary cells, those that are Sox2+/Sox9− have been proposed to be the multipotent stem cells (Fauquier et al. Citation2008).
4.3. Nestin expressing cells
Nestin is proposed as a stem cell marker for multiple tissues, including neural tissue (Rizzoti Citation2010) and pituitary tissue. During pituitary organogenesis, progenitor cells localize around Rathke's cleft. These marginal cells have been proposed as a niche of pituitary stem cells in the adult pituitary and were shown to express Nestin (Krylyshkina et al. Citation2005; Florio Citation2011; Nassiri et al. Citation2013). Also, Nestin expressing cells can differentiate into all hormonal lineages of the pituitary gland, one of the hallmarks of pituitary stem cells (Gleiberman et al. Citation2008; Florio Citation2011). However, on the basis that it is not present early on in the developing hypophysis and that it appeared in Sox-2 spheres in vitro (Fauquier et al. Citation2008), it is believed that it may play a role in the stem cell development rather than be a specific a marker for pituitary stem cells (Galichet et al. Citation2010).
5. Cancer stem cells within canine pituitary adenomas
Stem and progenitor cells are also thought to play a role in pituitary tumorigenesis (Melmed Citation2011). Similar to normal tissues, tumors display different cell populations. One population is thought to be a small group of tumor initiating cells, the so-called cancer stem cells (CSCs) (Reya et al. Citation2001). These cells are not necessarily transformed normal stem cells, but derive their name from their ability to drive the growth and development of a tumor (Florio Citation2011). It is thought that CSCs are both derived from normal stem cells and from differentiated cells (Soltysova et al. Citation2005). Stem cell fate is under strict control and deregulation of this controlled system is thought to play a role in tumorigenesis (Florio Citation2011). Furthermore, the recognition of putative stem cells in the adult pituitary gland also raised the suspicion of a role for CSCs in pituitary adenomas. It is important to further investigate the role of stem cells in pituitary adenomas, as they could represent a new target for anticancer treatment.
So far, publications on the role of stem cells in pituitary tumors are rare, especially in dogs. However, several recent studies indicate an important role for stem and progenitor cells in pituitary tumorigenesis, mostly in mouse models. Stem cell markers have been identified in Retinoblastoma (Rb) +/− mice, the most widely used mouse model to study pituitary adenomas (Gleiberman et al. Citation2008). Also, sphere-forming cells have been isolated from human pituitary adenomas (Xu et al. Citation2009) and SP isolation and characterization has been performed on human pituitary adenomas (Vankelecom & Gremeaux Citation2010). In a mouse model, Hosoyama et al. showed that a Pax7 expressing cell lineage gave rise to pituitary tumors, also suggesting a role for stem or progenitor cells (Hosoyama et al. Citation2010).
6. Summary and conclusions
From the current knowledge, it is likely that canine corticotroph adenomas contain stem cells that drive tumor growth and development. Further studies are needed to identify and characterize this cell population and to develop specific cell targeting therapeutic strategies as a new way of treating canine CD.
References
- Alatzoglou KS, Kelberman D, Dattani MT. 2009. The role of SOX proteins in normal pituitary development. J Endocrinol. 200:245–258.
- Asteria C. 2002. T-box and isolated ACTH deficiency. Eur J Endocrinol. 146:463–465.
- Baltus GA, Kowalski MP, Zhai H, Tutter AV, Quinn D, Wall D, Kadam S. 2009. Acetylation of sox2 induces its nuclear export in embryonic stem cells. Stem Cells. 27:2175–2184.
- Challen GA, Little MH. 2006. A side order of stem cells: the SP phenotype. Stem Cells. 24:3–12.
- Chen J, Gremeaux L, Fu Q, Liekens D, Van Laere S, Vankelecom H. 2009. Pituitary progenitor cells tracked down by side population dissection. Stem Cells. 27:1182–1195.
- Chen J, Hersmus N, Van Duppen V, Caesens P, Denef C, Vankelecom H. 2005. The adult pituitary contains a cell population displaying stem/progenitor cell and early embryonic characteristics. Endocrinology. 146:3985–3998.
- Daikoku S. 1983. Ontogenesis of hypothalamic immunoreactive ACTH cells in vivo and in vitro: role of rathke's pouch. Dev Biol. 97:81–88.
- Dasen JS, O’Connell SM, Flynn SE, Treier M, Gleiberman AS, Szeto DP, Hooshmand F, Aggarwal AK, Rosenfeld MG. 1999. Reciprocal interactions of Pit1 and GATA2 mediate signaling gradient–induced determination of pituitary cell types. Cell. 97:587–598.
- de Almeida João Paulo C, Sherman JH, Salvatori R, Quiñones-Hinojosa A. 2010. Pituitary stem cells: review of the literature and current understanding. Neurosurgery. 67:770–780.
- de Fornel P, Delisle F, Devauchelle P, Rosenberg D. 2007. Effects of radiotherapy on pituitary corticotroph macrotumors in dogs: a retrospective study of 12 cases. Can Vet J. 48:481–486.
- Drouin J, Lamolet B, Lamonerie T, Lanctôt C, Tremblay JJ. 1998. The PTX family of homeodomain transcription factors during pituitary developments. Mol Cell Endocrinol. 140:31–36.
- Fauquier T, Rizzoti K, Dattani M, Lovell-Badge R, Robinson ICAF. 2008. SOX2-expressing progenitor cells generate all of the major cell types in the adult mouse pituitary gland. Proc Natl Acad Sci USA. 105:2907–2912.
- Florio T. 2011. Adult pituitary stem cells: from pituitary plasticity to adenoma development. Neuroendocrinology. 94:265–277.
- Fu Q, Gremeaux L, Luque RM, Liekens D, Chen J, Buch T, Waisman A, Kineman R, Vankelecom H. 2012. The adult pituitary shows stem/progenitor cell activation in response to injury and is capable of regeneration. Endocrinology. 153:3224–3235.
- Fu Q, Vankelecom H. 2012. Regenerative capacity of the adult pituitary: Multiple mechanisms of lactotrope restoration after transgenic ablation. Stem Cells Dev. 21:3245–3257.
- Galac S, Kooistra H, Teske E, Rijnberk A. 1997. Urinary corticoid/creatinine ratios in the differentiation between pituitary-dependent hyperadrenocorticism and hyperadrenocorticism due to adrenocortical tumour in the dog. Vet Q. 19:17–20.
- Galichet C, Lovell-Badge R, Rizzoti K. 2010. Nestin-cre mice are affected by hypopituitarism, which is not due to significant activity of the transgene in the pituitary gland. PLoS ONE. 5:e11443.
- Garcia-Lavandeira M, Saez C, Diaz-Rodriguez E, Perez-Romero S, Senra A, Dieguez C, Japon MA, Alvarez CV. 2012. Craniopharyngiomas express embryonic stem cell markers (SOX2, OCT4, KLF4, and SOX9) as pituitary stem cells but do not coexpress RET/GFRA3 receptors. J Clin Endocrinol Metab. 97:E80–E87.
- Gleiberman AS, Michurina T, Encinas JM, Roig JL, Krasnov P, Balordi F, Fishell G, Rosenfeld MG, Enikolopov G. 2008. Genetic approaches identify adult pituitary stem cells. Proc Natl Acad Sci USA. 105:6332–6337.
- Goodell MA, Brose K, Paradis G, Conner AS, Mulligan RC. 1996. Isolation and functional properties of murine hematopoietic stem cells that are replicating in vivo. J Exp Med. 183:1797–1806.
- Hadnagy A, Gaboury L, Beaulieu R, Balicki D. 2006. SP analysis may be used to identify cancer stem cell populations. Exp Cell Res. 312:3701–3710.
- Halmi NS, Peterson ME, Colurso GJ, Liotta AS, Krieger DT. 1981. Pituitary intermediate lobe in dog: two cell types and high bioactive adrenocorticotropin content. Science. 211:72–74.
- Hanson JM, Hoofd MM, Voorhout G, Teske E, Kooistra HS, Meij BP. 2005. Efficacy of transsphenoidal hypophysectomy in treatment of dogs with pituitary-dependent hyperadrenocorticism. J Vet Intern Med. 19:687–694.
- Hosoyama T, Nishijo K, Garcia MM, Schaffer BS, Ohshima-Hosoyama S, Prajapati SI, Davis MD, Grant WF, Scheithauer BW, Marks DL. 2010. A postnatal Pax7 progenitor gives rise to pituitary adenomas. Genes Cancer. 1:388–402.
- Hullinger R. 1993. The endocrine system: Miller's anatomy of the dog. 3rd ed. Philadelphia, PA: WB Saunders; p. 560–567.
- Kooistra H, Galac S, Buijtels J, Meij B. 2009. Endocrine diseases in animals. Horm Res. 71:144–147.
- Kouki T. 2001. Developmental origin of the rat adenohypophysis prior to the formation of Rathke's pouch. Development. 128:959–963.
- Krylyshkina O, Chen J, Mebis L, Denef C, Vankelecom H. 2005. Nestin-immunoreactive cells in rat pituitary are neither hormonal nor typical folliculo-stellate cells. Endocrinology. 146:2376–2387.
- Lamolet B, Pulichino A, Lamonerie T, Gauthier Y, Brue T, Enjalbert A, Drouin J. 2001. A pituitary cell-restricted T box factor, tpit, activates POMC transcription in cooperation with pitx homeoproteins. Cell. 104:849–859.
- Mayer MN, Treuil PL. 2007. Radiation therapy for pituitary tumors in the dog and cat. Can Vet J. 48:316–318.
- Meij BP. 2001. Hypophysectomy as a treatment for canine and feline Cushing's disease. Vet Clin North Am Small Anim Pract. 31:1015–1041.
- Meij B, Voorhout G, Rijnberk A. 2002. Progress in transsphenoidal hypophysectomy for treatment of pituitary-dependent hyperadrenocorticism in dogs and cats. Mol Cell Endocrinol. 197:89–96.
- Melmed S. 2003. Mechanisms for pituitary tumorigenesis: the plastic pituitary. J Clin Invest. 112:1603–1618.
- Melmed S. 2011. Pathogenesis of pituitary tumors. Nat Rev Endocrinol. 7:257–266.
- Mullis PE. 2001. Transcription factors in pituitary development. Mol Cell Endocrinol. 185:1–16.
- Nassiri F, Cusimano M, Zuccato JA, Mohammed S, Rotondo F, Horvath E, Syro LV, Kovacs K, Lloyd RV. 2013. Pituitary stem cells: candidates and implications. Pituitary. 1–6.
- Pulichino A, Vallette-Kasic S, Tsai JP, Couture C, Gauthier Y, Drouin J. 2003. Tpit determines alternate fates during pituitary cell differentiation. Genes Dev. 17:738–747.
- Reya T, Morrison SJ, Clarke MF, Weissman IL. 2001. Stem cells, cancer, and cancer stem cells. Nature. 414:105–111.
- Rijnberk A, Kooistra HS. 2010. Clinical endocrinology of dogs and cats. Hannover: Schlütersche.
- Rijnberk A, Van Wees A, Mol J. 1988. Assessment of two tests for the diagnosis of canine hyperadrenocorticism. Vet Rec. 122:178–180.
- Rizzoti K. 2010. Adult pituitary progenitors/stem cells: from in vitro characterization to in vivo function. Eur J Neurosci. 32:2053–2062.
- Sasaki F, Nishioka S. 1998. Fetal development of the pituitary gland in the beagle. Anat Rec. 251:143–151.
- Scully KM, Rosenfeld MG. 2002. Pituitary development: regulatory codes in mammalian organogenesis. Science. 295:2231–2235.
- Simmons D, Voss J, Ingraham H, Holloway J, Broide R, Rosenfeld M, Swanson L. 1990. Pituitary cell phenotypes involve cell-specific pit-1 mRNA translation and synergistic interactions with other classes of transcription factors. Genes Dev. 4:695–711.
- Soltysova A, Altanerova V, Altaner C. 2005. Cancer stem cells. Neoplasma. 52:435.
- Suga H, Kadoshima T, Minaguchi M, Ohgushi M, Soen M, Nakano T, Takata N, Wataya T, Muguruma K, Miyoshi H. 2011. Self-formation of functional adenohypophysis in three-dimensional culture. Nature. 480:57–62.
- Taniguchi Y, Yasutaka S, Kominami R, Shinohara H. 2002. Proliferation and differentiation of rat anterior pituitary cells. Anat Embryol. 206:1–11.
- Treier M, Gleiberman AS, O’Connell SM, Szeto DP, McMahon JA, McMahon AP, Rosenfeld MG. 1998. Multistep signaling requirements for pituitary organogenesis in vivo. Genes Dev. 12:1691–1704.
- Treier M, O’Connell S, Gleiberman A, Price J, Szeto DP, Burgess R, Chuang PT, McMahon AP, Rosenfeld MG. 2001. Hedgehog signaling is required for pituitary gland development. Development. 128:377–386.
- van der Vlugt-Meijer RH, Voorhout G, Meij BP. 2002. Imaging of the pituitary gland in dogs with pituitary-dependent hyperadrenocorticism. Mol Cell Endocrinol. 197:81–87.
- Vankelecom H. 2007a. Stem cells in the postnatal pituitary? Neuroendocrinology. 85:110–130.
- Vankelecom H. 2007b. Non-hormonal cell types in the pituitary candidating for stem cell. Semin Cell Dev Biol. 18:559–570.
- Vankelecom H. 2010. Pituitary stem/progenitor cells: embryonic players in the adult gland? Eur J Neurosci. 32:2063–2081.
- Vankelecom H, Gremeaux L. 2010. Stem cells in the pituitary gland: a burgeoning field. Gen Comp Endocrinol. 166:478–488.
- van Rijn SJ, Gremeaux L, Riemers FM, Brinkhof B, Vankelecom H, Penning LC, Meij BP. 2012. Identification and characterisation of side population cells in the canine pituitary gland. Vet J. 192:476–482.
- Wagner J, Thomas PQ. 2007. Genetic determinants of mammalian pituitary morphogenesis. Front Biosci. 12:2085–2095.
- Willeberg P, Priester W. 1982. Epidemiological aspects of clinical hyperadrenocorticism in dogs (canine Cushing's syndrome). J Am Anim Hosp Assoc. 18:717–724.
- Wolf N, Kone A, Priestley G, Bartelmez S. 1993. In vivo and in vitro characterization of long-term repopulating primitive hematopoietic cells isolated by sequential hoechst 33342-rhodamine 123 FACS selection. Exp Hematol. 21:614.
- Xu Q, Yuan X, Tunici P, Liu G, Fan X, Xu M, Hu J, Hwang J, Farkas D, Black K. 2009. Isolation of tumour stem-like cells from benign tumours. Br J Cancer. 101:303–311.
- Yeung CM, Chan CB, Leung PS, Cheng CHK. 2006. Cells of the anterior pituitary. Int J Biochem Cell Biol. 38:1441–1049.
- Zhao L, Bakke M, Krimkevich Y, Cushman LJ, Parlow A, Camper SA, Parker KL. 2001. Steroidogenic factor 1 (SF1) is essential for pituitary gonadotrope function. Development. 128:147–154.