ABSTRACT
Background: Ruminants, including sheep and goats (small ruminants), are key agricultural animals in many parts of the world. Infectious diseases, including many viral diseases, are significant problems to efficient production of ruminants. Unfortunately, reagents tailored to viruses of ruminants, and especially small ruminants, are lacking compared to other animals more typically used for biomedical research.
Objective: The purpose of this study was to determine the permissibility of a stably immortalized, sheep microglial cell line to viruses that are reported to infect ruminants: bovine viral diarrhea virus (BVDV), bovine herpesvirus 1 (BoHV-1), small ruminant lentiviruses (SRLV), and bovine respiratory syncytial virus (BRSV).
Methods: Sublines A and H of previously isolated, immortalized, and characterized (CD14-positive) ovine microglial cells were used. Bovine turbinate cells and goat synovial membrane cells were used for comparison. Cytopathic changes were used to confirm infection of individual wells, which were then counted and used to calculate the 50% tissue culture infectious dose. Uninoculated cells served as negative controls and confirmed that the cells were not previously infected with these viruses using polymerase chain reaction (PCR).
Results: Inoculation of the two microglial cell sublines with laboratory and field isolates of BVDV, BoHV-1, and BRSV resulted in viral infection in a manner similar to bovine turbinate cells. Immortalized microglia cells are also permissive to SRLV, similar to goat synovial membrane cells.
Conclusion and clinical relevance: These immortalized sheep microglial cells provide a new tool for the study of ruminant viruses in ruminant microglial cell line.
1. Introduction
Ruminants, including small ruminants (sheep and goats), are economically important animals, with small ruminants being especially important in the world's impoverished areas (FAO Citation2013). Viral diseases remain a persistent problem for management and production of ruminants throughout the world. Diagnostic and investigative reagents for ruminants, including small ruminants, however, are lacking as contrasted with those for animals more typically used in biomedical research. Since small ruminants share viruses, such as bovine viral diarrhea virus (BVDV), with cattle (Sullivan et al. Citation1997), there is a need to study viruses in small ruminant-based systems.
One of the basic systems for viral diagnostics and research investigations is a permissive cell culture system. Primary and immortalized cell culture systems have been described for small ruminants, but remain poorly utilized. For example, a lung cell line that is permissive to bovine parainfluenza 3 (BPIV-3), bovine respiratory syncytial virus (BRSV), BVDV, and bovine herpesvirus 1 (BoHV-1) has been derived from bighorn sheep (Shanthalingam et al. Citation2010). However, the specific cell of origin of this cell line has not been fully reported. A cell line derived from a lamb testis was permissive to cytopathic BVDV (Gong et al. Citation1998). However, the permissibility of this cell line for other viruses has not been tested further. For the study of small ruminant lentiviruses (SRLV), the availability of permissive cell lines is similarly limited. An immortalized ovine synovial membrane cell line (Costa et al. Citation2005) and a caprine oviductal epithelial cell line (Lamara et al. Citation2002) have demonstrated permissibility to SRLV, but the range of permissibility of these cell lines to other viruses has not been tested. Collectively, for ruminant cells, the range of permissibility and the relevance of the cell type to infection is limited, e.g. bovine turbinate (BT)-derived fibroblasts (Gong et al. Citation1998).
Dendritic cells, circulating monocytes, and tissue-resident macrophages are relevant cells to many viral infections of economical importance as they are often involved in viral pathogenesis and protection. For instance, the ability to target these mononuclear cells is one factor that contributes to the ability of alpha-herpesviruses and lentiviruses to invade and spread into the subepithelial tissues (Maclachlan et al. Citation2011). Conversely, mononuclear cells are critical for the phagocytosis and inactivation of virions and the presentation of viral antigens to initiate an adaptive immune response (Maclachlan et al. Citation2011). Thus, not only are mononuclear/macrophage cells permissive for many viruses in vivo, but the virus--monocyte interaction is often critical to the pathogenesis making in vitro modeling of this interaction scientifically beneficial. Microglia are resident macrophages of the brain and provide a source of replication-competent cells for use in cell culture.
Previously, we determined that primary sheep microglial cells were permissive to SRLV infection (Stanton et al. Citation2008) and to the BVDV infection (Stanton et al. Citation2012). The aims of this study were to determine if permissiveness to these two viruses (i.e. SRLV and BVDV) was retained after immortalization of the cells (Muñoz-Gutiérrez et al. Citation2015), and if these immortalized cells are also permissive for BoHV-1 and BRSV.
2. Methods
Sublines A and H of previously isolated, immortalized, and characterized (CD14-positive) microglial cells (Muñoz-Gutiérrez et al. Citation2015) were used. Furthermore, two clones derived from subline H have transcriptomes consistent with microglia (Muñoz-Gutiérrez et al. Citation2016). BT cells and goat synovial membrane (GSM) cells were used for comparison to the sheep microglial. All cells were cultured in Opti-MEM® (Invitrogen) supplemented with 10% heat-inactivated fetal bovine serum, 2 mM L-glutamine, 10 IU penicillin, and 10 mg/mL streptomycin.
For titration of BVDV2-296c (Ridpath & Neill Citation2000), BoHV-1 (Washington Animal Disease Diagnostic Laboratory, Washington State University, Pullman, WA, USA) and BRSV (Washington Animal Disease Diagnostic Laboratory, Washington State University, Pullman, WA, USA) viral stocks were amplified on the sheep microglial cell subline H and in the BT cells. Microglial cells and BT cells were seeded into 96-well plates. When 80% confluent, the cells were inoculated with serial dilutions of the viral stocks and then incubated at 37 °C in 5% CO2. After seven days of incubation, the cells were examined under phase contrast microscopy for characteristic cytopathic effects. Cytopathic changes were used to confirm infection of individual wells, which were then counted and used to calculate the 50% tissue culture infectious dose (TCID50) (Reed & Muench Citation1938). Uninoculated cells served as negative controls and confirmed that the cells were not previously infected with these viruses (e.g. BVDV contamination of fetal bovine serum) using PCR as previously described (Stanton et al. Citation2012). For SRLV, sheep microglial cells and GSM cells were inoculated with the Cork strain of SRLV when 80% confluent. Cells were cultured as earlier for five days. Cells were then fixed in Z-fix (Anateck Ltd, United States) for 2 minutes and then either stained with modified Wright Giemsa stain following manufacturer's directions and evaluated for syncytial cells or immunostained for SRLV capsid protein. For immunostaining, the CAEP10A1 monoclonal antibody (VMRD, Inc.) was used at 1:500 to detect the viral capsid antigen and the immunostaining procedure was completed using the Ultra Streptavidin Horseradish Peroxidase kit (Covance, United States) with visualization of the immune complex deposits via 3-amino-9-ethylcarbazole (AEC; Dako, United States). Replicate cell cultures were probed with an irrelevant antibody as a negative control.
All statistical analyses were conducted using one-way ANOVA by means of JMP® Pro 11.2.0. A significance level (α) of 5% was considered significant for all tests.
3. Results
BRSV, BoHV-1, and BVDV derived from BT cell cultures resulted in typical cytopathic effects (data not shown) in BT cells (used as control target cells) and in both sublines of immortalized sheep microglial cells. To confirm full permissibility of cultured sheep microglia and to determine if the use of microglial cells had varying susceptibility as compared to BT cells, culture supernatant was collected from infected microglia subline H and from BT cells, and the relative titers were compared across differing target cell lines. For BT-propagated virus, the use of microglial cells as the target cell had no detectable impact (one-way ANOVA on log-transformed data, BoHV-1 P = .11, BRSV P = .33, BVDV P = .22) on the detection of infectivity for any of the three viruses ((A)). The results for microglial-derived virus were similar ((B), BoHV-1 P = .22, BRSV P = .12), with the exception that a significant difference in susceptibility was detected in the propagation of BVDV in BT cells as compared to in microglial subline A (one-way ANOVA, Tukey multiple comparison on log-transformed data, P = .02). While statistically significant, the magnitude of difference was minimal, and these results likely have little biological relevance. These results demonstrate that both microglial cell lines are permissive for BRSV, BoHV-1, and BVDV2-296c, and that the permissibility of the microglial cell lines is similar to that of the BT cells.
Figure 1. Virus titration as measured on bovine turbinate (BT) cells and two sublines of immortalized sheep microglia. Both microglial sublines are permissive to BoHV-1, BRSV, and BVDV. (A) BT cells and two sublines of sheep microglia were inoculated with BoHV-1, BRSV, and BVDV that were propagated in bovine turbinate cells. No significant differences in permissibility were detected, as measured by the TCID50 calculated on the differing cell types (one-way ANOVA, α = .05). (B) The same cells were inoculated with the same viruses as in (A), which were first propagated in microglial subline H. Only one statistical difference (BVDV: BT cells vs. Microglia A) in relative TCID50 was detected (*one-way ANOVA, Tukey post hoc, P = .02, α = .05). However, the magnitude of difference was small with the microglial cell line being slightly more permissive to BVDV.
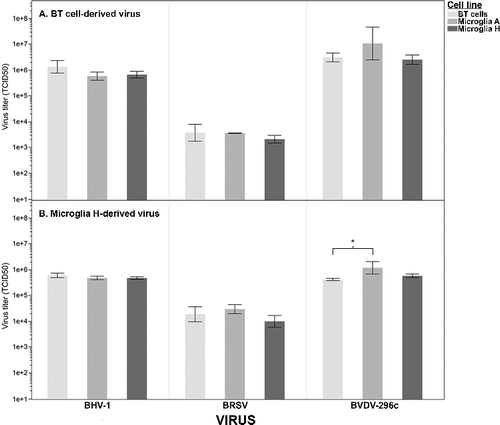
Five days following inoculation with SRLV, positive immunoreactivity for the SRLV capsid protein (subline A shown in (A)) was detected in both sublines. Additionally, syncytial cells were detected in both sublines using routine cell staining (subline B shown in (B)). These results demonstrate that both immortalized microglial cell lines are permissive to SRLV infection.
Figure 2. Photomicrographs of immortalized sheep microglia displaying evidence of infection after inoculation with SRLV-Cork. Immortalized microglial retains permissiveness to SRLV. (A) Five days after inoculation with SRLV cells demonstrated strong immunoreactivity (red-brown, intracytoplasmic staining) for SRLV nucleocapsid protein (subline A shown). 100×. (B) Five days after inoculation with SRLV and modified Wright Giemsa staining, microglial demonstrated scattered syncytial cells with numerous (>10) nuclei, a typical cytopathic effect following SRLV infection (subline H shown). 200×.
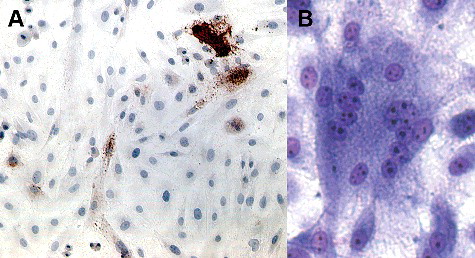
4. Discussion
The objectives of this study were to demonstrate that the immortalization of sheep-derived microglia cells resulted in retained permissibility to BVDV and SRLV, and to demonstrate that the immortalized microglial cells were also permissive to BRSV and BoHV-1. The hypothesis was based on previous results demonstrating in vivo infection of myeloid-derived monocytic cells to respiratory syncytial virus (Ponnuraj et al. Citation2001), to lentiviruses (Kure et al. Citation1991), and to pestiviruses (Ohmann Citation1982). The results demonstrate that these microglial cells are permissive to all tested viruses used, including SRLV, which is not infectious to a more typically used ruminant cell type (i.e. BT cells). Furthermore, the expression of a fusogenic SRLV envelope protein in the sheep microglial cultures is supportive of their microglial origin, as fusogenic envelope protein is not expressed in fibroblasts infected with SRLV-Cork (Chebloune et al. Citation1996; Narayan et al. Citation1980).
Small ruminants are an important commodity in many parts of the world, especially the Middle East and Africa. Research on many known viral diseases of small ruminants continues due to their economic importance to small ruminant producers worldwide and to the potential role of small ruminants as reservoirs of viruses that can be transmitted to cattle. For example, bluetongue virus (BTV) is estimated to cost sheep, goat, and cattle producers approximately $3 billion annually (Tabachnick et al. Citation1996) and the recent incursion of BTV into Europe (Maclachlan Citation2010) highlights the need to understand the diseases to create rational testing and control strategies. Such strategies will need to include small ruminants as well as cattle. Similarly, newly discovered viruses such as Schmallenberg virus (Hoffmann et al. Citation2012), make active surveillance for ruminant viruses vital. Ruminant-derived cell cultures will enhance the ability to diagnose and investigate these important viruses. Future testing to determine the permissibility of these immortalized sheep microglial cells to viruses such as Schmallenberg virus is suggested.
Not only are these cell lines tools for ruminant viruses specifically, but they also likely represent an important cell type that is pathophysiologically relevant to many viral infections of wild and domestic ruminants. Cell culture systems for sheep are limited as compared to more typical animal models (e.g. mice, cattle, and dogs). This cell line should enhance diagnostic and research capabilities for viruses of sheep, goats, and other ruminants, and is freely available to anyone on request.
5. Conclusions
Our results demonstrate that these immortalized sheep microglia are permissive to BRSV, BVDV, BoHV-1, and SRLV. These cells are derived from a ruminant species and a cell type that is relevant to many viral infections. The cells are available to researchers studying these viruses and other ruminant viruses.
Acknowledgments
We thank John VanderSchalie and Lorraine Tanaka for their excellent technical support. Mention of trade names or commercial products or enterprises in this article is solely for the purpose of providing specific information and does not imply recommendation or endorsement by the US Department of Agriculture.
Disclosure statement
The authors report no conflicts of interest.
Additional information
Funding
References
- Chebloune Y, Sheffer D, Karr BM, Stephens E, Narayan O. 1996. Restrictive type of replication of ovine/caprine lentiviruses in ovine fibroblast cell cultures. Virology. 222:21–30.
- Costa UM, Reischak D, da Silva J, Ravazzolo AP. 2005. Establishment and partial characterization of an ovine synovial membrane cell line obtained by transformation with Simian Virus 40 T antigen. J Virol Methods. 128:72–78.
- Food and Agriculture Organization of the United Nations (FAO). 2013. Supporting livelihoods and building resilience through Peste des Petits Ruminants (PPR) and small ruminant diseases control. In: Animal Production and Health Position Paper. Rome.
- Gong Y, Trowbridge R, Mackintosh S, Shannon A, Gowans EJ. 1998. A stable cell line with a proportion of cells persistently infected with bovine viral diarrhoea virus. Vet Microbiol. 63:117–124.
- Hoffmann B, Scheuch M, Hoper D, Jungblut R, Holsteg M, Schirrmeier H, Eschbaumer M, Goller KV, Wernike K, Fischer M et al., 2012. Novel orthobunyavirus in Cattle, Europe, 2011. Emerg Infect Dis. 18:469–472.
- Kure K, Llena JF, Lyman WD, Soeiro R, Weidenheim KM, Hirano A, Dickson DW. 1991. Human immunodeficiency virus-1 infection of the nervous system: an autopsy study of 268 adult, pediatric, and fetal brains. Hum Pathol. 22:700–710.
- Lamara A, Fieni F, Mselli-Lakhal L, Tainturier D, Chebloune Y. 2002. Epithelial cells from goat oviduct are highly permissive for productive infection with caprine arthritis-encephalitis virus (CAEV). Virus Res. 87:69–77.
- Maclachlan NJ. 2010. Global implications of the recent emergence of bluetongue virus in Europe. Vet Clin North Am Food Anim Pract. 26:163–171.
- Maclachlan NJ, Dubovi EJ, Fenner F. 2011. Pathogenesis of viral infections and diseases: mechanisms of viral spread and infection of target organs. In: Fenner's veterinary virology. 4th ed. London: Elsevier/Academic Press; p. 46–54.
- Muñoz-Gutiérrez JF, Aguilar Pierlé S, Schneider DA, Baszler TV, Stanton JB. 2016. Transcriptomic determinants of scrapie prion propagation in cultured ovine microglia. PloS One. 11:e0147727.
- Muñoz-Gutiérrez JF, Schneider DA, Baszler TV, Greenlee JJ, Nicholson EM, Stanton JB. 2015. hTERT-immortalized ovine microglia propagate natural scrapie isolates. Virus Res. 198:35–43.
- Narayan O, Clements JE, Strandberg JD, Cork LC, Griffin DE. 1980. Biological characterization of the virus causing leukoencephalitis and arthritis in goats. J Gen Virol. 50:69–79.
- Ohmann HB. 1982. Experimental fetal infection with bovine viral diarrhea virus. II. Morphological reactions and distribution of viral antigen. Can J Comp Med. 46:363–369.
- Ponnuraj EM, Hayward AR, Raj A, Wilson H, Simoes EA. 2001. Increased replication of respiratory syncytial virus (RSV) in pulmonary infiltrates is associated with enhanced histopathological disease in bonnet monkeys (Macaca radiata) pre-immunized with a formalin-inactivated RSV vaccine. J Gen Virol. 82:2663–2674.
- Reed LJ, Muench H. 1938. A simple method of estimating fifty per cent endpoints. Am J Epidemiol. 27:493–497.
- Ridpath JF, Neill JD. 2000. Detection and characterization of genetic recombination in cytopathic type 2 bovine viral diarrhea viruses. J Virol. 74:8771–8774.
- Shanthalingam S, Topliff C, Kelling CL, Srikumaran S. 2010. Bighorn sheep fetal lung cell line for detection of respiratory viruses. Can J Vet Res. 74:75–77.
- Stanton JB, Knowles DP, O'Rourke KI, Herrmann-Hoesing LM, Mathison BA, Baszler TV. 2008. Small-ruminant lentivirus enhances PrPSc accumulation in cultured sheep microglial cells. J Virol. 82:9839–9847.
- Stanton JB, Schneider DA, Dinkel KD, Balmer BF, Baszler TV, Mathison BA, Boykin DW, Kumar A. 2012. Discovery of a novel, monocationic, small-molecule inhibitor of scrapie prion accumulation in cultured sheep microglia and rov cells. PLoS One. 7:e51173.
- Sullivan DG, Chang GJ, Akkina RK. 1997. Genetic characterization of ruminant pestiviruses: sequence analysis of viral genotypes isolated from sheep. Virus Res. 47:19–29.
- Tabachnick WJ, Robertson MA, Murphy KE. 1996. Culicoides variipennis and bluetongue disease. Research on arthropod-borne animal diseases for control and prevention in the year 2000. Ann N Y Acad Sci. 791:219–226.