Abstract
Interest in mesenchymal stem cells (MSCs) both for regenerative and reparative therapies in dogs is emerging, as the current treatment options for several conditions often do not result either in the desired clinical outcome or in the patients’ return to normal function. In addition, canine MSCs have been evaluated in some experimental and preclinical studies on efficacy and safety testing of novel treatments for humans, since the dog is considered to be a superior model for humans than rodents. Although these MSCs can be derived from several sources, clinical use has favoured bone marrow and adipose tissue because of their relative ease of stem cell recovery and the minimal donor-site morbidity. Before any type of stem cell can be applied clinically, its unequivocal characterization by a set of specific functional or phenotypic markers is crucial. However, no uniform characterization criteria are available for canine MSCs so far. Moreover, although multi-lineage potential of canine MSCs has been demonstrated in a limited number of studies, research on the differentiation potential of MSCs towards tenocytes is still lacking in canine medicine. In contrast, this latter subject has been explored already in human as well as in equine medicine, demonstrating the need for a specific ‘niche’, i.e. factors with a positive influence on the MSC differentiation. Since most of these factors are still unknown regarding canine MSC, critical basic knowledge is urgently required to motivate and correctly translate the potential therapeutic applications of these stem cells in both dog and man.
Keywords:
1. Introduction
In veterinary medicine, the application of stem cells (SCs) for regenerative medicine and tissue engineering purposes has rapidly emerged both experimentally and clinically. A tissue or organ can renew itself because undifferentiated SCs can differentiate to yield the major specialized cell types of the tissue or organ (Chanda et al. Citation2010). SCs can be classified as either embryonic or adult SCs, depending on the developmental stage from which they have been obtained (Fortier Citation2005). Embryonic SCs are derived from the inner cell mass of the blastocyst, whereas adult SCs originate from tissues of endo-, meso- or ectodermal lineages (Fortier et al. Citation1998; Fortier Citation2005; De Schauwer et al. Citation2011). SCs isolated from the stroma of bone marrow (BM), more specifically the mesenchymal stem cells (MSCs), are the most frequently used adult SCs in tissue regeneration (Chanda et al. Citation2010). Therapeutic testing of these MSCs for different human conditions (cardiovascular repair, spinal cord injury, bone and cartilage repair) has involved a wide range of animal models, typically from rodent species (Barry & Murphy Citation2004; Csaki et al. Citation2007). However, preclinical efficacy and safety testing of novel treatments for humans must be evaluated using two animal species, i.e. first a rodent species (preferably rats) and second a non-rodent large animal that has closer similarities to humans (Borjesson & Peroni Citation2011). For research on MSCs for applications in humans, dogs are recognized to be superior to rodents. The similarities with regard to anatomy, pathogenesis, athletic performance and the larger joints – compared to rodents – make dogs a very attractive model species for humans as they allow a more detailed evaluation of therapeutic effects (Little & Smith Citation2008; Chu et al. Citation2010). For example, in a muscular dystrophy model, the Golden Retriever was successfully treated with mesoangioblast SC (Sampaolesi et al. Citation2006). In addition, the dog itself is frequently afflicted by bone and joint diseases. Up till now, existing treatment options for many canine orthopaedic and neurologic conditions often do not result either in the desired clinical outcome or in the patients’ return to normal function. Therefore, MSCs hold great promise in this specific field. However, only a few studies exist on the use of MSCs for bone and cartilage problems in dogs and studies on the therapeutic applications of canine MSCs for tendon/ligament problems are even lacking (Bruder et al. Citation1998; Barry & Murphy Citation2004; Black et al. Citation2007; Black et al. Citation2008; Hiyama et al. Citation2008; Guercio et al. Citation2012; Vilar et al. Citation2013). Consequently, MSCs have sometimes been implemented without demonstrating their efficacy either in vitro or in preclinical animal models (Fortier & Travis Citation2011). Several critical remarks are therefore in place when interpreting the results of MSC studies in general. The efficacy of MSC therapy can be difficult to evaluate especially when appropriate control groups are not included. Indeed, current clinical literature frequently relies on study designs that do not respond to the gold standard of evidence-based medicine, i.e. blinded randomized control trials. This set-up is difficult specifically in veterinary science because of the logistical and economical limitations associated with such a study design. Moreover, even in those studies that are properly controlled, the experimental power is often lacking because of the limited sample size and the inter-animal variability. Another complication is the fact that this treatment is often combined with diverse biological factors, including BM supernatant, autologous serum, platelet-rich plasma, etc. (Clegg & Pinchbeck Citation2011; De Schauwer et al. Citation2013). Finally, little information is available regarding short-term and long-term safety of SC therapy in veterinary medicine. Only some studies in horses and dogs investigated the possible adverse reactions, including increased thickening of the injured region after MSC injection and the presence of tumorogenicity (Smith et al. Citation2003; Guercio et al. Citation2012). Specifically for canine medicine many factors of MSC-based therapies are still unknown. For instance, the ideal cell transplantation number has not yet been reported, since almost no dose–response studies have been carried out so far (Fortier & Travis Citation2011; Kisiel et al. Citation2012). Conventionally, high numbers of MSCs have been used in both veterinary and human cell-based treatments (Kisiel et al. Citation2012). In human medicine, 1–5 million cells/kg are administered intravenously or directly into the tissue (Wagner et al. Citation2009). To obtain cell numbers of this magnitude, culture expansion would be required (Kisiel et al. Citation2012). Also, other fundamental questions remain unanswered regarding the cell yield per gram of donor tissue, the optimal tissue source for each clinical application, the preferred administration route, i.e. either intravenously or locally to the site of damage, whether scaffolds are necessary or not, the timing of administration and the use of autologous versus allogeneic MSCs (Fortier & Travis Citation2011; Kisiel et al. Citation2012).
In summary, critical basic knowledge regarding canine MSC is urgently required not only to motivate, but also to correctly translate the potential therapeutic applications of these SCs in both veterinary and human medicine.
2. Sources of canine mesenchymal stem cells
Depending on their therapeutic indication, MSCs can be obtained from various sources: mesodermal tissues (BM, trabecular bone, synovium, cartilage, fat, muscle and tonsil), endodermal tissues (thymus and liver) and ectoderm-derived tissues (skin, hair follicle, dura mater and dental pulp) (Phinney & Prockop Citation2007). Most canine experimental and clinical studies use BM-derived and adipose tissue-derived MSCs, since these are best characterized and are relatively comparable (Fortier & Travis Citation2011; Kisiel et al. Citation2012; Takemitsu et al. Citation2012). A distinction should be made between non-expanded, patient-side, autologous products such as BM concentrate and adipose-derived stromal vascular fraction on the one hand and culture-expanded, autologous or allogeneic products including BM-MSCs and adipose tissue-derived MSCs on the other hand (Borjesson & Peroni Citation2011). Advantages of the non-expanded cell products include the heterogenicity of the cell populations and the possibility of directly injecting the product without a preceding cell culture step (Borjesson & Peroni Citation2011; Fortier & Travis Citation2011). Culture-expanded cells are highly enriched in MSCs. However, MSC expansion can take two to three weeks, which precludes their use for acute injuries (Borjesson & Peroni Citation2011). BM in dogs can be easily and relatively non-invasively obtained and is usually aspirated either from the proximal humerus, the proximal femur or the tuber coxae using a 15- or 16-gauge Jamshidi biopsy needle (Bruder et al. Citation1998; Crovace et al. Citation2008; Fortier & Travis Citation2011) (). Furthermore, BM-MSCs have a greater capacity – compared to other types of MSCs – to differentiate into tissue originating from the musculoskeletal system, which is currently the most important indication for regenerative medicine in dogs (Hodgkiss-Geere et al. Citation2012b). Alternatively, MSCs derived from adipose tissue have been recently considered a highly attractive option compared to BM-MSCs, mainly because of the ease of tissue collection and the availability of adipose tissue at various sites in the body (Stewart & Stewart Citation2011). In dogs, adipose tissue can be collected by either a simple adapted liposuction procedure, or through biopsies or in routine veterinary surgery procedures (Vieira et al. Citation2010). Furthermore, high initial cell yields, robust in vitro proliferative capacity and higher in vitro immunomodulating characteristics are additional benefits of adipose tissue (Stewart & Stewart Citation2011).
Figure 1. Overview of accessible puncture sites of BM in the dog. 1: Approach of the proximal part of the femur. The needle is placed in the trochanteric fossa between the lesser and greater trochanters (Jamshidi biopsy needle). 2a: Dorsal approach of the wing of the ilium at the dorsal crest. The needle is directed almost parallel to the long axis of the iliac wing (Jamshidi biopsy needle). For this approach the dog is preferably restrained in sternal recumbency or standing. 2b: Lateral approach of the iliac wing approximately 1 cm below the dorsal crest. The direction of the needle is perpendicular to the iliac wing (Jamshidi biopsy needle). For this approach the dog is restrained in lateral recumbency. 3: Approach of the proximal humerus, just below the greater tubercle of the humerus. The needle is inserted at an angle of approximately 45°–90° from a line parallel to the long axis of the humerus (Jamshidi biopsy needle).
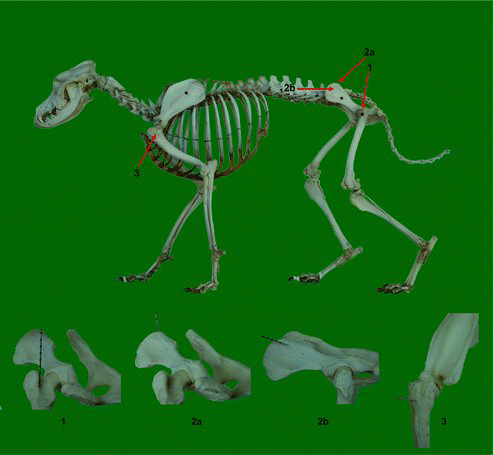
The MSC isolation protocol is similar to that used for other species and has been described by several groups (Martinello et al. Citation2011; Vieira et al. Citation2010; Mokbel et al. Citation2011; Guercio et al. Citation2012; Kisiel et al. Citation2012; Kang et al. Citation2012; Hodgkiss-Geere et al. Citation2012b).
3. Characterization of canine mesenchymal stem cells
Human MSCs are characterized by minimally three criteria according to the International Society for Cellular Therapy: (1) plastic adherence when maintained in standard culture conditions, (2) expression of a specific cell surface antigen marker panel (CD73, CD90 and CD105) and absence of other cell surface markers (CD11b, CD14, CD19, CD29α, CD34, CD45 and HLA-DE) to unequivocally distinguish MSCs from endothelial, epithelial and muscle cells and (3) at least tri-lineage (osteo-, chondro- and adipogenic) differentiation potential (Dominici et al. Citation2006; De Schauwer et al. Citation2011). However, in marked contrast to human MSCs, so far no uniform characterization criteria are available for MSCs from animal origin in general and from canine origin in specific (Jiang et al. Citation2002; Dominici et al. Citation2006; De Schauwer et al. Citation2011).
Nevertheless, some data are available for the dog, using the same criteria as for man (Martinello et al. Citation2011; Vieira et al. Citation2010; Takemitsu et al. Citation2012). Indeed, undifferentiated canine MSCs can be characterized morphologically, immunophenotypically and by their gene expression (). Morphologically, they are identified as spindle-shaped cells that grow on plastic in a monolayer and show varying cellular morphology, from very slender and elongated towards more cuboidal with shorter cytoplasmatic extensions (Csaki et al. Citation2007; De Schauwer et al. Citation2011). Immunophenotyping is performed by flow cytometry using specific cross-reaction antibodies to indicate surface antigen expression, which unfortunately has not been universally well characterized (Dominici et al. Citation2006; De Schauwer et al. Citation2012). Several studies have demonstrated the simultaneous expression of several cell surface markers (CD29, CD73, CD105 and CD44, Thy-1 CD90 glycoprotein and MHC-I, i.e. positive markers) in the absence of hematopoietic receptors (CD34, CD45, CD14, CD117), embryonic SC-specific markers (SSEA-3, SSEA-4, TRA-1-60, TRA-1-81), MHC-II and transmembrane glycoprotein CD146, i.e. negative markers, either in BM-derived or in adipose-derived canine MSCs (Csaki et al. Citation2007; Neupane et al. Citation2008; Vieira et al. Citation2010; Kisiel et al. Citation2012; Kang et al. Citation2012; Takemitsu et al. Citation2012) (). Characterization of these MSCs at the mRNA level is a valuable alternative when no (cross-reacting) monoclonal antibodies are available to characterize them at the protein level (De Schauwer et al. Citation2011). In human SC research, gene expression is frequently used to characterize undifferentiated MSCs and the most commonly studied genes with positive expression are the pluripotency-associated transcription factors NanoG, Oct4 and Sox2 (Ginis et al. Citation2004). In line with human MSCs, canine MSCs also appear to be positive for these three gene expression markers (Neupane et al. Citation2008; Guercio et al. Citation2012; Kisiel et al. Citation2012; Takemitsu et al. Citation2012) ().
Table 1. Overview of the current data on canine mesenchymal stem cells.
The capacity of MSCs to differentiate into multiple lineages, and more specifically their osteo-, chondro- and adipogenic potential, has been described as the unequivocal characterization of MSCs (Dominici et al. Citation2006; De Schauwer et al. Citation2011). The ability of this tri-lineage differentiation potential has also been demonstrated by three groups for canine MSCs, both obtained from BM as well as from adipose tissue as sources (Csaki et al. Citation2007; Vieira et al. Citation2010; Guercio et al. Citation2012) (). However, two other studies have reported on the encountered difficulties or even the inability of isolated and cultured presumed canine MSCs to differentiate towards the chondro- or adipogenic lineage (Neupane et al. Citation2008; Seo et al. Citation2009). Apparently, the commonly used induction media for adipogenic differentiation of human MSCs need significant optimization for the canine species (Neupane et al. Citation2008). More specifically, rabbit serum was found to be critical to induce adipogenesis in canine MSCs (Neupane et al. Citation2008). One study reported on the unsuccessful differentiation of MSCs into the chondrogenic lineage based on morphological and histochemical assessments, despite positive results reported by other studies (Csaki et al. Citation2007; Kisiel et al. Citation2012). Therefore, mRNA expression of chondrocyte markers can be especially useful to confirm differentiation towards chondrogenesis when morphological and histochemical results remain inconclusive (Vieira et al. Citation2010).
Table 2. Overview of the current data on differentiation of canine mesenchymal stem cells.
Osteogenesis is demonstrated by the polygonal appearance of the differentiated osteoblast containing nodular aggregates that stain positively with von Kossa and by the mRNA expression of osteoblast markers. Runx2, which is a specific transcription factor for early osteogenesis, Osteonectin, a calcium-binding glycoprotein that interacts with hydroxyapatite and initiates the mineralization of cartilage and bone, Osterix and BSP can be found in canine osteo-induced MSCs (Csaki et al. Citation2007; Neupane et al. Citation2008; Vieira et al. Citation2010; Kisiel et al. Citation2012) ().
Chondrogenesis is characterized by the presence of three-dimensional aggregates, which stain positive for alcian blue indicating the presence of sulphated proteoglycans. Expression of COL2A, Aggrecan, COMP, COL10A and Sox9 can be detected in the differentiated cells (Csaki et al. Citation2007; Neupane et al. Citation2008; Vieira et al. Citation2010) ().
Adipogenesis is demonstrated by the appearance of round-shaped cells with Oil Red O staining of lipid droplets (Vieira et al. Citation2010). Expression of PPARγ2, CEBPα, FABP4 and LPL is specific for adipo-induced cells (Csaki et al. Citation2007; Neupane et al. Citation2008; Vieira et al. Citation2010) ().
4. Differentiation of characterized canine MSCs into alternative lineages
Besides the osteo-, chondro- and adipogenic differentiation potential of canine MSCs, differentiation towards myo-, neuro- and cardiogenic lineages in specific induction media has been investigated (Kamishina et al. Citation2006; Seo et al. Citation2009; Vieira et al. Citation2010; Hodgkiss-Geere et al. Citation2012b; Martinello et al. Citation2011) (). Canine MSCs isolated from adipose tissue were able to differentiate towards myocytes, morphologically characterized by myoblast alignments with an initial fusion and formation of multinucleated structures. This myogenic differentiation was confirmed by the expression of myosin, myogenin, dystrophin, MyoD and desmin (Martinello et al. Citation2011; Vieira et al. Citation2010). BM-derived canine MSCs were not able to differentiate into cardiomyocytes, since rhythmic beating and expression of cardiac structural and functional genes were not convincingly seen (Hodgkiss-Geere et al. Citation2012b). This is in contrast with the successful cardiac differentiation of both rodent and human MSCs (Hodgkiss-Geere et al. Citation2012b). Differentiation towards neuronal cells has been described for both umbilical cord blood-derived and BM-derived canine MSCs (Kamishina et al. Citation2006; Seo et al. Citation2009). Neuronal cells can be characterized by a change from fibroblastic towards neuron-like morphological features with multiple branching processes of various lengths (Kamishina et al. Citation2006). Moreover, neuronal-associated protein markers (GFAP, Tuj-1, NF160, Nestin, MAP-2) were evidently expressed. This demonstrates the ability of canine MSCs to trans-differentiate into multiple germ layers, since neuronal cells belong to the ectoderm in contrast to the two other lineages, which belong to the mesoderm (Kamishina et al. Citation2006). As such, this finding underlines the plasticity of adult SC. While the differentiation potential of MSCs towards tenocytes is still being investigated in both human and equine medicine, unfortunately no studies are available in canine medicine.
5. Therapeutic applications of canine mesenchymal stem cells
The increased occurrence in both dogs and humans of, e.g. musculoskeletal diseases with osteochondrotic articular cartilage defects, for which current therapeutic strategies show minimal effectiveness, has accelerated the isolation and use of canine MSCs.
Initially, the main purpose of these canine MSCs was the conduction of experimental animal studies in which the dog served as a preclinical model for humans. Already in 1998, Bruder et al. investigated the effect of cultured autologous BM-MSCs on the healing of created defects in canine femurs. The group treated with a carrier loaded with MSCs showed substantial new bone formation, while the untreated femurs demonstrated atrophic non-union. It was stated that this technology could be extrapolated to man, eliciting the healing of bone defects in humans by using cells from the BM aspirated from the iliac crest of the patient. This may hold potential to provide an alternative strategy to autologous bone-grafting (Bruder et al. Citation1998).
A far more recent example is that of an experimental study involving a canine disc degeneration model for low back pain, which is a frequently diagnosed disease in man and is considered a major social problem (Hiyama et al. Citation2008). It is believed to be caused by disc degeneration, involving nucleus pulposus cells, although details of the pathogenesis of this condition are not clearly identified yet. So far, almost all studies on disc degeneration had been performed in small laboratory species whose nucleus pulposus cells differ from those of humans and larger animals. Only two recent studies have investigated degeneration of the intervertebral disc in both chondrodystrophic and non-chondrodystrophic dogs by studying the biomolecular signalling profiles associated with notochordal cell maintenance in the nucleus pulposus and replacement of these cells by chondrocyte-like cells (Smolders et al. Citation2012, Citation2013). To investigate the future usefulness of MSCs transplantation in humans, its effect has therefore been studied in dogs (Hiyama et al. Citation2008). Dogs in the latter study underwent a nucleotomy followed by injection of BM-MSCs and showed significant improvement compared with the nucleotomy-only group. Macroscopically, MSCs-treated dogs looked similar to the control group without a nucleotomy. However, they lacked the narrowed disc space and connective tissue invasion seen in the nucleotomy-only group. It was therefore prudently concluded that MSCs transplantation was partially effective in inhibiting disc degeneration. Another group studied the effects of MSCs-based therapy on induced severe spinal cord injuries in dogs (Jung et al. Citation2009). In 30 beagle dogs, lesions of the spinal cord were created and afterwards 10 dogs received autologous MSCs intrathecally, 10 dogs received allogenic MSCs intrathecally and 10 dogs were not treated. Both autologous and allogenic groups showed improvement in their pelvic limbs already in the first week after treatment in comparison to the control group. It was therefore stated that autologous and allogenic MSCs transplantation can be clinically useful therapeutic approaches for injuries of the spinal cord.
A third example is that of a recent study on the effect of direct intra-articular injection of autologous undifferentiated MSCs in healing of a partial thickness cartilage defect induced in experimental dogs (Mokbel et al. Citation2011). In 32 cases a cartilage lesion was induced: 8 cases did not receive treatment, 12 cases received MSCs intra-articularly one day after the created lesion and another 12 cases were injected with MSCs one month after the lesion. Compared to the control group, the two cell-treated groups recovered significantly both clinically and histologically with the injected MSCs incorporated within the newly formed cartilage. It was concluded that the use of autologous MSCs injected intra-articularly is a viable option for treating partial cartilage defects.
A fourth example involves the regeneration of muscular tissues, particularly cardiomyocytes, using canine MSCs. This evaluation has been performed in one experimental study in dogs and then successfully attempted in human patients with myocardial infarcts (Stamm et al. Citation2003; Memon et al. Citation2005). Unlike humans, dogs do not suffer naturally occurring clinical ischaemic heart disease and hence the relevance of this research for clinical veterinary medicine is more limited. Nevertheless, other cardiac diseases such as canine dilated cardiomyopathy cause significant morbidity and mortality, to over 50% of mortalities in the Cavalier King Charles Spaniel (Hodgkiss-Geere et al. Citation2012a). Canine MSCs therapy might thus provide a curative solution for the latter disease (Hodgkiss-Geere et al. Citation2012a).
Only a few studies on regenerative therapy in dogs have been performed and these mainly focused on the osteo- and chondrogenic potential to restore bone and cartilage defects and on inflammatory diseases, including osteoarthritis (Csaki et al. Citation2007; Crovace et al. Citation2008; Guercio et al. Citation2012). Black et al. Citation(2007, Citation2008), Vilar et al. Citation(2013) and Guercio et al. Citation(2012) evaluated the effects of canine MSCs on dogs affected by osteoarthritis of the coxofemoral joints and osteoarthritis of the humeroradial joints (Black et al. Citation2007; Black et al. Citation2008; Guercio et al. Citation2012; Vilar et al. Citation2013). The latter research group demonstrated considerable improvement in lameness and fewer signs of pain on range of motion after one month of treatment with MSCs in three dogs assessed by both a veterinarian and the owner, but no control group was used (Guercio et al. Citation2012). The two former research groups reported comparable results for 18, 14 and 8 MSCs-treated dogs, respectively, after a period of three or six months, compared to a placebo group that failed to improve (Black et al. Citation2007; Black et al. Citation2008; Vilar et al. Citation2013). One other study dating also from 2008 demonstrated the successful clinical application of BM-derived canine MSCs for the treatment of some orthopaedic lesions in dogs: bone cyst of the glenoid, non-union of the tibia and femur, lengthening of the radius, large bone defect of the distal radius and Legg–Perthés–Calvé disease (Crovace et al. Citation2008).
In marked contrast, regenerative MSCs-based therapy for tendon/ligament lesions – either traumatic or degenerative – has not been attempted at all in dogs, while this research field is extensively explored in both humans and horses (Park et al. Citation2010; Alberton et al. Citation2012; De Schauwer et al. Citation2013). However, many of the tendon/ligament injuries, i.e. partial/complete ruptures of the anterior cruciate ligament or the Achilles tendon and tendon/ligament-to-bone injuries, i.e. tennis’ and golfers’ elbow or flexor enthesopathy described in humans are also frequently diagnosed in dogs (Ralphs & Whitney Citation2002; Corr et al. Citation2010; de Bakker et al. Citation2013). Since healing of tendon/ligament lesions involves formation of extensive scar tissue leading to impaired elasticity resulting in reduced performance and substantial risk of re-injury, many of the conventional therapies have failed (Richardson et al. Citation2007; Smith Citation2008). This supports the need to further search for novel treatments, which should aim at restoring functionality and regenerating a tissue as close to the tendon as possible (Richardson et al. Citation2007). Several studies in horses reported on a positive therapeutic effect of equine MSCs for the treatment of tendon lesions (Pacini et al. Citation2007; Nixon et al. Citation2008; Alves et al. Citation2011; Godwin et al. Citation2012). These MSCs were applied either in combination with a tendon-inducing niche, containing factors such as bone morphogenetic proteins (BMPs), or in the absence of tendon-inducing media (Nixon et al. Citation2008; Violini et al. Citation2009).
6. Conclusion and future prospects
In conclusion, the use of MSCs as an alternative treatment option for several canine diseases, such as osteoarthritis, certainly has potential. Although experimental, some preclinical and clinical studies with canine MSCs exist, still many aspects on their mechanism of action are not fully understood. First of all, before any type of SC can be applied clinically, its unequivocal characterization by a set of specific functional or phenotypic markers is crucial. Nevertheless, in marked contrast to human MSCs no uniform characterization criteria are available for canine MSCs so far. While isolation and in vitro differentiation of canine MSCs have been reported in several studies, only a scarce number of studies have attempted to identify a panel of cell surface markers and of mRNA expression profiles for these SCs. In addition, the mechanism of MSCs-based therapy in tissue repair remains partly unknown. Do MSCs either differentiate into a tissue-specific cell line, or improve tissue repair through secretion of immunomodulatory and bioactive factors, or does a combination of both mechanisms of action occur? Another major concern is that SC therapies in veterinary medicine are not yet rigorously supervised by regulatory agencies in many countries. This has led to the implementation of therapies that have not proven their efficacy either in vitro or in preclinical animal studies. On the other hand, most veterinary clinical studies report on BM-derived MSCs, which have been expanded in vitro prior to their in vivo use. This sequential procedure provides a certain degree of quality control and the expectation that the obtained effect of the treatment is actually caused by the administered MSCs.
Finally, MSCs-based therapies in dogs have mainly been focusing on bone and cartilage repair and inflammatory diseases such as osteoarthritis. Nevertheless, an important share of musculoskeletal problems in dogs consists of tendon/ligament injuries, which are comparable to man. Since natural repair mechanisms often do not deliver functional recovery or current therapeutic strategies show minimal effectiveness, MSC-based therapies can be considered as potentially revolutionary in this field. Therefore, a challenging future prospect involves the research on MSC-based therapy for these canine tendon/ligament injuries. Since dogs are considered to be a superior animal model for humans, not only dogs, but also man can benefit from state-of-the-art research in this exciting field.
References
- Alberton P, Popov C, Pragert M, Kohler J, Shukunami C, Schieker M, Docheva D. 2012. Conversion of human bone marrow-derived mesenchymal stem cells into tendon progenitor cells by ectopic expression of scleraxis. Stem Cells Dev. Apr 10;21:846–858.
- Alves AG, Stewart AA, Dudhia J, Kasashima Y, Goodship AE, Smith RK. 2011. Cell-based therapies for tendon and ligament injuries. Vet Clin North Am Equine Pract. Aug;27:315–333.
- Barry FP, Murphy JM. 2004. Mesenchymal stem cells: clinical applications and biological characterization. Int J Biochem Cell Biol. Apr;36:568–584.
- Black LL, Gaynor J, Adams C, Dhupa S, Sams AE, Taylor R, Harman S, Gingerich DA, Harman R. 2008. Effect of intraarticular injection of autologous adipose-derived mesenchymal stem and regenerative cells on clinical signs of chronic osteoarthritis of the elbow joint in dogs. Vet Ther. 9:192–200.
- Black LL, Gaynor J, Gahring D, Adams C, Aron D, Harman S, Gingerich DA, Harman R. 2007. Effect of adipose-derived mesenchymal stem and regenerative cells on lameness in dogs with chronic osteoarthritis of the coxofemoral joints: a randomized, double-blinded, multicenter, controlled trial. Vet Ther. 8:272–284.
- Borjesson DL, Peroni JF. 2011. The regenerative medicine laboratory: facilitating stem cell therapy for equine disease. Clin Lab Med. Mar;31:109–123.
- Bruder SP, Kraus KH, Goldberg VM, Kadiyala S. 1998. The effect of implants loaded with autologous mesenchymal stem cells on the healing of canine segmental bone defects. J Bone Joint Surg Am. Jul;80:985–996.
- Chanda D, Kumar S, Ponnazhagan S. 2010. Therapeutic potential of adult bone marrow-derived mesenchymal stem cells in diseases of the skeleton. J Cell Biochem. Oct 1;111:249–257.
- Chu CR, Szczodry M, Bruno S. 2010. Animal models for cartilage regeneration and repair. Tissue Eng Part B Rev. Feb;16:105–115.
- Clegg PD, Pinchbeck GL. 2011. Evidence-based medicine and stem cell therapy: how do we know such technologies are safe and efficacious? Vet Clin North Am Equine Pract. 27:373–382.
- Corr SA, Draffan D, Kulendra E, Carmichael S, Brodbelt D. 2010. Retrospective study of Achilles mechanism disruption in 45 dogs. Vet Rec. Sep 11;167:407–411.
- Crovace A, Favia A, Lacitignola L, Di Comite MS, Staffieri F, Francioso E. 2008. Use of autologous bone marrow mononuclear cells and cultured bone marrow stromal cells in dogs with orthopaedic lesions. Vet Res Commun. Sep;32:S39–S44.
- Csaki C, Matis U, Mobasheri A, Ye H, Shakibaei M. 2007. Chondrogenesis, osteogenesis and adipogenesis of canine mesenchymal stem cells: a biochemical, morphological and ultrastructural study. Histochem Cell Biol. Dec;128:507–520.
- de Bakker E, Gielen I, Saunders JH, Polis I, Vermeire S, Peremans K, Dewulf J, van Bree H, Van Ryssen B. 2013. Primary and concomitant flexor enthesopathy of the canine elbow. Vet Comp Orthop Traumatol. Nov 12;26:425–434. doi:10.3415/VCOT-12-02-0025
- De Schauwer C, Meyer E, Van de Walle GR, Van Soom A. 2011. Markers of stemness in equine mesenchymal stem cells: a plea for uniformity. Theriogenology. 75:1431–1443.
- De Schauwer C, Piepers S, Van de Walle GR, Demeyere K, Hoogewijs MK, Govaere JL, Braeckmans K, Van Soom A, Meyer E. 2012. In search for cross-reactivity to immunophenotype equine mesenchymal stromal cells by multicolor flow cytometry. Cytom part A. 81A:312–323.
- De Schauwer C, Van de Walle GR, Van Soom A, Meyer E. 2013. Mesenchymal stem cell therapy in horses: useful beyond orthopedic injuries? Vet Q. May 22. doi:10.1080/01652176.2013.800250
- Dominici M, Le Blanc K, Mueller I, Slaper-Cortenbach I, Marini F, Krause D, Deans R, Keating A, Prockop D, Horwitz E. 2006. Minimal criteria for defining multipotent mesenchymal stromal cells. The International Society for Cellular Therapy position statement. Cytotherapy. 8:315–317.
- Fortier LA. 2005. Stem cells: classifications, controversies, and clinical applications. Vet Surg. Sep–Oct;34:415–423.
- Fortier LA, Nixon AJ, Williams J, Cable CS. 1998. Isolation and chondrocytic differentiation of equine bone marrow-derived mesenchymal stem cells. Am J Vet Res. Sep;59:1182–1187.
- Fortier LA, Travis AJ. 2011. Stem cells in veterinary medicine. Stem Cell Res Ther. 2:9. doi:10.1186/scrt50
- Ginis I, Luo Y, Miura T, Thies S, Brandenberger R, Gerecht-Nir S, Amit M, Hoke A, Carpenter MK, Itskovitz-Eldor J, Rao MS. 2004. Differences between human and mouse embryonic stem cells. Dev Biol. May 15;269:360–380.
- Godwin EE, Young NJ, Dudhia J, Beamish IC, Smith RK. 2012. Implantation of bone marrow-derived mesenchymal stem cells demonstrates improved outcome in horses with overstrain injury of the superficial digital flexor tendon. Equine Vet J. Jan;44:25–32.
- Guercio A, Di Marco P, Casella S, Cannella V, Russotto L, Purpari G, Di Bella S, Piccione G. 2012. Production of canine mesenchymal stem cells from adipose tissue and their application in dogs with chronic osteoarthritis of the humeroradial joints. Cell Biol Int. Feb;36:189–194.
- Hiyama A, Mochida J, Iwashina T, Omi H, Watanabe T, Serigano K, Tamura F, Sakai D. 2008. Transplantation of mesenchymal stem cells in a canine disc degeneration model. J Orthop Res. May;26:589–600.
- Hodgkiss-Geere HM, Argyle DJ, Corcoran BM, Whitelaw B, Milne E, Bennett D, Argyle SA. 2012a. Characterisation and cardiac directed differentiation of canine adult cardiac stem cells. Vet J. Feb;191:176–182.
- Hodgkiss-Geere HM, Argyle DJ, Corcoran BM, Whitelaw B, Milne E, Bennett D, Argyle SA. 2012b. Characterisation and differentiation potential of bone marrow derived canine mesenchymal stem cells. Vet J. Dec;194:361–368.
- Jiang Y, Vaessen B, Lenvik T, Blackstad M, Reyes M, Verfaillie CM. 2002. Multipotent progenitor cells can be isolated from postnatal murine bone marrow, muscle, and brain. Exp Hematol. Aug;30:896–904.
- Jung DI, Ha J, Kang BT, Kim JW, Quan FS, Lee JH, Woo EJ, Park HM. 2009. A comparison of autologous and allogenic bone marrow-derived mesenchymal stem cell transplantation in canine spinal cord injury. J Neurol Sci. Oct 15;285:67–77.
- Kamishina H, Deng J, Oji T, Cheeseman JA, Clemmons RM. 2006. Expression of neural markers on bone marrow-derived canine mesenchymal stem cells. Am J Vet Res. Nov;67:1921–1928.
- Kang BJ, Ryu HH, Park SS, Koyama Y, Kikuchi M, Woo HM, Kim WH, Kweon OK. 2012. Comparing the osteogenic potential of canine mesenchymal stem cells derived from adipose tissues, bone marrow, umbilical cord blood, and Wharton's jelly for treating bone defects. J Vet Sci. Sep;13:299–310.
- Kisiel AH, McDuffee LA, Masaoud E, Bailey TR, Esparza Gonzalez BP, Nino-Fong R. 2012. Isolation, characterization, and in vitro proliferation of canine mesenchymal stem cells derived from bone marrow, adipose tissue, muscle, and periosteum. Am J Vet Res. Aug;73:1305–1317.
- Little CB, Smith MM. 2008. Animal models of osteoarthritis. Curr Rheumatol Rev.4:1–8.
- Martinello T, Bronzini I, Maccatrozzo L, Mollo A, Sampaolesi M, Mascarello F, Decaminada M, Patruno M. 2011. Canine adipose-derived-mesenchymal stem cells do not lose stem features after a long-term cryopreservation. Res Vet Sci. Aug;91:18–24.
- Memon IA, Sawa Y, Miyagawa S, Taketani S, Matsuda H. 2005. Combined autologous cellular cardiomyoplasty with skeletal myoblasts and bone marrow cells in canine hearts for ischemic cardiomyopathy. J Thorac Cardiovasc Surg. Sep;130:646–653.
- Mokbel A, El-Tookhy O, Shamaa AA, Sabry D, Rashed L, Mostafa A. 2011. Homing and efficacy of intra-articular injection of autologous mesenchymal stem cells in experimental chondral defects in dogs. Clin Exp Rheumatol. 29:275–284.
- Neupane M, Chang CC, Kiupel M, Yuzbasiyan-Gurkan V. 2008. Isolation and characterization of canine adipose-derived mesenchymal stem cells. Tissue Eng Part A. Jun;14:1007–1015.
- Nixon AJ, Dahlgren LA, Haupt JL, Yeager AE, Ward DL. 2008. Effect of adipose-derived nucleated cell fractions on tendon repair in horses with collagenase-induced tendinitis. Am J Vet Res. Jul;69:928–937.
- Pacini S, Spinabella S, Trombi L, Fazzi R, Galimberti S, Dini F, Carlucci F, Petrini M. 2007. Suspension of bone marrow-derived undifferentiated mesenchymal stromal cells for repair of superficial digital flexor tendon in race horses. Tissue Eng. Dec;13:2949–2955.
- Park A, Hogan MV, Kesturu GS, James R, Balian G, Chhabra AB. 2010. Adipose-derived mesenchymal stem cells treated with growth differentiation factor-5 express tendon-specific markers. Tissue Eng Part A. Sep;16:2941–2951.
- Phinney DG, Prockop DJ. 2007. Concise review: mesenchymal stem/multipotent stromal cells: the state of transdifferentiation and modes of tissue repair–current views. Stem Cells. Nov;25:2896–2902.
- Ralphs SC, Whitney WO. 2002. Arthroscopic evaluation of menisci in dogs with cranial cruciate ligament injuries: 100 cases (1999-2000). J Am Vet Med Assoc. Dec 1;221:1601–1604.
- Richardson LE, Dudhia J, Clegg PD, Smith R. 2007. Stem cells in veterinary medicine - attempts at regenerating equine tendon after injury. Trends Biotechnol. 25:409–416.
- Sampaolesi M, Blot S, D’Antona G, Granger N, Tonlorenzi R, Innocenzi A, Mognol P, Thibaud JL, Galvez BG, Barthelemy I, et al. 2006. Mesoangioblast stem cells ameliorate muscle function in dystrophic dogs. Nature. Nov 30;444:574–579.
- Seo MS, Jeong YH, Park JR, Park SB, Rho KH, Kim HS, Yu KR, Lee SH, Jung JW, Lee YS, Kang KS. 2009. Isolation and characterization of canine umbilical cord blood-derived mesenchymal stem cells. J Vet Sci. Sep;10:181–187.
- Smith RK. 2008. Mesenchymal stem cell therapy for equine tendinopathy. Disabil Rehabil. 30:1752–1758.
- Smith RK, Korda M, Blunn GW, Goodship AE. 2003. Isolation and implantation of autologous equine mesenchymal stem cells from bone marrow into the superficial digital flexor tendon as a potential novel treatment. Equine Vet J. Jan;35:99–102.
- Smolders LA, Meij BP, Onis D, Riemers FM, Bergknut N, Wubbolts R, Grinwis GCM, Houweling M, Groot Koerkamp MJA, van Leenen D, et al. 2013. Gene expression profiling of early intervertebral disc degeneration reveals a down-regulation of canonical Wnt signaling and caveolin-1 expression: implications for development of regenerative strategies. Arthritis Res Ther. Jan;15:R23.
- Smolders LA, Meij, BP, Riemers FM, Licht R, Wubbolts R, Heuvel D, Grinwis GCM, Vernooij HCM, Hazewinkel HAW, Penning LC, Tryfonidou MA. 2012. Canonical Wnt signaling in the notochordal cell is upregulated in early intervertebral disk degeneration. J Orthop Res. Jun;30:950–957.
- Stamm C, Westphal B, Kleine HD, Petzsch M, Kittner C, Klinge H, Schumichen C, Nienaber CA, Freund M, Steinhoff G. 2003. Autologous bone-marrow stem-cell transplantation for myocardial regeneration. Lancet. Jan 4;361:45–46.
- Stewart MC, Stewart AA. 2011. Mesenchymal stem cells: characteristics, sources, and mechanisms of action. Vet Clin North Am Equine Pract. Aug;27:243–261.
- Takemitsu H, Zhao D, Yamamoto I, Harada Y, Michishita M, Arai T. 2012. Comparison of bone marrow and adipose tissue-derived canine mesenchymal stem cells. BMC Vet Res. 8:150.
- Vieira NM, Brandalise V, Zucconi E, Secco M, Strauss BE, Zatz M. 2010. Isolation, characterization, and differentiation potential of canine adipose-derived stem cells. Cell Transplant. 19:279–289.
- Vilar JM, Morales M, Santana A, Spinella G, Rubio M, Cuervo B, Cugat R, Carrillo JM. 2013. Controlled, blinded force platform analysis of the effect of intraarticular injection of autologous adipose-derived mesenchymal stem cells associated to PRGF-Endoret in osteoarthritic dogs. BMC Vet Res. 9:131.
- Violini S, Ramelli P, Pisani LF, Gorni C, Mariani P. 2009. Horse bone marrow mesenchymal stem cells express embryo stem cell markers and show the ability for tenogenic differentiation by in vitro exposure to BMP-12. BMC Cell Biol. 10:29.
- Wagner J, Kean T, Young R, Dennis JE, Caplan AI. 2009. Optimizing mesenchymal stem cell-based therapeutics. Curr Opin Biotechnol. Oct;20:531–536.