ABSTRACT
This review converses the Zika virus which has attained global concern due to its rapid pandemic potential and impact on humans. Though Zika virus was first isolated in 1947, till the recent large-scale outbreak which occurred in Micronesia, in 2007, the virus was placed into the innocuous pathogen category. The World Health Organization on 1 February 2016 declared it as a ‘Public Health Emergency of International Concern.’ Of the note, American as well as Pacific Island strains/isolates is relatively closer to Asian lineage strains. The African and American strains share more than 87.5% and 95% homologies with Asian strains/isolates, respectively. Asian strains form independent clusters, except those isolated from China, suggesting relatively more diversity than African strains. Prevention and control are mainly aimed at the vector population (mosquitoes) with Aedes aegypti being the main species. Surveys in Africa and Asia indicated seropositivity in various animal species. However, so far its natural reservoir is unknown. There is an urgent need to understand why Zika virus has shifted from being a virus that caused mild illness to unforeseen birth defects as well as autoimmune-neurological problems. Unfortunately, an effective vaccine is not available yet. Availability of cryo-electron microscopy based on 3.8 Å resolution revealing mature Zika virus structure and the probable virus attachment site to host cell would provide critical insights into the development of antiviral treatments and vaccines.
1. Introduction
The geographic modulations, in terms of population and climate dynamics, have become a potential threat to the global community health in past, in particular due to uprising infection rates of mosquito-borne diseases such as Malaria, Chikungunya, Dengue, West Nile, and Zika (Chen & Wilson Citation2010; Dhiman et al. Citation2010; Dhama, Tiwari, et al. Citation2013; Lee et al. Citation2013; Hubálek et al. Citation2014; Carneiro & Travassos Citation2016; Fauci & Morens Citation2016). The animal and vector-borne (primarily ticks and mosquitoes) viral diseases are of major concern due to their easy and accelerated migration across the continents (Hubálek et al. Citation2014). In the past 60 years, several deadly disease outbreaks in man resulted from the emergence of viruses of animal origin such as Henipaviruses (Hendra, Nipah, and Cedar virus) in Australia (Ong & Wong Citation2015), Hantavirus in the United States of America (Knust & Rollin Citation2013), Ebola virus in Africa (Martínez et al. Citation2015; Nunes-Alves Citation2016), several influenza subtypes (bird flu, swine flu, etc.) (Kapoor and Dhama Citation2014), severe acute respiratory syndrome (SARS) and the Middle East respiratory syndrome (MERS). Recently, it was Ebola that threatened the whole world by its outrageous nature (Dhama, Malik, et al. Citation2015; Martínez et al. Citation2015; Nunes-Alves Citation2016) and now it is time for Zika virus which has already set the floor on fire due to its rapid and wider spread (Fauci & Morens Citation2016). The Zika outbreaks are more challenging, as they are unpredictable and rapidly spreading among the affected people (Hayes Citation2009; Gautret & Simon Citation2016; Grard et al. Citation2014; Gatherer & Kohl Citation2016; Gulland Citation2016a). Moreover, least is known about disease ecology and further lack of effective prevention and treatment options worsen the situation (Elachola et al. Citation2016; Petersen, Wilson, et al. Citation2016). Zika virus raised concern in the recent past because of its worldwide spread whereas earlier it was found limited only to two of its dwelling continents, Africa and Asia (Heang et al. Citation2012; Brown Citation2015; Lucey & Gostin Citation2016; WHO Citation2016).
The viruses of the Flaviviridae family found in arthropods (primarily in ticks and mosquitoes) can occasionally infect humans (Chastel Citation2012; Choumet & Desprès Citation2015). Members of this family belong to a single genus, Flavivirus, associated with considerable morbidity and mortality throughout the world. Some of the tick-transmitted viruses are responsible for encephalitis and hemorrhagic diseases viz. tick-borne encephalitis (TBE), Kyasanur Forest Disease (KFD), Alkhurma Disease, and Omsk Hemorrhagic Fever (OHF). Several other flaviviruses such as Japanese encephalitis, Yellow Fever, West Nile, Dengue, Chikungunya, and Zika virus are being transmitted by the mosquitoes (Daep et al. Citation2014; Hubálek et al. Citation2014; Medlock & Leach Citation2015; Parham et al. Citation2015). North America has been victimized by the emergence of several arthropod-borne diseases, including Jamestown Canyon, Powassan, Chikungunya, and Zika diseases recently (Pastula et al. Citation2016). Due to recent outbreaks, Zika virus (Fauci & Morens Citation2016) is being considered as an emerging infectious disease with the potential to spread to new areas where the Aedes mosquito vector is present (Hayes Citation2009; Chen & Wilson Citation2010; Cao-Lormeau et al. Citation2014; Carneiro & Travassos Citation2016; CDC Citation2016; Hennessey et al. Citation2016) and reports also document the involvement of other vectors like Culex spp. (Marcondes et al. Citation2016).
Zika virus possesses an un-segmented, single-stranded, positive-sense RNA genome of approximately 11 kb and its single open reading frame (ORF) (10272 bp) encodes a polyprotein which includes three structural proteins viz., capsid, pre-membrane, and envelope and seven non-structural (NS) proteins (NS1, NS2a, NS2b, NS3, NS4a, NS4b, and NS5). Hitherto studies elucidate phylogenetic relationship among Zika virus strains targeting selected genes (e.g. envelope, NS3, NS5, etc.) from a few strains/isolates. Still, we are falling short of a broad spectrum picture about Zika virus evolution.
The first Zika virus isolation was done in 1947 (Dick et al. Citation1952). Since the Zika disease outbreak in Brazil during early 2015 (Zanluca et al. Citation2015; WHO Citation2016; Rodriguez-Morales Citation2015; Nereida Citation2015), the disease is spreading rapidly across South and Central America, and Mexico (Summers et al. Citation2015). After Brazil, Colombia is the most-affected country, with well over 25,000 suspected human cases reported and 1331 Zika virus cases confirmed in man since October 2015 (Petersen, Wilson, et al. Citation2016; Roa Citation2016). These outbreaks are more challenging as they are unpredictable and rapidly emerging/spreading among the people affected. Considering the above situation, the WHO on 1 February 2016, declared it as a ‘Public Health Emergency of International Concern’ (WHO Citation2016; Gulland Citation2016b; Higgs Citation2016).
As of 30th March 2016, locally transmitted (autochthonous) cases of Zika virus infection have been reported from 61 countries or territories worldwide (Zanluca et al. Citation2015; Calvet, Filippis, et al. Citation2016). In literature, Zika disease is described as a mild, self-limiting febrile illness lasting four to nine days without severe complications, no fatalities, and a low hospitalization rate (CDC Citation2016). There is a link between Zika virus spread and an increase in cases of fetal abnormalities like microcephaly, hydranencephaly, ventriculomegaly, cerebral calcifications, abnormally formed or absence of brain structures, cataracts of both eyes, calcifications of eye, and hydrops fetalis during pregnancy and yet an unproven association with Guillain–Barré syndrome (GBS) in adult people (Oehler et al. Citation2014; de Paula Freitas et al. Citation2016; Karwowski et al. Citation2016). Since October 2015, more than 4000 babies in Brazil had been born with abnormally small heads and brains – a rare condition known as microcephaly (Martines et al. Citation2016; Schuler-Faccini et al. Citation2016; Mlakar et al. Citation2016; Rodriguez-Morales Citation2016; Heymann et al. Citation2016). The Zika virus transmission occurs through the Aedes mosquito, perinatal transmission (Besnard et al. Citation2014), sexual intercourse, and blood transfusion (Musso, Nhan, et al. Citation2014; Franchini & Velati Citation2015). From infected pregnant mothers, the Zika virus can be transmitted via transplacental route and as a consequence might affect the developing brain of the fetus (Roa Citation2016; Rodriguez-Morales Citation2016; Torjesen Citation2016). The methods available for diagnosis of Zika virus include real-time (RT) polymerase chain reaction (PCR) (Faye et al. Citation2008; Gourinat et al. Citation2015) and IgM-based ELISAs, with the latter one suffering from limitation of cross-reaction of antibodies with other Flaviviruses (Buathong et al. Citation2015). Currently, there is no effective treatment for this disease. Though vaccination seems to be a promising option for controlling the disease, no effective vaccine against Zika disease is available yet (Dyer Citation2016a). Prevention and control are the only available weapon in the arsenal to keep this disease under check. Several approaches for controlling mosquito population like mechanical control, use of chemicals, and genetic control methods are available (Araújo et al. Citation2015). The WHO has issued an alert to the public regarding Zika disease on 1 February 2016 (Citrome Citation2016). The guidelines issued recently need to be followed to prevent further spread of disease. Strict biosecurity measures need to be adopted worldwide in order to prevent further spread of the disease to different parts of the world.
2. Etiology
The family Flaviviridae comprises of four genera viz., 1. Flavivirus (53 Species), Hepacivirus (one Species), Pegivirus (two Species), and Pestivirus (four Species) (http://www.ictvonline.org/virusTaxonomy.asp). Based on virus transmission, the genus Flavivirus is further divided into three major groups viz., (1) tick-borne viruses (mammalian tick-borne virus group, seabird tick-borne virus group), (2) mosquito-borne viruses (Aroa virus group, Dengue virus group, Japanese encephalitis virus group, Kokobera virus group, Ntaya virus group, Spondweni virus group, and Yellow Fever virus group), and (3) viruses with no known arthropod vector (Entebbe bat virus group, Modoc virus group, and Rio Bravo virus group). The Zika virus belongs to the genus Flavivirus and is a representative of the Spondweni virus group of mosquito-borne virus group.
The viruses in the genus flavivirus possess a single-stranded, positive-sense RNA genome of approximately 11 kbp with a 5′-type I cap structure that is methylated at the guanine N-7 and the ribose 2′-OH positions of the first transcribed nucleotide adenine (m7GpppAm) (Ray et al. Citation2006). The RNA cap structure is crucial for RNA stability and translation (Picard-Jean et al. Citation2013). The particle size of Zika virus (strain 766) was estimated to be between 30 and 45 nm (Dick Citation1952). The earlier reports speculated that phylogenetically Zika virus relates more closely to Spondweni virus; the two viruses are the only members of their clade within the mosquito-borne cluster of flaviviruses (Kuno et al. Citation1998; Cook & Holmes Citation2006; Lanciotti et al. Citation2008; Hayes Citation2009).
Recently, the Zika virus structure was decipehreed using cryo-electron microscopy with 3.8 Å resolution. Higher similarity exists with other Flavivirus members excepting for nearly 10 amino acids which enfold the Asn154 glycosylation site and constitutes the icosahedral shell. Furthermore, the probable virus attachment site to the host cell was predicted, where any change can alter further virus transmission and disease progression. This provides critical insights into the development of antiviral treatments and vaccines in days to come (Sirohi et al. Citation2016).
3. Zika virus genomic configuration
As on 31st March 2016, 59 complete coding sequences and/or genome information is available on Zika virus in the public database, representing human (n = 39), monkey (n = 7), and Aedes mosquito (n = 13) isolates (). In 2007, for the first time, two full-length sequences of Zika virus strain ‘MR 766’ from monkey (NC_012532 and AY632535) were deposited to the database by Kuno and Chang from the National Center for Zoonotic, Vector-Borne, and Enteric Diseases, USA. The former sequence is being considered as the reference sequence. With the advancement in next generation sequencing platforms, the database was continuously enriched with the addition of new strains/isolates, with the highest number of submission of Zika viral sequences (n = 44) in the year 2016 itself. As per the genomic information available, the ORF within the Zika virus genome ranges between 10,254 and 10,272 nucleotides, encoding a polyprotein of 3417 (e.g. isolates ARB15076 from mosquito and MR 766 from monkey) to 3423 amino acid residues as depicted in . The insertion of few amino acids is seen at position 441--442 in the genomic region endcoding E protein. This observation needs further analysis regarding their role in host–pathogen interaction or other functions. Of the note, all human Zika virus strains/isolates possess a polyprotein of 3423 amino acids, whereas, Zika virus with smaller polyprotein could only be isolated from mosquitos and monkey (). Deletion of certain amino acids in polyprotein may be attributed to the passage history of a particular strain; however, it needs strong experimental support (Haddow et al. Citation2012). The un-translated regions (UTRs) in Flaviviruses contain conserved sequences (designated as CS1, CS2, and CS3) that are believed to play important roles in genome stabilization and genomic cycle such as recognition by cellular or viral factors, translation, RNA packaging, replication, etc. (Lindenbach & Rice Citation2003; Kuno & Chang Citation2007). It is noteworthy that variation in CS1 and CS3 is also reported in Zika virus strain MR 766 (Kuno & Chang Citation2007). Likewise, polymorphism (additions as well as deletions) in length of Zika viral polyproteins is also reported in a few strain/isolates (Haddow et al. Citation2012; Berthet et al. Citation2014). It would be of interest to know what causes the polymorphism in length of Zika viral polyproteins and to explore if this phenomenon has any influence/role on viral replication and pathogenicity. The polyprotein is co- and post-translationally cleaved by host and viral proteases to generate three structural proteins, designated the capsid (C), membrane precursor/membrane (prM/M) and envelope (E) proteins, and seven NS proteins in the gene order: 5'–C–prM(M)–E–NS1–NS2A–NS2B–NS3–NS4A–2K–NS4B–NS5-3' (Rice et al. Citation1985; Blitvich & Firth Citation2015; Baronti et al. Citation2014). These three structural proteins (C, prM, and E) form capsids and seven NS proteins are involved in the assembly and viral replication. The Zika virus envelope plays a role in various aspects of the life cycle including binding and membrane fusion (Lindenbach & Rice Citation2003). The protein C (core protein; 11 kDa) is important for the formation of the nucleocapsid; protein E (50 kDa – the largest structural protein) is an envelope glycoprotein functioning as a fusion protein and it is the primary target for neutralizing antibodies. The protein M (8 kDa) is translated as precursor protein viz; ‘prM’ (26 kDa), which is cleaved by a cellular enzyme (furin) into mature protein M – and a ‘pr’ segment at the time of virion release from the cell. The prM complex protects protein E from degradation during virion assembly. The ‘pr’ and the M proteins can provide host protection from infection. The seven NS proteins play a vital role in replication of the flaviviruses and invoking cell-mediated immune response (Ryan et al. Citation1998; Zhang et al. Citation2003; Bollati et al. Citation2010; Shiryaev & Strongin Citation2010; Smit et al. Citation2011; Blitvich & Firth Citation2015). The structure of Zika virus and its genome is depicted in . The NS5, being the largest, serves as a multi-tasking protein, as its C-terminus performs RNA-dependent RNA polymerase (RdRP) activity while the N-terminus possesses methyltransferase activity and is involved in RNA capping (Lindenbach & Rice Citation2003).
Table 1. Details of Zika virus strains/isolates (full length or near to full length sequences) available in the database.
Figure 1. Structure of Zika virus and its genome. Zika possess ss+ve sense RNA which has seven non-structural genes and three structural genes (C, M, E). M exists as prM (immature form) which gets cleaved to pr and M with the aid of furin enzyme.
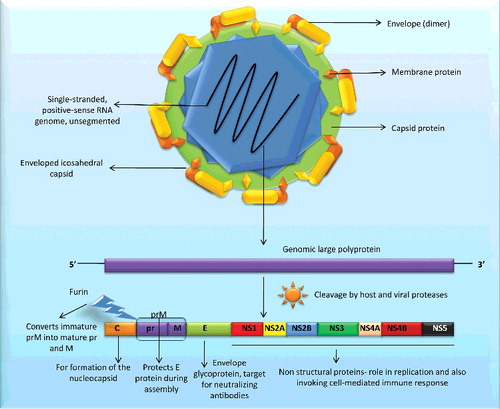
Zika virus genome encodes for only 10 proteins. Hence, it may be assumed that one or more proteins may possess post-translational modifications (PTM) to exert different functions. PTM is a crucial phenomenon that leads to altered protein function in various sub-cellular locations. It becomes further important when genome size is less and only a few proteins are translated as in the case of viruses. A number of putative N and O-linked glycosylation sites have been predicted in a few proteins viz., membrane, envelope, and NS1 of several Zika virus strains (Kuno & Chang Citation2007; Lanciotti et al. Citation2008; Haddow et al. Citation2012; Faye et al. Citation2014). N-linked glycosylation is known to be important in infectivity and assembly of flaviviruses (Mondotte et al. Citation2007; Lee et al. Citation2010). The glycosylation sites in Zika virus genome exhibit polymorphism and may have adaptive values during evolution. It is imperative to remark here that loss of glycosylation sites occurs in Zika viruses (Haddow et al. Citation2012; Hanna et al. Citation2005; Lee et al. Citation2010). Recently, the correlation between glycosylation sites polymorphism and the vector was suggested to cause the evolution of Zika viruses (Faye et al. Citation2014). Though the absence of glycosylation may be important for the sylvatic cycle of the virus (Mondotte et al. Citation2007), the functional importance of glycosylation is still a matter of research.
Moreover, we predicted putative phosphorylation sites in the polyprotein from different Zika virus strains isolated from human (Haiti/1225/2014, n = 156, and ZikaSPH2015, n = 155), monkey (MR 766, n = 148), and mosquito (ARB7701, n = 146) following the method described earlier (Chattopadhyay et al. Citation2010). Likewise, sites for potential SUMOylation could also be predicted with a high threshold in human (Haiti/1225/2014, n = 4, and ZikaSPH2015, n = 4), monkey (MR 766, n = 6), and mosquito (ARB7701, n = 5) Zika virus polyproteins. Till date, only glycosylation sites are reprted in Zika viral proteins, whereas predictions emerged out of the analysis we conducted suggest the presence of other PTM modifications also. Biological functions such as the protein–protein interaction of proteins are known to be influenced by phosphorylation, whereas SUMOylation provides functional flexibility to proteins and is involved in different biological phenomena such as protein localization and functions. Hence, future Zika virus research strategies should also include exploration of PTM in polyproteins and their biological significance.
4. Zika virus evolution
To study the Zika virus evolution, complete genome sequences encoding polyprotein were retrieved from NCBI database (n = 59) and subjected to the phylogenetic analysis with the help of MEGA (Molecular Evolutionary Genetic Analysis version 6.05) tool (Tamura et al. Citation2013). Nucleotide sequences were aligned using the default pairwise and multiple alignment parameters of ClustalW in MEGA and applied for selection of the best substitution model for further analysis using FindModel tool (http://www.hiv.lanl.gov/content/sequence/findmodel/findmodel.html). Gaps were positioned to minimize nucleotide mismatches and treated as missing data in all analyses. The Maximum likelihood statistical method was applied to construct a phylogenetic tree with the GTR + G + I model. The robustness of tree was tested using the bootstrap method based on 1000 replicates. Branch corresponding to partitions reproduced in <50% bootstrap replicates was collapsed. The genealogy of Zika virus was studied to identify the ancestral sequence(s) among the existing viral populations by TCS network (Clement et al. Citation2000) implemented in PopART i.e. Population Analysis with Reticulate Trees software version 1.7 (http://popart.otago.ac.nz).
Hitherto studies report evolutionary analysis of pandemic Zika virus with elucidation of genetic relationship among different Zika virus strains/isolates (Lanciotti et al. Citation2008; Haddow et al. Citation2012; Faye et al. Citation2014; Berthet et al. Citation2014; Grard et al. Citation2014; Buathong et al. Citation2015; Gatherer & Kohl Citation2016) based either on a few genes e.g. envelope, NS3, NS5, etc. (Lanciotti et al. Citation2008; Faye et al. Citation2014; Grard et al. Citation2014) or some strains/isolates (Haddow et al. Citation2012; Gatherer & Kohl Citation2016; Buathong et al. Citation2015). Use of envelope and other non-structural genes (NS5) for phylogenetic analysis in most of the studies could be attributed to the presence of high phylogenetic signal contents in these genes (Faye et al. Citation2014). Broadly, these studies have outlined that all Zika virus strains/isolates cluster into two major clades, one representing the African and the other the Asian lineage. The African lineage is further grouped into two sub-clades, the MR766 prototype clade from East Africa (Ugandan cluster) and another from West Africa (Nigerian cluster) (Haddow et al. Citation2012; Faye et al. Citation2014; Berthet et al. Citation2014; Grard et al. Citation2014; Gatherer & Kohl Citation2016; Buathong et al. Citation2015). The African and American strains share more than 87.5% and 95% homologies with Asian strains/isolates, respectively (data not shown). The phylogenetic analysis carried out herein, targeting ORF of polyprotein gene, was in agreement with previous observations based on selected genes. Moreover, it also supported the notion that American as well as Pacific Island strains/isolates are relatively closer to those from Asian lineage (Zanluca et al. Citation2015; Buathong et al. Citation2015; Alera et al. Citation2015; Enfissi et al. Citation2016). The African strains clustered together forming three sub-clades representing strains from West Africa, Central African Republic (CAR), and East Africa, respectively (). This observation was also evident in TCS network analysis, where Asian and African lineages also exhibit clear distance (). There were few variations observed in Asian and American lineage, as strains/isolates from Americas and Carrebian Islands exhibited diversity and formed four different clusters (). Asian strains formed independent clusters, except those isolated from China, suggesting relatively more diversity than African Zika viral strains. Between the two Zika viral lineages, the Asian lineage is thought to have evolved with higher epidemic potential (Kelser Citation2016) in line with with the diversity seen in Asian lineage strains. Furthermore, strains from Pacific Islands (two representations in our study) clustered distinctly in the phylogram. The strain that caused the outbreak in Micronasia (2007) clustered with those from Phillipines and Malaysia, while that from French Polynesia formed an independent cluster. It was concomitant with previous phylogenetic observations (Enfissi et al. Citation2016). Of the note, two isolates from Italy (Accession no. KU853012-13) were found to have closer similarity with Brazilian isolates. Further examination revealed that these two actually belong to Dominican Republic and were carried to Italy with a traveler (Barzon et al. Citation2016). Strains from Senegal in African lineage were present in two sub-clades from West and East Africa as observed earlier (Faye et al. Citation2014) which hypothesize the presence of more than one distinct lineage in Senegal (Faye et al. Citation2014). Likewise, strains from China as well as Brazil were also part of different sub-clades, suggesting circulation of more than one type of Zika viral population in those regions (). Especially Brazilian isolates exhibit highest diversity among them when compared to strains/isolates from any other country. This diverse Zika viral population may pose serious challenge to Zika viral vaccination research in the continent. Phylogenetic studies by other researchers on Zika virus also emphasized that it emerged in Uganda (most probably the strain ‘MR 766’ accession no. AY632535, as evidenced in our analysis also; ). This emergence is followed by movement of the virus towards East African countries such as Senegal, Nigeria, and CAR. The Asian lineage also emerged from African strain (from Uganda), moved towards Pacific Islands causing epidemic in Micronesia (Faye et al. Citation2014; Gatherer & Kohl Citation2016; Buathong et al. Citation2015; Alera et al. Citation2015) and eventually reached the Americas (Gatherer & Kohl Citation2016; Zanluca et al. Citation2015). Additionally, TCS network suggested two more putative ancestral sequences of human origin circulating in China (GD01; KU740184) and Brazil (BeH819966; KU365779). Our observation advocates the school of thought that the Zika virus was widely distributed throughout the distinctly situated continents such as Africa, Asia, and America since long (Faye et al. Citation2014). Therefore, time and place of isolation might not be linked to their spread.
Figure 2. Phylogenetic analysis of genes encoding polyprotein in Zika virus. Maximum likelihood tree was generated from nucleotide sequences of 59 Zika virus strains (complete open reading frame) retrieved from GenBank utilizing the GTR + G + I model. The tree was rooted with Spondweni virus reference sequence (GenBank accession number NC_029055). Numbers at the nodes represent percentage bootstrap support values based on 1000 replicates. Strains/isolates are represented according to their name, accession number, country of origin, and year of collection/isolation/submission. Strains from Americas (1a–1c) together with those from Asian and Oceania constituted the Asian-American lineage and grouped into 1a, 1b, 1c, and 1d. The African strains/isolates clustered to form the African lineage and grouped into east (2a), central (2b) and west (2c) African sub-clades.
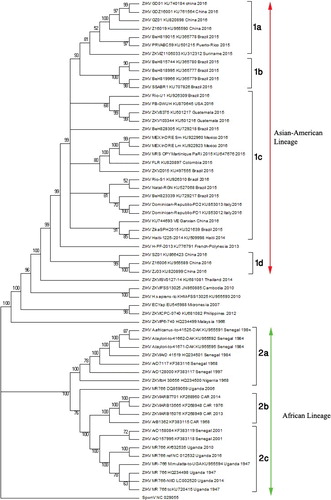
Figure 3. The genealogy of Zika viral strains/isolates. The TCS network showing 59 haplotypes connected parsimoniously. Individual discs indicate haplotypes with the size of the disc proportional to the number of accessions in the haplotype. Strains viz; ‘MR 766’ from monkey (AY632535), BeH819966 (KU365779) and GD01 (KU740184) both of human origin represented in dark eclipses were identified as basal (ancestral) haplotypes in Zika viral population. Hatch marks represent nucleotide changes during evolution.
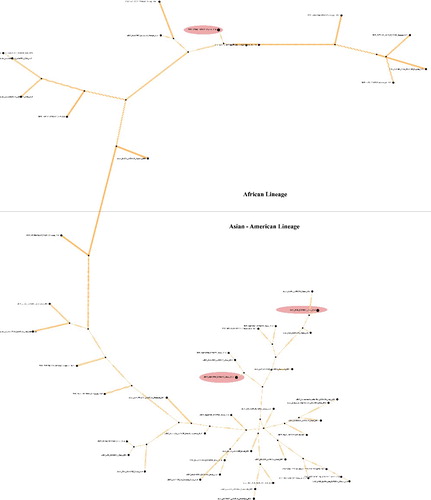
Recently, recombination events – although a rare phenomenon in Flaviviruses (Loriere and Holmes Citation2011) – are also found with significant statistical support in different Zika viral strains (Faye et al. Citation2014). The study advocated that recombinations are also being experienced by Zika viruses in the field. However, the occurrence of this phenomenon in Zika viruses should be addressed with additional strain/isolates available in the database and eventually must be evaluated for their impact on pathogen distribution, epidemiological, and zoonotic potential. Additionally, the study also identified that a few Zika viral genes e.g. envelope and NS5 are under strong negative selection pressure. Infection and transmission mode of RNA viruses such as Zika viruse permit the negatively selected sites and synonymous substitutions (Hanada et al. Citation2004). Hence, episodes of negative selections together with no sign of positive selection are indicative of eradication of unwanted polymorphism in genes with functional significance. One can assume that deletions that remove glycosylation sites in the envelope gene which, in turn, increase the infectivity of Zika virus and the outbreak of Zika virus is due to negative selection. However, all these speculations need experimental validation. One speculation is that Zika virus undergoes recombination and due to loss of its N154 glycosylation site in the envelope protein got adapted to Aedes dalzieli, a mosquito vector in Africa (Faye et al. Citation2014).
5. Epidemiology, transmission, and spread
In recent times, a lesser known mosquito-borne virus, Zika virus, is emerging as a serious public health concern (Chen & Hamer Citation2016; Fauci & Morens Citation2016; Higgs Citation2016; WHO Citation2016; CDC Citation2016). It produces a comparatively mild disease in adult humans. As shown in , Zika virus was first isolated from a rhesus monkey in 1947, at Zika forest in Uganda (Dick et al. Citation1952; Goma Citation1965; Derraik & Slaney Citation2015). Subsequently, the second isolation of Zika virus was made from a batch of wild-caught Aedes africanus in January 1948, in the same forest (Weinbren & Williams Citation1958; Haddow et al. Citation1964). A study conducted in semi-captive orangutans in Malaysia suggested that these animals were exposed to Zika virus at some point in time (Kilbourn et al. Citation2003). In 1954, during the investigation of an outbreak of jaundice suspected of being Yellow Fever in Eastern Nigeria, three cases were confirmed as positive for Zika virus infection; virus could be isolated from a serum sample of a 10-year-old girl with a history of fever and headache only, whereas rise in antibodies was detected against this virus in two other cases (MacNamara Citation1954). During the research on new strains of Zika virus isolated from A. africanus collected in Zika forest, one of the researchers became ill with maculopapular rash on the face, neck, trunk, and upper arms, slight malaise with pain in the back and febrile response and Zika virus could be isolated from a serum sample while he was febrile (Simpson Citation1964). Subsequently, virus isolation has been made from different countries including Nigeria (Moore et al. Citation1975; Fagbami Citation1979), Ivory Coast in 1999 (Akoua-Koffi et al. Citation2001), Cambodia (Heang et al. Citation2012), Thailand (Buathong et al. Citation2015), the Philippines (Alera et al. Citation2015), Indonesia (Perkasa et al. Citation2016), and Brazil (Calvet, Filippis, et al. Citation2016; Calvet, Aguiar, et al. Citation2016).
Figure 4. Landmarks in Zika virus epidemiology. The red area depicts Zika virus presence and/or serological reports (Since 1947–2016) and in blue denoted serological evidences of Zika in Southeast Asia.
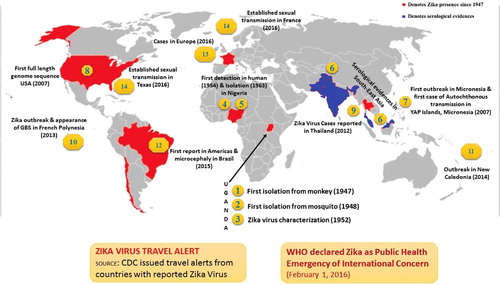
Serological surveys in Africa and Southeast Asia indicated a most likely silent Zika virus circulation with detection of specific antibodies in various animal species (large mammals such as orangutans, zebra, elephants, water buffaloes) and rodents (Geser et al. Citation1970; Jan et al. Citation1978; Lanciotti et al. Citation2008). However, so far the natural reservoir for Zika virus is unknown. Furthermore, serosurveys and viral isolation from mosquitoes and man had confirmed that before 2007, the geographical distribution of Zika virus was restricted to tropical Africa and Southeast Asia (Olson et al. Citation1983; Martinez-Pulgarin et al. Citation2016). Of note, in 2007, the first documented transmission and outbreak of Zika virus in man (with 185 confirmed cases), outside of its traditional endemic areas of Africa and Asia, was reported on Yap Island of the Federated States of Micronesia (Duffy et al. Citation2009).
Subsequently, between 2013 and 2015, several disease outbreaks were notified on islands and archipelagos from the Pacific region including a large scale outbreak in French Polynesia (Hancock et al. Citation2014; Musso, Nilles, et al. Citation2014; European CDC Citation2014; Attar Citation2016) and New Caledonia (Cao-Lormeau et al. Citation2014; Besnard et al. Citation2014; Teurlai et al. Citation2015; Tognarelli et al. Citation2015). In May 2015, the Pan American Health Organization (PAHO) issued an alert after the first confirmed Zika virus case in Brazil. The emergence of Zika virus in South America led to a rapid spread throughout South and Central America, reaching Mexico in November 2015 (Zanluca et al. Citation2015; WHO Citation2016). The first case of Zika virus infection in the USA was recorded recently in a traveler returning from Latin America to Texas (McCarthy Citation2016a). It has appeared sporadically in travelers from the United States of America and Europe but has not established person-to-person spread in those areas. As on 30 March 2016, 61 countries and territories were reporting local transmission of Zika virus (Zanluca 2015; Sabogal-Roman et al. Citation2015; Calvet, Filippis, et al. Citation2016; Petersen, , Wilson, et al. Citation2016; Roa Citation2016; Fauci & Morens Citation2016; Gulland Citation2016a).
In October 2015, Brazil public health authorities detected an unusual increase in human microcephaly cases in public and private healthcare facilities in Pernambuco state, Northeast Brazil. By 30th March 2016, 6776 suspected cases of microcephaly and/or central nervous system (CNS) deformations potentially linked to Zika virus have been reported in Brazil which are mainly concentrating in Northeastern parts. Furthermore, an increase in GBS has also been observed in Brazil, Colombia, El Salvador, Martinique, and Suriname (Campos et al. Citation2015). Lately, from the United States of America, locally acquired cases, around 14 in number without vector-borne transmission, likely to have been contracted by sexual contact, were reported (Musso, Roche, Robin, et al. Citation2015; McCarthy Citation2016b; McCarthy Citation2016c), and indirect evidence of local transmission were further documented in six additional countries (Lucey & Gostin Citation2016). There is not a single known case of someone contracting Zika virus from mosquitoes in the United States. Very recently in Thailand, a study showed that seven samples out of 21 tested showed immunoreactivity to Dengue, Chikungunya, Zika, and Japanese encephalitis by Western blot. Of note, two samples reacted only to Zika virus antigen confirming its presence in Thailand, which marked the first report of this virus in this part of the world (Wikan et al. Citation2016). Japan has also reported Zika virus in urine from a traveler visiting Thailand (Shinohara et al. Citation2016) and two earlier cases have been reported in traveler's returning from French Polynesia and Japan (Kutsuna et al. Citation2014). It was reported in an Australian returning from Indonesia. Hence, tourism and travel have also resulted in transmission of the disease (Kwong et al. Citation2013).
The geographical distribution of Zika virus has been steadily increasing since it was first detected in the Americas in 2015 (Gatherer & Kohl Citation2016; Hennessey et al. Citation2016). It is likely that Zika virus will continue to spread and will likely reach all countries and territories of the Americas where Aedes mosquitoes are found. In less than a year, Zika virus epidemic has evolved in a WHO-declared global health emergency (Jacob Citation2016; Kindhauser Citation2016). The research community urgently needs to understand why Zika virus has shifted from being a virus that caused mild illness to one associated with more than 4000 suspected microcephaly cases in Brazil (Petersen, , Wilson, et al. Citation2016), as well as possibly GBS (Lucey and Gostin Citation2016). The WHO has outlined its $56 million strategic plan to respond to the ongoing outbreaks of Zika virus disease in the Americas (McCarthy Citation2016d). The plan provides a framework for WHO and its partner organizations’ Zika response through to June 2016. Recently, there are four cases of Zika virus reported from the United Kingdom which may be due to travel to affected countries (O'Dowd Citation2016).
The Zika virus-specific antibodies could be detected from serum samples of six out of 99 residents of Uganda and one out of 15 wild monkeys tested (Dick Citation1952). Subsequently, the antibodies against Zika virus could be detected in man in Nigeria (MacNamara Citation1952), Uganda, and Tanganyika (United Republic of Tanzania) (Smithburn Citation1952). During 1952–1953, in India, serum samples have been collected from residents of 38 localities in 6 states and tested for neutralizing antibodies against 15 different arthropod-borne viruses and a significant number of samples showed neutralizing activity against Zika virus (Smithburn, Kerr, Gatne Citation1954). Subsequently, the presence of antibodies against Zika virus was reported in several countries – Malaysia (Smithburn Citation1954), Borneo (Smithburn Citation1954), Vietnam (Pond Citation1963), Thailand (Smithburn Citation1954), Egypt (Smithburn et al. Citation1954), Philippines (Hammon et al. Citation1958), Somalia (Henderson et al. Citation1968), Kenya (Geser et al. Citation1970), Gabon (Jan et al. Citation1978; Saluzzo et al. Citation1982), Nigeria (Monath et al. Citation1973; Fagbami Citation1977; Fagbami Citation1979; Adekolu-John & Fagbami Citation1983), Sierra-Leone (Robin & Mouchet Citation1975), Indonesia (Olson et al. Citation1981), Central African Republic (Saluzzo et al. Citation1981), Pakistan (Darwish et al. Citation1983), Senegal (Renaudet et al. Citation1978; Monlun et al. Citation1993), Nigeria (Baba et al. Citation1999), Côte d'Ivoire (Akoua-Koffi et al. Citation2001),Yap Island, Federated States of Micronesia (Lanciotti et al. Citation2008; Duffy et al. Citation2009), Cameroon (Fokam et al. Citation2010), USA (Foy et al. Citation2011), Cambodia (Heang et al. Citation2012), Zambia (Babaniyi et al. Citation2015), and French Polynesia (Aubry et al. Citation2015).
In non-human primates, antibodies against Zika virus have been reported both in wild and experimental animals in Borneo (Smithburn Citation1954; Wolfe et al. Citation2001) and Uganda (Smithburn Citation1952; Dick Citation1952; Dick et al. Citation1952; Weinbren & Williams Citation1958; Haddow et al. Citation1964; Henderson et al. Citation1968; Henderson et al. Citation1970; Kirya & Okia Citation1977; McCrae & Kirya Citation1982; Rodhain et al. Citation1989; Duffy et al. Citation2009). Since serological cross-reactivity may occur between Zika virus and other Flaviviruses (e.g. Dengue, Yellow Fever, St. Louis encephalitis, Japanese encephalitis, West Nile, etc.), molecular assay in addition to serology such as RT-PCR should be used for distinguishing these viruses.
Until the large-scale outbreak of Zika virus in 2007 in the Yap Island (comprises four closely grouped islands and several outer islands) of the Federated States of Micronesia in the North Pacific, 14 cases of human Zika virus were previously documented (Duffy et al. Citation2009) within Africa and Asia. The physicians on Yap Island noted an outbreak of illness characterized by rash, conjunctivitis, subjective fever, arthralgia, and arthritis. Ten out of 71 samples (14%) were confirmed as Zika virus positive by RT-PCR by CDC, Arbovirus Diagnostic, and Reference Laboratory in Fort Collins, Colorado, USA. Further investigation was carried out in the group of four main islands (total population of 7391 persons). From a total of 185 suspected cases, 49 (26%) were confirmed as positive and 59 (32%) classified as probable (based on a set of criteria mainly by detection of Zika virus RNA and serology) with an overall morbidity rate of 14.6 per 1000 Yap Island residents. No deaths or neurological complications were reported in this outbreak (Lanciotti et al. Citation2008; Duffy et al. Citation2009). Analysis of Zika virus RNA revealed that the outbreak on Yap Island originated in Southeast Asia (Lanciotti et al. Citation2008; Duffy et al. Citation2009; Haddow et al. Citation2012; Buathong et al. Citation2015).
In October 2013, Zika virus outbreak started in French Polynesia – a French overseas territory located in the South Pacific. A total of 8510 suspected cases were reported by the sentinel network from the beginning of the epidemic to 14 February 2014, leading the estimation at 29,000 (10% of the population), the number of patients having consulted for a Zika virus infection. The clinical symptoms observed in this outbreak were mild Dengue-like illness consisting of low fever (<38 °C), asthenia, wrist and fingers arthralgia, headache, rash, conjunctivitis, swollen ankles, and aphthous ulcers (Cao-Lormeau et al. Citation2014). Out of 746 patient samples, Zika virus RNA was confirmed in 396 (53%) by RT-PCR, in which 72 cases of severe presentations with acute neurological symptoms were notified. Among these, 40 GBS were also diagnosed within three months (Besnard et al. Citation2014; European CDC Citation2014; Cao-Lormeau et al. Citation2014; Ioos et al. Citation2014). The phylogenetic tree shows that the Zika virus that recently emerged in French Polynesia is similar to Cambodia 2010 and Yap State 2007 strains, which suggests an expansion of Zika virus of Asian lineage (Cao-Lormeau et al. Citation2014). The results of retrospective investigations were submitted to WHO. These reports indicate a possible association between Zika virus infection and congenital defects, severe neurological and autoimmune complications, in particular, an increase in the incidence of GBS. However, the Island was also experiencing an outbreak of Dengue in association with Zika virus infection and GBS (Ioos et al. Citation2014; Kindhauser et al. Citation2016). Further, in 2013–2014, the outbreak spread to other Pacific Islands: New Caledonia, Cook Islands, Easter Island, Vanuatu, and Solomon Islands (Roth et al. Citation2014; Cao-Lormeau et al. Citation2014; Musso, Nhan, et al. Citation2014).
The first autochthonous cases of Zika virus in Brazil (in the city of Natal, state of Rio Grande do Norte) was confirmed in May 2015. In the year 2015, a total of Zika virus cases in Brazil amounted to the tune of 440,000–1,300,000, along with 4783 cases of microencephaly, where 76 were associated with mortality in the northeast province of Brazil (Heukelbach et al. Citation2016). Symptoms observed in these cases were arthralgia, oedema of extremities, mild fever, maculopapular rashes frequently pruritic, headaches, retro-orbital pain, no purulent conjunctivitis, vertigo, myalgia, and digestive disorders. Eight out of 21 samples were positive for Zika virus RNA and shared a high nucleic acid identity with sequences from the Zika virus of Asian lineage (Zanluca et al. Citation2015). Since then, cases of Zika virus are being continuously reported from several states. The major concern is a several fold increase in infants with microcephaly after the introduction of the Zika virus into Brazil. Before 2015, the annual reported cases of microcephaly were consistently below 200 whereas the number of suspected cases reported between November 2015 and 13 February 2016 was 5280. In the initial screening of 1345 samples, Zika virus was detected in 41 samples (Victora et al. Citation2016) (http://apps.who.int/iris/bitstream/10665/204454/1/zikasitrep_19Feb2016_eng.pdf?ua = 1). Between 1 January 2007 and 17 February 2016, a total of 48 countries and territories reported local (autochthonous) transmission of Zika virus (http://apps.who.int/iris/bitstream/10665/204454/1/ zikasitrep_19Feb 2016_en g.pdf?ua = 1).
The Zika virus transmission can be classified into vector-borne and non-vector-borne. In the vector-borne mode, several species of the genus Aedes are involved. The non-vector-borne mode includes sexual transmission, blood transfusion, perinatal or intra-uterine transmission, postnatal transmission, and animal bites (Patiño-Barbosa et al. Citation2015).
Zika virus is maintained in the community through its – (1) sylvatic cycle, where the virus circulates between non-human primates and Aedes mosquitoes, (2) urban cycle, where the virus circulates between man and domestic mosquitoes. Till the year 2007, the most known facts about zika outbreaks demonstrate sylvatic origin/cycle (Weaver et al. Citation2016). The primary mode of transmission of vector-borne Zika virus is through the bites of female mosquitoes in the Aedes genus. The main species in the Zika virus mosquito transmission is Aedes aegypti and there are at least 20 other species that have been tested positive to Zika virus including Aedes albopictus, A. africanus, Aedes luteocephalus, Aedes vitattus, Aedes furcifer, Aedes hensilii, Aedes apicoargenteus, Aedes. polynesiensis, A. dalzieli, Aedes hirsutus, Aedes metalicus, Aedes taylori, Aedes unilineatus, A. apicoargenteus, Anopheles coustani, Culex perfuscus, and Mansonia uniformis (MacNamara Citation1952; Dick et al. Citation1952; Dick Citation1953; MacNamara Citation1954; Weinbren & Williams Citation1958; Haddow et al. Citation1964; Simpson Citation1964; Fagbami Citation1979; Haddow et al. Citation2012; Petersen, Wilson, et al. Citation2016; Zanluca & dos Santos Citation2016; Li et al. Citation2016). Male mosquitoes live only for 10 days while female mosquitoes live for one to two months suggesting that female mosquitoes have a role in transmission and harbouring of the virus (Chang et al. Citation2016). Since the Aedes mosquitoes are found in many parts of the world, it is likely that outbreaks of Zika virus might spread to new naïve areas (Petersen, Wilson, et al. Citation2016). Transmission potential of Zika virus was found to be similar to Dengue and Chikungunya since mosquito is mainly involved in all three diseases (Nishiura et al. Citation2016). Recently, in Senegal mosquito species like A. unilineatus, A. vittatus, and A. luteocephalus were studied for their vector competence and it was found that all species were susceptible to infection by oral route (Diagne et al. Citation2015). A study in Yap Island revealed that A. hensilli was the most important vector for transmission of Zika virus and Chikungunya (Ledermann et al. Citation2014). Similarly, a study from Singapore reports that A. Stegomyia is the potential vector for Zika virus transmission (Li et al. Citation2012).
In August 2008, two American scientists who worked in the village of Bandafassi in southeastern Senegal while performing a mosquito-sampling project in surrounding villages, after returning to the USA, showed Zika-like clinical illness within a week. Furthermore, the wife of one of the above researchers also showed clinical signs but she never traveled to Africa or Asia and had not left the United States of America since 2007. The Zika virus infection was confirmed by a serological assay. Circumstantial evidence suggests direct person-to-person, possibly sexual, transmission of the virus (Foy et al. Citation2011). In December 2013, during a Zika virus outbreak in French Polynesia, a patient in Tahiti sought treatment for hematospermia, and replicative Zika virus could be detected from semen samples (Musso, Roche, Robin, et al. Citation2015). Sexual transmission of Zika virus is not assumed to be a major factor in its spread. Over 80% of Zika virus infection is silent in persons without overt clinical signs including blood donors. Blood-borne transmission of Zika virus via blood transfusion is a major concern (Marano et al. Citation2015; Petersen, Wilson, et al. Citation2016). From November 2013 to February 2014, 42 (3%) of 1505 blood donors, although asymptomatic at the time of blood donation, were found positive for Zika virus by PCR (Musso, Nhan, et al. Citation2014).
During Zika virus outbreak in French Polynesia, two mothers and their newborns had detectable Zika virus RNA in serum collected within four days post-delivery by RT-PCR. The infants’ infection most probably occurred by transplacental transmission or during delivery (Besnard et al. Citation2014). Zika virus RNA could be detected from some of the microcephaly samples (Mlakar et al. Citation2016; Rubin et al. Citation2016) and from amniotic fluid of two pregnant women with microcephaly fetuses in Brazil (Calvet, Aguiar, et al. Citation2016). In the latter study, the virus could be isolated. The other plausible mode of transmission is monkey bite (Leung et al. Citation2015). Serological studies reveal that Zika antibodies can be detected in goats, sheep, bats, and rodents. Serosurvey studies have detected antibodies to Zika virus in bats, goats, and rodents of the species Bandicota bengalensis, Tatera indica, and Meriones hurrianae, but such serological data may be misleading since there is cross reaction between flaviviruses, however it cannot be excluded (Musso & Gubler, Citation2016). Hence, there is no well-documented reservoir animal for Zika and further studies will reveal such animals if found so.
6. The disease
6.1. Clinical symptoms
Zika virus has an incubation period of 3–12 days (Burke et al. Citation2016; Goeijenbier et al. Citation2016). Clinical signs include acute fever, maculopapular skin rashes, non-purulent conjunctivitis, arthralgia, headache, myalgia, asthenia, edema of hand and feet and less evident signs like anorexia, abdominal pain, vomiting, diarrrhoea, vertigo, burning sensation of sole and palm and at times there may be pain in the retro orbital region, and pruritits (Korhonen et al. Citation2016; Shinohara et al. Citation2016; Zammarchi, Stella, et al. Citation2015; Zammarchi, Tappe, et al. Citation2015; Basarab et al. Citation2016; Goeijenbier et al. Citation2016). Symptomps are usually self-limiting and may last for four to seven days (Barrera-Cruz et al. Citation2016). Haematuria and haematospermia (there is no report of haematospermia caused by other arboviruses) have been documented in some cases (Foy et al. Citation2011; Musso, Roche, Robin, et al. Citation2015). These clinical signs are overlapping with the clinical findings of Dengue and Chikungunya, but Zika virus-induced symptoms are milder than those of others (Carneiro & Travassos Citation2016; Tappe et al. Citation2016). Abrupt onset of fever and appearance of rashes, often pruritic which disappear quickly with time, are characteristic features. Occasionally, Zika virus may cause nerve-associated abnormalities tentatively referred to as GBS (Oehler et al. Citation2014; Gatherer & Kohl Citation2016; Pinto et al. Citation2015). Infected pregnant woman traveling to an area where Zika virus outbreak has recently occurred should be routinely subjected to ultrasound to assess the abnormal cranial development of the fetus (Schuler-Faccini et al. Citation2016; Dyer Citation2015; Dyer, Citation2016b; McCarthy Citation2016e). If the infants are getting an infection from their mother in utero, the virus has been reported to be a reason for the foetal** abnormalities like microcephaly, intracranial calcification, hydrops fetalis, hydranencephaly, and eye abnormalities mainly affecting macular and optic nerve development (McCarthy Citation2016f; Sarno et al., Citation2016; Vest Citation2016). A study reported 29% of the 42 pregnant women infected with Zika virus had fetal abnormalities revealed ultrasonically and 12% of the examined womens foetus had microcephaly, cerebral atrophy, and brain calcifications (Driggers et al. Citation2016). There was no adverse effect seen on retinal development in such infants (Ventura, Maia, Bravo-Filho, et al. Citation2016; Schuler-Faccini et al. Citation2016; Ventura, Maia, Ventura, et al. Citation2016). In the case of no cranial abnormalities in infants born from infected mothers, they need to be checked during the first year of life routinely by periodic cranial ultrasound, routine eye checkup, and hearing tests to diagnose subclinical cases (Staples et al. Citation2016). Microcephaly has been reported mainly from Latin American countries though Africa and Asia are endemic and the hypothesis suggested was that women exposed to zika virus at early age gain immunity against zika hence may resist fetal anomalies (Frank et al. Citation2016). Recently, hypertensive iridocyclitis in Zika virus infection has been reported (Fontes Citation2016). An ocular abnormality has been noticed which include chorioretinal atrophy and also gross pigment mottling as well as hypoplasia of the optic nerve (Ventura, Maia, Ventura, et al. Citation2016). Co-infection with Zika virus, Chikungunya, and Dengue virus has been reported in Colombia (Faccini-Martínez et al. Citation2016; Villamil-Gómez et al. Citation2016). An overview of Zika viral disease is presented in
6.2. Pathology and pathogenesis
Zika virus isolates from French Polynesia exhibited their affinity towards human immature dendritic cells, dermal fibroblasts, and epidermal keratinocytes. Zika virus may replicate in the midgut when Aedes mosquitoes feed blood meal as like other flaviviruses and later in the salivary glands. Vertical transmission of Zika virus by transovarian route also cannot be excluded (Chan et al. Citation2016). Aedes mosquito injects Zika virus while feeding on man and the virus enters the cells through receptors like AXL, DC-SIGN, Tyro3 and Tim-1 (lesser extent) that are found on the surface of both skin and nerve cells (Hamel et al. Citation2015). Inside the cells, they use host machinery and finally cause apoptosis and autophagy of the cells thereby entering other cells. Zika virus stimulates transcription of TLR-3, MDA5, RIG-I, and also interferon genes like OAS2, ISG15, and MX1. Viral replication in the cells causes the release of type I interferon (Hamel et al. Citation2015). Replication of Zika virus is increased by inducing autophagy in the host. Thus, agents inhibiting autophagy can decrease virus load in the cells (Carneiro & Travassos Citation2016). After replication locally, virus gets distributed to muscles, heart, CNS, and also to foetus by crossing the placental barrier through blood vessels and lymphatics (Chan et al. Citation2016). The damage in the eye of infants is presumed to be due to Zika virus infection and needs further detailed pathophysiological investigations (McCarthy Citation2016f). Zika virus might have evolved certain means to defeat the defence of the trophoblast thereby attacking neuronal tissues causing neuronal abnormalities in the fetus (Sadovsky et al. Citation2016).
In earlier reports, Zika virus had been shown to have affinity towards brain cells which was demonstrated with the aid of intraperitoneal injection of mice that showed that Zika virus can cross the blood–brain barrier (Dick Citation1952). Neurons and glial cells were infected by virus producing intracytoplasmic inclusions called viral factories having their origin from the endoplasmic reticulum and also from mitochondria and nucleus (Bell et al. Citation1971). Recent studies indicate that Zika virus can infect neuroblast cells in-vivo (Tang et al. Citation2016). Autophagy, as noticed in the dermal fibroblast cells is not noticed in the neurons. Microcephaly has been proposed to be due to abnormalities in the centrosomes yet several studies need to be conducted before confirmation (Tetro Citation2016). There was enlargement of astrocytes and there was also the destruction of hippocampus especially regarding pyriform cells (Bell et al. Citation1971). Inflammatory responses of the placenta due to Zika virus can also cause damage to the fetus (Mor Citation2016). Transient leukopenia and thrombocytopenia have been reported in few cases. Serum biochemistry shows that there may or may not be an increase in aspartate aminotransferase (AST) and alanine aminotransferase (ALT) activities (Tappe et al. Citation2014).
6.3. Immunobiology and immunity
Antibody-dependent enhancement (ADE) of infection is common among Dengue virus serotypes. The recent outbreak of Zika virus has caused more panic than earlier outbreaks and one reason that can be related is that most of the recent outbreaks occurred in hyper-endemic regions of Dengue virus. ADE may be a reason where antibody raised against Dengue virus might have caused enhancement of Zika infection (Lazear & Diamond Citation2016). Immunity against re-infection develops for an unidentified time period (Tappe et al. Citation2015). The increase in chemokines is shown at a higher level than cytokines in Zika viral disease and cytokines level declined during the recovery phase. During acute disease, there is a rise in IL-2, IFN-γ, IL- 4, IL-13, IL-17, and IL-9 (Tappe et al. Citation2015). Regulated on Activation, Normal T Cell Expressed and Secreted (RANTES) or CCL5 was elevated in the acute phase of disease while IP-10 was elevated in the later recovery phase (Tappe et al. Citation2015). Further studies are needed to elucidate the immune response of host against Zika virus disease. Recently, a study revealed that Zika virus and man have a peptide in common, hence this may be the reason for microcephaly and GBS (Lucchese & Kanduc, Citation2016).
7. Public health importance
Zika virus disease has been a major threat throughout the world, but it has shown its hideous face in Brazil. It is not only Zika virus but also Dengue and Chikungunya that have caused damage in Brazil. A recent report shows that there is co-infection of Zika, Dengue, and Chickungunya in Pernambuco, Brazil (Pessôa et al. Citation2016). This has kindled interest among researchers why these diseases affect mainly this part of the world. The study shows that it was mainly due to climate change causing an alteration in the vector population (Gautret & Simon Citation2016). Crowding at a particular place due to meetings like World cup, Olympics, etc. could also aid in transmission of diseases. Good knowledge about the diseases prevailing in the native population should be identified and they should be segregated so as to prevent transmission to the tourists. Arboviral diseases like Dengue, Chikungunya, and recently, Zika have created panic amongst tourists who planned to visit countries like Brazil where disease threat is more predominant (Gautret & Simon Citation2016). FIFA world cup was held in Brazil in the year 2014 and several studies were conducted to assess the arboviral diseases influenced by it and it was found that four to nine people out of 100,000 tourists who visited Brazil succumbed to Dengue (van Panhuis et al. Citation2014). Some researchers are also of the opinion that Zika virus has also entered during FIFA world cup 2014 into Brazil, but there is no evidence to support this speculation (Zanluca et al. Citation2015). Another major sports event took place later in August 2014 named as ‘Va'a World Sprint Championship canoe race’ where there were participants from French Polynesia, New Caledonia, Cook Islands, and Easter Islands, hence this was also speculated for the reason of disease introduction into Brazil. Hypothesis and possibilities were made that people from Chile has transmitted this disease to Brazil during this event (Salvador & Fujita Citation2015). It was speculated that Zika virus might have entered northeast of Brazil through Natal, Recife, Salvador, and Fortaleza where the 2014 World cup football took place. Phylogenetic analysis by researchers revealed that Zika virus might have been introduced in the year 2013 around May to December. By the end of 2015, Zika virus has spread the entire length of Brazil (Weaver et al. Citation2016). Summer Olympics 2016 is around the corner and Zika virus has pressed the alarm button on all health and sports officials in Brazil to look after the health issues of athletes traveling from different parts of the world (Citrome Citation2016).
8. Diagnosis, surveillance and monitoring
Zika virus infection can be diagnosed based upon clinical symptoms, the prevalence of vector in the region, and by serological and molecular detection assays. The isolation of Zika virus from mosquito samples was carried out using newborn Swiss albino mice through different routes like intraperitoneal, intracerebral, and subcutaneous injections (Marchette et al. Citation1969; Way et al. Citation1976). Passages from brain material revealed Zika virus which was further confirmed using hemagglutination inhibition test (Haddow et al. Citation1964). Zika virus can be cultivated in cell lines like Vero cells, rhesus monkey kidney cells like LLC- 192 MK2, and cell lines of mosquito origin like A. albopictus (C6/36) and A. psuedoscutellaris (MOS61 or AP-61) (Waggoner & Pinsky Citation2016). Vero and Vero E6 cells were used for isolation of Zika from saliva (Barzon et al. Citation2016). Recently, a murine model for studying Zika virus has also been reported which could aid in understanding the disease pattern (Rossi et al. Citation2016). Haemagglutination inhibition test, serum neutralization test, and complement fixation tests have been employed for the diagnosis of Zika virus disease (Fagbami Citation1979; Monath et al. Citation1980). The serological diagnosis involves detection of IgG and IgM antibodies. For detecting antibodies against Zika virus in serum of patients, IgM antibodies can be monitored by ELISA. It is positive if Zika virus neutralizing antibody titre is higher than fourfold from Dengue neutralizing antibodies, else the test is considered to be inconclusive (Staples et al. Citation2016). Cross reaction between other members of the flavirus hinders the use of ELISA techniques and hence plaque reduction neutralization test (PRNT) can be done to identify Zika virus induced antibodies (Petersen, Jamieson, et al. Citation2016). PRNT helps in distinguishing cross-neutralizing antibodies generated by various Flaviviruses (Staples et al. Citation2016). Seroconversion is confirmed by analyzing IgM and IgG titres in paired serum samples from the acute and convalescent phase of infection (Pyke et al. Citation2014). Earlier haemagglutination inhibition and mouse protection tests were also used to detect Zika virus antibodies in serum (Fagbami Citation1979; Kirya & Okia Citation1977).
Microcephaly is detected by measuring occipitofrontal circumference as suggested by standard charts (WHO 2006). Ultrasound scanning can be employed for detection of microcephaly in pregnant women (SMFM Publications Committee Citation2016; Oliveira et al. Citation2016). Several foetal abnormalities can be identified around five months of gestation, but microcephaly can only be identified around fix toseven months of gestation or after birth (Lazear et al. Citation2016). Further confirmation can be done using molecular and serological tests (Staples et al. Citation2016).
Blood picture reveals neutropenia and thrombocytopenia (Rafiei et al. Citation2016). Molecular diagnosis involves detecting RNA of Zika virus by RT-PCR. In the initial phase of the disease (first seven days) viral nucleic acids can be detected in the serum (Haug et al. Citation2016). Sample involves serum of patients or umbilical cord sample of infants, urine, nasopharyngeal swab, saliva, amniotic fluid, CSF, and frozen and fixed placenta (Staples et al. Citation2016; Shinohara et al. Citation2016; Tognarelli et al. Citation2015; Leung et al. Citation2015; Musso, Roche, Nhan, et al. Citation2015; Gourinat et al. Citation2015; Balm et al. Citation2012; de M Campos et al. Citation2016). Shedding of virus in different body fluids like urine (persists for 15–20 days) has to be elucidated to establish a better diagnostic assay combined with exact sample of choice (Moulin et al. Citation2016). A report suggests that viruria can be seen more than 15 days after the onset of symptomps (Rozé et al. Citation2016). Recently, in order to establish the relationship between Zika virus and microcephaly, scientists started to investigate antibodies against Zika virus in amniotic fluid and also in the blood of neonates (Jacob Citation2016). RT-PCR followed by DNA sequencing is considered confirmatory (Zanluca et al. Citation2015). Further sequencing of NS5, NS3, and envelope gene confirms the relation of various strains of Zika virus (Tognarelli et al. Citation2015; Fonseca et al. Citation2014; Grard et al. Citation2014). Real-time PCR (rRT-PCR) can be employed to detect the virus in the early stage of infection (Faye et al. Citation2013; Faye et al. Citation2008). Furthermore, it can be confirmed by employing immunohistochemical staining of antigen fixed on the umbilical cord and placenta of infected infants (Staples et al. Citation2016).
The rapid spread of the Zika virus and its epidemic potential along with the presence of other arboviruses create difficulties in the differential diagnosis (Pinto et al. Citation2015). Till now, no commercial diagnostic tests exist for diagnosing this viral infection. Advances in diagnostic techniques need to be applied for Zika virus detection viz., multiplex PCR, LAMP, recombinant diagnostics, gene sequencing and phylogenetic analysis, biosensors, biochips, microarrays, and nanotechnology-based diagnostics (Jain Citation2007; Kawadkar et al. Citation2011; Dhama, Karthik, et al. Citation2014; Ratcliff et al. Citation2007; Bergquist Citation2011; Van den Hurk and Evoy Citation2015). Apart from these newer tools for extensive epidemiological and surveillance studies, alternatives need to be explored for tracking and monitoring Zika virus infections and spread, such as geographical information system and establishing networking programs to counter this important pathogen. More recently, a mobile App named as ZIKATracker (zikatracker.net) has been developed in four languages to report the cases as they occur so that they can be effectively controlled and provided on time (Kelvin et al., Citation2016). Such early reporting helps in understanding the pattern of spread of the disease from one area to another. It is essential to use such techniques to report Zika virus cases at earliest possibility keeping in mind the rapid spread of the virus (Nishiura et al. Citation2016). All developments and timely progress would help in rapid detection of Zika virus and design effective disease prevention and control programmes.
9. Prevention and control strategies
Prevention and control of Zika virus are mainly aimed at prevention of vector population (mosquitoes) as they play an important role in the transmission of this virus (Ahmad et al. Citation2016). Control measures are similar to the measures adopted for Dengue and Chikungunya viruses that are also transmitted by vectors (Pinto et al. Citation2015). Mosquito of the genus Aedes is the vector transmitting Zika virus and also another important vector-borne disease namely Dengue (Araújo et al. Citation2015). Hence, control of vector population is of prime importance to control the transmission of these important dreadful diseases among humans. Day-time avoidance of the mosquito bites from infected patients can reduce a human-to-mosquito transmission thereby preventing transmission to other humans. These vectors can be controlled either by mechanical, chemical, and biological measures (Araújo et al. Citation2015).
Mechanical control methods involve removal of any objects that can aid in unwanted storage of water in the premises that serve as a breeding point for female mosquitoes. Removal of those objects like plastic bags, unused tyres, unused containers, bottles, and also closing water tanks with lids can prevent breeding areas of the mosquitoes (Araújo et al. Citation2015). Chemical control of insects and vectors is an age-old technique and can be used with caution as it can cause toxicity to animals (Dick et al. Citation2012). Recently, chemicals like pyrethroids, organochloride, and organophosphorus which acts on the nervous system of the vectors are being used to prevent mosquitoes. The major disadvantage in the use of these chemicals is the problem of resistance development and also these compounds are toxic to higher mammals thereby questioning its use for the control of vectors (CDC 2012). Preparations like N,N-diethyl-meta-toluamide (DEET) and picaridin insect repellent can be used as they are safe for pregnant women (Kline & Schutze Citation2016). Herbal therapy has not only been revolutionizing as an antimicrobial regimen but also has been implemented recently in the control of vectors. These botanical insecticides can be produced from leaves, seeds, and even fruits of different plant varieties (Ghosh et al. Citation2012). Some of the plants that can be used for extraction of botanical insecticides include Apium graveolens, Callistemon rigidus, Cipadessa baccifera, and Persea Americana (Kumar et al. Citation2014; Pierre et al. Citation2014). Extracts can be isolated from these plants and can be employed to find the cidal action against larvae and other stages of the mosquitoes so that it can be employed as a safe insecticide (Dias et al. Citation2014). These extracts can also be used as a repellent in the household day-to-day to effectively control mosquito bites so that prevention of deadly diseases like Zika virus becomes easy (Ramkumar & Karthi Citation2015). Cymbopogon citratus and Eucalyptus citriodora possess a principle named Citronella which has been used in mosquito repellents these days. Another study also reported Apium graveolens seed oil has a good repelling power against Aedes mosquito (Kumar et al. Citation2014). Biological control of vectors might also involve the use of bacteria namely Bacillus thuringiensis israelensis producing deadly endotoxins when mosquitoes ingest those bacteria (Boyce et al. Citation2013). Fungi like Beauveria bassiana has also been used to control Aedes mosquitoes (Darbro et al. Citation2012). Another approach is the use of larvivorous fishes (e.g. Gambusia affinis) in the water-logging areas and flower pots which can eliminate larvae of mosquitoes (Kant et al. Citation2013).
Lately, the use of microbes to control vector population gained interest and certain genus of bacteria named Asaia has been explored for its ability to colonize the gut and reproductive tract of mosquitoes and also to get transmitted vertically to its progeny (Favia et al. Citation2008). Another bacterium named Wolbachia has the potential to feminize male vectors thereby preventing the reproduction of vectors hence controlling their population (Brelsfoard & Dobson Citation2009). Trails are on the way using this bacterium and sterile males have been released into the environment to monitor the effect on reproduction (Frentiu et al. Citation2014). Trails are conducted in Australia, Vietnam, Indonesia, and also in Brazil using these sterile males created by the use of Wolbachia (Blagrove et al. Citation2012). Wolbachia-infected mosquitoes need to be realesed over a wide geographical area as the A. aegypti has a poor flight range and also, it can evolve mechanisms to resist Wolbachia (Weaver et al. Citation2016). Other techniques like irradiation have been carried out to generate sterile males to control the mosquito population (Alphey Citation2014).
Genetic modification of mosquitoes is another approach that can be used to control its population by interrupting their reproduction. Two different approaches can be adopted to achieve this as– (1) suppression or complete eradication of the vectors with the aid of genes that can kill the vector or make them sterile and (2) substitution method where a constructed gene will prevent the transmission of disease (Reis-Castro Citation2012; Carvalho et al. Citation2014). The approach of using sterile male is not new since it is an age-old approach which has successfully aided in local control of certain vectors like Cochliomyia hominivorax (New World screwworm fly) in the United States of America, Central America, and Mexico, the Ceratitis capitata (Mediterranean fruit fly) and Pectinophora gossypiella (pink bollworm) in the United States of America, Cydia pomonella (codling moth) in Canada and Glossina fuscipes (tsetse fly) in Zanzibar (Alphey et al. Citation2010; Burt Citation2014). Use of gamma irradiation that can damage the DNA leading to mutation has been the theme of sterile male techniques (Alphey Citation2014). Use of sterile male mosquitoes of A. aegypti in the Grand Cayman (first experiment of its kind) resulted in around 80% reduction in the population of wild mosquito (Harris et al. Citation2012). Oxitec released in a trial in Piracicaba, Brazil, an engineered mosquitoe with ‘self-limiting’ gene that halts the reproduction of other mosquitoes by not allowing their offsprings to develope into adult mosquitoes, revealed 82% larval reduction during last year (Al-Qahtani et al. Citation2016).
Apart from vector control, proper surveillance and monitoring has to be carried out at the highest level in countries where there are reports and also countries adjoining them. Strict biosecurity measures need to be ensured so that disease transmission can be kept under check (Musso & Nhan Citation2015). All international airports, harbors, and places of international tourist attraction is needed to be under the scanner for the screening of this important disease. Especially pregnant woman should be advised not to travel to countries under threat of Zika viral disease and is advised to avoid travel to areas below 2000 m of sea level since a study reported that mosquito-borne Zika viral infection is possible above 2000 m (Petersen et al. Citation2016c; Cetron Citation2016). Several guidelines have been issued periodically by CDC and other health departments regarding do's and dont's during an outbreak (Oster et al. Citation2016; Ahmad et al. Citation2016; Fleming-Dutra Citation2016) and need to be strictly followed. Since sexual transmission has been reported recently in humans, guidelines have been issued to both men and women that have to be adopted to prevent further spread. These guidelines include: (1) men returning from an area of Zika disease should avoid or have safe sex with partner (Oster et al. Citation2016), and (2) a recent study also reports that Zika virus has been detected in semen though it could not be detected in blood hence men who traveled to countries under disease outbreak or who had been bitten by Aedes mosquitoes should be screened since cases are mostly (80%) asymptomatic (Musso, Roche, Robin, et al. Citation2015). Summer Olympics 2016 is around the corner hence thorough surveillance is needed as Brazil is worst hit by this virus so as to prevent further damage to the human population (Bogoch et al. Citation2016). An overview of prevention and control of Zika virus is presented in
10. Vaccines
Vaccines are not yet available to counter the Zika virus infection (Borchardt Citation2016). The development of Zika virus vaccine requires a long time-line process. Only after the recent emergence and high public health concerns associated with this virus, efforts are being made towards developing a vaccine which could be in production by this year end (Dyer Citation2016a; O'Dowd Citation2016). As a front-runner in the race for a Zika virus vaccine development, this perspective Zika virus vaccine could easily be produced to very high levels in a short time period, and could meet the demand to some extent of any sudden emergency appearing in future. Around 23 groups from different countries are working on the development of vaccine against Zika (Gulland Citation2016c). Approaches employed for the development of vaccine against three Flaviviruses (Yellow fever virus, Japanses encephalitis virus, and tick-borne encephalitis virus) can be used for zika vaccine development. An ideal Zika virus vaccine should have important qualities like administration of a single dose vaccination protecting from Zika viral disease, a safe vaccine which can be used in pregnancy, and rendering long lasting immunity (Palacios et al. Citation2016). Apart from conventional vaccines (live attenuated or inactivated), newer and advanced technologies of vaccine development need to be explored to their full potential for developing effective vaccine against Zika virus such as DNA vaccines, subunit vaccines, recombinant vaccines, vectored vaccines, transgenic plant vaccines, reverse vaccinology, virus‐like particles, gene/mutant‐deleted vaccines, nanovaccines, and adjuvanted vaccines which are showing promising results for countering various infectious pathogens (Petrovsky and Aguilar Citation2004; Dhama, et al. Citation2008; Dhama, Wani, et al. Citation2013; Delany et al. Citation2014; Finco and Rappuoli Citation2014; Kim et al. Citation2014; Reed et al. Citation2013; Singh et al. Citation2015; Zhou et al. Citation2014; Pany et al. Citation2015; Ulmer et al. Citation2015). A futuristic perspective needs to have a better understanding of the immune mechanisms, immunobiology, pathogenesis and molecular characterization of Zika virus, necessary modifications of vaccine components with immunogenic antigens, and incorporating modern adjuvants such as TLRs, improving the vaccine delivery systems and appropriate immunomodulatory approaches.
11. Treatment
Currently, there is no antiviral drug that can save the human population against potential pandemic threats of Zika virus. Only supportive treatment is in use for Zika virus disease like rest, use of fluids, and analgesics to reduce pain and antipyretics to reduce fever (Petersen et al. Citation2016c). Very recently, there is a report from China on the use of Xiyanping injection combined with supportive therapy with promising results (Deng et al. Citation2016). Xiyanping is a semi-synthetic component extracted from Andrographis paniculata, a Chinese herb (Xiao et al. Citation2013). The chemical component present in Xiyanping is 9-dehydro-17-hydro-andrographolide and sodium 9-dehydro-17-hydro-andrographolide-19-yl sulfate and possesses anti-inflammatory and antiviral activity (Chong et al. Citation2013). Antipyretics-like acetaminophen or dipyrone can be used to reduce fever, while aspirin should be avoided to reduce hemorrhages until confirmation of Dengue (Rasmussen et al. Citation2011; Petersen, Staples, et al. Citation2016). Although aspirin and other non-steroidal anti-inflammatory drugs are not typically used in pregnancy, these medications should specifically be avoided until Dengue is ruled out (Petersen, Staples, et al. Citation2016). Pregnant women with confirmed Zika viral disease should regularly have a check up of the fetus to assess its health (Petersen, Staples, et al. Citation2016). Review of the literature reveals that upcoming and emerging therapeutic regimens have not been explored yet for possibilities to treat Zika viral disease, as have been studied recently with other infectious pathogens and are showing promising therapeutic values for treating various diseases including viral diseases. Like for countering recent pandemic threats of flu viruses (bird flu and swine flu), Tamiflu was developed and similarly for Ebola virus emergency ZMapp (humanized mouse antibodies) was developed. There is also a dire need to find out the suitable antiviral drug for prevention and control of Zika virus. Hence, seeing the public health significance of this virus and global concerns, research needs immediate attention to exploit valuable therapeutic options of novel and emerging/upcoming regimens such as cytokines, RNA polymerase inhibitors, microRNA (mi-RNAs), small interfering RNA (si-RNA), avian egg yolk antibodies (IgY), phages, toll-like receptors (TLRs), probiotics, herbs/plant extracts, nutritional immunomodulation, immunotherapeutic, and nanotechnology-based treatments along with attempting for drug discovery and developments (Blecher et al. Citation2011; Kawadkar et al. Citation2011; Dhama, Chakraborty, et al. Citation2013; Dhama,,Chakraborty, et al. Citation2014; Dhama, Saminathan, et al. Citation2015; Mahima et al. Citation2012; Malik et al. Citation2013; Junquera et al. Citation2014; Tiwari et al. Citation2014). For this purpose, much research and clinical trials are required to find a suitable and/or novel therapeutic to treat and control the disease
12. Conclusion and future prospectives
A few years back, Zika virus was thought to be innocuous but since its emergence into pandemic status, now the researchers are searching globally for a solution to stop its spread. The main mode of transmission of Zika virus is mosquito species (Aedes), which is also serving as a vector for other related Flaviviruses such as Dengue and Chikungunya. Although Zika virus is currently a concern in Western Hemisphere, it may not take the time to reach the remaining parts of the world, due to its vector and rapid movement of the human population globally. Though there are also other modes of virus transmission such as intrauterine, sexual intercourse, and blood transfusion, priority should be given to the mosquito control. For example, the use of Wolbachia pipientis – a common bacterium that infects mosquitoes as well as genetically modified mosquitoes – may reduce the spread of these viruses. However, much research needs to assess their effectiveness and safety. Since the pathogenic potential of Zika virus is comparatively new, not much information is available regarding its genetic characterization which is most important in understanding – why the virus became virulent?, What kind of mutation is involved regarding its virulence?, What are molecular differences between mosquito, mice, and human adopted by viruses? The other areas of interest are host–pathogen interaction studies which will provide the answers to how the viruses are crossing the placental barrier? Why it causes microcephaly and/or neurological disorders? Why is the Brazilian population more susceptible to Zika virus infection especially regarding fetal abnormalities? As the currently available serological assays including neutralization assay are cross-reactive with other related Flaviviruses such as Yellow Fever, Dengue, and Chikungunya, a subsequent confirmatory test is necessary. Therefore, identifying the unique portion of Zika virus genome and developing recombinant-based diagnostic assays are in immediate need. Abortion has been banned in many countries of the world, hence strict precautionary measures needs to be undertaken by the pregnant women residing in the countries with Zika virus disease so as to prevent damage to the foetus. Currently, there is no vaccine available and scientists should focus on isolation and development of vaccine on war foot basis. Currently, detailed epidemiological data are scarcly available and these should be extended, including developing countries, to be able to determine the true prevalence of the virus and disease manifestations within their borders. Can domestic animals get infected with Zika virus? – the veterinary community should think about it and start the research immediately. Finally, researchers and policy-makers should be generous enough to share their data between researchers/countries.
Acknowledgments
All the authors acknowledge the support from their respective institutions and universities.
Disclosure statement
Authors declare that there is no conflict of interest that could possibly arise.
References
- Adekolu-John EO, Fagbami AH. 1983. Arthropod-borne virus antibodies in sera of residents of Kainji Lake Basin, Nigeria 1980. Trans R Soc Trop Med Hyg. 77:149–151.
- Ahmad SS, Amin TN, Ustianowski A. 2016. Zika virus: management of infection and risk. BMJ. 352:i1062. doi:10.1136/bmj.i1062
- Akoua-Koffi C, Diarrassouba S, Benie VB, Ngbichi JM, Bozoua T, Bosson A, Akran V, Carnevale P, Ehouman A. 2001. Investigation surrounding a fatal case of Yellow Fever in Côte d'Ivoire in 1999. Bull Société Pathol Exot. 94:227–230.
- Alera MT, Hermann L, Tac-An IA, Klungthong C, Rutvisuttinunt W, Manasatienkij W, Villa D, Thaisomboonsuk B, Velasco JM, Chinnawirotpisan P, et al. 2015. Zika virus infection, Philippines, 2012. Emerg Infect Dis. 21:722–724.
- Alphey L. 2014. Genetic control of mosquitoes. Annu Rev Entomol. 59:205–224.
- Alphey L, Benedict M, Bellini R, Clark GG, Dame DA, Service MW, Dobson SL. 2010. Sterile-insect methods for control of mosquito-borne diseases: an analysis. Vector Borne Zoonotic Dis. 10:295–311.
- Al-Qahtani AA, Nazir N, Al-Anazi MR, Rubino S, Al-Ahdal MN. 2016. Zika virus: a new pandemic threat. J Infect Dev Ctries. 10:201–207.
- Araújo HRC, Carvalho DO, Ioshino RS, Costa-da-Silva AL, Capurro ML. 2015. Aedes aegypti control strategies in Brazil: incorporation of new technologies to overcome the persistence of dengue epidemics. Insects. 6:576–594.
- Attar N. 2016. ZIKA virus circulates in new regions. Nat Rev Microbiol. 14:62.
- Aubry M, Finke J, Teissier A, Roche C, Broult J, Paulous S, Desprès P, Cao-Lormeau VM, Musso D. 2015. Seroprevalence of arboviruses among blood donors in French Polynesia, 2011-2013. Int J Infect Dis. 41:11–12.
- Baba SS, Fagbami AH, Ojeh CK. 1999. Preliminary studies on the use of solid-phase immunosorbent techniques for the rapid detection of Wesselsbron virus (WSLV) IgM by haemagglutination-inhibition. Comp Immunol Microbiol Infect Dis. 22:71–79.
- Babaniyi OA, Mwaba P, Mulenga D, Monze M, Songolo P, Mazaba-Liwewe ML, Mweene-Ndumba I, Masaninga F, Chizema E, Eshetu-Shibeshi M, et al. 2015. Risk assessment for yellow fever in western and north-western provinces of Zambia. J Glob Infect Dis. 7:11–17.
- Balm MN, Lee CK, Lee HK, Chiu L, Koay ES, Tang JW. 2012. A diagnostic polymerase chain reaction assay for Zika virus. J Med Virol. 84:1501–1505.
- Baronti C, Piorkowski G, Charrel RN, Boubis L, Leparc-Goffart I, de Lamballerie X. 2014. Complete coding sequence of zika virus from a French Polynesia outbreak in 2013. Genome Announc. 2:e00500–e00514.
- Barrera-Cruz A, Díaz-Ramos RD, López-Morales AB, Grajales-Muñiz C, Viniegra-Osorio A, Zaldívar-Cervera JA, Arriaga-Dávila JJ. 2016. Technical guidelines for the prevention, diagnosis and treatment of Zika virus infection. Rev Med Inst Mex Seguro Soc. 54:211–224.
- Barzon L, Pacenti M, Berto A, Sinigaglia A, Franchin E, Lavezzo E, Brugnaro P, Palù G. 2016. Isolation of infectious Zika virus from saliva and prolonged viral RNA shedding in a traveller returning from the Dominican Republic to Italy, January 2016. Euro Surveill. 21:1–5.
- Basarab M, Bowman C, Aarons EJ, Cropley I. 2016. Zika virus. BMJ. 352:1–7.
- Bell TM, Field EJ, Narang HK. 1971. Zika virus infection of the central nervous system of mice. Arch Gesamte Virusforsch. 35:183–193.
- Bergquist R. 2011. New tools for epidemiology: a space odyssey. Mem Inst Oswaldo Cruz. 106:892–900.
- Berthet N, Nakouné E, Kamgang B, Selekon B, Descorps-Declère S, Gessain A, Manuguerra JC, Kazanji M. 2014. Molecular characterization of three Zika flaviviruses obtained from sylvatic mosquitoes in the Central African Republic. Vector Borne Zoonotic Dis. 14:862–865.
- Besnard M, Lastere S, Teissier A, Cao-Lormeau V, Musso D. 2014. Evidence of perinatal transmission of Zika virus, French Polynesia, December 2013 and February 2014. Euro Surveill. 19:1–4.
- Blagrove MSC, Arias-Goeta C, Failloux AB, Sinkins SP. 2012. Wolbachia strain wMel induces cytoplasmic incompatibility and blocks dengue transmission in Aedes albopictus. Proc Natl Acad Sci. 109:255–260.
- Blecher K, Nasir A, Friedman A. 2011. The growing role of nanotechnology in combating infectious disease. Virulence, 2:395–401.
- Blitvich BJ, Firth AE. 2015. Insect-specific flaviviruses: a systematic review of their discovery, host range, mode of transmission, superinfection exclusion potential and genomic organization. Viruses. 7:1927–1959.
- Bogoch II, Brady OJ, Kraemer MU, German M, Creatore MI, Kulkarni MA, Brownstein JS, Mekaru SR, Hay SI, Groot E, et al. 2016. Anticipating the international spread of Zika virus from Brazil. Lancet. 387:335–336.
- Bollati M, Alvarez K, Assenberg R, Baronti C, Canard B, Cook S, Coutard B, Decroly E, de Lamballerie X, Gould EA. 2010. Structure and functionality in flavivirus NS-proteins: perspectives for drug design. Antiviral Res. 87:125–148.
- Borchardt RA. 2016. Zika virus: a rapidly emerging infectious disease. JAAPA. 29:48–50.
- Boyce R, Lenhart A, Kroeger A, Velayudhan R, Roberts B, Horstick O. 2013. Bacillus thuringiensis israelensis (Bti) for the control of dengue vectors: systematic literature review. Trop Med Int Health. 18:564–577.
- Brelsfoard CL, Dobson SL. 2009. Wolbachia-based strategies to control insect pests and disease vectors. Asia Pac J Mol Biol Biotechnol. 17:55–63.
- Brown C. 2015. Zika virus outbreaks in Asia and South America. CMAJ 188:1.
- Buathong R, Hermann L, Thaisomboonsuk B, Rutvisuttinunt W, Klungthong C, Chinnawirotpisan P, Manasatienkij W, Nisalak A, Fernandez S, Yoon IK, et al. 2015. Detection of Zika virus infection in Thailand, 2012-2014. Am J Trop Med Hyg. 93:380–383.
- Burke RM, Pandya P, Nastouli E, Gothard P. 2016. Zika virus infection during pregnancy: what, where, and why? Br J Gen Pract. 66:122–123.
- Burt A. 2014. Heritable strategies for controlling insect vectors of disease. Philos Trans R Soc Lond B Biol Sci. 369:1–8.
- Calvet GA, Filippis AM, Mendonça MC, Sequeira PC, Siqueira AM, Veloso VG, Nogueira RM, Brasil P. 2016. First detection of autochthonous Zika virus transmission in a HIV-infected patient in Rio de Janeiro, Brazil. J Clin Virol. 74:1–3.
- Calvet G, Aguiar RS, Melo ASO, Sampaio SA, de Filippis I, Fabri A, Araujo ESM, de Sequeira PC, de Mendonça MCL, de Oliveira L, et al. 2016. Detection and sequencing of Zika virus from amniotic fluid of fetuses with microcephaly in Brazil: a case study. Lancet Infect Dis. Available from: http://dx.doi.org/10.1016/S1473-3099(16)00095-5
- Campos GS, Bandeira AC, Sardi SI. 2015. Zika virus outbreak, Bahia, Brazil. Emerg Infect Dis. 21:1885–1886.
- Cao-Lormeau VM, Roche C, Teissier A, Robin E, Berry AL, Mallet HP, Sall AA, Musso D. 2014. Zika virus, French Polynesia, South Pacific, 2013. Emerg Infect Dis. 20:1085–1086.
- Carneiro LA, Travassos LH. 2016. Autophagy and viral diseases transmitted by Aedes aegypti and Aedes albopictus. Microbes Infect. 18:169–171.
- Carvalho DO, Costa-da-Silva AL, Lees RS, Capurro ML. 2014. Two step male release strategy using transgenic mosquito lines to control transmission of vector-borne diseases. Acta Trop. 132:1–8.
- CDC. 2016. Media statement: CDC adds 4 destinations to interim travel guidance related to Zika virus. 1 February 2016 [Internet]. Atlanta: CDC.
- Cetron M. 2016. Revision to CDC's Zika travel notices: minimal likelihood for mosquito-borne Zika virus transmission at elevations above 2000 meters. Morb Mortal Wkly Rep. 65:267–268.
- Chan JF, Choi GK, Yip CC, Cheng VC, Yuen KY. 2016. Zika fever and congenital Zika syndrome: an unexpected emerging arboviral disease. J Infect. 72:507–524.
- Chang C, Ortiz K, Ansari A, Gershwin ME. 2016. The Zika outbreak of the 21st century. J Autoimmun. 68:1–13.
- Chastel C. 2012. When some Flaviviruses are throwing our certainties. Bull Soc Pathol Exot. 105:251–255.
- Chattopadhyay S, Bagchi P, Dutta D, Mukherjee A, Kobayashi N, Chawlasarkar M. 2010. Computational identification of posttranslational modification sites and functional families reveal possible moonlighting role of rotaviral proteins. Bioinformation. 4:448–451.
- Chen LH, Hamer DH. 2016. Zika virus: rapid spread in the western hemisphere. Ann Intern Med. doi:10.7326/M16-0150
- Chen LH, Wilson ME. 2010. Dengue and chikungunya infections in travelers. Curr Opin Infect Dis. 23:438–444.
- Chong L, Chen W, Luo Y, Jiang Z. 2013. Simultaneous determination of 9-dehydro-17-hydro-andrographolide and sodium 9-dehydro-17-hydro-andrographolide-19-yl sulfate in rat plasma by UHPLC-ESI-MS/MS after administration of xiyanping injection: application to a pharmacokinetic study. Biomed Chromatogr. 27:825–830.
- Choumet V, Desprès P. 2015. Dengue and other flavivirus infections. Rev Sci Tech. 34:473–478.
- Citrome L. 2016. Zika and You. Int J Clin Pract. 70:181–182.
- Clement M, Snell Q, Walker P, Posada D, Crandall K. 2000. TCS: estimating gene genealogies. Mol Ecol. 9:1657–1659.
- Cook S, Holmes EC. 2006. A multigene analysis of the phylogenetic relationships among the flaviviruses (family: Flaviviridae) and the evolution of vector transmission. Arch Virol. 151:309–325.
- Daep CA, Muñoz-Jordán JL, Eugenin EA. 2014. Flaviviruses, an expanding threat in public health: focus on dengue, West Nile, and Japanese encephalitis virus. J. Neurovirol. 20:539–560.
- Darbro JM, Johnson PH, Thomas MB, Ritchie SA, Kay BH, Ryan PA. 2012. Effects of Beauveria bassiana on survival, blood-feeding success, and fecundity of Aedes aegypti in laboratory and semi-fi eld conditions. Am J Trop M. 86:656–664.
- Darwish MA, Hoogstraal H, Roberts TJ, Ahmed IP, Omar F. 1983. A sero-epidemiological survey for certain arboviruses (Togaviridae) in Pakistan. Trans R Soc Trop Med Hyg. 77:442–445.
- Delany I, Rappuoli R, De Gregorio E. 2014. Vaccines for the 21st century. EMBO Mol Med. 6:708–720.
- de M Campos R, Cirne-Santos C, Meira GL, Santos LL, de Meneses MD, Friedrich J, Jansen S, Ribeiro MS, da Cruz IC, Schmidt-Chanasit J, Ferreira DF. 2016. Prolonged detection of Zika virus RNA in urine samples during the ongoing Zika virus epidemic in Brazil. J Clin Virol. 77:69–70.
- Deng Y, Zeng L, Bao W, Xu P, Zhong G. 2016. Experience of integrated traditional Chinese and Western medicine in first case of imported Zika virus disease in China. Zhonghua Wei Zhong Bing Ji Jiu Yi Xue. 28:106–109.
- de Paula Freitas B, de Oliveira Dias JR, Prazeres J, Sacramento GA, Ko AI, Maia M, Belfort R Jr. 2016. Ocular findings in infants with microcephaly associated with presumed Zika virus congenital infection in Salvador, Brazil. JAMA Ophthalmol. 134:529–535.
- Derraik JG, Slaney D. 2015. Notes on Zika virus–an emerging pathogen now present in the South Pacific. Aust N Z J Public Health. 39:5–7.
- Dhama K, Chakraborty S, Mahima , Wani MY, Verma AK, Deb R, Tiwari R, Kapoor S. 2013. Novel and emerging therapies safeguarding health of humans and their companion animals: a review. Pak J Biol Sci. 16:1–11.
- Dhama K, Chakraborty S, Tiwari R, Verma AK, Saminathan M, Malik YS, Nikousefat Z, Javdani M, Khan RU. 2014. A concept paper on novel technologies boosting production and safeguarding health of humans and animals. Res Opin Anim Vet Sci. 4:353–370.
- Dhama K, Karthik K, Chakraborty S, Tiwari R, Kapoor S, Kumar A, Thomas P. 2014. Loop-mediated isothermal amplification of DNA (LAMP)–a new diagnostic tool lights the world of diagnosis of animal and human pathogens: areview. Pak J Biol Sci. 17:151–166.
- Dhama K, Mahendran M, Gupta PK, Rai A. 2008. DNA vaccines and their applications in veterinary practice: current perspectives. Vet Res Commun. 32:341–356.
- Dhama K, Malik YS, Malik SV, Singh RK. 2015. Ebola from emergence to epidemic: the virus and the disease, global preparedness and perspectives. J Infect Dev Ctries. 9:441–455.
- Dhama K, Saminathan M, Jacob SS, Singh M, Karthik K, Amarpal , Tiwari R, Sunkara LT, Malik YS, Singh RK. 2015. Effect of immunomodulation and immunomodulatory agents on health with some bioactive principles, modes of action and potent biomedical applications. Int J Pharmacol. 11:253–290.
- Dhama K, Tiwari R, Chakraborty S, Kumar A, Karikalan M, Singh R, Rai RB. 2013. Global warming and emerging infectious diseases of animals and humans: current scenario, challenges, solutions and future perspectives–a review. Int J Curr Res. 5:1942–1958.
- Dhama K, Wani MY, Deb R, Karthik K, Tiwari R, Barathidasan R, Kumar A, Mahima , Verma AK, Singh SD. 2013. Plant based oral vaccines for human and animal pathogens–a new era of prophylaxis: current and future perspectives. J Exp Biol Agri Sci. 1:1–12.
- Dhiman RC, Pahwa S, Dhillon GP, Dash AP. 2010. Climate change and threat of vector-borne diseases in India: are we prepared? Parasitol Res. 106:763–773.
- Diagne CT, Diallo D, Faye O, Ba Y, Faye O, Gaye A, Dia I, Faye O, Weaver SC, Sall AA, Diallo M. 2015. Potential of selected Senegalese Aedes spp. mosquitoes (Diptera: Culicidae) to transmit Zika virus. BMC Infect Dis. 15:492–497.
- Dias CN, Fernandes D, Moraes, C. 2014. Essential oils and their compounds as Aedes aegypti L. (Diptera: Culicidae) larvicides : review. Parasitol Res. 113:565–592.
- Dick GW. 1952. Zika virus. II. Pathogenicity and physical properties. Trans R Soc Trop Med Hyg. 46:521–534.
- Dick GW. 1953. Epidemiological notes on some viruses isolated in Uganda; Yellow fever, Rift Valley fever, Bwamba fever, West Nile, Mengo, Semliki forest, Bunyamwera, Ntaya, Uganda S and Zika viruses. Trans R Soc Trop Med Hyg. 47:13–48.
- Dick GW, Kitchen SF, Haddow AJ. 1952. Zika virus. I. Isolations and serological specificity. Trans R Soc Trop Med Hyg. 46:509–520.
- Dick OB, San Martín JL, Montoya RH, del Diego J, Zambrano B, Dayan GH. 2012. Review: the history of dengue outbreaks in the Americas. Am J Trop Med Hyg. 87:584–593.
- Driggers RW, Ho CY, Korhonen EM, Kuivanen S, Jääskeläinen AJ, Smura T, Rosenberg A, Hill DA, DeBiasi RL, Vezina G, et al. 2016. Zika virus infection with prolonged maternal viremia and fetal brain abnormalities. N Engl J Med. doi:10.1056/NEJMoa1601824
- Duffy MR, Chen TH, Hancock WT, Powers AM, Kool JL, Lanciotti RS, Pretrick M, Marfel M, Holzbauer S, Dubray C, et al. 2009. Zika virus outbreak on Yap Island, Federated States of Micronesia. N Engl J Med. 360:2536–2543.
- Dyer O. 2015. Zika virus spreads across Americas as concerns mount over birth defects. BMJ. 351:1–2.
- Dyer O. 2016a. Zika vaccine could be in production by year's end, says maker. BMJ. 352:1.
- Dyer O. 2016b. Sixty seconds on Zika virus. BMJ. 352:1.
- Elachola H, Gozzer E, Zhuo J, Memish ZA. 2016. A crucial time for public health preparedness: Zika virus and the 2016 Olympics, Umrah, and Hajj. Lancet. 387:630–632.
- Enfissi A, Codrington J, Roosblad J, Kazanji M, Rousset D. 2016. Zika virus genome from the Americas. Lancet. 387:227–228.
- European CDC. 2014. Rapid risk assessment: Zika virus infection outbreak, French Polynesia. 14 February 2014. Stockholm: ECDC.
- Faccini-Martínez ÁA, Botero-García CA, Benítez-Baracaldo FC, Pérez-Díaz CE. 2016. With regard about the case of Dengue, Chikungunya and Zika co-infection in a patient from Colombia. J Infect Public Health. doi:10.1016/j.jiph.2016.01.001
- Fagbami A. 1977. Epidemiological investigations on arbovirus infections at Igbo-Ora, Nigeria. Trop Geogr Med. 29:187–191.
- Fagbami AH. 1979 Zika virus infections in Nigeria: virological and seroepidemiological investigations in Oyo State. J Hyg (Lond). 83:213–219.
- Fauci AS, Morens DM. 2016. Zika virus in the Americas–yet another Arbovirus threat. N Engl J Med. 374:601–604.
- Favia G, Ricci I, Marzorati M, Negri I, Alma A, Sacchi L, Bandi C, Daffonchio D. 2008. Bacteria of the genus Asaia: a potential paratransgenic weapon against malaria. Adv Exp Med Biol. 627:49–59.
- Faye O, Faye O, Diallo D, Diallo M, Weidmann M, Sall AA. 2013. Quantitative real-time PCR detection of Zika virus and evaluation with field-caught mosquitoes. Virol J. 10:311–318.
- Faye O, Faye O, Dupressoir A, Weidmann M, Ndiaye M, Alpha Sall A. 2008. One-step RT-PCR for detection of Zika virus. J Clin Virol. 43:96–101.
- Faye O, Freire CC, Iamarino A, Faye O, de Oliveira JV, Diallo M, Zanotto PM, Sall AA. 2014. Molecular evolution of Zika virus during its emergence in the 20(th) century. PLoS Negl Trop Dis. 8:1–10.
- Finco O, Rappuoli R. 2014. Designing vaccines for the twenty-first century society. Front Immunol. 5:12–17.
- Fleming-Dutra, KE. 2016. Update: interim guidelines for health care providers caring for infants and children with possible Zika virus infection—United States. Morb. Mort. Wkly Rep. 65:182–187.
- Fokam EB, Levai LD, Guzman H, Amelia PA, Titanji VP, Tesh RB, Weaver SC. 2010. Silent circulation of arboviruses in Cameroon. East Afr Med J. 87:262–268.
- Fonseca K, Meatherall B, Zarra D, Drebot M, MacDonald J, Pabbaraju K, Wong S, Webster P, Lindsay R, Tellier R. 2014. First case of Zika virus infection in a returning Canadian traveler. Am J Trop Med Hyg. 91:1035–1038.
- Fontes BM. 2016. Zika virus-related hypertensive iridocyclitis. Arq Bras Oftalmol. 79:63.
- Foy BD, Kobylinski KC, Chilson Foy JL, Blitvich BJ, Travassos da Rosa A, Haddow AD, Lanciotti RS, Tesh RB. 2011. Probable non-vector-borne transmission of Zika virus, Colorado, USA. Emerg Infect Dis. 17:880–882.
- Franchini M, Velati C. 2015. Blood safety and zoonotic emerging pathogens: now it's the turn of Zika virus! Blood Transfus. 14:93–94.
- Frank C, Faber M, Stark K. 2016. Causal or not: applying the Bradford Hill aspects of evidence to the association between Zika virus and microcephaly. EMBO Mol Med. 8:305–307.
- Frentiu FD, Zakir T, Walker T, Popovici J, Pyke AT, van den Hurk A, McGraw EA, O'Neill SL. 2014. Limited dengue virus replication in field-collected Aedes aegypti mosquitoes infected with Wolbachia. PLoS Negl Trop Dis. 8:e2688.
- Gatherer D, Kohl A. 2016. Zika virus: a previously slow pandemic spreads rapidly through the Americas. J Gen Virol. 97, 269–273.
- Gautret P, Simon F. 2016. Dengue, chikungunya and Zika and mass gatherings: what happened in Brazil, 2014. Travel Med Infect Dis. 14:7–8.
- Geser A, Henderson BE, Christensen S. 1970. A multipurpose serological survey in Kenya. 2. Results of arbovirus serological tests. Bull World Health Organ. 43:539–552.
- Ghosh A, Chowdhury N, Chandra G. 2012. Plant extracts as potential mosquito larvicides. Indian J Med Res. 135:581–598.
- Goeijenbier M, Slobbe L, van der Eijk A, de Mendonça Melo M, Koopmans MPG, Reusken CBEM. 2016. Zika virus and the current outbreak: an overview. Netherlands J Med. 74:104–109.
- Goma LK. 1965. The flight activity of some East African mosquitoes (Diptera, Culicidae). I. Studies on a high steel tower in Zika forest, Uganda. Bull Entomol Res. 56:17–35.
- Gourinat AC, O'Connor O, Calvez E, Goarant C, Dupont-Rouzeyrol M. 2015. Detection of Zika virus in urine. Emerg Infect Dis. 21:84–86.
- Grard G, Caron M, Mombo IM, Nkoghe D, Mboui Ondo S, Jiolle D, Fontenille D, Paupy C, Leroy EM. 2014. Zika virus in Gabon (Central Africa)–2007: a new threat from Aedes albopictus? PLoS Negl Trop Dis. 8:e2681.
- Gulland A. 2016a. WHO urges countries in dengue belt to look out for Zika. BMJ. 352:i595.
- Gulland A. 2016b. Zika virus is a global public health emergency, declares WHO. BMJ. 352:i657.
- Gulland A. 2016c. WHO's Chan warns over spread of Zika virus beyond Latin America. BMJ. 352:i1739. doi:10.1136/bmj.i1739.
- Haddow AJ, Williams MC, Woodall JP, Simpson DI, Goma LK. 1964. Twelve isolations of zika virus from aedes (stegomyia) africanus (theobald) taken in and above a Uganda forest. Bull World Health Organ. 31:57–69.
- Haddow AD, Schuh AJ, Yasuda CY, Kasper MR, Heang V, Huy R, Guzman H, Tesh RB, Weaver SC. 2012. Genetic characterization of Zika virus strains: geographic expansion of the Asian lineage. PLoS Negl Trop Dis. 6:e1477.
- Hamel R, Dejarnac O, Wichit S, Ekchariyawat P, Neyret A, Luplertlop N, Perera-Lecoin M, Surasombatpattana P, Talignani L, Thomas F, et al. 2015. Biology of Zika virus infection in human skin cells. J Virol. 89:8880–8896.
- Hammon WM, Schrack WD, Sather GE. 1958. Serological survey for a arthropod-borne virus infections in the Philippines. Am J Trop Med Hyg. 7:323–328.
- Hanada K, Suzuki Y, Gojobori T. 2004. A large variation in the rates of synonymous substitution for RNA viruses and its relationship to a diversity of viral infection and transmission modes. Mol Biol Evol. 21:1074–1080.
- Hancock WT, Marfel M, Bel M. 2014. Zika virus, French Polynesia, South Pacific, 2013. Emerg Infect Dis. 20:1960.
- Hanna SL, Pierson TC, Sanchez MD, Ahmed AA, Murtadha MM. 2005. N-linked glycosylation of West Nile virus envelope proteins influences particle assembly and infectivity. J Virol. 79:13262–13274.
- Harris AF, McKemey AR, Nimmo D, Curtis Z, Black I, Morgan SA, Oviedo MN, Lacroix R, Naish N, Morrison NI, et al. 2012. Successful suppression of a field mosquito population by sustained release of engineered male mosquitoes. Nat Biotechnol. 30:828–830.
- Haug CJ, Kieny MP, Murgue B. 2016. The Zika Challenge. N Engl J Med. 374:1801–1803.
- Hayes EB. 2009. Zika virus outside Africa. Emerg Infect Dis. 15:1347–1350.
- Heang V, Yasuda CY, Sovann L, Haddow AD, Travassos da Rosa AP, Tesh RB, Kasper MR. 2012. Zika virus infection, Cambodia, 2010. Emerg Infect Dis. 18:349–351.
- Henderson BE, Kirya GB, Hewitt LE. 1970. Serological survey for arboviruses in Uganda, 1967-69. Bull World Health Organ. 42:797–805.
- Henderson BE, Metselaar D, Cahill K, Timms GL, Tukei PM, Williams MC. 1968. Yellow fever immunity surveys in northern Uganda and Kenya and eastern Somalia, 1966-67. Bull World Health Organ. 38:229–237.
- Hennessey M, Fischer M, Staples JE. 2016. Zika Virus spreads to new areas - region of the Americas, May 2015–January 2016. MMWR Morb Mortal Wkly Rep. 65:55–58.
- Heukelbach J, Alencar CH, Kelvin AA, De Oliveira WK, Pamplona de Góes Cavalcanti L. 2016. Zika virus outbreak in Brazil. J Infect Dev Ctries. 10:116–120.
- Heymann DL, Hodgson A, Sall AA, Freedman DO, Staples JE, Althabe F, Baruah K, Mahmud G, Kandun N, Vasconcelos PF, et al. 2016. Zika virus and microcephaly: why is this situation a PHEIC? Lancet. 387:719–721.
- Higgs S. 2016. Zika virus: emergence and emergency. Vector Borne Zoonotic Dis. 16:75–76.
- Hubálek Z, Rudolf I, Nowotny N. 2014. Arboviruses pathogenic for domestic and wild animals. Adv Virus Res. 89:201–275.
- Ioos S, Mallet HP, Leparc Goffart I, Gauthier V, Cardoso T, Herida M. 2014. Current Zika virus epidemiology and recent epidemics. Med Mal Infect. 44:302–307.
- Jacob JA. 2016. Researchers focus on solving the Zika riddles. JAMA. 315:1097–1099.
- Jain KK. 2007. Applications of nano biotechnology in clinical diagnostics. Clin Chem. 53:2002–2009.
- Jan C, Languillat G, Renaudet J, Robin Y. 1978. A serological survey of arboviruses in Gabon. Bull Soc Pathol Exot Filiales. 71:140–146.
- Junquera EC, Mateos-Hernández L, de la Fuente J, de la Lastra JM. 2014. Recent advances in the development of anti-Infective prophylactic and/or therapeutic agents based on toll-like receptor (TLRs). Recent Pat Antiinfect Drug Discov. 9:14–24.
- Kant R, Haq S, Srivastava HC, Sharma VP. 2013. Review of the bioenvironmental methods for malaria control with special reference to the use of larvivorous fishes and composite fish culture in central Gujarat, India. J Vector Borne Dis. 50:1–12.
- Kapoor S, Dhama K. 2014. Insight into influenza viruses of animals and humans. Cham: Springer International Publishing AG.
- Karwowski MP, Nelson JM, Staples JE, Fischer M, Fleming-Dutra KE, Villanueva J, Powers AM, Mead P, Honein MA, Moore CA, Rasmussen SA. 2016. Zika virus disease: A CDC update for pediatric health care providers. Pediatrics. pii: peds.2016-0621.
- Kawadkar J, Chauhan MK, Maharana M. 2011. Nanobiotechnology: application of nanotechnology in diagnosis, drug discovery and drug development. Asian Pharmaceut Clin Res. 4:23–28.
- Kelser EA. 2016. Meet dengue's cousin, Zika. Microbes Infect. 18:163–166.
- Kelvin AA, Banner D, Pamplona L, Alencar C, Rubino S, Heukelbach J. 2016. ZIKATracker: a mobile App for reporting cases of ZIKV worldwide. J Infect Dev Ctries. 10:113–115.
- Kilbourn AM, Karesh WB, Wolfe ND, Bosi EJ, Cook RA, Andau M. 2003. Health evaluation of free-ranging and semi-captive orangutans (Pongo pygmaeus pygmaeus) in Sabah, Malaysia. J Wildl Dis. 39:73–83.
- Kim M, Park JY, Shon Y, Kim G, Shim G, Oh Y. 2014. Nanotechnology and vaccine development. Asian J Pharm Sci. 9:227–235.
- Kindhauser MK, Allen T, Frank V, Santhana RS, Dye C. 2016. Zika: the origin and spread of a mosquito-borne virus [Submitted]. Bull World Health Organ. E-pub: 9 Feb 2016.
- Kirya BG, Okia NO. 1977. A yellow fever epizootic in Zika Forest, Uganda, during 1972: Part 2: monkey serology. Trans R Soc Trop Med Hyg. 71:300–303.
- Kline MW, Schutze GE. 2016. What pediatricians and other clinicians should know about Zika virus. JAMA Pediatr. 170:309–310.
- Knust B, Rollin PE. 2013. Twenty-year summary of surveillance for human Hantavirus infections, United States. Emerg Infect Dis. 19:1934–1937.
- Korhonen EM, Huhtamo E, Smura T, Kallio-Kokko H, Raassina M, Vapalahti O. 2016. Zika virus infection in a traveller returning from the Maldives, June 2015. Euro Surveill. 21. doi:10.2807/1560-7917.ES.2016.21.2.30107
- Kumar S, Mishra M, Wahab N, Warikoo R. 2014. Larvicidal, repellent, and irritant potential of the seed-derived essential oil of Apium graveolens against dengue vector, Aedes aegypti L. (Diptera: Culicidae). Front Public Health. 2:1–6.
- Kuno G, Chang GJ. 2007. Full-length sequencing and genomic characterization of Bagaza, Kedougou, and Zika viruses. Arch Virol. 152:687–696.
- Kuno G, Chang GJ, Tsuchiya KR, Karabatsos N, Cropp CB. 1998. Phylogeny of the genus Flavivirus. J Virol. 72:73–83.
- Kutsuna S, Kato Y, Takasaki T, Moi M, Kotaki A, Uemura H, Matono T, Fujiya Y, Mawatari M, Takeshita N, et al. 2014. Two cases of Zika fever imported from French Polynesia to Japan, December 2013 to January 2014. Euro Surveill. 19:20683.
- Kwong JC, Druce JD, Leder K. 2013. Zika virus infection acquired during brief travel to Indonesia. Am J Trop Med Hyg. 89:516–517.
- Lanciotti RS, Kosoy OL, Laven JJ, Velez JO, Lambert AJ, Johnson AJ, Stanfield SM, Duffy MR. 2008. Genetic and serologic properties of Zika virus associated with an epidemic, Yap State, Micronesia, 2007. Emerg Infect Dis. 14:1232–1239.
- Lazear HM, Diamond MS. 2016. Zika virus: new clinical syndromes and its emergence in the Western Hemisphere. J Virol. 90:4864–4875.
- Lazear HM, Stringer EM, de Silva AM. 2016. The emerging Zika virus epidemic in the Americas research priorities. JAMA. 315:1945–1946.
- Ledermann JP, Guillaumot L, Yug L, Saweyog SC, Tided M, Machieng P, Pretrick M, Marfel M, Griggs A, Bel M, et al. 2014. Aedes hensilli as a potential vector of Chikungunya and Zika viruses. PLoS Negl Trop Dis. 8:e3188.
- Lee E, Leang SK, Davidson A, Lobigs M. 2010. Both E protein glycans adversely affect dengue virus infectivity but are beneficial for virion release. J Virol. 84:5171–5180.
- Lee SH, Nam KW, Jeong JY, Yoo SJ, Koh YS, Lee S, Heo ST, Seong SY, Lee KH. 2013. The effects of climate change and globalization on mosquito vectors: evidence from Jeju Island, South Korea on the potential for Asian tiger mosquito (Aedes albopictus) influxes and survival from Vietnam rather than Japan. PLoS One. 8:e68512.
- Leung GH, Baird RW, Druce J, Anstey NM. 2015. Zika virus infection in Australia following a monkey bite in Indonesia. Southeast Asian J Trop Med Public Health. 46:460–464.
- Li MI, Wong PS, Ng LC, Tan CH. 2012. Oral susceptibility of Singapore Aedes (Stegomyia) aegypti (Linnaeus) to Zika virus. PLoS Negl Trop Dis. 6:e1792.
- Li XF, Han JF, Shi PY, Qin CF. 2016. Zika virus: a new threat from mosquitoes. Sci China Life Sci. 59:440–442.
- Lindenbach BD, Rice CM. 2003. Molecular biology of flaviviruses. Adv Virus Res. 59:23–61.
- Loriere SE, Holmes EC. 2011. Why do RNA viruses recombine? Nat Rev Microbiol 9:617–626.
- Lucchese G, Kanduc D. 2016. Zika virus and autoimmunity: from mycrocephaly to Guillain-Barré syndrome, and beyond. Autoimmun Rev. pii: S1568-9972(16)30077-5.
- Lucey DR, Gostin LO. 2016. The emerging Zika pandemic: enhancing preparedness. JAMA. 315:865–866.
- MacNamara F. 1952. Annual Report, Virus Research Institute, Nigeria, 1951–1952.
- Macnamara FN. 1954. Zika virus: a report on three cases of human infection during an epidemic of jaundice in Nigeria. Trans R Soc Trop Med Hyg. 48:139–145.
- Mahima Rahal A, Deb R, Latheef SK, Samad HA, Tiwari R, Verma AK, Kumar A, Dhama K. 2012. Immunomodulatory and therapeutic potentials of herbal, traditional/indigenous and ethnoveterinary medicines. Pak J Biol Sci. 15:754–774.
- Malik YS, Sharma K, Jeena LM, Kumar N, Sircar S, Rajak KK, Dhama K. 2013. Toll-like receptors: the innate immune receptors with ingenious anti-viral paradigm. South Asian J Exp Biol. 3:207–213.
- Marano G, Pupella S, Vaglio S, Liumbruno GM, Grazzini G. 2015. Zika virus and the never-ending story of emerging pathogens and transfusion medicine. Blood Transfus. 5:1–6.
- Marchette NJ, Garcia R, Rudnick A. 1969. Isolation of Zika virus from Aedes aegypti mosquitoes in Malaysia. Am J Trop Med Hyg. 18:411–415.
- Marcondes CB, Ximenes MF. 2016. Zika virus in Brazil and the danger of infestation by Aedes (Stegomyia) mosquitoes. Rev Soc Bras Med Trop. 49:4–10. doi: doi.org/10.1590/0037-8682-0220-2015
- Martines RB, Bhatnagar J, Keating MK, Silva-Flannery L, Muehlenbachs A, Gary J, Goldsmith C, Hale G, Ritter J, Rollin D, et al. 2016. Notes from the field: evidence of Zika virus infection in Brain and placental tissues from two congenitally infected newborns and two fetal losses - Brazil, 2015. MMWR Morb Mortal Wkly Rep. 65:159–160.
- Martínez MJ, Salim AM, Hurtado JC, Kilgore PE. 2015. Ebola virus infection: overview and update on prevention and treatment. Infect Dis Ther. 4:365–390.
- Martinez-Pulgarin DF, Acevedo-Mendoza WF, Cardona-Ospina J, Rodríguez-Morales AJ, Paniz-Mondolfi AE. 2016. A bibliometric analysis of global Zika research. Travel Med Infect Dis. 14:55–57.
- McCarthy M. 2016a. First US case of Zika virus infection is identified in Texas. BMJ. 352:i212.
- McCarthy M. 2016b. Zika virus was transmitted by sexual contact in Texas, health officials report. BMJ. 352:i720.
- McCarthy, M. 2016c. US health officials investigate sexually transmitted Zika virus infections. BMJ 352: i1180.
- McCarthy M. 2016d. WHO sets out $56m Zika virus response plan. BMJ. 352:i1042.
- McCarthy M. 2016e. Zika virus outbreak prompts US to issue travel alert to pregnant women. BMJ. 352:i306.
- McCarthy M. 2016f. Severe eye damage in infants with microcephaly is presumed to be due to Zika virus. BMJ. 352:i855
- McCrae AW, Kirya BG. 1982. Yellow fever and Zika virus epizootics and enzootics in Uganda. Trans R Soc Trop Med Hyg. 76:552–562.
- Medlock JM, Leach SA. 2015. Effect of climate change on vector-borne disease risk in the UK. Lancet Infect Dis. 15:721–730.
- Mlakar J, Korva M, Tul N, Popović M, Poljšak-Prijatelj M, Mraz J, Kolenc M, Resman Rus K, Vesnaver Vipotnik T, Fabjan Vodušek V, et al. 2016. Zika virus associated with microcephaly. N Engl J Med. 352:i855.
- Monath TP, Craven RB, Muth DJ, Trautt CJ, Calisher CH, Fitzgerald SA. 1980. Limitations of the complement-fixation test for distinguishing naturally acquired from vaccine-induced yellow fever infection in flavivirus-hyperendemic areas. Am J Trop Med Hyg. 29:624–634.
- Monath TP, Wilson DC, Casals J. 1973. The 1970 yellow fever epidemic in Okwoga district, Benue Plateau State, Nigeria. 3. Serological responses in persons with and without pre-existing heterologous group B immunity. Bull World Health Organ. 49:235–244.
- Mondotte JA, Lozach P-Y, Amara A, Gamarnik AV. 2007. Essential role of dengue virus envelope protein N glycosylation at asparagine-67 during viral propagation. J Virol. 81:7136–7148.
- Monlun E, Zeller H, Le Guenno B, Traoré-Lamizana M, Hervy JP, Adam F, Ferrara L, Fontenille D, Sylla R, Mondo M. 1993. Surveillance of the circulation of arbovirus of medical interest in the region of eastern Senegal. Bull Soc Pathol Exot. 86:21–28.
- Moore DL, Causey OR, Carey DE, Reddy S, Cooke AR, Akinkugbe FM, David-West TS, Kemp GE. 1975. Arthropod-borne viral infections of man in Nigeria, 1964-1970. Ann Trop Med Parasitol. 69:49–64.
- Mor G. 2016. Placental inflammatory response to Zika virus may affect fetal brain development. Am J Reprod Immunol. 75:421–422.
- Moulin E, Selby K, Cherpillod P, Kaiser L, Boillat-Blanco N. 2016. Simultaneous outbreaks of dengue, chikungunya and Zika virus infections: diagnosis challenge in a returning traveller with nonspecific febrile illness. New Microbes New Infect. 11:6–7. doi:10.1016/j.nmni.2016.02.003
- Musso D, Gubler DJ. 2016. Zika Virus. Clin Microbiol Rev 29:487–524.
- Musso D, Nhan T. 2015. Emergence of Zika Virus. Clin Microbiol. 4:222.
- Musso D, Nhan T, Robin E, Roche C, Bierlaire D, Zisou K, Shan Yan A, Cao-Lormeau VM, Broult J. 2014. Potential for Zika virus transmission through blood transfusion demonstrated during an outbreak in French Polynesia, November 2013 to February 2014. Euro Surveill. 19 pii::20761.
- Musso D, Nilles EJ, Cao-Lormeau VM. 2014. Rapid spread of emerging Zika virus in the Pacific area. Clin Microbiol Infect. 20:O595–O596.
- Musso D, Roche C, Nhan TX, Robin E, Teissier A, Cao-Lormeau VM. 2015. Detection of Zika virus in saliva. J Clin Virol. 68:53–55.
- Musso D, Roche C, Robin E, Nhan T, Teissier A, Cao-Lormeau VM. 2015. Potential sexual transmission of Zika virus. Emerg Infect Dis. 21:359–61.
- Nereida V. 2015. Zika virus: another emerging arbovirus in Venezuela? Invest Clin. 56:241–242.
- Nishiura H, Kinoshita R, Mizumoto K, Yasuda Y, Nah K. 2016. EquationTitle: transmission potential of Zika virus infection in the South Pacific. Int J Infect Dis. 45:95–97.
- Nunes-Alves C. 2016. Public health: Ebola update. Nat Rev Microbiol. 14:131.
- O'Dowd A. 2016. UK records four cases of Zika virus in past six weeks. BMJ. 352:i875. doi:10.1136/bmj.i875
- Oehler E, Watrin L, Larre P, Leparc-Goffart I, Lastere S, Valour F, Baudouin L, Mallet H, Musso D, Ghawche F. 2014. Zika virus infection complicated by Guillain-Barre syndrome-case report, French Polynesia, December 2013. Euro Surveill. 19:20720.
- Oliveira Melo AS, Malinger G, Ximenes R, Szejnfeld PO, Alves Sampaio S, Bispo de Filippis AM. 2016. Zika virus intrauterine infection causes fetal brain abnormality and microcephaly: tip of the iceberg? Ultrasound Obstet Gynecol. 47:6–7.
- Olson JG, Ksiazek TG, Gubler DJ, Lubis SI, Simanjuntak G, Lee VH, Nalim S, Juslis K, See R. 1983. A survey for arboviral antibodies in sera of humans and animals in Lombok, Republic of Indonesia. Ann Trop Med Parasitol. 77:131–137.
- Olson JG, Ksiazek TG, Suhandiman , Triwibowo . 1981. Zika virus, a cause of fever in Central Java, Indonesia. Trans R Soc Trop Med Hyg. 75:389–393.
- Ong KC, Wong KT. 2015. Henipavirus Encephalitis: recent developments and advances. Brain Pathol. 25:605–613.
- Oster AM, Brooks JT, Stryker JE, Kachur RE, Mead P, Pesik NT, Petersen LR. 2016. Interim guidelines for prevention of sexual transmission of Zika virus — United States, 2016. MMWR. 65:120–121.
- Palacios R, Poland GA, Kalil J. 2016. Another emerging arbovirus, another emerging vaccine: targeting Zika virus. Vaccine. 34:2291–2293.
- Pany SS, Mohanty NN, Sarangi LN, Mohapatra JK, Shivachandra SB. 2015. Vaccination: a future perspective. Adv Anim Vet Sci 3:1–8.
- Parham PE, Waldock J, Christophides GK, Michael E. 2015. Climate change and vector-borne diseases of humans. Philos Trans R Soc Lond B Biol Sci. 370. pii: 20140377.
- Pastula DM, Smith DE, Beckham JD, Tyler KL. 2016. Four emerging arboviral diseases in North America: Jamestown Canyon, Powassan, chikungunya, and Zika virusdiseases. J Neurovirol. doi:10.1007/s13365-016-0428-5
- Patiño-Barbosa AM, Medina I, Gil-Restrepo AF, Rodriguez-Morales AJ. 2015. Zika: another sexually transmitted infection? Sex Transm Infect. 91:359.
- Perkasa A, Yudhaputri F, Haryanto S, Hayati RF, Ma'roef CN, Antonjaya U, Yohan B, Myint KSA, Ledermann JP, Rosenberg R. 2016. Isolation of Zika virus from febrile patient, Indonesia. Emerg Infect Dis. [Internet]. [cited 2016 Feb 21]; 22. Available from: http://wwwnc.cdc.gov/eid/article/22/5/15-1915_article.html.
- Pessôa R, Patriota JV, Lourdes de Souza Md, Felix AC, Mamede N, Sanabani SS. 2016. Investigation into an outbreak of Dengue-like illness in Pernambuco, Brazil, revealed a cocirculation of Zika, Chikungunya, and Dengue virus Type 1. Medicine (Baltimore). 95:e3201. doi:10.1097/MD.0000000000003201
- Petersen E, Wilson ME, Touch S, McCloskey B, Mwaba P, Bates M, Dar O, Mattes F, Kidd M, Ippolito G, et al. 2016. Rapid spread of Zika virus in the Americas - implications for public health preparedness for mass gatherings at the 2016 Brazil Olympic Games. Int J Infect Dis. 44:11–15.
- Petersen EE, Staples JE, Meaney-Delman D, Fischer M, Ellington SR, Callaghan WM, Jamieson DJ. 2016. Interim guidelines for pregnant women during a Zika virus outbreak – United States, 2016. MMWR Morb Mortal Wkly Rep. 65:30–33.
- Petersen LR, Jamieson DJ, Powers AM, Honein MA. 2016. Zika virus. N Engl J Med. 374:1552–1563.
- Petrovsky N, Aguilar JC. (2004) Vaccine adjuvants: current state and future trends. Immunol cell boil. 82:488–496.
- Picard-Jean F, Tremblay-Ltourneau M, Serra E, Dimech C, Schulz H, Anselin M, Dutilly V, Bisaillo M. 2013. RNA 5′-end maturation: a crucial step in the replication of viral genomes. In: Romanowski V, editor. Current issues in Molecule Virology - Viral Genetics and Biotechnological Applications. [Internet]. [place unknown]: InTech; [cited 2016 Feb 23]. Available from: http://www.intechopen.com/books/current-issues-in-molecular-virology-viral-genetics-and-biotechnological-applications/rna-5-end-maturation-a-crucial-step-in-the-replication-of-viral-genomes.
- Pierre DYS, Okechukwu EC, Nchiwan NE. 2014. Larvicidal and phytochemical properties of Callistemon rigidus R. Br. (Myrtaceae) leaf solvent extracts against three vector mosquitoes. J Vector Borne Dis. 51:216–223.
- Pinto VL Jr., Luz K, Parreira R, Ferrinho P. 2015. Zika virus: a review to clinicians. Acta Med Port. 28:760–765.
- Pond WL. 1963. Arthropod-borne virus antibodies in sera from residents of South-East Asia. Trans R Soc Trop Med Hyg. 57:364–371.
- Pyke AT, Daly MT, Cameron JN, Moore PR, Taylor CT, Hewitson GR, Humphreys JL, Gair R. 2014. Imported zika virus infection from the Cook Islands into Australia. PLoS Curr. 6. pii: ecurrents.outbreaks.4635a54dbffba2156fb2fd76dc49f65e.
- Rafiei N, Hajkowicz K, Redmond A, Taylor C. 2016. First report of Zika virus infection in a returned traveller from the Solomon Islands. Med J Aust 204 :186.
- Ramkumar G, Karthi, S. 2015. Adulticidal and smoke toxicity of Cipadessa baccifera (Roth) plant extracts against Anopheles stephensi, Aedes aegypti, and Culex quinquefasciatus. Parasitol Res. 114:167–173.
- Rasmussen SA, Kissin DM, Yeung LF, MacFarlane K, Chu SY, Turcios-Ruiz RM, Mitchell EW, Williams J, Fry AM, Hageman J, et al. 2011. Pandemic influenza and pregnancy working group. Preparing for influenza after 2009 H1N1: special considerations for pregnant women and newborns. Am J Obstet Gynecol. 204:S13–S20.
- Ratcliff RM, Chang G, Kok T, Sloots TP. 2007. Molecular diagnosis of medical viruses. Curr Iss Molr Biol. 9:87–102.
- Ray D, Shah A, Tilgner M, Guo Y, Zhao Y, Dong H, Deas TS, Zhou Y, Li H, Shi P-Y. 2006. West Nile virus 5’-cap structure is formed by sequential guanine N-7 and ribose 2’-O methylations by nonstructural protein 5. J Virol. 80:8362–8370.
- Reed SG, Orr MT, Fox CB 2013. Key roles of adjuvants in modern vaccines. Nature Med. 19:1597–1608.
- Reis-Castro L. 2012. Genetically modified insects as a public health tool: discussing the different bio-objectification within genetic strategies. Croat Med J. 53:635–638.
- Renaudet J, Jan C, Ridet J, Adam C, Robin Y. 1978. A serological survey of arboviruses in the human population of Senegal. Bull Soc Pathol Exot Filiales. 71:131–140.
- Rice CM, Lenches EM, Eddy SR, Shin SJ, Sheets RL, Strauss JH. 1985. Nucleotide sequence of yellow fever virus: implications for flavivirus gene expression and evolution. Science 229:726–733.
- Roa M. 2016. Zika virus outbreak: reproductive health and rights in Latin America. Lancet. 387:843.
- Robin Y, Mouchet J. 1975. Serological and entomological study on yellow fever in Sierra Leone. Bull Soc Pathol Exot Filiales. 68:249–258.
- Rodhain F, Gonzalez JP, Mercier E, Helynck B, Larouze B, Hannoun C. 1989. Arbovirus infections and viral haemorrhagic fevers in Uganda: a serological survey in Karamoja district. Trans R Soc Trop Med Hyg. 83:851–884.
- Rodriguez-Morales AJ. 2015. Zika: the new arbovirus threat for Latin America. J Infect Dev Ctries. 9:684–685.
- Rodriguez-Morales AJ. 2016. Zika and microcephaly in Latin America: An emerging threat for pregnant travelers? Travel Med Infect Dis. 14:5–6.
- Rossi SL, Tesh RB, Azar SR, Muruato AE, Hanley KA, Auguste AJ, Langsjoen RM, Paessler S, Vasilakis N, Weaver SC. 2016. Characterization of a novel murine model to study Zika virus. Am J Trop Med Hyg. doi:10.4269/ajtmh.16-0111
- Roth A, Mercier A, Lepers C, Hoy D, Duituturaga S, Benyon E, Guillaumot L, Souares Y. 2014. Concurrent outbreaks of dengue, chikungunya and Zika virus infections – an unprecedented epidemic wave of mosquito-borne viruses in the Pacific 2012-2014. Euro Surveill. 19: pii: 20929.
- Rozé B, Najioullah F, Fergé JL, Apetse K, Brouste Y, Cesaire R, Fagour C, Fagour L, Hochedez P, Jeannin S, et al. 2016. Zika virus detection in urine from patients with Guillain-Barré syndrome on Martinique. Euro Surveill. 21. doi:10.2807/1560-7917.ES.2016.21.9.30154
- Rubin EJ, Greene MF, Baden LR. 2016. Zika virus and microcephaly. N Engl J Med. 374:984–985.
- Ryan MD, Monaghan S, Flint M. 1998. Virus-encoded proteinases of the Flaviviridae. J Gen Virol. 79:947–959.
- Sabogal-Roman JA, Murillo-García DR, Yepes-Echeverri MC, Restrepo-Mejia JD, Granados-Álvarez S, Paniz-Mondolfi AE, Villamil-Gómez WE, Zapata-Cerpa DC, Barreto-Rodriguez K, Rodríguez-Morales AJ. 2015. Healthcare students and workers' knowledge about transmission, epidemiology and symptoms of Zika fever in four cities of Colombia. Travel Med Infect Dis. 14:52–54.
- Sadovsky Y, Clifton VL, Knöfler M. 2016. Editorial: ZIKA virus and placenta. Placenta. 40:A1. doi:10.1016/j.placenta.2016.03.008
- Saluzzo JF, Gonzalez JP, Hervé JP, Georges AJ. 1981. Serological survey for the prevalence of certain arboviruses in the human population of the south-east area of Central African Republic (author's transl). Bull Soc Pathol Exot Filiales. 74:490–499.
- Saluzzo JF, Ivanoff B, Languillat G, Georges AJ. 1982. Serological survey for arbovirus antibodies in the human and simian populations of the South-East of Gabon (author's transl). Bull Soc Pathol Exot Filiales. 75:262–266.
- Salvador FS, Fujita DM. 2015. Entry routes for Zika virus in Brazil after 2014 world cup: new possibilities. Travel Med Infect Dis. 14:49–51.
- Sarno M, Sacramento GA, Khouri R, do Rosário MS, Costa F, Archanjo G, Santos LA, Nery N Jr, Vasilakis N, Ko AI, de Almeida AR. 2016. Zika virus infection and stillbirths: a case of hydrops fetalis, hydranencephaly and fetal demise. PLOS Negl Trop Dis. 10: e0004517.
- Schuler-Faccini L, Ribeiro EM, Feitosa IM, Horovitz DD, Cavalcanti DP, Pessoa A, Doriqui MJ, Neri JI, Neto JM, Wanderley HY, et al. 2016. Possible association between Zika virus infection and microcephaly - Brazil, 2015. MMWR Morb Mortal Wkly Rep. 65:59–62.
- Shinohara K, Kutsuna S, Takasaki T, Moi ML, Ikeda M, Kotaki A, Yamamoto K, Fujiya Y, Mawatari M, Takeshita N, et al. 2016. Zika fever imported from Thailand to Japan, and diagnosed by PCR in the urines. J Travel Med. 23. pii: tav011.
- Shiryaev SA, Strongin AY. 2010. Structural and functional parameters of the flaviviral protease: a promising antiviral drug target. Future Virol. 5:593–606.
- Simpson DI. 1964. Zika virus infection in man. Trans R Soc Trop Med Hyg. 58:335–338.
- Singh RK, Badasara SK, Dhama K, Malik YPS. 2015. Progress and prospects in vaccine research. In: Malik YPS, Sagar P, Dhama K, Singh RK, editors. Current trends and future research challenges in vaccines and adjuvants. Souvenir, National Workshop Organized at Indian Veterinary Research Institute, Izatnagar 243122, Bareilly, Uttar Pradesh, India during 19–20 November 2015; pp. 1–19.
- Sirohi D, Chen Z, Sun L, Klose T, Pierson TC, Rossmann MG, Kuhn RJ. 2016. The 3.8 Å resolution cryo-EM structure of Zika virus. Science. 352:467–470.
- SMFM Publications Committee. 2016. SMFM statement: ultrasound screening for fetal microcephaly following Zika virus exposure. Am J Obstet Gynecol. doi:10.1016/j.ajog.2016.02.043
- Smit JM, Moesker B, Rodenhuis-Zybert I, Wilschut J. 2011. Flavivirus cell entry and membrane fusion. Viruses. 3:160–171.
- Smithburn KC. 1952. Neutralizing antibodies against certain recently isolated viruses in the sera of human beings residing in East Africa. J Immunol Baltim Md. 69:223–234.
- Smithburn KC. 1954. Neutralizing antibodies against arthropod-borne viruses in the sera of long-time residents of Malaya and Borneo. Am J Hyg. 59:157–163.
- Smithburn KC, Kerr JA, Gatne PB. 1954. Neutralizing antibodies against certain viruses in the sera of residents of India. J Immunol Baltim Md. 1950. 72:248–257.
- Smithburn KC, Taylor RM, Rizk F, Kader A. 1954. Immunity to certain arthropod-borne viruses among indigenous residents of Egypt. Am J Trop Med Hyg. 3:9–18.
- Staples JE, Dziuban EJ, Fischer M, Cragan JD, Rasmussen SA, Cannon MJ, Frey MT, Renquist CM, Lanciotti RS, Muñoz JL, et al. 2016. Interim guidelines for the evaluation and testing of infants with possible congenital Zika virus infection - United States. MMWR Morb Mortal Wkly Rep. 65:63–67.
- Summers DJ, Acosta RW, Acosta AM. 2015. Zika virus in an American recreational traveler. J Travel Med. 22:338–340.
- Tamura K, Stecher G, Peterson D, Filipski A, Kumar S. 2013. MEGA6: molecular evolutionary genetics analysis version 6.0. Mol Biol Evol. 12:2725–2729.
- Tang H, Hammock C, Ogden SC, Wen Z, Qian X, Li Y, Yao B, Shin J, Zhang F, Lee EM, et al. 2016. Zika virus infects human cortical neural progenitors and attenuates their growth. Cell Stem Cell. 18:587–590.
- Tappe D, Nachtigall S, Kapaun A, Schnitzler P, Günther S, Schmidt-Chanasit J. 2015. Acute Zika virus infection after travel to Malaysian Borneo, September 2014. Emerg Infect Dis. 21:911–913.
- Tappe D, Pérez-Girón JV, Zammarchi L, Rissland J, Ferreira DF, Jaenisch T, Gómez-Medina S, Günther S, Bartoloni A, Muñoz-Fontela C, Schmidt-Chanasit J. 2016. Cytokine kinetics of Zika virus-infected patients from acute to reconvalescent phase. Med Microbiol Immunol. 205:269–273.
- Tappe D, Rissland J, Gabriel M, Emmerich P, Gunther S, Held G, Smola S, Schmidt-Chanasit J. 2014. First case of laboratory-confirmed Zika virus infection imported into Europe, November 2013. Euro Surveill. 19:20685.
- Tetro JA. 2016. Zika and microcephaly: causation, correlation, or coincidence? Microbes Infect. pii: S1286-4579(16)00008-3.
- Teurlai M, Menkès CE, Cavarero V, Degallier N, Descloux E, Grangeon JP, Guillaumot L, Libourel T, Lucio PS, Mathieu-Daudé F, Mangeas M. 2015. Socio-economic and climate factors associated with Dengue fever spatial heterogeneity: a worked example in New Caledonia. PLoS Negl Trop Dis. 9:e0004211.
- Tiwari R, Chakraborty S, Dhama K, Wani MY, Kumar A, Kapoor S. 2014. Wonder world of phages: potential biocontrol agents safeguarding biosphere and health of animals and humans - current scenario and perspectives. Pak J Biol Sci. 17:316–328.
- Tognarelli J, Ulloa S, Villagra E, Lagos J, Aguayo C, Fasce R, Parra B, Mora J, Becerra N, Lagos N, et al. 2015. A report on the outbreak of Zika virus on Easter Island, South Pacific, 2014. Arch Virol. 161:665–668.
- Torjesen I. 2016. Zika virus outbreaks prompt warnings to pregnant women. BMJ. 352:i500.
- Ulmer JB, Mansoura MK, Geall AJ. 2015. Vaccines' on demand': science fiction or a future reality. Expert Opin Drug Discov. 10:101–116.
- Van den Hurk R, Evoy S. 2015. A review of membrane-based biosensors for pathogen detection. Sensors. 15:14045–14078.
- van Panhuis WG, Hyun S, Blaney K, Marques ET Jr, Coelho GE, Siqueira JB Jr, Tibshirani R, da Silva JB Jr, Rosenfeld R. 2014. Risk of dengue for tourists and teams during the World Cup 2014 in Brazil. PLoS Negl Trop Dis. 8:e3063.
- Ventura CV, Maia M, Bravo-Filho V, Góis AL, Belfort R Jr. 2016. Zika virus in Brazil and macular atrophy in a child with microcephaly. Lancet 387:228.
- Ventura CV, Maia M, Ventura BV, Linden VV, Araújo EB, Ramos RC, Rocha MA, Carvalho MD, Belfort R Jr, Ventura LO. 2016. Ophthalmological findings in infants with microcephaly and presumable intra-uterus Zika virus infection. Arq Bras Oftalmol. 79:1–3.
- Vest KG. 2016. Zika virus: a basic overview of an emerging arboviral infection in the Western Hemisphere. Disaster Med Public Health Prep. 29:1–6.
- Victora CG, Schuler-Faccini L, Matijasevich A, Ribeiro E, Pessoa A, Barros FC. 2016. Microcephaly in Brazil: how to interpret reported numbers? Lancet. 387:621–624.
- Villamil-Gómez WE, González-Camargo O, Rodriguez-Ayubi J, Zapata-Serpa D, Rodriguez-Morales AJ. 2016. Dengue, chikungunya and Zika co-infection in a patient from Colombia. J Infect Public Health. pii: S1876-0341(15)00221-X.
- Waggoner JJ, Pinsky BA. 2016. Zika virus: diagnostics for an emerging pandemic threat. J Clin Microbiol. 54:860–867.
- Way JH, Bowen ET, Platt GS. 1976. Comparative studies of some African arboviruses in cell culture and in mice. J Gen Virol. 30:123–130.
- Weaver SC, Costa F, Garcia-Blanco MA, Ko AI, Ribeiro GS, Saade G, Shi PY, Vasilakis N. 2016. Zika virus: history, emergence, biology, and prospects for control. Antiviral Res. 130:69–80.
- Weinbren MP, Williams MC. 1958. Zika virus: further isolations in the Zika area, and some studies on the strains isolated. Trans R Soc Trop Med Hyg. 52:263–268.
- Wikan N, Suputtamongkol Y, Yoksan S, Smith DR, Auewarakul P. 2016. Immunological evidence of Zika virus transmission in Thailand. Asian Pac J Trop Med. 9:141–144.
- Wolfe ND, Kilbourn AM, Karesh WB, Rahman HA, Bosi EJ, Cropp BC, Andau M, Spielman A, Gubler DJ. 2001. Sylvatic transmission of arboviruses among Bornean orangutans. Am J Trop Med Hyg. 64:310–316.
- WHO. 2016. Disease outbreak news: Zika virus infection - Dominican Republic. 27 January 2016 [Internet]. Geneve: WHO; 2016.
- Xiao XW, Fu HZ, Luo YH, Wei XY. 2013. Potential anti-angiogenic sulfates of andrographolide. J Asian Nat Prod Res. 15:809–818.
- Zammarchi L, Stella G, Mantella A, Bartolozzi D, Tappe D, Günther S, Oestereich L, Cadar D, Muñoz-Fontela C, Bartoloni A, Schmidt-Chanasit J. 2015. Zika virus infections imported to Italy: clinical, immunological and virological findings, and public health implications. J Clin Virol. 63:32–35.
- Zammarchi L, Tappe D, Fortuna C, Remoli ME, Günther S, Venturi G, Bartoloni A, Schmidt-Chanasit J. 2015. Zika virus infection in a traveller returning to Europe from Brazil, March 2015. Euro Surveill. 20. pii: 21153.
- Zanluca C, de Melo VC, Mosimann AL, Dos Santos GI, Dos Santos CN, Luz K. 2015. First report of autochthonous transmission of Zika virus in Brazil. Mem Inst Oswaldo Cruz. 110:569–572.
- Zanluca C, dos Santos CND. 2016. Zika viruse an overview. Microb Infect. 18:295–301.
- Zhang Y, Corver J, Chipman PR, Zhang W, Pletnev SV, Sedlak D, Baker TS, Strauss JH, Kuhn RJ, Rossmann MG. 2003. Structures of immature flavivirus particles. EMBO J. 22:2604–2613.
- Zhou Y, Hao W, Zhao Q, Chen Y, Chen Y, Tao Y, Bai H. 2014. Recent advances in transgenic plant-derived vaccines. Med Plant 5:57–60.