ABSTRACT
Rabies is a zoonotic, fatal and progressive neurological infection caused by rabies virus of the genus Lyssavirus and family Rhabdoviridae. It affects all warm-blooded animals and the disease is prevalent throughout the world and endemic in many countries except in Islands like Australia and Antarctica. Over 60,000 peoples die every year due to rabies, while approximately 15 million people receive rabies post-exposure prophylaxis (PEP) annually. Bite of rabid animals and saliva of infected host are mainly responsible for transmission and wildlife like raccoons, skunks, bats and foxes are main reservoirs for rabies. The incubation period is highly variable from 2 weeks to 6 years (avg. 2–3 months). Though severe neurologic signs and fatal outcome, neuropathological lesions are relatively mild. Rabies virus exploits various mechanisms to evade the host immune responses. Being a major zoonosis, precise and rapid diagnosis is important for early treatment and effective prevention and control measures. Traditional rapid Seller's staining and histopathological methods are still in use for diagnosis of rabies. Direct immunofluoroscent test (dFAT) is gold standard test and most commonly recommended for diagnosis of rabies in fresh brain tissues of dogs by both OIE and WHO. Mouse inoculation test (MIT) and polymerase chain reaction (PCR) are superior and used for routine diagnosis. Vaccination with live attenuated or inactivated viruses, DNA and recombinant vaccines can be done in endemic areas. This review describes in detail about epidemiology, transmission, pathogenesis, advances in diagnosis, vaccination and therapeutic approaches along with appropriate prevention and control strategies.
1. Introduction
Rabies otherwise ‘rabere’ in Latin means ‘to be mad.’ The disease is known since the advent of civilization. The first official documentation of rabies appeared in the pre-mosaic Eshmuna code of Babylon in the twenty-third century BC. However, it was Louis Pasteur in 1880's who identified a virus as the cause of the disease. Though rabies is a preventable viral zoonosis by vaccines still it remains an important public health issue in the developing countries which is evident from the fact that globally this devasting disease is responsible for more than 60,000 human deaths, while approximately 15 million people receive rabies post-exposure prophylaxis (PEP) annually (Dietzschold et al. Citation2003; Kuzmin et al. Citation2005; Leung et al. Citation2007; Wilde et al. Citation2013). Despite of global vast attempt and implementation of extensive control schemes and public health awareness programmes, still over 95% of the mortality happens in Asia and Africa, where canine rabies is enzootic (WHO Citation2013). In India, about 20,000 human deaths occur each year by the bite of rabid dog (Sudarshan et al. Citation2006a). Rabies in human always occurs as fatal disease inspite of advanced therapeutic measures (Jackson Citation2007a). Based on severity of mortality in humans, rabies stays in seventh position among the infectious diseases present in the globe (Wyatt Citation2007).
Rabies in mammals is fatal due to involvement of nervous system (NS) and is caused by a neurotropic, negative sense, non-segmented, single-stranded RNA virus that belongs to the Lyssavirus genus of the Rhabdoviridae family and Mononegavirale order (Madhusudana et al. Citation2012). The interesting feature of the lyssavirus is presence of seven distinct genotypes. The rabies virus (RABV) genome is approx. 12 kb size, which carries five structural proteins namely, nucleoprotein (N), phosphoprotein (P), matrix protein (M), glycoprotein (G) and RNA-dependent RNA polymerase (L) (Albertini et al. Citation2011). The RABV genome composed of N, P and L proteins, which forms ribonucleoprotein complex that helps in multiplication of virus in the cytoplasm of host cells. The G protein of the RABV is alone expressed on the viral surface, which is responsible for the viral pathogenicity, and induces protective immunity against rabies (Albertini et al. Citation2011; Zhu and Guo Citation2016). However, still the chance of increase in the number of these viruses is possible with more widespread and intensive sampling. It specifically causes acute encephalomyelitis affecting primarily carnivores and bats but has got the capability to affect all warm-blooded animals including humans as well as a wide variety of wildlife species that act as reservoirs for infection predominantly and it influences the population dynamics accordingly (Rupprecht and Gibbons Citation2004). Significance of rabies lies in the facts that it is mostly fatal with no specific antiviral treatment and is distributed gobally (Beran Citation1993; Green Citation1997; Radostits et al. Citation2000; Bender and Schulman Citation2004; Arai Citation2005; Fooks Citation2007; Wilkins and Piero Citation2007; Gruzdev Citation2008; Feder et al. Citation2012; Hatz et al. Citation2012; Hemachudha et al. Citation2013). Except Antarctica and Australia, distribution of the disease encompasses all continents (Rupprecht et al. Citation2008). In Asia and Africa, the disease raises a burning public health issues. In the Asian subcontinent, it is predominantly high in Bangladesh and India followed by Nepal, Myanmar, Bhutan, Thailand and Indonesia, wherein it is prevalent moderately. Its prevalence has been documented from 20% to 50% in different species of domestic animals. The susceptibility of animals varies greatly depending upon the animal species, genetic makeup, animal's age, strain, biotype or dose of the virus and exposure route. Worldwide rabies is endemic, which is a major concern but in countries like USA control programmes have facilitated the process of reducing the number of cases (Steele and Fernandez Citation1991; WHO Citation2005; Sudarshan et al. Citation2006b; Vanrompay et al. Citation2007). In many developing countries, mortality in humans due to rabies infection are low because of under-reporting, cultural beliefs, poor or inadequate rabies diagnostic units and poor knowledge on the mode of transmission and prevention of the disease (Otolorin et al. Citation2015). Under-reporting of rabies in endemic developing countries has resulted in the disease being ignored by medical professionals and subsequently poor assistance from international community and donor agencies (Otolorin et al. Citation2015). A serious concern about lyssavirus is the occurrence of multiple genotypes in several areas of the world and most of the genotypes cause disease in humans. It is problematic to diagnose the disease rapidly in human due to the presence of virus at low levels in samples that are accessible viz., saliva and cerebrospinal fluid (CSF) (Fooks et al. Citation2003; Paweska et al. Citation2006).
Non-bite transmission methods are inhaling RABV particles, organ and cornea transplants, and infection of open wounds, abrasions and mucous membranes with saliva and brain tissue from a rabid animal containing RABV (Takayama Citation2005). In most of the European countries, bats are protected legally under certain international treaties and national nature-conservation legislations. Bat rabies research is however important for gaining insight into whether rabies in bats can be a real problem for public health or not. It is equally essential to know the rabies incidence in various species of bats. Passive surveillance of rabies in bats thus seems to be a sufficient mean to obtain information about the occurrence of rabies in bats, which is not in conflict with conservation of bats. Knowledge about the bat rabies occurrence, prevalence of rabies in particular bat species and possible risk for public as well as animal health is also crucial to improve awareness among public for conservation of bats in conjunction with health of public. Thus, there should be good cooperation between bat conservationists and bodies involved in bat research (Mc Coll et al. Citation2000; Stantic-Paylinic Citation2005; Lina and Hutson Citation2006).
The detection of Negri bodies by Sellar's staining is a traditional method for diagnosis of rabies. The direct fluorescent antibody technique (dFAT) is a gold standard test for diagnosis of rabies and approved by WHO, because of short duration, low cost and high sensitivity. In addition to dFAT, mouse inoculation test is also carried out especially in developing countries, which is also a highly sensitive method (Chhabra et al. Citation2005; Manjunathareddy et al. Citation2016). Detection of RABV nucleic acid in the clinical samples such as CSF, saliva, skin biopsy and corneal impression smear by polymerase chain reaction (PCR) seems to be reliable diagnostic tool for the antemortem diagnosis of rabies (Madhusudana and Sukumaran Citation2008). Auspiciously, rabies is preventable by vaccination, if PEP is administered on time and accurately (Zhu and Guo Citation2016). The therapeutic approach for rabies in animals and humans is a main challenging issue in medicine, and the development of effective therapy may depend on a better understanding of basic mechanisms underlying the pathogenesis of rabies (Jackson Citation2016). The present review describes the etiology of rabies, prevalence and epidemiology, species affected and reservoirs, pathology, immuno pathology and pathogenesis, and advances in diagnosis, vaccination, therapy, management, prevention and control of this important viral pathogen having high public health concerns ().
Table 1. Highlights of this rabies virus review.
2. History
Rabies is the ancient disease and great dreaded infection of humans and animals (). It has existed for thousands of years as shown by Blancou (Citation2003). Rabies was first recognized in Egypt around 2300 BC and in ancient Greece, where it was well described by Aristotle. Canine rabies was also described in the sixth century BC, in the Avesta (Persia), first century BC in the Susrutasamhita (India). The infectious nature of saliva from infected dogs was recognised by Zinke in 1804. No effective preventive or curative treatment in animals was available before Pasteur's discovery in 1885. Pasteur in 1881 demonstrated the neurotropism of the virus. In 1885, Pasteur discovered and administered a rabies vaccine, prior to the structure and properties of the RABV were understood. In the same year, first time he administered the rabies vaccine to Joseph Meister, who was attacked worsely by rabies-affected animal. That day was the milestone for the beginning of the modern science in the aera of infectious diseases targeting control and prevention of diseases. In 1903, Remlinger and Riffat-Bay identified the RABV. During 1940s, RABV emerged in red foxes (Vulpes vulpes) in the Kaliningrad area and then spread to Central and Western Europe within a few decades (Hanlon and Childs Citation2013). The first rabies oral vaccination campaign for wildlife was conducted during 1978 in Switzerland, and then subsequently other European countries. A field trial of three oral vaccination campaigns and compulsory vaccination to dogs in the outbreak area was initiated using SAD B19 bait in 1988 and Finland was declared as rabies-free country again in 1991 (Nyberg et al. Citation1992).
Table 2. Journey of rabies virus.
3. Epidemiology
Among the viral diseases, rabies is unique and it can affect a wide range of victims including all warm-blooded animals. Rabies is prevalent throughout the world except in Islands. Many of the countries are endemic for rabies, except Australia and Antarctica. The countries free from rabies in Asian subcontinent are Bahrain, Cyprus, Hong Kong, Japan, Malaysia, Maldives, Qatar, Singapore, Lakshdweep, Andaman and Nicobar islands of India and Timor-Leste. Countries such as Antigua and Barmuda, Bahamas, Barbados, Belize, Falkland, Jamaica, Saintkitts and Nevis, Trinidad and Tobago, Uruguay of America subcontinent and Albania, E.Y.R. of Macedona, Finland, Gibraltar, Greece, Iceland, Isle of Man, Malta, Portugal, Norway (except Svalbard), United Kingdom and Spain (except Melill + ceuta) have also got rabies-free status. Among the African countries Cape Verde, Congo, Libya Mauritius, Reunion and Seychelles are free from rabies. Oceana group of Islands like Fiji, Cook Islands, Vanuatu, Guam, French Polynesia, New Zealand, New Caledonia, Solomon Islands and Papua New Guinea have also got rabies-free status (Yousaf et al. Citation2012). As per the definition of World Health Organization (WHO), a country that has no record of indigenously acquired case of human or animal rabies within two years period due to surveillance and import regulations can claim rabies-free status. But susceptibility to reintroduction from neighbouring countries does exist in spite of undertaking vaccination programmes in wildlife (Dutta and Dutta Citation1994; Rose Citation1999). Travellers visiting developing nations having sympathy for pet animals find it difficult to avoid feral dogs and cats thereby violate the precautionary measures (Meslin Citation2005; Mudur Citation2005). Any country maintaining the rabies-free status requires strict continuous monitoring, quarantine of imported animals and regulations to avoid the entrance of virus particularly with the import or introduction of infected animals (Castrodale et al. Citation2008). There is need of an up-to-date official list for a country to be rabies-free. Mere presence of rabies-related virus cannot prevent a country from achieving rabies-free status and same is the case with United Kingdom and Australia (McKay and Wallis Citation2005). In the UK, rabies was officially eradicated in 1920 (Pounder Citation2003). But in 2002, one death occurred in an unvaccinated bat conservationist in Dundee who contracted an RABV (European bat lyssavirus type 2) and did not receive PEP (Fooks et al. Citation2003).
If we consider status of rabies in Asia it is clear that majority of the developing nations of this subcontinent are the fatal sufferer of rabies (Hampson et al. Citation2015). As per the WHO global vaccines research forum, over 3 billion people are affected with dog rabies and more than 30,000 deaths occur annually in Asian continent means every 15 min, mortality of one Asian. But the painful fact is that among the rabies induced mortality in human, 15% of mortality occurred in children under 15 years of age (Yousaf et al. Citation2012). Officially reported human rabies cases really do not tally with actual incidence of rabies cases in most instances. This usually happens in the most of developing countries, especially in Africa (Tenzin and Ward Citation2012). While in WHO South East Asian Region (SEAR) member countries, it is more serious public health problem accounting for 99% (approx.) human mortality worldwide (WHO Citation2002). Rabies is predominant in Bangladesh and India followed by Nepal, Myanmar, Bhutan, Thailand and Indonesia. Nepal is one of the nations in the world, where the number of human rabies deaths is maximal (Yousaf et al. Citation2012). In Africa, maximum mortality rates are documented in children and underprivileged agrarian people. The most important reason for transmission of rabies in Africa is dog population and urbanization. In Europian continent, though rabies is still exist, but rabies cases in human have been vanished from most of the European nations most likely due to the enforcement of policies regarding vaccination in animals especially in dogs.
Dog slaughterhouses are considered as vital risk factor in the epidemiology of rabies in some of the Asian and African countries. RABV recovered from Burkino-Faso (De Benedictis et al. Citation2010) and Vietnam (Nguyen et al. Citation2011) having homology with isolates recovered from Mauritania and China, respectively, indicated that trans-boundary spread of RABV, which gives alarming information that the importance of trading and slaughtering of dogs in the rabies epidemiology. There is evidently a link exists between rabies dynamics and environmental hygiene in most abandoned rural communities particularly in densely populated areas, which enhances the risk of transmission of rabies in dogs and human, and vice versa (Atuman et al. Citation2014).
India is one of the countries with the largest rabies burden (Tenzin and Ward Citation2012; Hampson et al. Citation2015). The Association of the Prevention and Control of Rabies in India (APCRI) conducted a nationwide analysis, which reveals that 18,500 mortalities in human have occurred due to rabies annually. Around 40,000–70,000 human deaths are still reported worldwide (WHO Citation2005; Hampson et al. Citation2011). Andaman and Nicobar and Lakshdweep islands are however free of the disease, while in other parts of the country it is very much prevalent (Sehgal and Bhatia Citation1985). Prevalence of rabies in different species have been documented as 48% in dogs, 21.9% in cats, 61.4% in cattle and buffalo, 48.7% in goats and 45% in horses (WHO Citation1998). In the country numerous outbreaks of rabies have well been documented in domestic animals, wherein dog was suspected as the source of infection (Singh et al. Citation1995; Jindal and Narang Citation1998). The animal reservoirs of rabies vary from geographical region to region (Rupprecht et al. Citation2002; Yang et al. Citation2013).
The RABV circulating in dogs are responsible for more than 99% of the cases in humans worldwide. Inspite of its role as a vector of disease in humans, the extent as well as structure of viral biodiversity in this key vector species, mode and time scale of its evolution have been studied only in limited geographical scale (Knobel et al. Citation2005). It is thought that the development of trans-oceanic travel during the fifteenth century is responsible for rabies transmission to all the continents. This has resulted in the dissemination of the so-called cosmopolitan RABV lineage of dog worldwide (Leonard et al. Citation2002; Verginelli et al. Citation2005). Even though the hypothesis of dispersal of RABV via trans-oceanic travel is reported often, it has never been subjected to thorough examination by the use of modern molecular phylogenetics. For determination of the biodiversity of rabies in dogs along with its spatial as well as temporal distribution, sequencing and analysis of a large data set of RABV that include several isolates from 55 different nations of the world have been done. For enhancement of the power of phylogenetic analysis evolutionary patterns have been investigated by the use of sequences of both the complete nucleoprotein as well as glycoprotein genes (David et al. Citation2000; Bourhy et al. Citation2008). Spread of the disease from neighbouring infected areas to rabies-free areas has been reported in the border region of South Korea and Flores Island of Indonesia. It is therefore necessary to give special attention to rabies as an important emerging as well as re-emerging disease even in countries that are free from the disease (Kim et al. Citation2005; Sugiyama and Ito Citation2007).
To decipher the molecular diversity of Indian RABV isolated from the brain of dogs, cats, cattle and wildlife partial sequencing of N and P genes are done. The results of phylogenetic analysis using N gene revealed that all the Indian isolates are closely related with a single-cluster under arctic/arctic-like viruses. However, two distinct clusters were realized in P gene-based phylogeny (Reddy et al. Citation2011). Furthermore, Cherian et al. (Citation2015) reported that G gene of RABV isolated from the brain of six different species during 2001 to 2014 from the southern parts of India (Kerala and Karnataka), northern parts of India (Uttar Pradesh, Madhya Pradesh, Rajasthan, Delhi) and Gujarat showed all the Indian isolates were genetically related to Arctic-like 1a lineage viruses. Furthermore, all the Indian RABV isolates had 95.5%–100% homology with geography, but not with host species.
4. The virus and genome organization
Rabies virion, a bullet shaped enveloped infectious particle (180 nm x 75 nm in size), having 12 Kb negative sense single-stranded RNA genome, belongs to the Lyssavirus genus of the Rhabdoviridae family and Mononegavirale order (http://ictv.global/9th-report/). The RABV is not viable outside the host and can be inactivated by sunlight, heat and desiccation (Leung et al. Citation2007). Based on sequence and phylogenetic studies, 7 distinct genotypes of RABV are known to occur in the nature (Heaton et al. Citation1999). The classical RABV (RV-genotype 1) and its field strains are known world over and cause rabies in majority of the cases in humans and animals. Rabies-related viruses (RRVs), namely Lagos bat virus (genotype 2), Mokola virus (genotype 3) and Duvenhage virus (genotype 4) are widely distributed in Africa, while European bat Lyssavirus (EBLs 1 and 2; genotype 5 and 6, respectively) are limited to western and eastern Europe. Australian bat Lyssavirus (ABLV), a new 7th genotype, has been identified (Gould et al. Citation1998; Fauquet et al. Citation2004; Paweska et al. Citation2006). In Australia, presently RABV is absent in land dwelling animals however, ABLV is present in bats, and can be transmitted from bats to humans and animals. The ABLV was first identified in 1996 and till now, only three cases of RABV infection caused by ABLV in human have been reported in Queensland due to scratch or bite by bats (Hanna et al. Citation2000; Francis et al. Citation2014). Furthermore, four novel rabies-related lyssaviruses have been recovered from insectivorous bats in Eurasia; Bokeloh bat lyssavirus (Freuling et al. Citation2011); Irkut, Aravan, Khujand and West Caucasian Bat Viruses (Arai et al. Citation2003; Kuzmin et al. Citation2003; Liu et al. Citation2013) and Ikoma lyssavirus (Marston et al. Citation2012). The RRVs except Lagos bat virus are also well defined causal agent of fatal classical rabies-like disease in humans. So far, five human deaths have been distinctly related with RRVs (Smith Citation1996).
The ssRNA of RABV contains five monocistronic genes relate to five viral proteins whose order is highly conserved (from 3' leader sequence, N, NS, M, G and L) (Yousaf et al. Citation2012). There are intergenic sequences between the protein coding regions of the genome; N–NS, NS–M, M–G and G–L, where the long intergenic region between G and L is called a pseudogene (Tordo et al. Citation1986). Five proteins encoded by viral genome are glycoprotein (G), nucleoprotein (N), matrix protein (M), non-structural proteins (NS) and RNA-dependent RNA polymerase protein (L) (Woldehiwet Citation2005). N gene (1334 bases) encodes for nucleoprotein (a major structural protein of capsid that encapsulates the viral unsegmented negative-stranded RNA, 1250 nucleoprotein copies) and NS (978 bases) and L (6381 bases) genes encode for non-structural proteins known as transcriptase-associated phosphoprotein and virion-associated transcriptase, respectively, and these together with RNA constitute nucleoprotein core of the virion (Anilionis et al. Citation1981; Rupprecht et al. Citation2002). If the nucleoprotein is not phosphorylated, both viral transcription and replication are decreased (Wu et al. Citation2002). Till now, cell surface receptors of rhabdoviruses were not described; however, some research findings revealed that the phospholipids, particularly phophatidyl serine act as cell surface receptor. The RABV polymerase produces 5 distinct mRNAs for every protein. These mRNAs undergo capping, methylation and polyadenylation. The negative-sense genomic RNA is transcribed into positive sense strand by RNA polymerase. The amount of N protein of RABV determines the transition between transcription and replication activities of genomic RNAs (Yousaf et al. Citation2012). M gene (840 bases) encodes matrix protein (1800 copies of 229 amino acid residue), which helps to condense the nucleocapsid into helical form. G gene (1674 bases) encodes the transmembrane glycoprotein (495 amino acids residue with 3 ectodomains), which is located in lipid bilayer envelope as spikes. The G protein (serotype specific) acts as protective and neutralising antigen (Cox et al. Citation1977). Biologically, it is responsible for binding target cell receptors (myocytes, neurons, acini of salivary glands) mediating the binding of envelope of virus with host endosomal membrane, which is pH-dependent (Gaudin et al. Citation1991; Whitt et al. Citation1991), enhances the entry of virus from the peripheral area into central nervous system (CNS) (Mazarakis et al. Citation2001), transsynaptic spread within the CNS (Coulon et al. Citation1983; Kucera et al. Citation1985; Etessami et al. Citation2000) and budding of virus (Mebatsion et al. Citation1999). Any change in the sequence of amino acids of G protein epitopes brings about changes in the pathogenicity, antigenicity or immunological characters of the virus. Although, all the strains of RABV are not antigenically similar owing to the difference in G protein ectodomain and therefore do not cross-neutralise with antisera from other RABVs. Yet, these strains are well protected by the laboratory vaccine strains, which have been in use since quite long. The development of monoclonal antibodies (mAbs) rose against G and N antigens has facilitated serotyping and viral differentiation of RABVs (Warrell and Warrell Citation2004). The N protein is involved in encapsidation of the genomic RNA, where each N protein possess two lobes angled together to create a cavity which encloses 7 or 9 RNA bases and thus forms an active cytoplasmic nucleocapsid (NC) necessary for replication of virus (Yang et al. Citation1998). The group specific N protein exhibits precipitating, complement fixing, immunoflourescent and enzyme-linked immunosorbant assay (ELISA) activity and thus plays a major role in diagnosis and virus identification.
Other than these two major proteins three other proteins viz., nominal phosphoprotein (NS), matrix proteins (M) and RNA-dependent RNA polymerase are vital for the survival and replication of RABV (Larson et al. Citation1991, Citation1992; Wunner Citation1991). The P protein forms NC that wraps around the viral RNA along with the N and L proteins and is involved in transcription and replication along with the L protein (Nadin-Davies et al. 2002; Kobayashi et al. Citation2007). The P protein also functions as a chaperone of soluble nascent N protein (Gigant et al. Citation2000) and is an important determinant in retrograde transport of the virus within axons after binding with dynein light chain LC8 (Jacob et al. Citation2000). The M protein is responsible for the assembly and budding of virus by regulating the balance of virus transcription and replication and also interacts with the transmembrane spikes of G protein (Finke and Conzelmann Citation2003). L gene which codes for polymerase also plays role in pathogenesis along with G gene (Finke et al. Citation2000).
Detailed transcription, replication (Albertini et al. Citation2011) and structural aspects (Albertini et al. Citation2008) of RABV replication have been reported. RABV glycoprotein acts as crucial factor in the pathogenic mechanisms and induction of innate immune responses (Zhang et al. Citation2013a). Phosphoprotein (P) of RABV helps in replication of virus in muscle cells by blocking the interferon system of host, which ultimately, promotes the RABV infection in the peripheral NS (Brzozka et al. Citation2006; Yamaoka et al. Citation2013).
Strong cytotoxic lymphocytes and weak T-helper cells responses are also generated by these NS proteins (Larson et al. Citation1991). In virus assembly and budding through interaction with the cytoplasmic domain of G protein and ribonucleoprotein complex (RNP), the M proteins play essential role (Wunner Citation1991). RNA-dependent RNA polymerase is also known as L protein and contains 2,142 amino acids and mainly plays role in various processes of viral RNAs (Banerjee Citation1987; Wunner Citation1991).
5. Mode of transmission
From CNS RABV reaches the salivary glands via cranial nerves (facial and glossopharyngeal nerves) and then it is excreted in saliva, which is ready to be transmitted to a new host. Most common way of transmission for rabies (90%) is bite of infected animals like dogs and cats, because of their intimate association with human being (Baer Citation1991; Chhabra and Ichhpujani Citation2003; Blanton et al. Citation2009). Most of the nations in the world, particularly in Asia and Africa, dog bite are responsible for 85%–95% of rabies cases in human (Tang et al. Citation2005; Fitzpatrick et al. Citation2012), which is generally occurs to the victims in the form of physical and emotional trauma (Dwyer et al. Citation2007). Usually RABV gains entry into the body via the wounds or cuts, not through the intact skin. So, spread needs deposition of RABV from the saliva or infected neural tissue into the bite wounds, open cuts in the skin and mucous membranes (Wyatt Citation2007; Aghahowa and Ogbevoen Citation2010). The risk of rabies infection by bite is 5%–80%, which is approximately 50 times more than by a licks or scratches, occurrence of which is 0.1%–1% (Hemachudha et al. Citation2013). Mortality in RABV infection depends on the severity of infection, location of the bite wound and sufficient amount of virus in the saliva (Warrell and Warrell Citation2004; Hemachudha et al. Citation2013). RABV isolates from bats are more virulent when injected superficially into the epidermis because the virus replicates more rapidly in non-neuronal cells and at lower temperatures than do dog RABVs. Percutaneous infection probably occurs during unnoticed skin contact and superficial bite and scratches.
In the last 50 years, few non-bite exposures have been documented in humans (Gibbons Citation2002). But the number of cases of rabies not being transmitted by animal bite is less (Dietzschold and Koprowski Citation2004; nBronnert et al. Citation2007). The non-bite exposure includes inhalation of aerosolized RABV into the body system at higher concentration, organ and cornea transplants, and contamination of open wounds, abrasions, mucous membranes with rabies antigen laden saliva or with infectious material such as brain tissue from a rabid animal. Disease transmission through organ transplantation especially corneal transplantation was reported in German patients during 2005 (Javadi et al. Citation1996; Hellenbrand et al. Citation2005). Similar case was documented in the United States during 2004 when 4 transplant recipients became infected from an infected organ donor, all resulting in the deaths of the organ recipients (Krebs et al. Citation2005; Srinivasan et al. Citation2005; Jackson Citation2008). So, it was suggested that donors, mainly those with nervous signs, must be tested for rabies (Dietzschold and Koprowski Citation2005). Theoretically, bites from rabies infected humans can transmit the disease but it was less well confirmed (Helmick et al. Citation1987; Krebs et al. Citation1995; Leung et al. Citation2007). There is only one report of a human with encephalitic rabies biting another human (Feder et al. Citation2012). Relatives and health workers can be of potential risk while having unprotected contact with infected people and direct contact with secretions containing high concentration of virus (Helmick et al. Citation1987; Fekadu et al. Citation1992). Standard barrier precautions should be implemented while caring for an infected patient to minimize any risk of the transmission. Cases have been documented due to airborne exposure in laboratories during vaccine production (Winkler et al. Citation1973) and in caves occupied by many bats infected with RABV (Gibbons Citation2002). Transmission of rabies from the gastro-intestinal tract was also documented (Constantine Citation1962; CDC Citation1999). Exposure to vaccines during vaccination for animals may have potential risk when vaccines containing live attenuated virus. Pre- and post-exposure prophylaxis is required to tackle these conditions.
Skinning and handling of carcasses infected with rabies in refrigeration plants, butcher shops and slaughterhouses including veterinarians are at risk. So far, neither rabies transmission through transfusion of blood nor viraemia during RABV infection has been reported in both animals and Citationhumans (Consales and Bolzan Citation2007). During incubation stage, RABV strictly remains in the intra-neuronal condition. Still not known, during incubation stage of rabies whether apparently healthy blood donors can transmit the disease to recipients. So, after PEP against rabies in human, blood and organ donation is not allowed for one year. RABV may be excreted from milk of woman, and one case has been suspected for transmission of rabies from a mother to infant through breast milk feeding (Dutta Citation1998). Transplacental transmission has been reported in animals but still has not been documented in humans. Furthermore, many pregnant women with encephalitis due to rabies infection were delivered healthy babies.
Dog slaughtering is also one of the modes of transmission in many countries because dog meat is a delicacy in nations like China (Rupert Citation2002; Clifton Citation2003; Tang et al. Citation2005), Cambodia (ACPA Citation2013), South Korea (Kim et al. Citation2005; Podberscek Citation2009), Vietnam (Avieli Citation2011; Nguyen et al. Citation2011; Ares and Burke Citation2015), Thailand (Podberscek Citation2009; Avieli Citation2011), Indonesia (Shepherd Citation2012; Mahardika et al. Citation2014; Ares and Burke Citation2015), India (North-eastern states of India, especially Mizoram, Nagaland and Manipur) (Mao Citation2010; Lal Citation2014) and in African continent including Ghana, Nigeria and Cameroon (Simmons Citation1994; Ekanem et al. Citation2013; Garba et al. Citation2013; Ajoke et al. Citation2014). Presence of RABV in the brains of apparently healthy dogs used for slaughtering in many dog markets has been reported (Nguyen et al. Citation2011), which predisposes the butchers to rabies (Hambolu et al. Citation2013; Mshelbwala et al. Citation2013). Factors that are considered important in the epidemiology of rabies in dog slaughterhouses include the dog trade, slaughter processes and the consumption of dog meat. Dog without any medical history are assembled in a tight cage, increasing the spread of rabies among the dogs (Ekanem et al. Citation2013). The middle man involved in capturing of the dogs are at potential risk as they are often having the threat of scratching and bite, thus exposing them to rabies (Mshelbwala et al. Citation2013). Most of the dog markets are located in discrete areas, which are close to residential areas (Ekanem et al. Citation2013) and, moreover they are not government owned. The unhygienic conditions of the slaughterhouses are alarming and this has been shown to aid the transmission of rabies and other infectious diseases (Dacheux et al. Citation2012). During dog slaughter, the organs or parts of dogs were freely disseminated in the environment due to poor hygienic practices and unorganized slaughterhouses; which further favours the spread of the RABV to remote places where the meat and by-products of dogs were supplied to ultimate consumers. However, actually eating of dog meat does not cause the disease (Garba et al. Citation2013), but during catching, handling, loading, holding, transportation after transport keeping in the cages and during slaughtering of dogs increases the risk of transmission. Furthermore, majority of the butchers working in the slaughterhouses are having no basic or little education and absence of basic knowledge regarding the zoonotic significance of rabies (Garba et al. Citation2013).
6. Epidemiological importance of bats in transmission of RABV
Bats are unique true flying mammals and have a specialized habit of eating various kinds of diet including fruits, night-flying insects, etc. Hematophagous (vampire) bats solely feed on blood and responsible for transmission of various emerging and re-emerging diseases including rabies (Uieda et al. Citation1995; Rupprecht et al. Citation2011), which created an inspiration among scientists to work on biology of bats and various risk associated with bats. In Latin America, Vampire and non-hematophagous bats usually attack the humans and plays significant role in the transmission of RABV to humans (Dantas-Torres Citation2008). The first report of death in humans due to vampire bat attack was reported from the time of the Spanish colonization of the Americas during sixteenth century (De Oviedo and Valdes Citation1950). Transmission of RABV from vampire bats to cattle has been reported 100 years before (Haupt and Rehaag Citation1921). The first outbreak of rabies in humans due to vampire bats has been documented in Trinidad during 1927 (Pawan Citation1936). Still, similar outbreaks are continuing in the present and are staying as a challenging threat to veterinary and public health agencies. The increased number of vampire bats in the America's is directly associated with disease outbreak risk in humans and animals (Langoni et al. Citation2008). Rabies transmission through bites of vampire bats have been reported from various states in the United States of America (USA) as well as in Chile, Venezuela, Mexico, Peru, Colombia and other New World countries (Schneider et al. Citation1996; Warner et al. Citation1999; Velasco-Villa et al. Citation2002; da Rosa et al. Citation2006). First case of rabies in bats has been reported from Germany in 1954 and till now 1064 cases of rabies have been documented from 16 European countries in 11 out of 45 indigenous species of bats (WHO Citation2016). Data from over a period of time indicated that developed nations like Canada and USA, mostly bats were responsible for the development of rabies in humans where as in developing countries like India canines were predominately responsible.
Generally in urban areas, big colonies of bats are residing close to human habitat and the contact between human and bats can ultimately leads to transmission of disease in humans (Mccall et al. Citation2000). Bats are considered as important wildlife reservoirs for RABV variants. Latest findings suggested that small, apparently insignificant and unnoticed bites from bats plays important role in the transmission of RABV, because saliva from infected bats contains RABV. The infection can also be transmitted via open wounds and mucous membranes contaminated with saliva from an infected bats (da Rosa et al. Citation2006). Most of the time bats infected with rabies may not show any clinical signs and the disease can be identified through laboratory tests only. The clinical signs in infected bats are disorientation, difficulty in flying, staring expression on eyes and behavioural changes like more aggression.
7. Species affected and reservoirs
All mammals are susceptible to rabies and can transmit the RABV, but there is great interspecies variability exists among mammals in the capability of acting as reservoirs. The primary reservoir for rabies is carnivorous mammals throughout the world (Krebs et al. Citation2005). Below 10% of the documented rabies cases occur in domesticated animals including cats, cattle and dogs predominantly (Singh et al. Citation1995; Jindal and Narang Citation1998; John Citation2005; Ngoepe et al. Citation2009). Raccoons, skunks, bats and foxes are the wild animals from which the huge proportion of rabies cases are reported every year (Davis et al. Citation2012, Citation2013; Streicker et al. Citation2012, Citation2013; Weant and Baker Citation2013; Ellison et al. Citation2013; Kuzmina et al. Citation2013a, Citation2013b). Wildlife is the main reservoirs for the disease (Winkler and Bogel Citation1992; Rupprecht and Smith Citation1994; Yousaf et al. Citation2012). A spatial model to predict the emergence of rabies in raccoon has been developed (Recuenco et al. Citation2012).
The RABV circulates with two epidemiological cycles, which are interrelated i.e. urban and sylvatic cycle, having mainly pet dogs, cats and wild mammals like fox, raccoon, jackal, wolf, badger, mongoose and bats, etc., as vectors/reservoirs, respectively (Kuzmin et al. Citation2012; Blackwood et al. Citation2013; Condori-Condori et al. Citation2013; Escobar et al. Citation2013). However, both cycles may overlap in some geographical situations. Mainly community and stray dog population maintains the urban cycle and spill-over to pet dogs creats additional burden to human with a risk of rabies. One or at the most two species act as a vector for RABV in a particular geographical area (Blanton et al. Citation2009). In India, dogs and jackals were the major vector or reservoir. In urban areas of our country, dogs are the main reservoirs and transmit the disease through bite to humans as well as animals (Gongal and Wright Citation2011). More important factors for the sustenance of the stray dog population in India are probably the bad garbage policy and open slaughter facilities (Devleesschauwer et al. Citation2013). Again urbanization and the growth of slum areas further create favourable conditions for the sustenance of stray dog populations in developing countries like India and Nepal (Gongal Citation2005; Muzzini and Aparicio Citation2013). Decline in the vulture population, since in the 1990s, in the Indian subcontinent results in absence of a competitor for stray dogs for availability of food (Shultz et al. Citation2004). The second most important group is domestic animals like cattle, sheep and goats, camels, donkeys and then wild cats. Equine rabies is a relatively uncommon disease but still is invariably fatal and often having significant potential for human exposure (Weese Citation2002). In majority of rabies cases concerning horses, rabid skunks are the culprits but foxes, raccoons, bats and unimmunized dogs and cats also can transmit the disease (Pawaiya et al. Citation2010). A rhesus macaque is also believed to have transmitted rabies to a 10-year-old Australian boy in India (Pandey et al. Citation2002). In densely populated rural areas with different wildlife resources surrounding them may potentiate the risk of RABV infection (Karki and Thakuri Citation2010).
Few countries viz., Great Britain, New Zealand, Australia and Iceland claim to be free of disease due to either their island status, successful elimination programmes and enforcement of rigorous quarantine regulations (Arai Citation2005; Cleaveland et al. Citation2006, Citation2007; Nel and Rupprecht Citation2007; Leung et al. Citation2007; Gruzdev Citation2008; Seimenis Citation2008; Blancou Citation2008; Blanton et al. Citation2008). With the exception in Australia where other types of zoonotic lyssaviruses transmitted by flying foxes/ bats, the disease is endemic in mammals and other warm-blooded vertebrates (Wilkins and Piero Citation2007). To date, in Australia RABV does not occur in land dwelling animals. However, closely related virus but not identical to RABV like ABLV often present in Australia, and can be transmitted from bats to humans and animals (Hanna et al. Citation2000; Francis et al. Citation2014).
8. Pathogenesis of rabies
The RABV causes relatively slow but progressive disease without initial clinical signs which turns fatal after onset of clinical signs. The virus at the injected site remains hidden (eclipse) for variable time (a threshold must exceed to cause disease). The incubation or eclipse period is highly variable from 2 weeks to 6 years (avg. 2–3 months), which entirely depends on the concentration of the virus inoculated, inoculation site and density of innervations (Greene and Rupprecht Citation2006). Greatest risk factor is bites on the hands, neck, face and head mainly with bleeding lead to shorter incubation period due to the decreased length and greater number of neurons. Generally RABV can persist in the muscle for prolonged duration, which may give a chance for post-exposure treatment and clearance of RABV by the immune system of the host (Hemachudha et al. Citation2002). It gets attached through G-protein receptors to the target cells (myocytes, local sensory and motor neurons) and amplifies in muscle cells and in macrophages (Tsiang et al. Citation1986). It may then persist there up to 18 days. Then, through muscle spindles of sensory nerves or neuromuscular junction of motor nerves the virus ascends centripetally along the nerves (3 mm/hr, experimental data) and reaches the CNS to infect the nerve cells. The RABV travels along the course of peripheral nerves (through fast axonal transport system) and the transport is sternly retrograde, that suggests the infection is via both motor and sensory nerves (Mazarakis et al. Citation2001). Due to the presence of large inoculums at the site of bite, the virus may also enter in blood.
In spite of significant advancement in diagnosis, control and prevention of rabies, its pathogenesis especially in rodents using fixed strains is not clearly understood (Winkler and Bogel Citation1992; Jackson Citation2002). The advent of reverse genetics technology made it possible to identify viral elements that determine the pathogenic phenotype of RV and to obtain a better insight into the mechanisms involved in the pathogenesis of rabies. The pathogenesis begins after the entry of virus into the skin or mucous membranes. The virus begins replication in the myocytes and enters into the local sensory and motor neurons at the site of the bite (Tsiang et al. Citation1986). Experimental studies in striped skunks using Canadian isolate of street RABV obtained from skunk salivary glands to explore the events that take place during the incubation period showed that RABV is present at or near the site of the bite during most of the incubation period (Jackson Citation2010). The replication in muscle fibers may be a critical pathogenetic step for the virus to gain access to the peripheral NS (Jackson Citation2010). Usually skin and subcutaneous tissues are rich in sensory and autonomic innervations, which are involved in infection due to deeper biting of vectors, but bats generally inflict more superficial bites than terrestrial vectors making the host usually unaware of the bite. In vitro studies showed that bat RABV multiplies efficiently during lesser body temperature (34°C) when compared to normal, and has more virulence due to well adaptability in epithelial cells and fibroblasts located in the dermis results in more replication of RABV in the dermis locally. At low temperature (34°C) the possibility of low pH-dependent fusion and cell-to-cell spread of bat RABV is larger. Furthermore, bat RABV has special cellular tropism at lower temperature (Morimoto et al. Citation1996). It is followed by rapid ascending movement by binding to membrane surface molecules or neuronal receptors, like nicotinic acetylcholine receptor (Lentz et al. Citation1982), low-affinity nerve growth factor receptor (NTR75) (Tuffereau et al. Citation1998) and neural cell adhesion molecule (CD56) (Thoulouze et al. Citation1998; Jackson Citation2010) located in the endoneurium of the Schwann cells results in reaching the CNS. But there is no clear documentation whether these neuronal receptors really are necessary to complete the life cycle of RABV. Furthermore, it is recently seen that RVG–p75NTR interaction is not required for RABV replication in the primary neurons (Tuffereau et al. Citation2007). After binding with the receptor via G protein, RABV is internalized through receptor-mediated or adsorptive endocytosis (Lewis and Lentz Citation1998). RABV migrates towards the CNS through sensory and motor axons via a fast axonal retrograde transport system with a speed of 12-100 mm/day (Kelly and Strick Citation2000). After receptor mediated endocytosis, viral membranes fuse with endosomal membrane and liberate RNP into the cytoplasm, which is induced by acidic pH and mediated by the G protein (Gaudin et al. Citation1991). Rabies virus glycoprotein (RVG) located on the virus is responsible for the uptake of virus through the interaction with putative cellular receptors that promote rapid uptake, which is associated with a molecule present on the antigenic site III of RVG (Dietzschold et al. Citation1983). So any change in the amino acid sequence located in the antigenic site of RVG can lead to hindrance to uptake or re-emergence of the pathogenic phenotype (Etessami et al. Citation2000; Faber et al. Citation2005). However, in addition to RVG, rabies virus matrix protein (RVM) is also responsible for virus spread and trans-synaptic transport, and maximum interaction of M with G might be responsible for spread of virus from cell-to-cell, and transcription and replication of viral RNA (Pulmanausahakul et al. Citation2008). This is followed by transcription of the 5 genes of virus and then multiplication of negative- and positive-polarity within the cytoplasm (Lewis and Lentz Citation1998; Lafon et al. Citation2006). Phosphoprotein (P) delivers RNA-dependent RNA polymerase protein (L) to active template via N–P interaction, and L–P binding with the N–RNA template causes structural changes in the NC, which allows the polymerase access to RNA. Unlike many other viruses, the pathogenicity of RVs correlates inversely with the rate of viral RNA synthesis and the production of infectious virus particles (Pulmanausahakul et al. Citation2008), and viral RNA transcription and replication are regulated by several factors like RVM, which has been identified as a trans-acting factor that mediates the switch from initial high levels of mRNA synthesis to genomic RNA synthesis (Mebatsion et al. Citation1999). The kinetics of viral RNA replication, as well as virus particle production, is largely controlled by the RVG protein (Schnell et al. Citation1994). So, both G and M play an important role in RV pathogenesis influencing on virus replication. To evade the immune response and to preserve integrity of the neuronal network, pathogenic RV strains, but not attenuated strains, can regulate their growth rate. A lower replication level probably benefits the pathogenic RV strains by conserving the structure of neurons that are used by these viruses to reach the CNS and to escape from the immune system of host. The virus spreads from the site of replication by retrograde fast axonal transport via peripheral nerves to the neuronal cell body possibly by cytoplasmic dynein (Wang et al. Citation2005, Citation2013). Latest research findings indicated that hitch-hiking axonal vesicle transport may act as a key tactic mechanism for transport of virions for prolonged distances in axons (Klingen et al. Citation2008). Furthermore, an interaction between the dynein light chain and RVP results in linking of the RABV RNP with the host cell transport system, in that way enhancing the retrograde axonal transport of virus (Jacob et al. Citation2000; Raux et al. Citation2000). However, in the retrograde axonal transport, RABV protein is not directly involved (Tan et al. Citation2007). The replications occur first in the dorsal root ganglion and then progressively move through the CNS. Within the CNS, transmission occurs by trans-synaptic spread. Neuronal infection by RABV causes abnormalities in the neurotransmitters such as serotonin, GABA and muscarinic acetylcholine (Warrell and Warrell Citation2004), dysfunction of sodium-potassium ion channels (Iwata et al. Citation1999) and increased nitric oxide production (Sukathida et al. Citation2001; Madhu et al. Citation2016a, Citation2016b). RABV do not affect blood–brain barrier (BBB) integrity and therefore effector molecules of immune system do not enter into the CNS during pathogenic strains RABV infection in mice, inspite of the production of specific innate immunity against RABV in CNS and peripheral lymphoid organs. Attenuated RABV variants reach and replicate in the CNS, but are removed by the immune molecules that are infiltrating the CNS after crossing the BBB (Roy and Hooper Citation2007). However, Liao et al. (Citation2012) have reported alteration of BBB permeability after pathogenic RABV infection in a rat model. TNF-α-modulated ICAM-1 expression is initially upregulated in the brain of RABV infected mice before the onset of BBB permeability change, which attracts circulating immune cells (Madhu et al. Citation2016a). However, Phares et al. (Citation2007) proposed that CD4+ T cells through an IFN-γ-dependent process enhanced BBB permeability favouring the delivery of B cells and antibody to CNS. Increased secretion of chemokines such as RANTES (CCL5) and IP-10 (CXCL10) by attenuated virus, correlated with the increased permeability of BBB and inflammatory cells infiltration (Kuang et al. Citation2009; Zhao et al. Citation2009). RABV infects CNS leads to behavioural changes, probablely due to lesions in the neurons of limbic system, enhances the biting behaviour in animals to result in increased transmission of rabies to other animals. Generally, RABV travels away from the CNS along the neuronal pathways through centrifugal spread, especially via the parasympathetic NS, which is responsible for infection of the salivary glands and skin (Jackson Citation2007b).
Actin affects the uptake of RABV into epithelial cells by clathrin-mediated endocytosis (Piccinotti et al. Citation2013). Dendritic cells passively carry the RABV (Senba et al. Citation2013). The expression levels of RABV glycoprotein do not play a significant role in its pathogenicity (Wirblich and Schnell Citation2011). However, adequate N-glycosylation in the street RABV glycoprotein at 37 position, but not at 146 position was found to reduce its pathogenicity (Yamada et al. Citation2014). Other key amino acid changes associated with virus replication and virulence have also been identified (Yu et al. Citation2014). Dendritic injury and F-actin depolymerization in the hippocampus were found to be caused by street RABV (Song et al. Citation2013). The role of Hsp70 protein in the regulation of RABV infection has been demonstrated (Lahaye et al. Citation2012). The activation of interleukin-1β release in murine dendritic cells occurs on recognition of RABV by the NLRP3 inflammasome (Lawrence et al. Citation2013). Lytle et al. (Citation2013) reported that RABV based vaccines infects and activates the B cells. The phosphoprotein of wild-type RABV is responsible for sensitivity of virus to treatment with type I interferon (Niu et al. Citation2013). Studies have been conducted on infectivity of RABV-exposed macrophages (Naze et al. Citation2013). N and P protein complex of RABV recruits CCTγ to Negri bodies (NBs) and also chaperonin CCTγ identified as a host factor, which enhances the intracellular replication of RABV (Zhang et al. Citation2013b). RABV escapes from the host defences by killing the protective migrating T cells, and also sneak into the NS without causing apoptosis of infected nerve cells and maintaining the network of neurons (Lafon Citation2011). In cerebrum, RABV antigen and RNA levels have been found to be higher in furious form than paralytic form. The inflammation of brainstem is higher in paralytic form and further may inhibit the viral replication in cerebral hemispheres (Shuangshoti et al. Citation2013). Studies on alterations in the proteomes of brainstem, hippocampus and spinal cord during natural RABV infection in dogs have helped in clear understanding of molecular pathways of rabies to distinguish furious and paralytic forms (Thanomsridetchai et al. Citation2011). The patterns of monosynaptic tracing in rabies were observed to be different in studies conducted with RABV glycoprotein variants (Mori and Morimoto Citation2014). The neurotropic RABV has evolved with various mechanisms to evade from the host immune system, for better infection and replication in the NS results in fatal encephalomyelitis. It was observed that innate immune system of host enhances the infiltration of T cells and also stimulates elimination of CD8+ T cells. Therefore, RABV utilizes, to the some extent, the innate immune system to create a immunoevasive approach (Chopy et al. Citation2011a, Citation2011b; Madhu et al. Citation2016b). At the same time, during most of the rabies cases in human for 7 to 10 days after the onset of clinical signs, no immune response is identifiable which is supported with the information that immunosuppression either has no effect on the outcome of rabies or is detrimental. Generally, in rabies victims minimal level of immune response is often detectable because it cannot be ascribed to weak immunogenicity of RABV antigens. However, RABV-G and RABV-N proteins are potent B- and T-cell mitogens when injected parenterally (Dietzschold et al. Citation2003).
Despite severe neurological signs of rabies, the neuropathological findings (under natural conditions) are comparatively mild and degenerative neuronal changes are not uniquely identified (Jackson Citation2000; Iwasaki and Tobita Citation2002). Although rabies is regarded as almost fatal, few cases in humans and dogs have survived after the development of neurological illness (abortive rabies in experimental infection) (Badrane and Tordo Citation2001; Jackson Citation2003a).
Role of apoptosis in rabies viral encephalitis was investigated in different host species (Suja et al. Citation2011; Madhu et al. Citation2016a, Citation2016b). Apoptotic death of the neurons in mice was caused by a fixed strain of RV. However, the RV associated with the silver-haired bat does not induce apoptosis in mice. Apoptotic death of neurons is characteristic in brain of mice of various ages which were inoculated intracerebrally (IC) with CVS strain but not in natural rabies though the natural rabies strain is more pathogenic (Jackson and Rossiter Citation1997; Jackson and Park Citation1998; Madhu et al. Citation2016a, Citation2016b). The inhibition of apoptosis could be a strategy employed by neurotropic virus to favour its progression through the NS as there is an inverse correlation between the induction of apoptosis and the capacity of an RABV strain to invade the brain (Thoulouze et al. Citation2003). Street RABV strains express low levels of RABV-G, which cannot produce apoptosis until late stage of the infection, indicating that the virulence of a particular virus strain associated inversely with level of the expression of RABV-G and ability to produce apoptosis (Morimoto et al. Citation1999; Faber et al. Citation2002). It has been hypothesized that when the level of expression of RABV-G protein exceeding a certain threshold results in severe disturbtion of the cell membrane, leads to the stimulation of the molecules that induce apoptosis cascade (Faber et al. Citation2002). Furthermore, it has been speculated that in the CNS cells which are undergoing apoptosis without being immediately removed by phagocytic cells, will ultimately undergo secondary necrosis. In addition to these, overexpression of RABV-G protein may induce pyroptosis, a cell death pathway similar to apoptosis. Pyroptosis involves the activation of caspase 1, when compared to apoptosis and thereby results in necrosis (Ting et al. Citation2008). The amount of necrosis or pyroptosis induced by RABV infection may play an important role in the stimulation of antiviral immunity. Interestingly, apoptotic cells preserve the integrity of their cell membranes, which ultimately leads to absence of innate immune responses, while in necrotic cells the cell membrane integrity is lost and results in release of endogenous adjuvants, which can stimulate a strong innate immune response (Kono and Rock Citation2008). Apoptosis is a defence process of host deployed to restrict the spread of virus and does not have any role in the rabies pathogenesis (Jackson Citation2007b; Jackson et al. Citation2008). Furthermore, less gross and histopathological (HP) alterations in the human brain suffering from rabies have been observed inspite of the severity in the clinical neurological signs of rabies. In the brain infected with RV, necrosis of tissue or haemorrhages is not usually noticed unlike other acute viral infections affecting the CNS (Jackson and Rossiter Citation1997). Impaired neuronal dysfunction can be the characteristic of rabies (Scott et al. Citation2008; Jackson Citation2010). It has been observed that housekeeping gene expression is decreased markedly in neurons infected with RV that results in a generalized protein synthesis inhibition (Fu et al. Citation1993; Prosniak et al. Citation2001). Dhingra et al (Citation2007) found that decreased expression of various proteins in the brain homogenates of mice are responsible for docking and fusion of synaptic vesicles with the presynaptic membrane leading to neuronal dysfunction using proteomic profiling. Both the release as well as binding of serotonin (a neurotransmitter responsible for the control of sleep cycle as well as perception of pain and behaviour) is impaired in the rat brain infected with RV (Ceccaldi et al. Citation1993). Infection due to RABV affects both ion channels and neurotransmission. There is reduced functional expression of voltage-dependent sodium channels, inward rectifier potassium channels, and lower resting membrane potential reflecting membrane depolarization in the infected neuroblastoma cells of mouse. That prevents infected neurons from firing action potential and generating synaptic potentials, which ultimately results in functional impairment (Bouzamondo et al. Citation1993; Ceccaldi et al. Citation1993; Jackson and Rossiter Citation1997; Iwata et al. Citation1999; Schnell et al. Citation2010; Jackson Citation2010).
9. Immunopathology
In rabies, interestingly almost complete lack of an inflammatory response within the CNS and neuronal dysfunction, rather than neuronal death, is probably responsible for the fatal outcome of rabies under normal conditions. Besides the protective role, the immune mechanisms often have pathological consequences depending on the extent of the infection when immune effectors come into play especially in the nervous tissue. The neurotropic nature of RABV is responsible for this process, as nervous tissue is normally hidden from the immune system (Hooper et al. Citation1998). Glycoprotein of RABV is the target for neutralizing antibodies (Faber et al. Citation2002; Préhaud et al. Citation2003). It has been found that inappropriate and wide spread immune response against rabies in the CNS results in extensive immune-mediated damage in the CNS tissues (Iwasaki et al. Citation1997). ‘Early death’ mechanism is noticed in RABV infection in which more rapid mortality was reported in insufficiently vaccinated mice than unvaccinated controls. Similarly, if the immunosupressed mice were administered with immune serum and/or re-appearance of immune response against rabies results in increased mortality and clinical signs, which suggest the role of antiviral antibody in rabies immunopathogenesis (Smith et al. Citation1982). Infected immune cells may carry the virus from poorly to strongly innervated areas like lymph nodes and CNS, which might disclose how RABV can reach the CNS when introduced through organ transplants (CDC Citation2004). The astrocytes and microglia present RABV to T cells and signalling is through cytokines particularly during infiltration of mononuclear cells into the CNS. Activated T cells by RABV infection secrete IFN-γ, which stimulates microglia and astrocytes to express class I and class II major histocompatibility complex (MHC) antigens and to sensitize these MHC antigens for production of cytokines subsequently. The stimulation of microglia and astrocytes may responsible for the initiation and development of intracerebral inflammatory and immune responses. Various molecules present in the CNS namely, prostaglandin, cytokines such as IFN-α, IFN-β and IFN-γ, and endogenous neuropeptides like vasointestinal peptides and norepinephrine, can decrease the inflammatory responses by blocking the cytokine, and class I and class II MHC expression by glial cells. The initial activation and subsequent inhibition of the cytokine production and immune responses within the CNS is dependent on various factors like dynamic interaction between various peripheral immune cells and cells of CNS, activity of these cells, secretion of various cytokines like IL-1, IL-6, IFN-γ, IFN-α, and others, location and level of expression of these cytokines in the CNS, and temporal sequence in which interaction of cytokines with a specific cell occurs (Consales and Bolzan Citation2007). Defective neurotransmission involving neurotransmitters like acetylcholine, gamma-aminobutyric acid (GABA) and serotonin could be important in the pathogenesis of rabies (Consales and Bolzan Citation2007). Nitric oxide neurotoxicity may mediate neuronal dysfunction in rabies (Ubol et al. Citation2001; Madhu et al. Citation2016a, Citation2016b). Preservation of the neurons and limitation of such network by inhibiting apoptosis and limiting inflammation and destruction of T cells that invade the CNS in response to the infection is crucial for the RV neuroinvasion and transmission to another animal (Préhaud et al. Citation2003; Baloul and Lafon Citation2003). The P protein has been identified as a crucial factor for the inhibition of the IFN system (Conzelmann Citation2005).
If we consider host immune responses in the periphery after injection of RABV intramuscularly or after nasal instillation, then it is seen that in the lymph node, blood and spleen, RABV infection triggers the appearance of activated lymphocytes (CD69+) secreting cytokines (Camelo et al. Citation2001), expressing collapsin response mediator protein 2 (CRMP2) and production of circulating neutralizing antibodies. Thus, the immunosubversive strategy developed by RABV to escape the host immune response does not take place in the periphery.
10. Evasion of rabies virus from host immune responses
Laboratory adapted RABV strains like challenge virus standard (CVS) causes fatal encephalitis in animal models (Park et al. Citation2006; Lafon Citation2011; Madhu et al. Citation2016a, Citation2016b). Some of the mutant strains of RABV like Pasteur virus (PV) cause less pathogenicity with transient nonfatal abortive infection in the CNS lead to irreversible paralysis of limbs (Galelli et al. Citation2000). As we know being obligatory nature for successful replication of virus and subsequent transmission to a new host depends upon the evolution strategies that exploit the cellular machinery and modulate host cell signalling pathways, in particular, that governing premature cell death and promoting cell survival. Similarly, RABV has selected multiple sophisticated strategies to achieve its virus cycle into the host CNS from the place of inoculation (bite) to the salivary glands, where it will be excreted to infect a new host. Interestingly, progression of the RABV is not interrupted either by the immune response of host or destruction of the infected neuron, once it has entered the NS. For adequate infection in the CNS, RABV escapes the host immune response and protects the infected neurons against apoptosis or premature destruction of neurons.
T cells are inefficient for restriction of RABV infection in CNS due to inactivation of T cells by RABV (Lafon Citation2008). RABV causes death of leukocytes, and neurons were intact, which was evident through immunohistochemistry (Tobiume et al. Citation2009). These findings were confirmed in experimental mice model with encephalitic CVS strain of RABV, which revealed neuronal cytoplasm of brain and spinal cord heavily infected with RABV by immunocytochemistry, but affected neurons do not undergo death. On the contrary, migrating CD3+ T cells undergo apoptosis (Lafon Citation2005; Kojima et al. Citation2009; Rossiter et al. Citation2009). Furthermore, CVS strain also causes same pathogenesis in Nu/Nu Balb/c and Balb/c mice, which are immunocompetent, suggesting that T cells were inefficient in controlling the infection (Lafon Citation2005). In contrast, lack of T cells resulted in transformation of abortive infection into encephalitic infection, which is similar to CVS strain induced encephalitis, indicating that T cells are crucial elements in controlling of abortive RABV strain PV infection. Furthermore, abortive RABV strain PV causes apoptosis of neurons, but not T cells in the spinal cord of immunocompetent mice (Galelli et al. Citation2000). These findings suggested that T cells have a protective role in restriction of RABV infection in the NS however; T cells were inefficient to control the infection caused by encephalitic RABV strain CVS.
The reason why T cells are protective during an abortive strain of RABV infection, but not during encephalitic strain is the level of T-cell activation in the periphery (Lafon Citation2011). Abortive strain of RABV causes strong activation of T cells after abortive RABV infection and encephalitic strain of RABV causes low activation. However, this is an unlikely hypothesis because injection of an encephalitic RABV strain (silver-haired bat RABV or less pathogenic virus (CVS-F3) in the periphery resulted in same immune responses (Roy and Hooper Citation2007). Minimal entry of T cells into the NS might be the reason for absence of T-cell protection against an encephalitic strain of RABV infection. This is also an unlikely hypothesis because encephalitic strain of RABV causes strong expression of marker for blood T-cell activator (CD69) and T-cell polarization and migration marker known as collapsing response mediator protein 2 (CRMP2). As RABV infection progresses, the brain parenchyma becomes continuously infiltrated with CD3+ T cells in PV strain of RABV, whereas reduced accumulation of T cells was noticed in encephalitic strain (Lafon Citation2011). The destruction of T cells during CVS infection in brain was correlated with increased number of apoptotic cells in the NS. These findings indicated that CVS strain, but not PV strain, promotes unconducible environment for T-cell survival.
It may be surmised that RABV utilizes various intrinsic factors secreted by the NS like calcitonin-gene-related peptide, vasointestinal peptide, norepinephrine, etc., to attenuate the function of migratory T cells (Lafon Citation2011). Furthermore, it was confirmed that RABV infected brain especially non infected neurons enhance the expression of somatostatin, calcitonin-related gene peptide and vasointestinal peptides, which are responsible for dampening of T-cell activity in the NS (Weihe et al. Citation2008). Neurons infected with RABV upregulate immunosubversive molecules like B7-H1, FasL and HLA-G, which activate apoptosis cascade in activated T cells resulted in killing of migratory T cells, which is an immune evasive strategy followed by RABV similar to that of tumour cells (Lafon et al. Citation2005; Megret et al. Citation2007). Furthermore, CVS-infected neurons up regulates the FasL expression, but not PV strain. The mice lacking a functional FasL had reduced morbidity and mortality due to decreased apoptosis of T cells in the NS. RABV also up regultes B7-H1 expression in neurons and B7-H1 deficient mice had less clinical signs and mortality due to less CD8+ T cell apoptosis. B7-H1 inhibits T-cell activation and cell-mediated toxic function of T cells. Tumor necrosis factor-alpha (TNF-α), interferons (IFNs) and Toll-like receptor (TLR) stimulation are potent activators of B7-H1 expression (Schreiner et al. Citation2004; Liu et al. Citation2007; Lafon et al. Citation2008; Pulko et al. Citation2009). IFN-γ and TNF-α are less potent activators (Lafon et al. Citation2008). Therefore, in order to express B7-H1 in the neurons of RABV infected CNS and neurons should require IFN to be produced during the course of RABV infection due to up regulation of TLRs (Lafon et al. Citation2006; Tang et al. Citation2007, Citation2008; Peltier et al. Citation2010). This may be seen as an unexpected situation, as IFN is supposed to fight infection instead of promoting infection (Lafon et al. Citation2008; Sommereyns et al. Citation2008; Pulko et al. Citation2009). Dampening the IFN response favours RABV infection (Ito et al. Citation2010). In conclusion, IFN might be required to promote B7-H1-mediated immune evasion because B7-H1 is an IFN-dependent gene. As B7-H1 is critical for the successful immunoevasive strategy of RABV, it can be surmised that RABV pathogenicity relies paradoxically on the protective mechanism of IFN production, which is triggered by the host to fight the infection.
Other mechanisms responsible for RABV mediated immunoevasive strategies are limited neuroinflammation (Fu et al. Citation1993; Meuth et al. Citation2008). If the virus strain is more pathogenic, then inflammatory response is less acute (Hicks et al. Citation2009). Pathogenic RABV strains cause low inflammation resulted in impermeability of the BBB, but non-pathogenic RABV strains cause transient opening of the BBB (Roy and Hooper Citation2007). Furthermore, during the course of encephalitic strain of RABV infection, entirely B cells are absent in brain, which are responsible for secretion of neutralizing antibodies against RABV (Kojima et al. Citation2010), indicating that limited entry or targeted killing of migratory B cells could also responsible for immunoevasive strategy.
Preservation of neurons and neuronal network integrity is a critical factor for successful infection of RABV that spread in the NS using axonal transport and virus transmission at synapses (Tsunoda et al. Citation2007). RABV replicates in the CNS of host and travels by transneural transfer exclusively in a retrograde direction in from of axonal vesicles (Klingen et al. Citation2008). Viral proteins are detected in the dendrites, but not in axons (Ugolini Citation1995, Citation2010). So synthesis of RABV proteins and viral particle assembly occur in cell bodies and dendrites, whereas axons were responsible for transport of viral particles to the adjacent neurons. Hence, for adequate replication of RABV in the NS needs neuronal cell bodies, which are not destroyed by premature apoptosis and neural integrity is to be preserved up to the time the virus reaches the salivary glands (Jackson et al. Citation2008; Jackson Citation2010; Ugolini Citation2010). This RABV neuroinvasiveness may be favoured by the capacity of its G protein to promote survival-signalling in the infected neurons (Prehaud et al. Citation2003). Another strategy adopted by RABV to evade host immunity is sequestration of TLR3 into Negri bodies (Daffis et al. Citation2008; Menager et al. Citation2009). TLR3 expression is required for Negri body formation and the hijacking of TLR3 into Negri bodies could be an attempt of the virus to protect the infected neuron against apoptosis. So Negri bodies can also contribute to the survival strategy of RABV.
11. Pathology of rabies
Though rabies is characterized by fatal outcome and severe neurologic signs, but gross pathological changes in the CNS are relatively less or absent due to mild inflammatory reaction. After RABV infection, peripheral nerves, spinal cord and brain show degeneration of ganglion cells, perineural as well as perivascular infiltration of mononuclear cells and neuronophagia. Neuronal degeneration leading to dysfunction of neurons, rather than death of neurons, is responsible for the production of disease (Jackson Citation2007b). Infection of gangliocytes results in an early ‘axotomy response’ with numerous autophagic compartments subsequently. At advanced stages of degeneration, there are partially membrane-bound empty vacuoles in gangliocytes (Rossiter et al. Citation2009). In the mid brain and medulla, inflammation is most marked. In the paralytic form of the rabies, the spinal cord gets mostly affected. Vascular lesions like thrombosis and haemorrhages in the brainstem, hypothalamus and limbic system are observed. Towards the late stage of development of severe clinical neurological disease, there is beading and swelling of axons and dendrites of the layer V cortical pyramidal neurons, more damage to the axons of the brainstem and the inferior cerebellar peduncle, and severe abnormalities affecting axons of cerebellar mossy fibers. Vacuolation in cortical neurons with swollen mitochondria, vacuolation in the neuropil of the cerebral cortex with axonal swellings and vacuolations were observed ultrastructurally in axons and in pre-synaptic nerve endings. These fundamental defects in RABV-infected neurons are not apparent in routine HP studies (Jackson Citation2010). Degeneration of the salivary as well as lacrymal glands, pancreas and adrenal medullae are observed focally outside the NS (Jackson Citation2003a; McKay and Wallis Citation2005). The intracytoplasmic Negri bodies are detectable in the neurons infected with RABV, which are consisting of aggregates of nucleocapsids ().
Figure 1. Gross and histopathological lesions in rabies. (A) Marked congestion of blood vessels in sulci of swollen cerebral hemispheres. (B) Massive perivascular infiltration with mononuclear cells (lymphocytes and macrophages) around dilated blood vessels in white matter of camel brain. H&E x400. (C) Dense eosinophilic and sharply outlined Negri bodies (arrow) of various sizes in the cytoplasm of intact neuron in camel brainstem section. H&E x400. (D) Brain section showing degenerated neurons, severe cuffing with mononuclear cells and edema. A Negri body is also visible in the degenerated neuron (arrow) having mild diffuse gliosis. H&E x200.
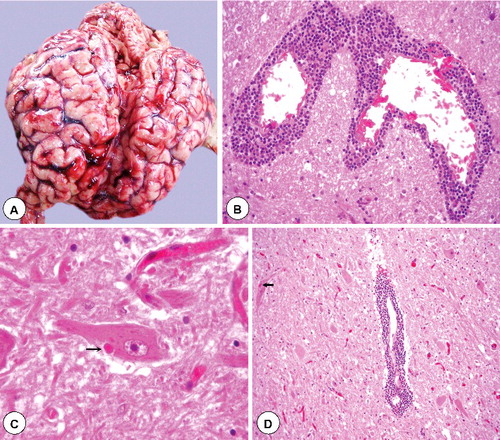
12. Diagnosis of rabies
12.1. Based on history and clinical signs
The clinical signs of rabies are confused with other neurological sings caused by other neurotropic etiological agents. Rabies infection has variable and lengthy incubation period in humans and animals generally last up to 20 to 90 days (Jackson Citation2010; Hemachudha et al. Citation2013). In 75% of dogs, the early signs (2–5 days duration) progress to paralytic or dumb forms. This is very risky while attending the dumb form of cases. Paralysis as well as mortality happens in both clinical forms at 4 to 8 days after the appearance of clinical signs. Based on some typical clinical sings in dogs and other animal species, a tentative diagnosis can be made (Blanton et al. Citation2009, Citation2010). The animals showing abnormal behaviour should be kept in isolation where they cannot bite others for 10 days. Marked changes in behaviour are seen in the prodromal phase of rabies. They vary with the species like irritable, increased sensitivity to noise and light, more alert, restless and friendly, aggressive (more in cat) and attack without provocation, or become depressed, hiding in dark places, slight pyrexia, impaired corneal reflex and self-mutilation at the site of the bite. In excitative phase, nervous signs like irritability, vicious bite and attack, muscle tremors, flaccidity or in-coordination, pica, spasm and paralysis of deglutination, change in voice, difficulty in swallowing, drooling as well as frothing of saliva, dropping of jaw, paralysis, coma and death. No specific lesions are observed grossly in the brain. However, wound due to the bite and presence of foreign bodies in stomach due to pica may be suspected for rabies. The disease should be differentiated from canine distemper, canine, equine and bovine encephalitis, hepatic encephalopathy, thiamine deficiency (cats), poisoning due to lead and organochloride compounds, benzoic acid, strychnine poisoning, pseudorabies, spongiform encephalopathy and listeriosis (Campbell and Charlton Citation1988; Baer Citation1991; Suraweera et al. Citation2012). Bat-acquired rabies may present with different clinical manifestations than dog-acquired rabies. These findings may help in improving the early diagnosis of rabies (Udow et al. Citation2013).
Human rabies may manifest in encephalitic (furious) or paralytic (dumb) forms, where brainstem is involved in both clinical forms (Hemachudha et al. Citation2002). Prodromal symptoms of rabies includes itching, pain, or parasthesia at the site of bite wound (Jackson Citation2007a; Nigg and Walker Citation2009) and gastrointestinal upset, whereas furious rabies is characterized by irritability, agitation, hyperaesthesia, autonomic disturbances with pathognomonic symptom of hydrophobia due to a triad of inspiratory muscle spasm, painful laryngospasm, terror (fear of swallowing), aerophobia and generalised flaccid paralysis (Meslin Citation2005; Nigg and Walker Citation2009). In human beings, furious form of rabies should be differentiated from delirium tremens, botulism, diphtheria, drug ingestion (phenothiazines and amphetamines), and plant ingestion (Datura fastuosa), whereas paralytic rabies should be differentiated from Guillain-Barre syndrome, polio and herpesvirus simiae because of its slower nature and more diffuse neurological features (Leung et al. Citation2007). Typical symptoms of brain involvement are spasms in response to auditory, tactile, olfactory and visual stimuli (hydrophobia and aerophobia) alternating with periods of confusion, agitation, lucidity and symptoms of autonomic dysfunction. In paralytic form of rabies, excitation is less common, and only 50% of these patients have phobic spasms (Consales and Bolzan Citation2007). Non-classical signs can be noticed in patients infected with bat-related RABVs, which include radicular pain, neuropathic pain, objective motor and sensory deficits, and during the prodromal phase choreiform movements in the bitten limb.
Being a major zoonosis, precise and rapid detection of rabies is required in suspected cases for early treatment and effective prevention/control measures. The clinical signs of rabies differ from species to species that depends upon the damage caused by the virus to the limbic system. This in turn controls the behaviour and so the gross lesions are also not pathognomonic (Frana et al. Citation2008). Results of magnetic resonance imaging (MRI) technique were same for both paralytic and furious forms of rabies in human; however, they were more pronounced in paralytic forms of rabies than furious form in dogs, in which diagnosis should be made earlier in the course of disease. Newer imaging techniques like diffusion tensor imaging and diffusion-weighted imaging have been used in the RABV-infected dogs and results were compared with normal dogs to create mean diffusivity maps and fractional anisotropy (Laothamatas et al. Citation2011). Biomarkers for rabies have been identified using quantitative proteomics (Venugopal et al. Citation2013). Traditional rapid Seller's staining and HP methods which are still in use, however, fail to detect positive rabies cases in the absence of Negri bodies and cannot be relied upon (). To overcome such difficult situations, standardization of number of laboratory tests have been done to detect the RABV, its antibodies and the viral nucleic acid (Barrat et al. Citation2006; Frana et al. Citation2008; Fooks et al. Citation2009; Faizee et al. Citation2012).
Figure 2. Diagnostic techniques for rabies. (A) Brain impression smear showing oval or round magenta coloured Negri bodies (arrow) in the cytoplasm of neuron. Seller's stain x1000. (B) Intense brown colour signals of rabies virus antigen in the cytoplasm of pyramidal neurons in experimentally infected mice brain with challenge virus standard (CVS) strain of rabies virus. IHC-DAB-MH x200. (C) Brain impression smear showing bright apple green dusty fluorescent signals of rabies virus antigen in the cytoplasm of neurons in a spotted deer. Inset showing plenty of specific signals in the cytoplasmic processes and stroma of neuron. dFAT x200. (D). Bright apple green fluorescent signals of rabies virus antigen in the smear of saliva in a dog. dFAT x200.
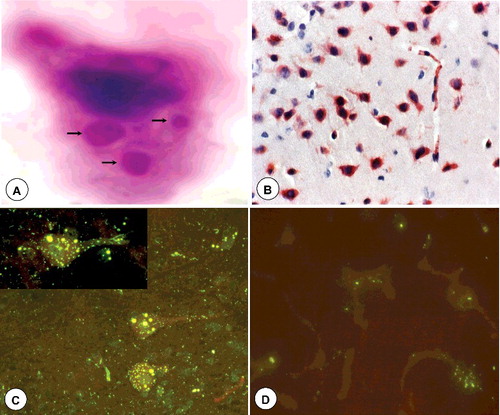
12.2. Clinical samples
The antemortem diagnosis is done in the clinical samples like CSF, saliva, swabs from the nasal mucosa, eye and throat, impression smears from eyeball and cornea, biopsies from facial and nuchal skin and the tissues from the brain (brainstem, cerebellum and hippocampus) in the dead animals (Warrell and Warrell Citation2004). An intra-vitam test is the first indicated diagnostic test for rabies, applicable to either dead or living individuals. The cornea test is a new method for the intra-vitam diagnosis of rabies. Samples for diagnosis should be transported using glycerol or saline at low temperature (Shankar Citation2009). Skin biopsies are useful material for ante- and postmortem diagnosis of rabies in humans (Blenden et al. Citation1986) and animals (Blenden et al. Citation1983). The viral antigen was identified in the peripheral nerves surrounding the hair follicles, and the positive rate of diagnosis enhanced as infection progresses (Blenden et al. Citation1986). Tactile hairs (follicle-sinus complex) in the muzzle skin of dogs can be used as an alternative material for postmortem diagnosis of rabies. The RABV antigen was detected in the outer root sheath at the ring sinus, which was overlapped with Merkel cell markers staining such as cytokeratin 20 and CAM5.2. Merkel cells are targets of the RABV in rabid dogs (Shimatsu et al. Citation2016).
12.3. Isolation of the virus
The isolation of RABV in 2 days old new-born albino mice or 3–4-weeks-old or in cell lines (mouse neurobrastoma cell line – Neuro2a/CCL 131, baby hamster kidney (BHK) 21/C13, American type culture collection (ATCC), Vero cell and McCoy cell) are the most reliable methods of diagnosis of rabies. The replication of virus in both systems is revealed by direct fluorescent antibody test (dFAT). The CCL 131 (ATCC) neuroblastoma cell line is most susceptible to street RABV without any adaptation step. It is therefore used routinely for isolation. The results are obtained after 18 hours of inoculation. The rabies tissue culture infection test (RTCIT) on BHK21/C13 cells also yields result within 24 to 48 h after staining with dFAT (Zavadova and Svrcek Citation1994). The addition of diethyl aminoethyl cellulose (DEAE)-dextran to the cell culture increases the invasiveness of the virus strains slightly (vnukovo-32/107 and street strain of fox origin). Rudd and Trimarchi (Citation1989) optimised the sensitivity of mouse neuroblastoma cell culture test for isolating the street RABV employing DEAE-dextran and minimum 4-day incubation of virus. In comparison to FAT, mouse inoculation test (MIT) procedure is superior and in New York State laboratories is used MIT as a routine diagnosis. The McCoy cells have shown cytopathic effect within 24–72 hour following infection with fixed and street RABV. They act as a useful tool based on cytopathic effect (CPE), more production of virus and high interferon (IFN) sensitivity (Consales et al. Citation1990; Frana et al. Citation2008).
12.4. Detection of virus and its antigens
In enzootic areas, diagnostic tests that are cost effective are required for studying the prevalence as well as distribution and transmission of RABV among reservoir hosts by detecting RABV antigen. Demonstration of Negri bodies as acidophilic, oval or round and highly refringent to haematoxylin-eosin staining, magenta color Negri bodies with small (0.2–0.5 µm) and dark-blue interior basophilic granules by Seller's staining and red colour to Mann's staining can be done in impression smears and nervous tissue sections using light microscopy. The methods employed in histological sections like Seller's stain can no longer be recommended because they have very low sensitivity and should be abandoned. Electron microscopy can be used to detect viral inclusions and virus particles ().
An indirect rapid immunohistochemistry test (IRIT) has been developed to detect as well as differentiate RV variants further evaluating by traditional microscopy ((B)). This causes reduction in association costs of acquiring as well as maintaining laboratory equipments that are specialized. This particular test utilizes touch impressions of frozen brain or monolayer cell culture fixed in buffered formalin and a panel of anti-nucleoprotein monoclonal antibodies of murine origin and goat anti-mouse antibody (biotin labelled) which is commercially available. Antigenic localization and characterization during rabies infection in humans and animals is augmented by the use of such laboratory diagnostic method (Diaz et al. Citation1994; Dyer et al. Citation2013).
The direct detection of RABV antigen is carried out by immunofluorescence, immunoperoxidase technique (IPT) and enzyme immunoassays (Liu et al. Citation2010; Robardet et al. Citation2011; Mani & Madhusudana Citation2013). These tests are reliable and quicker. Immunoperoxidase tests (avidin-biotin complex i.e. ABC and streptavidin-biotin complex, peroxidase antiperoxidase i.e. PAP) are used in tissue sections fixed with formalin and paraffin-embedded. The reaction in both ABC and PAP is seen as sharply demarcated brown precipitate within the neuronal cytoplasm. The ABC/ horseradish peroxidase test produced clearer and more deeply coloured precipitate than PAP (Kotwal et al. Citation1988; Last et al. Citation1994; Jayakumar et al. Citation1994, Citation1995; Frana et al. Citation2008). Hamir and Moser (Citation1994) described the ABC test useful in early diagnosis of rabies, when conventional HP and FAT fail to detect lesion or viral antigen. Reetz et al. (Citation1990) detected rabies antigen in pericaryon and processes of neurons of experimental mice brain in both PAP and protein-A-gold silver technique (PAGs). Even on higher dilution, the PAGs were found effective. Jayakumar and Ramadas (Citation1991) employed dFAT, IPT, counterimmunoelectrophoresis (CIEP) and rapid rabies enzyme immuno-diagnosis (RREID) for detection of rabies in suspected cases. All four tests had 100% specificity. IPT and CIEP had 92.9% and 78.6% specificity, respectively, while dFAT and RREID had 100% sensitivity. Zimmer et al. (Citation1990) evaluated IPT with other tests using 187 rabies suspected samples. A specific positive reaction visualised only in neurons in which pericaryon as well as cell processes were affected. FAT and PAP detected 98% each, MIT 95%, cell culture 81% and HP only 53% cases. PAP test was stated to be highly reliable when only formalin-fixed, paraffin-embedded material was available.
Direct immunofluoroscent test (dFAT) is used routinely by all the laboratories engaged in diagnosis of rabies ((C) and (D)). The dFAT is the most widely recommended test for diagnosis of rabies by both OIE and WHO (OIE Citation2009; WHO Citation2013). The dFAT is the gold standard test for detection of RABV in fresh nervous tissues of dogs (Shimatsu et al. Citation2016). In fresh brain tissues, the sensitivity of the dFAT is 100% and confirmative diagnosis can be made within 2 h. However, this technique is laborious and has a high danger of virus exposure to the laboratory diagnostician. Furthermore, this technique is unsuitable due to lower sensitivity when the brain samples become autolysed during warm temperature (David Citation2012; McElhinney et al. Citation2014), and sampling of brain from dog is laborious, poses a high danger of virus exposure, and needs costly equipment and expertise. The antigen is detected in fresh or glycerolised tissues and in brain impression smears fixed in chilled acetone. The dFAT has been found suitable to detect 100% rabies positive cases even in formalin-fixed, paraffin-embedded materials and compared with PCR targeting a short region of the N gene in the fresh tissue samples from dogs, cats, foxes, racoon dogs and badger, which also detected 100% cases (Kulonen et al. Citation1999). The highest sensitivity of 99.6% was observed by FAT in brainstem than in cerebellum (99.3%), cerebrum (98.9%) and hippocampus (98.7%) (Tepsumethanon et al. Citation1997). Davis et al. (Citation1997) designed microwave oven method for fixing the brain impression slides for 55–60 sec at 50% power using PBS (pH 7.2) supplemented with 3% Tween-20 as fixative medium. Results were obtained within 1.5 h. The problem of non-specific fluorescence in brain and salivary gland impression slides could have been overcome by adsorption of the diagnostic conjugate with suspension of healthy mouse brain or counter staining with Evans blue (Skrzynecki and Januszewska Citation1996). Dutta et al. (Citation1992) found that FAT has 100% agreement with MIT and more sensitive than CIE in detecting the antigen in nervous and non-nervous tissues, while HP could detect 67.6% cases. Shashen’ ko et al. (Citation1990) could detect 141 positive cases by FAT out of 159 rabies suspected brain samples fixed in 10% formalin and 50% buffered glycerine after treating them twice with 0.4% pepsin solution for 60 min and then with 0.25% trypsin solution for 90 min. Jayakumar et al. (Citation1989) reported that dFAT and RREID were 100% efficient and equally sensitive in detecting the rabies antigen in 28 experimentally infected and in 28 rabies suspected dogs. Kang et al. (Citation2007) developed another rapid immunodiagnostic test (RIDT), which is simple and can be applied in the field and in developing nations having very less diagnostic facilities. As an alternative to FAT, immunoperoxidase methods can be used (having similar sensitivity) but proper care must be taken for avoiding non-specific false-positive results. Use of peroxidase conjugate on fresh nervous tissues or formalin-fixed tissue sections for immunohistochemical test is one additional advantage (Lembo et al. Citation2006).
An immunochromatographic technique using lateral flow principle facilitated the diagnosis of different rabies virus strains (RABV species 1, 5, 6 and 7) and showed 100% specificity and more than 88% sensitivity when compared to RTCIT and FAT (Servat et al. Citation2012). In the brain of humans and animals RABV can directly be detected by rapid immunochromatographic test using monoclonal antibody (Ahmed et al. Citation2012). A direct rapid immunohistochemical test (dRIT) for quick detection of rabies in humans and animals has been developed (Madhusudana et al. Citation2012). RT-PCR–ELISA has recently been identified for the diagnosis of RABV (AravindhBabu et al. Citation2014).
12.5. Detection of anti-rabies antibodies
Serological tests are rarely used in the diagnosis of rabies as the animals do not survive the disease long enough to release sufficient antibodies. However, in some cases post-infection antibodies persist. These tests are predominantly used for assessment of potency of various rabies vaccines (Watanabe et al. Citation2012; Wasniewski and Cliquet Citation2012; Wasniewski et al. Citation2013, Citation2014). Counter current immuno-electrophoresis (CCIE) test has been used for rabies diagnosis but not found specific and sensitive in comparison to FAT and MIT. Serological tests usually employed for detection of rabies antibodies by targeting serotype specific outer transmembrane G-protein epitopes and group specific major N-protein of RABV capsid. Serum neutralising tests are employed for virus serotyping that requires mouse neuroblastoma cells and G-protein reactive monoclonal antibodies (mAb-Gs). Analysis with reactive mAb-G antigenically is done by a varying virus and constant antibody method with a dilution of mAb-G sufficient to neutralise 3-4 log10 of homologous virus. Nadin et al. (Citation2000) reported that based on competitive binding assay and classified 27 mAbs with anti-P reactivity from recombinant RABV phosphoprotein product (GST-P) and 24 of them recognised linear epitopes in immunoblot. These anti-P mAbs were found exclusively useful for identification of lyssavirus as well as their discrimination. Jayakumar et al. (Citation1995) found avidin-biotin dot ELISA for rabies antigen detection to be more sensitive and specific than conventional ELISA. Out of 17 mAbs developed against RABV, 9 mAbs (IgG 2b) recognised G and 8 mAbs (IgG1) identified by N protein on Western blot. These mAbs reacted only with conformational epitopes on G or N protein (Shimazaki et al. Citation2000). N protein based ELISA in RABV virion captured by RABV specific polyclonal Ab on ELISA plate could detect both infective and defective interfering particles (DI) but these did not correlate highly with that of MIT (Katayama et al. Citation1999). Jayakumar et al. (Citation1994) observed dipstick ELISA in comparison to dFAT to be more specific and reliable as it did not give non-specific false positive results. A liquid phase blocking ELISA for diagnosis of antibody to N-protein of RV was developed by Esterhuysen et al. (Citation1995), which was found good for rapid determination of Ab against RABV in serum of any animal species and for epidemiological studies. Kasempimolporn et al. (Citation2000) used latex particles, coated with anti-rabies γ-globulin for detection of rabies antigen in dog saliva on glass slides. On paired saliva-brain samples from 238 dogs, the test was found to be 99% specific and 95% sensitive compared with FAT on brain smears. Xu (Citation1998) developed sandwich ELISA (SEIA) by coating microplates with cocktail of mAbs (against G and N protein) and then captured rabies street virus in the supernatant of 21 mouse brain samples. This was later treated by peroxidase conjugating mAbs against rabies M protein. Both SEIA and MIT detected 17 samples. Two samples tested initially negative for MIT but were found positive for SEIA. In another experiment Xu et al. (Citation2007) developed a MAb based capture ELISA for diagnosing specimens suspected for rabies, wherein RABV nucleocapsid was detected by a combination of four monoclonal antibodies. The assay is nowadays known as WELYSSA (a simple sandwich ELISA) that permits detection of seven genotypes of lyssavirus which are circulating in different continents. The test is valuable for its high specificity and sensitivity in comparison to other recommended tests for rabies having a low threshold of detection (0.8 ng/ml). A novel serological method for identification of RABV antibodies following vaccination has been reported (Ma et al. Citation2012). Rapid neutralizing antibody (RAPINA) detection test for identification of RABV neutralizing antibodies qualitatively and semi-quantitatively in humans and dogs has been developed (Nishizono et al. Citation2012).
For the diagnosis of RABV specific antibodies in the serum samples of dogs, Tao and Li (Citation2014) developed an immunochromatographic test strip using colloidal gold-coated staphylococcal protein A (SPA). The specificity and sensitivity of the test is 93.1% and 92.2%, respectively. It is simple, cheap and rapid technique for detection of RABV and monitor immunity during vaccination in endemic developing countries. The methods currently recommended by WHO for estimation of RABV neutralizing antibodies are rapid fluorescent focus inhibition test (RFFIT) and fluorescent antibody virus neutralization test (FAVN) (Smith et al. Citation1996; Cliquet et al. Citation1998). RFFIT is most commonly used for estimating the seroconversion following prophylactic vaccination, and to help ante-mortem diagnosis of rabies in suspected cases. Though this technique is rapid and commonly employed in rabies diagnostic laboratories, the requirement of costly fluorescent microscope and fluorochrome antibody conjugate against rabies limit its use. Madhusudana et al. (Citation2014) developed a cost-effective novel immunohistochemistry-based neutralization test and widely evaluated it along with RFFIT and showed it might serve as diagnostic tool in resource poor developing countries. The results obtained from the 25 international collaborative laboratories showed that BioPro ELISA rabies Ab kit is a good diagnostic tool for assessing the rabies antibody level in wildlife samples, while monitoring the effectiveness of oral rabies vaccination (ORV) campaigns in Europe (Wasniewski et al. Citation2016). Moeschler et al. (Citation2016) developed a novel neutralization test using modified vesicular stomatitis virus from which glycoprotein G gene was deleted and substituted with RABV envelope glycoprotein. This pseudotype virus system allows the safe, fast and sensitive detection of neutralizing antibodies against different lyssaviruses and the test was correlated with RFFIT. Bedeković et al. (Citation2016) reported that BioPro ELISA is reliable tool for diagnosis of antibodies against RABV from poor-quality samples like haemolytic thoracic fluid and muscle extracts after oral vaccination in foxes.
12.6. Detection of viral nucleic acid
Several nucleic acid (NA) based tests (in situ hybridization, PCR, genomic sequencing, etc.) have been developed, which are in use (WHO or OIE reference laboratories) for diagnosis of rabies (Wacharapluesadee et al. Citation2011; Meng et al. Citation2013). These methods detect highly specific molecular subunits of rabies RNA in brain material either in experimental or in routine specimens. The NA hybridization technique thus provides higher degree of sensitivity as well as specificity than any other tests. In situ hybridization (ISH) for detecting rabies genomic RNA and mRNA can also on tissues fixed with formalin and paraffin-embedded along with simultaneous morphological analysis of tissue section. The ISH involves interaction of specific rabies probes with complementary target sequence of viral RNA to form hybrids. The probes used in ISH are either radioactive label (3H, 35S) or non-radioactive label (digoxigenin). Jackson and Wunner (Citation1991) used 3H-ssRNA probes against 5 rabies viral proteins for detecting RNA in paraffin embedded sections of mice brain infected with fixed (CVS-11) RABV and in human rabies brain. They demonstrated multifocal genomic RNA signals in pericarya or infected neurons in mice and found diffuse distribution of mRNA around pericarya more abundant than the genomic RNA in both CVS-11 as well as street virus. The difference in distribution of signals was obtained to be due to loss of mRNA owing to post-mortem changes or poor fixation. Jackson and Reimer (Citation1989) employed 35S and 3H labelled probes specific to N-protein to detect rabies RNA in paraffin embedded sections of mice brain. The ISH signals were compared with IPT antigen detection system. Use of non-radioactive label probes has increased in rabies diagnosis lately as these are safer as well as cheaper and easier to perform (Warner et al. Citation1997; Baba Citation1999; Jackson Citation2003b). PCR product (442 bp) from rabies positive brain was labelled with digoxigenin and it could detect 63/64 brain samples that were also positive by FAT (Ganesh and Jayakumar Citation1999). PCR based tests are also used for studying viral pathogenesis and epidemiological work apart from diagnosis. Though FAT can detect RABV and RRVs within 2 h of received sample but it is not sensitive with RRVs and fails in the decomposed tissues (Heaton et al. Citation1999). In contrast, PCR is less dependent on such conditions thereby detecting even few numbers of viral particles in such samples. To carry out PCR nucleotide sequence the target genome of the (viral) pathogen must be known. The PCR coupled with sequencing of amplified product can further provide accurate genotyping of RABV. Heaton et al. (Citation1999) developed a rapid air thermocycler PCR assay (hemi-nested PCR) and detected six genotypes of RABV and RRVs within 5 h using TRIzol method of RNA extraction. They also sequenced all the RABVs with automatic sequencer within 16 h of receiving samples. The authors suggested that this technique should be included in the panel of available tests for diagnosis of rabies. PCR detection of a short chain of 139 bp target sequence near the 5' end of N gene of rabies and FAT detection of rabies antigen were found useful in determining the rabies status in fixed, paraffin-embedded materials (Kulonen et al. Citation1999). By single step reverse transcriptase PCR (RT-PCR), Arai et al. (Citation1997) studied N-gene of 11 RABVs by amplifying the most variable region using primers containing conserved regions. The sequence analysis using PILEUP (GCG package) programme helped in virus grouping. Sacramento et al. (Citation1991) designed a primer set mapping N-protein cistron allowing amplification of infected brains specifically and with higher sensitivity (fixed RABV or wild RABV strains and RRVs). Southern or dot-blot assay using labelled probes also produced identical results.
Black et al. (Citation2000) developed RT-PCR for detection of RABV (genotype 1) and RRV (EBLs 5 and 6 genotypes). Genotypes 5 and 6 were distinguished from genotype 1 when combined with specific oligonucleotide probe and PCR-ELISA. By incorporation of TaqMan probes, a rapid as well as sensitive RT-PCR has been developed for differentiating among the six established genotypes of RABV as well as RRVs. The assay causes amplification of a part of the N gene of all the rabies and rabies-related viruses. The assay can rapidly identify the viruses in the clinical specimen due to incorporation of probes specific to the particular genotypes (Kissi et al. Citation1995; Black et al. Citation2002). A simplified version of hemi-nested RT-PCR (hnRT-PCR) has been developed for determination of nucleoprotein gene of RABV specifically by Picard-Meyer et al. (Citation2004). Determination of the specificity of this method has been done by the use of seven genotypes of lyssavirus by RT-PCR along with Southern blot and sequence analysis. A higher sensitivity is shown by hnRT-PCR in order to detect small amounts of RABV nucleoprotein gene in comparison to the other diagnostic methods. This new hnRT-PCR is found to be an important diagnostic tool in the context of epidemiological investigation in relation to rabies. Alternative use of hnRT-PCR assay has been recommended in laboratories without having access to real-time-PCR equipments, which are expensive (Coertse et al. Citation2010). RT-PCR–ELISA has been developed for the diagnosis of RABV (AravindhBabu et al. Citation2014). Diagnosis of rabies has been carried out by RT-PCR assay on brain samples obtained from different species of animals after postmortem (Babu et al. Citation2012). Ante-mortem and post-mortem rabies diagnosis by RT-PCR has been demonstrated (David Citation2012). TaqMan real time-PCR was found to be quite sensitive for the diagnosis of rabies from body secretion/excretion (Dandale et al. Citation2012, Citation2013; Mani et al. Citation2014). Absence of vaccine-associated rabies cases following oral vaccination was demonstrated with the help of pyrosequencing of the RVG gene (De Benedictis et al. Citation2013).
DNA microarray has been used as a diagnostic tool for detection of nucleic acid segment of lyssaviruses including RABV (Lung et al. Citation2013). The tailored microarray has got the capability of detecting as well as distinguishing all the seven recognised members of the genus Lyssavirus by use of 624 70mer probes, both in humans and animals. The target for the microarray is the viral N gene because of the lower degrees of conservation among the seven genotypes of the lyssavirus. It further enables to generate species-specific probes. For detection as well as classification of isolates of lyssavirus into their respective genotypes, extensive use of the N gene has been done (Kissi et al. Citation1995; Bourhy et al. Citation1999).
Dupuis et al. (Citation2015) compared the quantitative RT-PCR (qRT-PCR) technique with the gold standard dFAT and results were comparable between the both techniques, with one extra sample diagnosed by qRT-PCR when compared to dFAT. Suin et al. (Citation2014) validated a two-step lyssavirus qRT-PCR for the diagnosis of various lyssavirus species using degenerate primers and the assay was highly sensitive, specific and reproducible. The specificity was 100% and the sensitivity was superior when compared to dFAT.
13. Pre-exposure vaccination and management
In modern days, the key stone to prevent and control of rabies is management of animals (Meltzer and Rupprecht Citation1998; Larghi Citation2004; Cleaveland et al. Citation2006; Rupprecht et al. Citation2006; Fooks Citation2007; Leung et al. Citation2007; Wilkins and Piero Citation2007; Rupprecht et al. Citation2008; Blancou Citation2008). On primary ground, vaccination of dogs and cats alongside eliminating stray animals and public health education, etc., are the components of animal rabies control. Subtle differences prevail concerning vaccination of horses against rabies, but in no way the vaccination of companion animals including horse can be overemphasized. The potential for exposure to rabies for horses equals that for dogs and cats and generally justifies protection to rabies through vaccination. In horses, vaccination against rabies is often overlooked unfortunately because there are not much clinical cases of equine rabies. Because of the fatal nature of the disease and human health significance, veterinarians and horse owners should strongly consider rabies vaccination in horses (Brun et al. Citation1980; Green Citation1993, Citation1997; Weese Citation2002; Monaco et al. Citation2006; www.thehorse.com, Citation2008). Preventive immunization is suggested by the WHO for all the staffs involved in handling of materials that are infected or suspected. Three injections are included in the immunization protocol at 0, 7 and 28 days. Serological estimation of antibodies should be done 1 to 3 weeks after the last immunization. Re-examination should be done in every 6 months for persons working in laboratory or every 2 years for other diagnosticians. Even if the titre goes below 0.5 International Units (IU) per ml, booster vaccination is recommended for sure. When serological monitoring is not available, booster vaccination is recommended at 1 year followed by vaccination at every 1–3 years (Cliquet and Aubert Citation2004; Nadin-Davis and Fehlner-Gardiner Citation2008). Humans and horses travelling interstate having frequent contact should be currently vaccinated against rabies (Bender and Schulman Citation2004). Two rabies vaccine manufacturers who are marketing in Canada, Rabvac 3® (Ayerst, Guayama, USA) and RM Imrab 3® (Merial, Duluth, USA), recommend vaccinating horses with a sole dose of 2 ml vaccine that starts at three months of age and repeated at one year of age followed by revaccination annually. Merial recommends administration of RM Imrab 3® by either intramuscular or subcutaneous route. Ayerst recommends administration of Rabvac 3® by intramuscular injection only. Pregnant mares must not be vaccinated with either of these vaccines. Rabies vaccine can only be sold to a licensed veterinarian. A new equine rabies vaccine: EquiRab (Intervet/Schering-Plough Animal Health, New Jersey, USA) can be aseptically injected intramuscularly that offers protection against rabies on long-term basis in a single low-dose injection annually (www.thehorse.com, Citation2008). State or provincial authorities must be approached by practitioners regarding the need for vaccination in their area. Because of the zoonotic significance veterinary technicians, veterinarians and other animal health workers should be vaccinated against rabies (Green Citation1993; Weese Citation2002; Wilkins and Piero Citation2007).
For animal use, rabies vaccines consist of live attenuated virus (e.g. Flury high egg passage, Flury low egg passage, Street-Alabama-Dufferin and Kelev), chemically or physically inactivated virus and recombinant vaccines. Embryonated eggs, CNS tissues from newborn animals and cell cultures are preferred for cultivation of virus (Lau and Hohl Citation2013). Rabies vaccines are usually lyophilised but inactivated with an adjuvant and storage can be done in liquid form. Recently, for management of rabies in free-ranging bats, modified-attenuated vaccinia Ankara (MVA) and raccoon poxvirus (RCN) vaccine vectors were administered in the Brazilian free-tailed bat (Tadarida brasiliensis) by oronasal and intramuscular routes (Stading et al. Citation2016). Significant levels of neutralizing antibody titers against rabies were identified in the serum of immunized bats. These studies highlighted the safety and immunogenicity of attenuated poxviruses and their potential use as vaccine vectors in bats. Immunization of domestic cattle and two-humped camel with canine inactivated rabies vaccine in Ningxia Hui and Inner Mongolia Autonomous Region of northwest China provides protection against rabies for at least one year (Liu et al. Citation2016).
The development of vaccines has been influenced by the advent of genetic engineering that provides more opportunities to produce inactivated antigens and to attenuate different viruses through direct mutation (Yang et al. Citation2013). Various novel approaches like application of vectors, lipopeptide vaccines, plasmid DNA and plant virus-based rabies vaccines are also being explored (Ohara et al. Citation2013). Also, the paucity of vaccine adjuvants, essentially limited to aluminum salts, is corrected at last by producing new emulsions (oil-in-water), toll-like receptor agonists, liposomes, cytokines, cpG oligonucleotides and other materials (Yusibov et al. Citation2002; Fischer et al. Citation2003; Consales and Bolzan Citation2007; Dhama et al. Citation2008; Montaner et al. Citation2012; Dhama et al. Citation2013).
There are limitations of applying vaccine preparations that are injectable especially in carnivores and wildlife. Oral vaccine formulation, therefore, has been attempted continuously with significant results in laboratory as well as field trials (Baer et al. Citation1971; Leblois et al. Citation1990; Muller et al. Citation2001; Cliquet et al. Citation2007). Oral vaccines are mainly based on the avirulent mutants or the use of recombinant vaccines (Muller et al. Citation2001; Xiang et al. Citation2003).
The efficacy of killed vaccinia rabies-glycoprotein recombinant vaccines is also proven. The preprations of these vaccines involve insertion of non-infectious nucleic acid of RABV into a vector viz., vaccinia or canary pox (Yang et al. Citation2013). It is important to note that there must not be any restriction over the entry of animals vaccinated with rabies-glycoprotein recombinant vaccines into any country since these vaccines do not contain live virus (Kieny et al. Citation1984; Brochier et al. Citation1991). For developing recombinant rabies vaccine at present adenoviruses are targeted as such with mixed success rate. Pre-existing immunity has not impaired such trials with adenoviral vectors in mice (Xiang et al. Citation2003, Citation2014). A long lasting anti-rabies immunity develops by oral immunization of dogs (Canis familiaris) with baits consist of recombinant canine adenovirus type-2 and rabies vaccine (Zhang et al. Citation2008; Xiang et al. Citation2014). Recombinant Orf virus containing the RABV glycoprotein (G) has been used as a vaccine (Amann et al. Citation2013). A recombinant RABV containing two copies of G gene has been reported to protect dogs against a virulent challenge (Liu et al. Citation2014). Inactivated vaccine consisting of recombinant rCVS-11-G strain encoding two copies of G protein from the pathogenic wild-type CVS-11 strain can act as a promising vaccine candidate for its enhanced immunogenicity (Xue et al. Citation2014). Recombinant RABVs expressing GM-CSF or flagellin have been found to be successful vaccines for oral and intramuscular vaccinations (Zhou et al. Citation2013). RABV vaccine containing ICAM-1 appears to be quite promising (Norton et al. Citation2014).
The quality of rabies vaccines can be analysed by measuring the RVG content by electrochemiluminescence assay (Smith et al. Citation2013). G proteins of rabies are proven RABV immunogenic unit and have thus been targeted for developing transgenic vegetables and food materials with versatile immunogenic response (Yang et al. Citation2013; van Dolleweerd et al. Citation2014). The expression of RABV G protein in transgenic tomatoes (McGarvey et al. Citation1995); carrots (Daucus carota) (Rojas-Anaya et al. Citation2009) and in maize (Loza-Rubio et al. Citation2012) are very examples of such expressions. A major breakthrough in developing a vaccine successfully in the form of transgenic food stuff is the stimulation of a protective immune response against RABV after oral vaccination by feeding transgenic maize to the sheep that encodes glycoprotein of RABV (Loza-Rubio et al. Citation2012).
There is need of improving antibody response induction ability in large mammals for the purpose of DNA vaccine to be a practical alternative to conventional vaccine (Ullas et al. Citation2012). In horses, anti-rabies DNA vaccine has been used for enhancing antibody response. There is improvement of both onset as well as intensity of serological responses due to the injection of DNA vaccine containing the aluminium phosphate but is not sufficiently effective for achieving seroconversion in all the horses that are vaccinated. However, on formulating DNA vaccine with the cationic lipid 1, 2 dimyristylowxypropyl-3-dimethyl-hydroxy ethyl ammonium bromide-dioleoyl phosphatidyethanolamine (DMRIE-DOPE) instead of aluminium phosphate, it has been observed that there is a very strong impact on both onset as well as intensity of serological responses. Such study is indicative of the fact that DNA vaccination in horses for rabies is feasible. Furthermore, it also suggests that DNA vaccines that are properly formulated can generate immune responses in large species of animals at a level, which can be compared with the response achieved with conventional vaccines (Fischer et al. Citation2003). The advantages and limitations of intradermal pre-exposure rabies vaccination have been reported (Mills Citation2013). The worldwide incidence of rabies and the inability of currently used vaccination strategies to provide highly potent and cost-effective therapy indicate the need for alternate control strategies (Joob Citation2013; Shah et al. Citation2014). A replication-deficient RABV-based vaccine in which the matrix gene is deleted (RABV-ΔM) was shown to be safe and induced rapid and potent virus neutralizing antibodies (McGettigan et al. Citation2014). The role of CD4+ T cell-independent B cell, IgM and IL-21 in the vaccine induced-immunity has been defined (Dorfmeier et al. Citation2012, Citation2013a, Citation2013b).
13.1. SAG2 vaccine (RABIGEN®, Virbac Laboratories, Carros, France)
Most of the modified-live RABV oral vaccines were derived from the attenuated Evelyn Rokitnicki Abelseth (ERA) virus strain. ERA strain was originated from Street-Alabama-Dufferin (SAD) RABV strain (Mähl et al. Citation2014). The original SAD strain was recovered from the salivary glands of a dog infected with RABV during 1935 in the USA. Later, this was subsequently passaged in chick embryos, mice and different cell lines resulted in production of attenuated ERA strain (Abelseth Citation1964). Furthermore, the SAD Bern strain was derived by cell-adaptation of the ERA strain, and subsequently utilized for the first trial of oral immunization in Switzerland (Steck et al. Citation1982). The SAD 5/88 and SAD B19 strains were obtained from the SAD Bern by passaging into BHK21 cells (Schneider and Cox Citation1983). But, SAD B19, SAD Bern and SAD P5/88 strains were showed mild pathogenic lesions in domestic and wild carnivores, and rodents by oral route of administration (Artois et al. Citation1992; Leblois and Flamand Citation1988; Leblois et al. Citation1990; Vos et al. Citation1999). Therefore, using all three strains in the field during oral vaccination programmes result in potential risk of disease outbreak and spread in target and non-target host species (Hostnik et al. Citation2014). SAG 2 strain (SAD Avirulent Gif) is a modified live rabies vaccine strain, which was developed from SAD Bern by two successive mutations in amino acid (Lafay et al. Citation1994). Currently in Europe, RABV strains like SAG 2, SAD Bern and SAD B19 were used for oral immunization in wildlife for rabies control programmes (Cliquet and Aubert Citation2004). The SAG 2 vaccine contains liquid suspension and packed within a PVC or aluminium sachet (Nokireki et al. Citation2016). The sachet is coated with bait matrix made of fat and fish ingredients (Lafay et al. Citation1994). The vaccine safety has been assessed in both target like red fox and raccoon dog, and various non-target host species by administering 10 times higher than the suggested dosage of the vaccine resulted in no adverse reactions in raccoon dogs (Cliquet et al. Citation2006). However, this oral bait vaccine causes occasional hypersensitivity reaction in dogs due to presence of tetracycline and gentamycin as a biomarker (Nokireki et al. Citation2016). Before 2011, no adverse reactions were reported, but in 2011, the competent authority had received a few reports of adverse reactions in dogs due to consumption of oral rabies bait vaccine. The adverse reactions in dogs are gastrointestinal symptoms like inappetence, vomiting, diarrhoea or constipation, and behavioural symptoms like listlessness, restlessness and unwillingness for hunting (Nokireki et al. Citation2016).
13.2. Post-exposure management
It is mandatory to strictly follow quarantine for 6 months for any animal exposed to a confirmed or suspected rabid animal (). Upon entry into isolation or 1 month before release, administration of rabies vaccine should be done. There are no licensed biologicals currently for PEP of domestic animals, which are not vaccinated previously. There is no reliable evidence that the sole use of vaccine will prevent the disease in these animals (Green Citation1997; Hanlon et al. Citation2002). There is need to evaluate animals overdue for a booster vaccination on a case-by-case basis for severity of exposure, time lapse since last vaccination, number of previous vaccinations, current health status and rabies epidemiology locally. In all species of livestock, rabies infection can occur but most frequently reported species are cattle and horses (Blanton et al. Citation2008). Upon exposure, there is requirement of revaccination immediately followed by observation for 45 days. Unvaccinated livestock must be kept under close observation for a period of 6 months. It is suggested to euthanize animals suggestive of developing signs of rabies and head must be shipped for testing (Green Citation1993, Citation1997).
Table 3. Type of contact, exposure and recommended post-exposure prophylaxis.
Rabies vaccines among all the vaccines can be administered uniquely pre- and post-exposure to virus. For travellers as well as veterinarians and researchers pre-exposure vaccination is appropriate, especially in regions where the disease is endemic in nature (Parola and Gautret Citation2012; Gautret and Parola Citation2012, Citation2013; Carrara et al. Citation2013; Permpalung et al. Citation2013; Weant and Baker Citation2013). As it is easy to identify the event of exposure (usually a bite) and the incubation period is adequately long post-exposure immunization is possible for inducing a protective immune response. Principally, this is possible by the production of neutralizing antibodies. Usually the immunization at post-exposure is followed by injection of immunoglobulins specific for RABV either of equine or human origin. Collectively this is called as PEP, which can be decided by the exposure level. In spite of the extreme consequences of development of a disease, it is an important factor in those areas of the world which are resource-poor (Verma et al. Citation2011; Hicks et al. Citation2012; Joseph et al. Citation2013).
14. Theraputic approaches
Rabies is almost without exception a lethal disease and once CNS signs appear, the mortlity reaches up to 100% in spite of administration of PEP. Expression of transantigen and subsequent antiviral immune response due to inoculation of plasmid vectors that encode an RABV glycoprotein is helpful against viral challenge. Due to co-inoculation of a plasmid expressing interferon-γ (IFNγ) anti-viral immune response gets reduced (Xiang and Ertl Citation1995). Adenoviral vector-delivered small interfering RNA has strong antiviral potential particularly against rabies. This is achieved by targeting the polymerase (L) and nucleoprotein (N) genes by developing adenoviral vectors (rAdV) (replication-defective) that encode siRNAs. It is found to be effective provided the siRNA is delivered in vitro in BHK-21 cells. Such approach is proven to be very much effective as alternative therapy (Sonwane et al. Citation2012). In another study by Gupta et al. (Citation2012), the potential of two small interfering RNAs (siRNAs) targeting RABV nucleoprotein (N) and polymerase (L) genes as antiviral agents against rabies have been proven. There is both in vitro as well as in vivo inhibition of viral multiplication by adenovirus expressing siRNA against N gene that confers protection against lethal viral challenge significantly. Human and equine rabies immunoglobulins are currently available for passive immunization against rabies (Both et al. Citation2012). A new generation of anti-rabies material for passive immunotherapy has recently been reported. Antibody engineering approach to synthesize a trivalent single-chain Fv (scFv50AD1-Fd), that recognizes the RABV glycoprotein, was genetically fused to the trimerization domain of the bacteriophage T4 fibritin, termed ‘foldon’ (Fd). The scFv50AD1-Fd was expressed as soluble recombinant protein in bacterial periplasmic space and purified through affinity chromatography (Turki et al. Citation2014). Aptamers have been designed to inhibit RABV replication both in vitro and in vivo (Liang et al. Citation2013, Citation2014). Feasibility studies on RABV-vectored immunocontraception in a mouse model have been carried out (Wu et al. Citation2014).
With the ability of manipulating the genome of the virus a new avenue for research on biology of the RABV and development of rabies vaccines has been done (Zhu and Guo Citation2016). In the field of anti-rabies therapy a persistent challenge is the question of treating patients developing rabies beyond palliative care. A small number of successes have been achieved due to development as well as application of therapeutic coma recently. It has been evident from experimentation that a strong innate immune response is produced in the mammalian host due to rabies infection in brain. However, there is strong indication that this immune response is strongly antagonized to certain level by the viral phosphoprotein. Failure of the innate as well as adaptive immune responses for the control of RABV infection is responsible for immunosubversive strategy of RABV. These results were promoted the treatment of rabies with modified RABV genome therapeutically appears to decrease the infection with a strain that is highly virulent. This provides the opportunity in future for successful treatment in order to mitigate the most horrible outcomes of rabies (McKimmie et al. Citation2005; Brzozka et al. Citation2006; Hooper et al. Citation2011). Shivasharanappa et al. (Citation2014) explored the therapeutic potential of TLR-3 against rabies. TLR-3 treatment resulted in delayed onset of clinical signs, more survival time, less histo-pathological lesions, and higher expression of TLR-3 and its associated cytokines.
Treatment with recombinant human interferon alpha (rhIFN α−2A) in rabies infected mice resulted in delayed mortality and significantly reduced expression of TNF-α and IL-6 both in the pre-exposure and post-exposure groups. These findings suggested that regulation of cytokine secretion using exogenous cytokines may offer novel therapeutic approaches for the treatment of rabies (Mehta et al. Citation2015). Recombinant RABVs expressing the NanoLuc luciferase (NanoLuc) system facilitates screening of small molecules for inhibition of RABV infection (Anindita et al. Citation2016). A rabies infected patient recovered from disease without administration of any PEP (Willoughby et al. Citation2005). The patient had neutralizing antibodies at the time of admitting to hospital and her therapeutic protocol for coma included midazolam and supplemental phenobarbital, and supportive treatment like ketamine, ribavirin and amantadine (Jackson Citation2016). Sajjanar et al. (Citation2016) reported that nicotinic acetylcholine receptor alpha 1 (nAChRα1) subunit peptides had specific affinity towards the RABV and these receptors may act as receptor decoy molecules results in inhibition of attachment of RABV to the neuronal cell receptors, which ultimately decrease viral replication. Torpedo peptide sequence (T-32) showed more antiviral action when compared to bovine, human and rat peptide sequences (Sajjanar et al. Citation2016).
15. Outbreak prevention and control
Countries like Saudi Arabia, Oman, Yemen, Israel, Iran and Turkey in the Middle East region are facing increasing problems due to wildlife rabies (Seimenis Citation2008). Bats act as wildlife reservoirs apart from carnivore species on which control activities are traditionally focussed (van der Poel et al. Citation2006; Rupprecht et al. Citation2008). Rabies causes almost 100% fatality in humans (Mazigo et al. Citation2010), and it is also 100% preventable (Tekki et al. Citation2013). At present, communication and coordination between veterinary and public health officials are limited, which further complicates the control of rabies in developing countries. The first and foremost part of rabies control programme is to define case by identification of the rabid animal after diagnosis by a qualified laboratory. Confirmation in the laboratory should be done either by dFAT or RABV isolation in cell culture. Rabies prevention and control programme require enhanced surveillance based on laboratory and variant typing. For this, accurate and timely information and reporting are necessary to determine the management of potentially exposed animals aiding in emerging pathogen discovery. There are always chances of introduction of virus via entry of infected animals (Castrodale et al. Citation2008). This helps in describing the disease epidemiology and to evaluate the requirement of an effective immunization programmes for wild and domestic animals. For evaluating the surveillance trends, all the animals presented for diagnosis of rabies should be recorded and reported along with implementation of electronic laboratory reporting and notification of rabies in animals. Point location along with history of vaccination, RABV variant (if rabid) and exposure to rabid animals are essential for providing optimal information on animals. In this regard, incorporation of standard public health reporting systems are also required (Blanton et al. Citation2009; Deb et al. Citation2012; Fisman Citation2014). Huge epidemiological data are necessary to decipher the accurate burden of rabies in humans and animals. More and dynamic partnership is required both inside the country, within the stae and outside the country for successful control and eradication of this devasting disease.
New RABV variants emergence or non-indigenous virus introduction enhances a risk to humans, domestic animals and wildlife significantly. A quick and complete response for successful control and eradication of the disease consist of following measures:
(1) | Characterization of the RABV at regional reference laboratory, national and international level. | ||||
(2) | Identification and control of the source of entry of virus. | ||||
(3) | Enhancement of laboratory-based surveillance in domestic and wild animals. | ||||
(4) | Increased rate of vaccination in animals against rabies. | ||||
(5) | Restriction of animal movement. | ||||
(6) | Control of vector population is required. | ||||
(7) | Coordination of a multi-agency response (Seneschall and Luna-Farro Citation2013). | ||||
(8) | Provision for public and professional outreach and education. |
Appropriate precautions should be taken at the time of handling suspicious or confirmed rabies cases because of the zoonotic importance. All veterinary personnel need to be adequately protected. Potential source of infection are tissues or materials from infected animals carrying virus like salivary glands, saliva and central NS (Weese Citation2002). There is great reduction in the possibility of disease transmission by following strict personal hygienic measures and wearing of barrier precautions while handling of body fluids, infected animals and post-mortem specimens. The appropriate authorities and all in-contact individuals should be taken care of as to determine whether PEP is required by observing the likelihood of rabies under veterinarian's notification (Beran Citation1993; CDC Citation1999; Weese Citation2002; Bender and Schulman Citation2004; Hankins and Rosekrans Citation2004; Leung et al. Citation2007; Dendle and Looke Citation2008). Awareness campaigns through mass media outlets and workshops (Dzikwi et al. Citation2012) should be done by emphasizing public health importance of rabies in association with dog slaughtering, trading, and consumption of dog meat. Transported dogs should have adequate vaccination history against rabies, which must be well documented.
A quantitative estimate of the burden of rabies has got value, which must not be under estimated. The perception that rabies is rather an insignificant human disease in developing countries hampers the initiatives of disease control (Zinsstag Citation2013). The first priority to decrease the burden of rabies in humans should be aimed at controlling rabies in canine, particularly in free-ranging dogs. The inadequate medical laboratory facilities in majority of the rabies endemic nations are major impediment for the diagnosis of rabies cases in human with encephalitis. Furthermore, the animal husbandry and wildlife departments are often unable to conduct the systematic surveillance and reporting of rabies in animals. Rabies will continue to remain a undetermined and present in everywhere, particularly in poverty-affected countries of the world, due to inadequate and inefficient risk assessment system that is currently used (Banyard et al. Citation2013). The elimination of canine rabies using ‘One Health’ strategy is required to generate continuous immunity among dog population, by applying standard immunization approaches at the rural level, along with humane population management and community education (Franka et al. Citation2013). Control of rabies is further seen as the responsibility of veterinary authorities. But if the public health importance along with the benefits of disease control (both in terms of disability-adjusted life year and reduction in cost of post-exposure treatment) are demonstrated, it will lead to encouragement of health sector to undertake initiatives for control. It is crucial to integrate the medical as well as veterinary sectors for controlling the disease effectively. This is evident from the rabies control programme in Central, South America, Latin America and the Caribbean, wherein there has been implementation of mass vaccination programmes in dogs (Cliquet and Aubert Citation2004; Coleman et al. Citation2004; Lembo et al. Citation2011; Shwiff et al. Citation2013; Vigilato et al. Citation2013).
16. Conclusion and future perspectives
Rabies can be excluded on the basis of the results of diagnostic tests or progress of the disease in most of the early instances. It is however of utmost importance to consider rabies at the very early stage along with precautionary measures. Moreover, there is a need to enhance the surveillance for RABV variants currently circulating in rabid animals particularly in wildlife. Exact prevalence of the rabies in wild animals is presently lacking, which should be explored to know the role of these animals in disease transmission. The continuous monitoring of less common non-reservoir species is also important for detecting newly introduced RABV variants. Strict barrier precautions are mandatory as the RABV as such is transmissible through contact of saliva with skin (broken) as well as mucous membrane. From the side of the veterinarians, it is important that they notify the appropriate authorities along with appropriate reporting of the disease though it can vary with jurisdiction. Medical and veterinary professionals must be aware about the route of rabies transmission to create the public awareness regarding precautionary measures while living in rural areas and also in persons working in abattoirs, which would further eliminate the chances of disease spread. Reporting to the physicians by all in-contact individuals and giving importance to PEP is essential. Advancement in the molecular diagnosis with the development of sequence specific RT-PCR technology has made the diagnosis very much target specific. Advanced molecular diagnostic techniques should be developed using recent biotechnological tools for early confirmatory diagnosis and differentiation of various genotypes of RABV. More researches should be focussed in the near future for unravelling the unknown aspects of pathology and pathogenesis of rabies, which would pave the way for developing suitable antiviral therapeutic strategies. Along with this development, the molecular biology has also made it possible to develop newer generation vaccines to control animal rabies and its spread to human population. Novel therapies including cytokine and siRNA therapies have also been found to be fruitful approaches. However, suitable vaccination along with monitoring for antibody titers every two years is necessary for persons with more likelihood of being getting exposed to rabies for ensuring adequate protection. Last but not the least, it is sad that children are at the greatest risk of contracting rabies from dog bites especially in developing world where endemic rabies occurs. So travellers moving to remote rural areas in particular should take decision on pre-exposure rabies vaccination. For this assessment of the risk of exposure to rabid dog bites along with local access to rabies immunoglobulins and vaccines is the need of hour in different endemic zones of the world. Though available oral/bait vaccines offer protection, they are not fully effective in all species of wild animals; the stability of vaccines in the field should be evaluated and post vaccination immune status should be assessed. Taking advantages of recombinant DNA technology, novel immune-prophylactic and environmentally stable agents like subunit vaccines, DNA vectored vaccines or DNA vaccines could be developed to nullify the drawbacks of conventional vaccines. Edible vaccine especially for domestic and wild ruminants should be developed to curtail the rabies. Many countries having the status of high-risk areas but most of the countries around the globe gained the status of rabies-free territories. This shows that rabies can be successfully ruled out from the high-risk areas by taking preventing measures. The advent of scientific medicine also makes rabies control possible. Public awareness in this regard can play major role. To accomplish these recommendations, high-level political commitment is also simultaneously essential.
Acknowledgments
The authors are highly thankful to the Director, ICAR-IVRI for strengthening facilities for rabies research at IVRI, Izatnagar.
Disclosure statement
The authors declare that they have no competing interests.
References
- Abelseth MK. 1964. An attenuated rabies vaccine for domestic animals produced in tissue culture. Can Vet J. 5:279–286.
- ACPA (Asia Canine Protection Alliance). 2013. Risk assessment-the risk the dog meat trade poses to rabies transmission and the ASEAN plus 3 countries’ pledge to eliminate rabies by 2020. 1–13. Available from: http://www.acpagroup.org/images/resources/Risk%20Assessment_TheIllegalTradeinDogsandRabiesTransmission_ACPA.pdf.
- Aghahowa SE, Ogbevoen RN. 2010. Incidence of dog bite and anti-rabies vaccine utilization in the, University of Benin Teaching Hospital, Benin City, Nigeria: a 12-year assessment. Vaccine. 28(30):4847–50.
- Ahmed K, Wimalaratne O, Dahal N, Khawplod P, Nanayakkara S, Rinzin K, Perera D, Karunanayake D, Matsumoto T, Nishizono A. 2012. Evaluation of a monoclonal antibody-based rapid immunochromatographic test for direct detection of rabies virus in the brain of humans and animals. Am J Trop Med Hyg. 86(4):736–740.
- Ajoke E, Solomon A, Ikhide E. 2014. The role of dog trading and slaughter for meat in rabies epidemiology with special reference to Nigeria – a review. J Exp Biol Agric Sci. 2(2):130–136.
- Albertini AA, Ruigrok RW, Blondel D. 2011. Rabies virus transcription and replication. Adv Virus Res. 79:1–22.
- Albertini AA, Schoehn G, Weissenhorn W, Ruigrok RW. 2008. Structural aspects of rabies virus replication. Cell Mol Life Sci. 65(2):282–294.
- Amann R, Rohde J, Wulle U, Conlee D, Raue R, Martinon O, Rziha HJ. 2013. A new rabies vaccine based on a recombinant Orf virus (Parapoxvirus) expressing the rabies virus glycoprotein. J Virol. 87(3):1618–1630.
- Anilionis A, Wunner WH, Curtis P. 1981. Structure of the glycoprotein gene of rabies virus. Nature (London). 294:275–278.
- Anindita PD, Sasaki M, Nobori H, Sato A, Carr M, Ito N, Sugiyama M, Orba Y, Sawa H. 2016. Generation of recombinant rabies viruses encoding NanoLuc luciferase for antiviral activity assays. Virus Res. 215:121–128.
- Arai YT. 2005. Epidemiology of rabies virus and other lyssaviruses. Nippon rinsho Jpn J Clin Med. 63:2167–2172.
- Arai YT, Kuzmin IV, Kameoka Y, Botvinkin AD. 2003. New lyssavirus genotype from the lesser mouse-eared bat (Myotis blythi), Kyrghyzstan. Emerg Infect Dis. 9(3):333–337.
- Arai YT, Yamada K, Kamecka Y, Horimoto T, Yamamoto K, Yabe S, Nakayama M, Tashiro M. 1997. Nucleoprotein gene analysis of fixed and street rabies virus variants using RT-PCR. Arch Virol. 142:1787–1796.
- AravindhBabu RP, Manoharan S, Ramadass P. 2014. Diagnostic evaluation of RT-PCR–ELISA for the detection of rabies virus. Indian J Virol. 25(1):120–124.
- Ares E, Burke M. 2015. Dog meat trade. House of Commons Library. Number 07360, 3 November 2015. Available from: http://researchbriefings.files.parliament.uk/documents/CBP-7360/CBP-7360.pdf.
- Artois M, Guittre C, Thomas I, Leblois H, Brochier B, Barrat J. 1992. Potential pathogenicity for rodents of vaccines intended for oral vaccination against rabies: a comparison. Vaccine. 10:524–528.
- Atuman YJ, Ogunkoya AB, Adawa DAY, Nok AJ, Biallah MB. 2014. Dog ecology, dog bites and rabies vaccination rates in Bauchi State, Nigeria. Int J Vet Sci Med. 2:41–45.
- Avieli N. 2011. Dog meat politics in a Vietnamese town. Ethnology. 50(1):59–78.
- Baba SS. 1999. Detection of rabies virus RNA and antigen in tissues from naturally infected Nigerian dogs: in situ hybridization and immunohistochemical studies. Revue - d, Elevage -et-de-Medecine - Vetenrinaire-des-Pays-Tropicaux. 52:85–91.
- Babu RA, Manoharan S, Ramadass P, Chandran NDJ. 2012. Evaluation of RT-PCR assay for routine laboratory diagnosis of Rabies in post mortem brain samples from different species of animals. Indian J Virol. 23(3):392–396.
- Badrane H, Tordo N. 2001. Host switching in lyssavirus history from the Chiroptera to the Carnivora orders. J Virol. 75:8096–8104.
- Baer GM, editor. 1991. The natural history of rabies. Boca Raton (FL): CRC Press.
- Baer GM, Abelseth MK, Debbie JG. 1971. Oral vaccination of foxes against rabies. Am J Epidemiol. 93:487–490.
- Baloul L, Lafon M. 2003. Apoptosis and rabies virus neuroinvasion. Biochem. 85:777–788.
- Banerjee AK. 1987. Transcription and replication of rhabdovirus. Microbiol Rev. 51:66–87.
- Banyard AC, Horton DL, Freuling C, Müller T, Fooks AR. 2013. Control and prevention of canine rabies: the need for building laboratory-based surveillance capacity. Antiviral Res. 98(3):357–364.
- Barrat J, Picard-Meyer E, Cliquet F. 2006. Rabies diagnosis. Dev Biol. 125:71–77.
- Bedeković T, Šimić I, Krešić N, Lojkić I, Mihaljević Ž, Sučec I, Janković IL, Hostnik P. 2016. Evaluation of ELISA for the detection of rabies virus antibodies from the thoracic liquid and muscle extract samples in the monitoring of fox oral vaccination campaigns. BMC Vet Res. 12:76.
- Bender J, Schulman, S. 2004. Reports of zoonotic disease outbreaks associated with animal exhibits and availability of recommendations for preventing zoonotic disease transmission from animals to people in such settings. J Am Vet Med Assoc. 224:1105–1109.
- Beran GW. 1993. Zoonoses in practice. Vet Clin North Am Small Anim Pract. 23:1085–1097.
- Black EM, Lowings JP, Smith J, Heaton PR, Mc Elhinney M. 2002. A rapid RT-PCR method to differentiate six established genotypes of rabies and rabies-related viruses using TaqMan technology. J Virol Methods. 105:25–35.
- Black EM, McElhinney LM, Lowings JP, Smith J, Johnstone P, Heaton PR. 2000. Molecular methods to distinguish between classical rabies and the rabies related European bat lyssa viruses. J Virol Methods. 87:123–131.
- Blackwood JC, Streicker DG, Altizer S, Rohani P. 2013. Resolving the roles of immunity, pathogenesis, and immigration for rabies persistence in vampire bats. Proc Natl Acad Sci USA. 110(51):20837–20842.
- Blancou J. 2003. History of surveillance and control of transmissible animal diseases. Paris: Office International des Epizootics; p. 193–219.
- Blancou J. 2008. The control of rabies in Eurasia: overview, history and background. Dev Biol (Basel). 131:3–15.
- Blanton JD, Kis Robertson MPH, Palmer D, Rupprecht CE. 2009. Rabies surveillance in the United States during 2008. J Am Vet Med Assoc. 235(6):676–689.
- Blanton JD, Palmer D, Christian KA, Rupprecht CE. 2008. Rabies surveillance in the United States during 2007. J Am Vet Med Assoc. 233:884–897.
- Blanton JD, Palmer D, Christian KA, Rupprecht CE. 2010. Rabies surveillance in the United States during 2009. J Am Vet Med Assoc. 237(6):646–657.
- Blenden DC, Bell JF, Tsao AT, Umoh JU. 1983. Immunofluorescent examination of the skin of rabies-infected animals as a means of early detection of rabies virus antigen. J Clin Microbiol. 18(3):631–636.
- Blenden DC, Creech W, Torres-Anjel MJ. 1986. Use of immunofluorescence examination to detect rabies virus antigen in the skin of humans with clinical encephalitis. J Infect Dis. 154(4):698–701.
- Both L, Banyard AC, van Dolleweerd C, Horton DL, Ma JK, Fooks AR. 2012. Passive immunity in the prevention of rabies. Lancet Infect Dis. 12(5):397–407.
- Bourhy H, Kissi B, Audry L, Smreczak M, Sadkowska-Todys M, Kulonen K, Tordo N, Zmudzinski JF, Holmes EC. 1999. Ecology and evolution of rabies virus in Europe. J Gen Virol. 80(10):2545–2557.
- Bourhy H, Reynes JM, Dunham EJ, Dacheux L, Larrous F, Huong VTQ, Xu G, Yan J, Miranda MEG, Holmes EC. 2008. The origin and phylogeography of dog rabies virus. J Gen Virol. 89:2673–2681.
- Bouzamondo E, Ladogana A, Tsiang H. 1993. Alteration of potassium-evoked 5-HT release from virus-infected rat cortical synaptosomes. Neuroreport. 4:555–558.
- Brochier B, Kieny MP, Costy F, Coppens P, Bauduin B, Lecocq JP, Languet B, Chappuis G, Desmetre P, Afiademanyo K, et al. 1991. Large-scale eradication of rabies using recombinant vaccinia rabies vaccine. Nature. 354:520–522.
- Bronnert J, Wilde H, Tepsumethanon V, Lumlertdacha B, Hemachudha T. 2007. Organ transplantations and rabies transmission. J Travel Med. 14:177–180.
- Brun A, Duret C, Devaux B, Calmels D. 1980. Simple, simultaneous or combined vaccinations of horses against equine influenza, rabies and tetanus (author's transl). Comp Immunol Microbiol Infect Dis. 3:93–99.
- Brzozka K, Finke S, Conzelmann KK. 2006. Inhibition of interferon signaling by rabies virus phosphoprotein P: activation-dependent binding of STAT1 and STAT2. J Virol. 80:2675–2683.
- Camelo S, Lafage M, Galelli A, Lafon M. 2001. Selective role for the p55 Kd TNF-alpha receptor in immune unresponsiveness induced by an acute viral encephalitis. J Neuroimmunol. 113:95–108.
- Campbell JB, Charlton KM, editors. 1988. Rabies. Boston (MA): Kluwer Academic Publications.
- Carrara P, Parola P, Brouqui P, Gautret P. 2013. Imported human rabies cases worldwide, 1990–2012. PLoS Negl Trop Dis. 7(5):e2209.
- Castrodale L, Walker V, Baldwin J, Hofmann J, Hanlon C. 2008. Rabies in a puppy imported from India to the USA, March 2007. Zoonoses Public Health. 55(8–10):427–430.
- CDC. 1999. Mass treatment of humans who drank unpasteurized milk from rabid cows. Morb Mort Wkly Rep. 48:228–230.
- CDC. 2004. Investigation of rabies infections in organ donor and transplant recipients – Alabama, Arkansas, Oklahoma, and Texas, 2004. Morb Mort Wkly Rep. 53:1–3.
- Ceccaldi PE, Fillion MP, Ermine A, Tsiang H, Fillion G. 1993. Rabies virus selectively alters 5-HT1 receptor subtypes in rat brain. Eur J Pharmacol. 245:129–138.
- Cherian S, Singh R, Singh KP, Manjunatha Reddy GB, Anjaneya , Ravi Kumar GV, Sumithra TG, Singh RP. 2015. Phylogenetic analysis of Indian rabies virus isolates targeting the complete glycoprotein gene. Infect Genet Evol. 36:333–338.
- Chhabra M, Bhardwaj M, Ichhpujani RL, Lal S. 2005. Comparative evaluation of commonly used laboratory tests for post-mortem diagnosis of rabies. Indian J Pathol Microbiol. 48(2):190–193.
- Chhabra M, Ichhpujani RL. 2003. Animal bites: the current management guidelines. Indian J Pediatr. 70(1):S11–S16.
- Chopy D, Detje CN, Lafage M, Kalinke U, Lafon M. 2011b. The type I interferon response bridles rabies virus infection and reduces pathogenicity. J Neurovirol. 17(4):353–367.
- Chopy D, Pothlichet J, Lafage M, Mégret F, Fiette L, Si-Tahar M, Lafon M. 2011a. Ambivalent role of the innate immune response in rabies virus pathogenesis. J Virol. 85(13):6657–6668.
- Cleaveland S, Hampson K, Kaare M. 2007. Living with rabies in Africa. Vet Rec. 161:293–294.
- Cleaveland S, Kaare M, Knobel D, Laurenson MK. 2006. Canine vaccination-providing broader benefits for disease control. Vet Microbiol. 117:43–50.
- Clifton M. 2003. Four shelter serving Beijing. Anim People. 12:18–19.
- Cliquet F, Aubert M. 2004. Elimination of terrestrial rabies in Western European countries. Dev Biol (Basel). 119:185–204.
- Cliquet F, Aubert M, Sagné L. 1998. Development of a fluorescent antibody virus neutralisation test (FAVN test) for the quantitation of rabies-neutralising antibody. J Immunol Methods. 212(1):79–87.
- Cliquet F, Guiot AL, Munier M, Bailly J, Rupprecht CE, Barrat J. 2006. Safety and efficacy of the oral rabies vaccine SAG2 in raccoon dogs. Vaccine. 24:4386–4892.
- Cliquet F, Gurbuxani JP, Pradhan HK, Pattnaik B, Patil SS, Regnault A, Begouen H, Guiot AL, Sood R, Mahl P, et al. 2007. The safety and efficacy of the oral rabies vaccine SAG2 in Indian stray dogs. Vaccine. 25:3409–3418.
- Coertse J, Weyer J, Nel LH, Merkotter W. 2010. Improved PCR methods for detection of African rabies and rabies-related lyssaviruses. J Clin Microbiol. 48(11):3949–3955.
- Coleman PG, Fevre EM, Cleaveland S. 2004. Estimating the public health impact of rabies. Emerg Infect Dis. 10(1):140–142.
- Condori-Condori RE, Streicker DG, Cabezas-Sanchez C, Velasco-Villa A. 2013. Enzootic and epizootic rabies associated with Vampire bats, Peru. Emerg Infect Dis. 19(9):1463–1469.
- Consales CA, Bolzan VL. 2007. Rabies review: immunopathology, clinical aspects and treatment. J Venom Anim Toxins incl Trop Dis. 13(1):5–38.
- Consales CA, Mendonea RZ, Gallina NMF, Pereira CA. 1990. Cytopathic effect induced by rabies virus in McCoy cells. J Virol Methods. 27:277–285.
- Constantine DG. 1962. Rabies transmission by non-bite route. Public Health Rep. 77:287–295.
- Conzelmann KK. 2005. Transcriptional activation of alpha/beta interferon genes: interference by nonsegmented negative-strand RNA viruses. J Virol. 79:5241–5248.
- Coulon P, Rollin PE, Flamand A. 1983. Molecular basis of rabies virus virulence. II. Identification of a site on the CVS glycoprotein associated with virulence. J Gen Virol. 64(3):693–696.
- Cox JH, Dietzschold B, Schneider LG. 1977. Rabies virus glycoproteins. II. Biological and serological characterization. Infect Immun. 16:754–759.
- da Rosa EST, Kotait I, Barbosa TFS, Carrieri ML, Brandão PE, Pinheiro AS, Begot AL, Wada MY, de Oliveira RC, Grisard EC, et al. 2006. Bat-transmitted human rabies outbreaks, Brazilian Amazon. Emerg Infect Dis. 12(8):1197–1202.
- Dacheux L, Delmas O, Bourhy H. 2012. Human rabies encephalitis prevention and treatment: progress since Pasteur's discovery. Biologicals. 40:251–299.
- Daffis S, Samuel MA, Suthar MS, Gale M Jr, Diamond MS. 2008. Toll-like receptor 3 has a protective role against West Nile virus infection. J Virol. 82(21):10349–10358.
- Dandale M, Singh CK, Ramneek V, Deka D, Bansal K, Sood NK. 2013. Sensitivity comparison of nested RT-PCR and TaqMan real time PCR for intravitam diagnosis of rabies in animals from urine samples. Vet World. 6(4):189–192.
- Dandale M, Singh CK, Ramneek V, Deka D, Sandhu BS, Bansal K, Sood NK. 2012. Ante mortem diagnosis of rabies from body secretion/excretion by TaqMan real time PCR. Int J Agro Vet Med Sci. 6(4):257–262.
- Dantas-Torres F. 2008. Bats and their role in human rabies epidemiology in the Americas. J Venom Anim Toxins incl Trop Dis. 14(2):193–202.
- David D. 2012. Role of the RT-PCR method in ante-mortem and post-mortem rabies diagnosis. Indian J Med Res. 135(6):809.
- David D, Yakobson B, Smith JS, Stram Y. 2000. Molecular epidemiology of rabies virus isolates from Israel and other middle and near eastern countries. J Clin Microbiol. 38:755–762.
- Davis A, Gordy P, Rudd R, Jarvis JA, Bowen RA. 2012. Naturally acquired rabies virus infections in wild-caught bats. Vector-Borne Zoonot Dis. 12(1):55–60.
- Davis AD, Jarvis JA, Pouliott CE, Shannon MD, Rudd RJ. 2013. Susceptibility and pathogenesis of little brown bats (Myotis lucifugus) to heterologous and homologous rabies viruses. J Virol. 87(16):9008–9015.
- Davis C, Neil S, Pushker R. 1997. Microwave fixation of rabies specimens for fluorescent antibody testing. J Virol Methods. 68:177–182.
- De Benedictis P, De Battisti C, Marciano S, Mutinelli F, Capua I, Cattoli G. 2013. Pyrosequencing of the rabies virus glycoprotein gene to demonstrate absence of vaccine-associated rabies cases following oral vaccination. Veterinary J. 195(3):388–390.
- De Benedictis P, Sow A, Fusaro A, Veggiato C, Talbi C, Kaboré A, Dundon WG, Bourhy H, Capua I. 2010. Phylogenetic analysis of rabies viruses from Burkina Faso, 2007. Zoonoses Public Health. 57(7–8):e42–e46.
- De Oviedo , Valdes F. 1950. Sumario de la Natural historia de las Indias, 1526. Mexico City: Fond de Cultura Economica. (Spanish)
- Deb R, Chakraborty S, Singh U, Kumar S, Sharma A. 2012. Infectious diseases of cattle. New Delhi: Satish Serial Publishing House; p. 224.
- Dendle C, Looke D. 2008. Review article: Animal bites: an update for management with a focus on infections. Emerg Med Australas. 20:458–467.
- Devleesschauwer B, Pruvot M, Joshi DD, De Craeye S, Jennes M, Ale A, Welinski A, Lama S, Aryal A, Victor B, et al. 2013. Seroprevalence of zoonotic parasites in pigs slaughtered in the Kathmandu Valley of Nepal. Vector Borne Zoonotic Dis. 13(12):872–876.
- Dhama K, Chakraborty S, Mahima , Wani, MY, Verma AK, Deb R, Tiwari R, Kapoor S. 2013. Novel and emerging therapies safeguarding health of humans and their companion animals: A review. Pak J Biol Sci. 16(3):101–111.
- Dhama K, Mahendran M, Gupta PK, Rai A. 2008. DNA Vaccines and their applications in veterinary practice: current perspectives. Vet Res Commun. 32:341–356.
- Dhingra V, Li X, Liu Y, Fu ZF. 2007. Proteomic profiling reveals that rabies virus infection results in differential expression of host proteins involved in ion homeostasis and synaptic physiology in the central nervous system. J Neurovirol. 13(2):107–111.
- Diaz AM, Papo S, Rodriguez A, Smith JS. 1994. Antigenic analysis of rabies-virus 281 isolates from Latin America and the Caribbean. Zentralbl Veterinarmed B. 41:153–160.
- Dietzschold B, Faber M, Schnell MJ. 2003. New approaches to the prevention and eradication of rabies. Expert Rev Vaccines. 2:399–406.
- Dietzschold B, Koprowski H. 2004. Rabies transmission from organ transplants in the USA. Lancet. 364:648–649.
- Dietzschold B, Koprowski H. 2005. Screening of organ and tissue donors for rabies. Lancet. 365:1305.
- Dietzschold B, Wunner WH, Wiktor TJ, Lopes AD, Lafon M, Smith CL, Koprowski H. 1983. Characterization of an antigenic determinant of the glycoprotein that correlates with pathogenicity of rabies virus. Proc Natl Acad Sci USA. 80:70–74.
- Dorfmeier CL, Lytle AG, Dunkel AL, Gatt A, McGettigan JP. 2012. Protective vaccine-induced CD4+ T cell-independent B cell responses against rabies infection. J Virol. 86(21):11533–11540.
- Dorfmeier CL, Shen S, Tzvetkov EP, McGettigan JP. 2013a. Reinvestigating the role of IgM in rabies virus postexposure vaccination. J Virol. 87(16):9217–9222.
- Dorfmeier CL, Tzvetkov EP, Gatt A, James P. McGettigan JP. 2013b. Investigating the role for IL-21 in rabies virus vaccine-induced Immunity. PLoS Negl Trop Dis. 7(3):e2129.
- Dupuis M, Brunt S, Appler K, Davis A, Rudd R. 2015. Comparison of automated quantitative reverse transcription-PCR and direct fluorescent-antibody detection for routine rabies diagnosis in the United States. J Clin Microbiol. 53(9):2983–2989.
- Dutta JK. 1998. Rabies transmission by oral and other non-bite routes. J Indian Med Assoc. 96:359.
- Dutta JK, Dutta TK. 1994. Rabies in endemic countries. Br Med J. 308:488–489.
- Dutta MK, Saha V, Sarkar P. 1992. Effect of experimentally inoculation of rabies fixed virus in mice using intra-cerebral and subcutaneous route. Indian J Anim Health. 31:15–20.
- Dwyer JP, Douglas TS, van As AB. 2007. Dog bite injuries in children-a review of data from a South African paediatric trauma unit. S Afr Med J. 97(8):597–600.
- Dyer JL, Niezgoda M, Orciari LA, Yager PA, Ellison JA, Rupprecht CE. 2013. Evaluation of an indirect rapid immunohistochemistry test for the differentiation of rabies virus variants. J Virol Methods. 190(1):29–33.
- Dzikwi AA, Ibrahim AS, Umoh JU. 2012. Knowledge, attitude and practice about rabies among children receiving formal and informal education in Samaru, Zaria, Nigeria. Glob J Health Sci. 4(5):132–139.
- Ekanem EE, Eyong KI, Philip-Ephraim EE, Eyong ME, Adams EB, Asindi AA. 2013. Stray dog trade fuelled by dog meat consumption as a risk factor for rabies infection in Calabar, southern Nigeria. Afr Health Sci. 13:1170–1173.
- Ellison JA, Johnson SR, Kuzmina N, Gilbert A, Carson WC, Vercauteren KC, Rupprecht CE. 2013. Multidisciplinary approach to epizootiology and pathogenesis of bat rabies viruses in the United States. Zoonoses Public Health. 60(1):46–57.
- Escobar LE, Peterson AT, Favi M, Yung V, Pons DJ, Medina-Vogel G. 2013. Ecology and geography of transmission of two bat-borne rabies lineages in Chile. PLoS Negl Trop Dis. 7(12):e2577.
- Esterhuysen JJ, Prehaud C, Thomson GR. 1995. A liquid phases blocking ELISA for the detection of antibodies to rabies virus. J Virol Methods. 51:31–42.
- Etessami R, Conzelmann KK, Fadai-Ghotbi B, Natelson B, Tsiang H, Ceccaldi PE. 2000. Spread and pathogenic characteristics of a G-deficient rabies virus recombinant: an in vitro and in vivo study. J Gen Virol. 81:2147–2153.
- Faber M, Faber ML, Papaneri A, Bette M, Weihe E, Dietzschold B, Schnell MJ. 2005. A single amino acid change in rabies virus glycoprotein increases virus spread and enhances virus pathogenicity. J Virol. 79:14141–14148.
- Faber M, Pulmanausahakul R, Hodawadekar SS, Spitsin S, McGettigan JP, Schnell MJ, Dietzschold B. 2002. Overexpression of the rabies virus glycoprotein results in enhancement of apoptosis and antiviral immune response. J Virol. 76:3374–3381.
- Faizee N, Hailat NQ, Ababneh MMK, Hananeh WM, Muhaidat A. 2012. Pathological, immunological and molecular diagnosis of rabies in clinically suspected animals of different species using four detection techniques in Jordan. Transbound Emerg Dis. 59(2):154–164.
- Fauquet CM, Mayo MA, Maniloff J, Desselberger U, Ball LA. 2004. Virus taxonomy: the classification and nomenclature of viruses. Eighth report of the International Committee on Taxonomy of Viruses. San Diego (CA): Academic Press; p. 623–631.
- Feder Jr HM, Petersen BW, Robertson KL, Rupprecht CE. 2012. Rabies: still a uniformly fatal disease? Historical occurrence, epidemiological trends, and paradigm shifts. Curr Infect Dis Rep. 14(4):408–422.
- Fekadu M, Endeshaw T, Wondimagegnehu A, Teshager T, Olson JG. 1992. Possible human-to-human transmission of rabies in Ethiopia. Ethiop Med J. 34:123–127.
- Finke S, Conzelmann KK. 2003. Dissociation of rabies virus matrix protein functions in regulation of viral RNA synthesis and virus assembly. J Virol. 77(22):12074–12082.
- Finke S, Cox JH, Conzelmann KK. 2000. Differential transcription attenuation of rabies virus genes by intergenic regions: generation of recombinant viruses overexpressing the polymerase gene. J Virol. 74(16):7261–7269.
- Fischer L, Minke J, Dufay N, Baudu Ph, Audonnet JC. 2003. Rabies DNA vaccine in the horse: strategies to improve serological responses. Vaccine. 21:4593–4596.
- Fisman DN. 2014. Taking the bite out of rabies, putting teeth into one health. Ann Intern Med. 160(2):132–133.
- Fitzpatrick MC, Hampson K, Cleaveland S, Meyers LA, Townsend JP, Galvani AP. 2012. Potential for rabies control through dog vaccination in wildlife-abundant communities of Tanzania. PLoS Negl Trop Dis. 6(8):e1796.
- Fooks AR. 2007. Rabies – the need for a ‘one medicine’ approach. Vet Rec. 161:289–290.
- Fooks AR, Johnson N, Freuling CM, Wakeley PR, Banyard AC, McElhinney LM, Marston DA, Dastjerdi A, Wright E, Weiss RA, et al. 2009. Emerging technologies for the detection of rabies virus: challenges and hopes in the 21st century. PLoS Negl Trop Dis. 3:e530.
- Fooks AR, McElhinney LM, Pounder DJ, Finnegan CJ, Mansfield K, Johnson N, Brookes SM, Parsons G, White K, McIntyre PG, et al. 2003. Case report: isolation of a European bat lyssavirus type 2a from a fatal human case of rabies encephalitis. J Med Virol. 71(2):281–289.
- Frana TS, Clough NE, Gatewood DM, Rupprecht CE. 2008. Postmarketing surveillance of rabies vaccines for dogs to evaluate safety and efficacy. J Am Vet Med Assoc. 232:1000–1002.
- Francis JR, Nourse C, Vaska VL, Calvert S, Northill JA, McCall B, Mattke AC. 2014. Australian bat lyssavirus in a child: the first reported case. Pediatrics. 133(4):e1063–e1067.
- Franka R, Smith TG, Dyer JL, Wu X, Niezgoda M, Rupprecht CE. 2013. Current and future tools for global canine rabies elimination. Antiviral Res. 100(1):220–225.
- Freuling CM, Beer M, Conraths FJ, Finke S, Hoffmann B, Keller B, Kliemt J, Mettenleiter TC, Mühlbach E, Teifke JP, et al. 2011. Novel lyssavirus in Natterer's bat, Germany. Emerg Infect Dis. 17(8):1519–1522.
- Fu ZF, Weihe E, Zheng YM, Schafer MK, Sheng H, Corisdeo S, Rauscher FJ, 3rd, Koprowski H, Dietzschold B. 1993. Differential effects of rabies and borna disease viruses on immediate-early- and late-response gene expression in brain tissues. J Virol. 67(11):6674–6681.
- Galelli A, Baloul L, Lafon M. 2000. Abortive rabies virus central nervous infection is controlled by T lymphocyte local recruitment and induction of apoptosis. J Neurovirol. 6(5):359–372.
- Ganesh V, Jayakumar R. 1999. Dot blot hybridization for diagnosis of rabies virus in animals. Indian J Virol. 15:77–79.
- Garba A, Dzikwi AA, Okewole PA, Chitunya-Wilson BB, Tirmidhi AB, Kazeem HM, Umoh JU. 2013. Evaluation of dog slaughter and consumption practices related to the control of rabies in Nigeria. J Exp Biol Agric Sci. 1:125–130.
- Gaudin Y, Tuffereau C, Segretain D, Knossow M, Flamand A. 1991. Reversible conformational changes and fusion activity of rabies virus glycoprotein. J Virol. 65:4853–4859.
- Gautret P, Parola P. 2012. Rabies pretravel vaccination. Curr Opin Infect Dis. 25(5):500–506.
- Gautret P, Parola P. 2013. Intradermal route for rabies vaccination should be generalized in travelers. Clin Infect Dis. 56(10):1508–1509.
- Gibbons RV. 2002. Cryptogenic rabies, bats, and the question of aerosol transmission. Ann Emerg Med. 39:528–536.
- Gigant B, Iseni F, Gaudin Y, Knossow M, Blondel D. 2000. Neither phosphorylation nor the amino-terminal part of rabies virus phosphoprotein is required for its oligomerization. J Gen Virol. 81(7):1757–1761.
- Gongal G, Wright AE. 2011. Human rabies in the WHO Southeast Asia Region: forward steps for elimination. Adv Prev Med. 2011:383870.
- Gongal G. 2005. Anti-rabies drive in Nepal. In: Proceedings of Medicine and Health in the Tropics, IVth European Congress on Tropical Medicine and International Health. Marseille; p. 45.
- Gould AR, Hyatt AD, Lunt R, Kattenbelt JA, Hengstberger S, Blackwell SD. 1998. Characterization of novel lyssa virus from Pteropid bats in Australia. Virus Res. 54:165–187.
- Green SL. 1993. Equine rabies. Vet Clin North Am Equine Pract. 9:337–347.
- Green SL. 1997. Rabies. Vet Clin North Am Equine Pract. 13:1–11.
- Greene CE, Rupprecht CE. 2006. Rabies and other lyssavirus infections. In: Greene CE, editor. Infectious diseases of the dog and cat. St Louis: Elsevier Saunders; p. 167–183.
- Gruzdev KN. 2008. The rabies situation in Central Asia. Dev Biol (Basel). 131:37–42.
- Gupta PK, Sonwane AA, Singh NK, Meshram CD, Dahiya SS, Pawar SS, Gupta SP, Chaturvedi VK, Saini M. 2012. Intracerebral delivery of small interfering RNAs (siRNAs) using adenoviral vector protects mice against lethal peripheral rabies challenge. Virus Res. 163(1):11–18.
- Hambolu SE, Dzikwi AA, Kwaga JKP, Kazeem HM, Umoh JU, Hambolu DA. 2013. Rabies and dog bites cases in Lagos State Nigeria: A prevalence and retrospective studies (2006-2011). Glob J Health Sci. 6:2014.
- Hamir AN, Moser G. 1994. Immunoperoxidase test for rabies: utility as diagnostic test. J Vet Diagn Invest. 6:148–152.
- Hampson K, Cleaveland S, Maganga S, Coudeville L. 2011. Reassessment of the socio-economic global burden of rabies: Human and animal cost of global rabies. In: Global Conference on Rabies Control: Towards Sustainable Prevention at Source: Incheon (Republic of Korea). 7–9 September 2011. Incheon: OIE; 26.
- Hampson K, Coudeville L, Lembo T, Sambo M, Kieffer A, Attlan M, Barrat J, Blanton JD, Briggs DJ, Cleaveland S, et al. 2015. Estimating the global burden of endemic canine rabies. PLoS Negl Trop Dis. 9(4):e0003709.
- Hankins DG, Rosekrans JA. 2004. Overview, prevention and treatment of rabies. Mayo Clin Proc. 79:671–676.
- Hanlon C, Childs J. 2013. Epidemiology. In: Jackson A, editor. Rabies scientific basis of the disease and its management. Oxford: Elsevier; p. 61–122.
- Hanlon CA, Niezgoda MN, Rupprecht CE. 2002. Postexposure prophylaxis for prevention of rabies in dogs. Am J Vet Res. 63:1096–1100.
- Hanna JN, Carney IK, Smith GA, Tannenberg AE, Deverill JE, Botha JA, Serafin IL, Harrower BJ, Fitzpatrick PF, Searle JW. 2000. Australian bat lyssavirus infection: a second human case, with a long incubation period. Med J Aust. 172(12):597–599.
- Hatz CF, Kuenzli E, Funk M. 2012. Rabies: relevance, prevention, and management in travel medicine. Infect Dis Clin North Am. 26(3):739–753.
- Haupt H, Rehaag B. 1921. Durch Fledermäuse verbreitete seuchenhafte Tolwut unter Viehbeständen in Santa Catharina (Süd-Brasilien) (German) Ztschr. F Infectionskr. 22:104–127.
- Heaton PR, McElhinney LM, Lowings JP. 1999. Detection and identification of rabies and rabies related viruses using rapid - cycle PCR. J Virol Methods. 81:63–69.
- Hellenbrand W, Meyer C, Rasch G, Steffens I, Ammon A. 2005. Cases of rabies in Germany following organ transplantation. Eurosurveillance Weekly Release. 10:050217.
- Helmick CG, Tauxe RV, Vernon AA. 1987. Is there a risk to contracts of patients with rabies? Rev Infect Dis. 9:511–518.
- Hemachudha T, Laothamatas J, Rupprecht CE. 2002. Human rabies: a disease of complex neuropathogenic mechanism and diagnostic challenges. Lancet Neurol. 1:101–109.
- Hemachudha T, Ugolini G, Wacharapluesadee S, Sungkarat W, Shuangshoti S, Laothamatas J. 2013. Human rabies: neuropathogenesis, diagnosis, and management. Lancet Neurol. 12(5):498–513.
- Hicks DJ, Fooks AR, Johnson N. 2012. Development in rabies vaccines. Clin Exp Immunol. 169:199–204.
- Hicks DJ, Nunez A, Healy DM, Brookes SM, Johnson N, Fooks AR. 2009. Comparative pathological study of the murine brain after experimental infection with classical rabies virus and European bat lyssaviruses. J Comp Pathol. 140(2–3):113–126.
- Hooper DC, Morimoto K, Bette M, Weihe M, Koprowski H, Dietshchold B. 1998. Collaboration of antibody and inflammation in the clearance of rabies virus from the CNS. J Virol. 72:3711–3719.
- Hooper DC, Roy A, Kean RB, Phares TW, Barkhouse DA. 2011. Therapeutic immune clearance of rabies virus from the CNS. Future Virol. 6(3):387–397.
- Hostnik P, Picard-Meyer E, Rihtaric D, Toplak I, Cliquet F. 2014. Vaccine-induced rabies in a red fox (Vulpes vulpes): isolation of vaccine virus in brain tissue and salivary glands. J Wildl Dis. 50:397–401.
- Ito N, Moseley GW, Blondel D, Shimizu K, Rowe CL, Ito Y, Masatani T, Nakagawa K, Jans DA, Sugiyama M. 2010. Role of interferon antagonist activity of rabies virus phosphoprotein in viral pathogenicity. J Virol. 84(13):6699–6710.
- Iwasaki Y, Gerhad W, Clark HF. 1997. Role of host immune response in the development of either encephalitic or paralytic disease after experimental rabies infection in mice. Infect Immun. 18:220–225.
- Iwasaki Y, Tobita M. 2002. Pathology. In: Rabies. Jackson AC, Wunner WH, editors. San Diego (CA): Academic Press; p. 283–306.
- Iwata M, Komori S, Unno T, Minamoto N, Ohashi H. 1999. Modification of membrane currents in mouse neuroblastoma cells following infection with rabies virus. Br J Pharmacol. 126:1691–1698.
- Jackson AC. 2000. Rabies in the critical care unit: diagnostic and therapeutic approaches. Can J Neurol Sci. 27:278–282.
- Jackson AC. 2002. Pathogenesis. In: Rabies. Jackson AC and Wunner WH, editors. San Diego (CA): Academic Press; p. 245–282.
- Jackson AC. 2003a. Neuronal apoptosis in experimental rabies: role of the route of viral entry. Neurology. 60:A102.
- Jackson AC. 2003b. Rabies virus infection: an update. J NeuroVirol. 9:253–258.
- Jackson AC. 2007a. Human disease. In: Jackson AC, Wunner WH, editors. Rabies. 2nd ed. London: Elsevier Academic Press; p. 309–340.
- Jackson AC. 2007b. Pathogenesis. In: Jackson AC, Wunner WH, editors. Rabies. 2nd ed. London: Elsevier Academic Press; p. 341–381.
- Jackson AC. 2008. Rabies. Neurol Clin. 26(3):717–26, ix.
- Jackson AC. 2010. Rabies pathogenesis update. Rev Pan-Amaz Saude. 1(1):167–172.
- Jackson AC. 2016. Human rabies: a 2016 Update. Curr Infect Dis Rep. 18(11):38.
- Jackson AC, Park H. 1998. Apoptotic cell death in experimental rabies in suckling mice. Acta Neuropathol. 95(2):159–164.
- Jackson AC, Randle E, Lawrance G, Rossiter JP. 2008. Neuronal apoptosis does not play an important role in human rabies encephalitis. J Neurovirol. 14(5):368–375.
- Jackson AC, Reimer DL. 1989. Pathogenesis of experimental rabies in mice: an immunohistochemical study. Acta Neuropathol. 78(2):159–165.
- Jackson AC, Rossiter JP. 1997. Apoptosis plays an important role in experimental rabies virus infection. J Virol. 71:5603–5607.
- Jackson AC, Wunner WH. 1991. Detection of rabies virus genomic RNA and mRNA in mouse and human brains by using in situ hybridization. J Virol. 65:2839–2844.
- Jacob Y, Badrane H, Ceccaldi PE, Tordo N. 2000. Cytoplasmic dynein LC8 interacts with lyssavirus phosphoprotein. J Virol. 74:10217–10222.
- Javadi M, Fayaz A, Mirdehghan SA, Ainollahi B. 1996. Transmission of rabies by corneal graft. Cornea. 15:431–433.
- Jayakumar R, Nachimuthu K, Padmanaban VD. 1995. A comparison between avidin-biotin peroxidase complex (ABC) and unlabelled antibody (PAP) procedures in rabies diagnosis. Indian Vet J. 71:866–869.
- Jayakumar R, Padmanaban VD, Jayakumar R. 1994. A dipstick dot ELISA immunoassay for detection of rabies antigen. Zentralblatt für Bakteriologie, Mikrobiologie und Hygiene. 280:382–385.
- Jayakumar R, Ramadas P. 1991. Evaluation of diagnostic test for rabies in dogs. Indian Vet J. 68:765–768.
- Jayakumar R, Ramadas P, Raghavan N. 1989. Comparison of enzyme immunodiagnosis with immunofluorescence for rapid diagnosis of rabies in dogs. Zentralblatt für Bakteriologie, Mikrobiologie und Hygiene. 271:501–503.
- Jindal N, Narang G. 1998. An outbreak of rabies in buffaloes in Haryana. Indian Vet J. 75:839–840.
- John TJ. 2005. What is appropriate post-exposure rabies prophylaxis? Indian Pediatr. 42(10):1062–1063.
- Joob S. 2013. Rabies vaccination in special scenarios: a review. Adv Trop Med Pub Health Int. 3(1):1–4.
- Joseph J, Sangeetha N, Khan AM, Rajoura OP. 2013. Determinants of delay in initiating post-exposure prophylaxis for rabies prevention among animal bite cases: Hospital based study. Vaccine. 32(1):74–77.
- Kang B, Oh J, Lee C, Park BK, Park Y, Hong K, Lee K, Cho B, Song D. 2007. Evaluation of a rapid immunodiagnostic test kit for rabies virus. J Virol Methods. 145:30–36.
- Karki S, Thakuri KC. 2010. Epidemiological situation of animal rabies and its control strategy in Nepal. In: Proceedings of the 9th Conference of Nepal Veterinary Association. Kathmandu; p. 105–110.
- Kasempimolporn S, Saengseesom W, Lumlertdacha B, Sitpriga V. 2000. Detection of rabies virus antigen in dog saliva using a latex agglutination test. J Clin Microbiol. 38:3098–3099.
- Katayama S, Yamanaka M, Ota S, Shimizu Y. 1999. A new quantitative method for rabies virus by detection of nucleoprotein in virion using ELISA. J Virol Methods. 77:69–74.
- Kelly RM, Strick PL. 2000. Rabies as a transneuronal tracer ofcircuits in the central nervous system. J Neurosci Methods. 103(1):63–71.
- Kieny MP, Lathe R, Drillien R, Spehner D, Skory S, Sschmitt D, Wiktor T, Koprowski H, Lecocq JP. 1984. Expression of rabies virus glycoprotein from a recombinant vaccinia virus. Nature. 312:163–166.
- Kim JH, Hwang EK, Sohn HJ, Kim DY, So BJ, Jean YH. 2005. Epidemiological characteristics of rabies in South Korea from 1993 to 2001. Vet Rec. 157:3–56.
- Kissi B, Tordo N, Bourhy H. 1995. Genetic polymorphism in the rabies virus nucleoprotein gene. Virology. 209:526–537.
- Klingen Y, Conzelmann KK, Finke S. 2008. Double-labeled rabies virus: live tracking of enveloped virus transport. J Virol. 82:237–245.
- Knobel DL, Cleaveland S, Coleman PG, Fevre EM, Meltzer MI, Miranda ME, Shaw A, Zinsstag J, Meslin FX. 2005. Reevaluating the burden of rabies in Africa and Asia. Bull World Health Organ. 83:360–368.
- Kobayashi Y, Okuda H, Nakamura K, Sato G, Itou T, Carvalho AA, Silva MV, Mota CS, Ito FH, Sakai T. 2007. Genetic analysis of phosphoprotein and matrix protein of rabies viruses isolated in Brazil. J Vet Med Sci. 69(11):1145–1154.
- Kojima D, Park CH, Satoh Y, Inoue S, Noguchi A, Oyamada T. 2009. Pathology of the spinal cord of C57BL/6J mice infected with rabies virus (CVS-11 strain). J Vet Med Sci. 71(3):319–324.
- Kojima D, Park CH, Tsujikawa S, Kohara K, Hatai H, Oyamada T, Noguchi A, Inoue S. 2010. Lesions of the central nervous system induced by intracerebral inoculation of BALB/c mice with rabies virus (CVS-11). J Vet Med Sci. 72(8):1011–1016.
- Kono H, Rock KL. 2008. How dying cells alert the immune system to danger. Nat Rev Immunol. 8:279–289.
- Kotwal S, Narayan KG, Deva AK. 1988. Peroxidase-antiperoxidase test in the diagnosis of rabies. Indian J Comp Microbiol Immunol Infect Dis. 9:154–155.
- Krebs JW, Mandel EJ, Swerdlow DL, Rupprecht CE. 2005. Rabies surveillance in the United States during 2004. J Am Vet Med Assoc. 227:1912–1925.
- Krebs JW, Wilson ML, Childs JE. 1995. Rabies- Epidemiology, prevention, and future research. J Mammal. 76(3):681–694.
- Kuang Y, Lackay SN, Zhao L, Fu ZF. 2009. Role of chemokines in the enhancement of BBB permeability and inflammatory infiltration after rabies virus infection. Virus Res. 144(1–2):18–26.
- Kucera P, Dolivo M, Coulon P, Flamand A. 1985. Pathways of the early propagation of virulent and avirulent rabies strains from the eye to the brain. J Virol. 55:158–162.
- Kulonen K, Fakadu M, Whitfield S, Warner CK. 1999. An evaluation of immunofluoresence and PCR. Methods for detection of rabies in archival Carnoy-fixed, paraffin-embedded brain tissue. J Vet Med Series B. 46(3):151–155.
- Kuzmin IV, Hughes GJ, Botvinkin AD, Orciari LA, Rupprecht CE. 2005. Phylogenetic relationships of Irkut and West Caucasian bat viruses within the Lyssavirus genus and suggested quantitative criteria based on the N gene sequence for lyssavirus genotype definition. Virus Res. 111:28–43.
- Kuzmin IV, Orciari LA, Arai YT, Smith JS, Hanlon CA, Kameoka Y, Rupprecht CE. 2003. Bat lyssaviruses (Aravan and Khujand) from Central Asia: phylogenetic relationships according to N, P and G gene sequences. Virus Res. 97(2):65–79.
- Kuzmin IV, Shi M, Orciari LA, Yager PA, Velasco-Villa A, Kuzmina N A, Streicker DG, Bergman DL, Rupprecht CE. 2012. Molecular inferences suggest multiple host shifts of rabies viruses from bats to mesocarnivores in Arizona during 2001–2009. PLoS Pathog. 8(6):e1002786.
- Kuzmina NA, Kuzmin IV, Ellison JA, Taylor ST, Bergman DL, Dew B, Rupprecht CE. 2013b. A reassessment of the evolutionary timescale of bat rabies viruses based upon glycoprotein gene sequences. Virus Genes. 47(2):305–310.
- Kuzmina NA, Lemey P, Kuzmin IV, Mayes BC, Ellison JA, Orciari LA, Hightower D, Taylor ST, Rupprecht CE. 2013a. The phylogeography and spatiotemporal spread of South-central skunk rabies virus. PloS One. 8(12):e82348.
- Lafay F, Benejean J, Tuffereau C, Flamand A, Coulon P. 1994. Vaccination against rabies: construction and characterization of SAG2, a double avirulent derivative of SADBern. Vaccine. 12:317–320.
- Lafon M. 2005. Modulation of the immune response in the nervous system by rabies virus. Curr Top Microbiol Immunol. 289:239–258.
- Lafon M. 2008. Immune evasion, a critical strategy for rabies virus. Dev Biol (Basel). 131:413–419.
- Lafon M. 2011. Evasive strategies in rabies virus infection. Adv Virus Res. 79:33–53.
- Lafon M, Megret F, Lafage M, Prehaud C. 2006. The innate immune facet of brain: human neurons express TLR-3 and sense viral dsRNA. J Mol Neurosci. 29(3):185–194.
- Lafon M, Megret F, Meuth SG, Simon O, Velandia Romero ML, Lafage M, Chen L, Alexopoulou L, Flavell RA, Prehaud C, et al. 2008. Detrimental contribution of the immuno-inhibitor b7-h1 to rabies virus encephalitis. J Immunol. 180(11):7506–7515.
- Lafon M, Prehaud C, Megret F, Lafage M, Mouillot G, Roa M, Moreau P, Rouas-Freiss N, Carosella ED. 2005. Modulation of HLA-G expression in human neural cells after neurotropic viral infections. J Virol. 79(24):15226–15237.
- Lahaye X, Vidy A, Fouquet B, Blondel D. 2012. Hsp70 protein positively regulates rabies virus infection. J Virol. 86:4743–4751.
- Lal S. 2014. Do people of Northeast India eat dogs? Is it true or just a hoax? Updated on 16 September 2014. Available from: https://www.quora.com/Do-people-of-Northeast-India-eat-dogs-Is-it-true-or-just-a-hoax
- Langoni H, Souza LC, Zetun CB, Silva TCC, Hoffmann JL, Silva RC. 2008. Serological survey for rabies in serum samples from vampire bats (Desmodus rotundus) in Botucatu Region, Sp., Brazil. J Venom Anim Toxins Trop Dis. 14:651–659.
- Laothamatas J, Sungkarat W, Hemachudha T. 2011. Neuroimaging in rabies. Adv Virus Res. 79:309–327.
- Larghi OP. 2004. Perspectives for rabies control and eradication from domestic species in developing countries. Dev Biol (Basel). 119:205–212.
- Larsan JK, Wunner WH, Etrl HCJ. 1992. Immune response to nominal phosphoproteins of rabies virus. Virus Res. 23:73–88.
- Larson JK, Wunner WH, Otvos L Jr, Ertl HC. 1991. Identification of an immunodominant epitope within the phosphoprotein of rabies virus that is recognized by both class I- and class II-restricted T cells. J Virol. 65(11):5673–5679.
- Last RD, Jardine JE, Smit MME, Lugt JJ, Vander Van-der-Lugt JJ. 1994. Application of immunoperoxidase techniques to formalin fixed brain tissue for the diagnosis of rabies in southern Africa. Onderstepoort J Vet Res. 61:183–187.
- Lau CL, Hohl, N. 2013. Immunogenicity of a modified intradermal pre-exposure rabies vaccination schedule using a purified chick embryo cell vaccine: an observational study. Travel Med Infect Dis. 11(6):427–430.
- Lawrence TM, Hudacek AW, de Zoete MR, Flavell RA, Schnell MJ. 2013. Rabies virus is recognized by the NLRP3 inflammasome and activates interleukin-1β release in murine dendritic cells. J Virol. 87(10):5848–5857.
- Leblois H, Flamand A. 1988. Studies on pathogenicity in mice of rabies virus strains used for oral vaccination of foxes in Europe. In: Pastoret PP, Brochier B, Thomas I, Blancou J, editors. Vaccination to control rabies in foxes. Brussels: Office for Official Publications of the European Communities; p. 101–104.
- Leblois H, Tuffereau C, Blancou J, Artois M, Aubert A, Flamand A. 1990. Oral immunization of foxes with avirulent rabies virus mutants. Vet Microbiol. 23:259–266.
- Lembo T, Attlan M, Bourhy H, Cleaveland S, Costa P, de Balogh K, Dodet B, Fooks AR, Hiby E, Leanes F, et al. 2011. Renewed global partnerships and redesigned roadmaps for rabies prevention and control. Vet Med Int. 2011:923149.
- Lembo T, Niezgoda M, Velasco-Villa A, Cleaveland S, Ernest E, Rupprecht CE. 2006. Evaluation of a direct, rapid immunohistochemical test for rabies diagnosis. Emerg Inf Dis. 12(2):310–313.
- Lentz TL, Burrage TG, Smith AL, Crick J, Tignor GH. 1982. Is the acetylcholine receptor a rabies virus receptor? Science. 215:182–184.
- Leonard JA, Wayne RK, Wheeler J, Valadez R, Guillen S, Vila C. 2002. Ancient DNA evidence for Old World origin of New World dogs. Science. 298:1613–1616.
- Leung AK, Davies HD, Hon KL. 2007. Rabies: Epidemiology, pathogenesis, and prophylaxis. Adv Ther. 24:1340–1347.
- Lewis P, Lentz TL. 1998. Rabies virus entry into cultured rat hippocampal neurons. J Neurocytol. 27:559–573.
- Liang HR, Hu GQ, Xue XH, Li L, Zheng XX, Gao YW, Yang ST, Xia XZ. 2014. Selection of an aptamer against rabies virus: A new class of molecules with antiviral activity. Virus Res. 184C:7–13.
- Liang HR, Liu Q, Zheng XX, Gai WW, Xue XH, Hu GQ, Wu HX, Wang HL, Yang ST, Xia XZ. 2013. Aptamers targeting rabies virus-infected cells inhibit viral replication both in vitro and in vivo. Virus Res. 173(2):398–403.
- Liao PH, Hsu YH, Yang HH, Wang MH, Chen LK. 2012. Involvement of extraneural tissues and upregulation of inducible nitric oxide synthase after experimental infection with rabies virus in BALB/c mice and LEW/SsN rats. Pathol Int. 62(9):619–627.
- Lina PH, Hutson AM. 2006. Bat rabies in Europe: a review. Dev Biol (Basel). 125:245–254.
- Liu J, Hamrouni A, Wolowiec D, Coiteux V, Kuliczkowski K, Hetuin D, Saudemont A, Quesnel B. 2007. Plasma cells from multiple myeloma patients express B7-H1 (PD-L1) and increase expression after stimulation with IFN-{gamma} and TLR ligands via a MyD88-, TRAF6-, and MEK-dependent pathway. Blood. 110(1):296–304.
- Liu X, Feng X, Tang Q, Wang Z, Qiu Z, Li Y, Wang C, Feng Z, Zhu J, Guan X. 2010. Characterization and potential diagnostic application of monoclonal antibodies specific to rabies virus. J Biomed Res. 24(5):395–403.
- Liu X, Yang Y, Sun Z, Chen J, Ai J, Dun C, Fu ZF, Niu X, Guo X. 2014. A recombinant rabies virus encoding two copies of the glycoprotein gene confers protection in dogs against a virulent challenge. PLoS One. 9(2):e87105.
- Liu Y, Zhang HP, Zhang SF, Wang JX, Zhou HN, Zhang F, Wang YM, Ma L, Li N, Hu RL. 2016. Rabies outbreaks and vaccination in domestic camels and cattle in Northwest China. PLoS Negl Trop Dis. 10(9):e0004890.
- Liu Y, Zhang S, Zhao J, Zhang F, Hu R. 2013. Isolation of Irkut virus from a Murina leucogaster bat in China. PLoS Negl Trop Dis. 7(3):e2097.
- Loza-Rubio E, Rojas-Anaya E, Lopez J, Olivera-Flores MT, Gomez-Lim M, Tapia-Perez G. 2012. Induction of a protective immune response to rabies virus in sheep after oral immunization with transgenic maize, expressing the rabies virus glycoprotein. Vaccine. 30:5551–5556.
- Lung O, Nadin-Davis S, Fisher M, Erickson A, Knowles MK, Furukawa-Stoffer T, Ambagala A. 2013. Microarray for identification of the chiropteran host species of rabies virus in Canada. Microarrays. 2(2):153–169.
- Lytle AG, Norton JE, Dorfmeier CL, Shen S, McGettigan JP. 2013. B cell infection and activation by rabies virus-based vaccines. J Virol. 87(16):9097–9110.
- Ma X, Niezgoda M, Blanton JD, Recuenco S, Rupprecht CE. 2012. Evaluation of a new serological technique for detecting rabies virus antibodies following vaccination. Vaccine. 30:5358–5362.
- Madhu BP, Singh KP, Saminathan M, Singh R, Shivasharanappa N, Sharma AK, Malik YS, Dhama K, Manjunatha V. 2016b. Role of nitric oxide in the regulation of immune responses during rabies virus infection in mice. Virus Dis. 27(4):387–399.
- Madhu BP, Singh KP, Saminathan M, Singh R, Tiwari AK, Manjunatha V, Harish C, Manjunathareddy GB. (2016a.). Correlation of inducible nitric oxide synthase (iNOS) inhibition with TNF-α, caspase-1, FasL and TLR-3 in pathogenesis of rabies in mouse model. Virus Genes. 52(1):61–70.
- Madhusudana SN, Malavalli BV, Thankappan UP, Sundramoorthy S, Belludi AY, Pulagumbaly SB, Sanyal S. 2014. Development and evaluation of a new immunohistochemistry-based test for the detection of rabies virus neutralizing antibodies. Hum Vaccin Immunother. 10(5):1359–1365.
- Madhusudana SN, Subha S, Thankappan U, Ashwin YB. 2012. Evaluation of a direct rapid immunohistochemical test (dRIT) for rapid diagnosis of rabies in animals and humans. Virol Sin. 27:299–302.
- Madhusudana SN, Sukumaran SM. 2008. Antemortem diagnosis and prevention of human rabies. Ann Indian Acad Neurol. 11(1):3–12.
- Mahardika GN, Dibia N, Budayanti NS, Susilawathi NM, Subrata K, Darwinata AE, Wignall FS, Richt JA, Valdivia-Granda WA, Sudewi AA. 2014. Phylogenetic analysis and victim contact tracing of rabies virus from humans and dogs in Bali, Indonesia. Epidemiol Infect. 142(6):1146–1154.
- Mähl P, Cliquet F, Guiot AL, Niin E, Fournials E, Saint-Jean N, Aubert M, Rupprecht CE, Gueguen S. 2014. Twenty year experience of the oral rabies vaccine SAG2 in wildlife: a global review. Vet Res. 45:77.
- Mani RS, Madhusudana SN. 2013. Laboratory diagnosis of human rabies: recent advances. Scientific World J. 2013(2013):10.
- Mani RS, Madhusudana SN, Mahadevan A, Reddy V, Belludi AY, Shankar SK. 2014. Utility of real-time Taqman PCR for antemortem and postmortem diagnosis of human rabies. J Med Virol. 86(10):1804–1812.
- Manjunathareddy GB, Sumana K, Yogisharadhya R, Cherian, S, Prajapati A, Patil SS, Balamurugan V, Singh KP, Singh R, Rahman H. 2016. Diagnosis of animal rabies: comparison of direct fluorescent antibody test (dFAT), reverse transcriptase-PCR and real-time PCR. J Exp Biol Agric Sci. 4(Spl-3-AD PCIAD):S69–S74.
- Mao C. 2010. Tribal Naga Dog meat delicacy. Demotix. Available from: http://www.demotix.com/news/302632/tribal-naga-dog-meatdelicacy. accessed on 17/10/2013.
- Marston DA, Horton DL, Ngeleja C, Hampson K, McElhinney LM, Banyard AC, Haydon D, Cleaveland S, Rupprecht CE, Bigambo M, et al. 2012. Ikoma lyssavirus, highly divergent novel lyssavirus in an African civet. Emerg Infect Dis. 18(4):664–667.
- Mazarakis ND, Azzouz M, Rohell JB. 2001. Rabies virus glycoprotein pseudotyping of lentiviral vectors enables retrograde axonal transport and access to the nervous system after peripheral delivery. Hum Mol Genet. 10:2109–2121.
- Mazigo HD, Okumu FO, Kweka EJ, Mnyone LL. 2010. Retrospective analysis of suspected rabies cases reported at bugando referral hospital, mwanza, Tanzania. J Global Infect Dis. 2(3):216–220.
- McCOLL KA, Tordo N, Aguilar-Setien AA. 2000. Bat lyssa virus infections. Rev Sci Technol. 19(1):177–196.
- Mccall BJ, Epstein JH, Neill AS, Heel K, Field H, Barrett J, Smith GA, Selvey LA, Rodwell B, Lunt R. 2000. Potential exposure to Australian bat lyssavirus, Queensland, 1996–1999. Emerg Infect Dis. 6:259–264.
- McElhinney LM, Marston DA, Brookes SM, Fooks AR. 2014. Effects of carcase decomposition on rabies virus infectivity and detection. J Virol Methods. 207:110–113.
- McGarvey PB, Hammond J, Dienelt MM, Hooper DC, Fu ZF, Dietzschold B, Koprowski H, Michaels FH. 1995. Expression of the rabies virus glycoprotein in transgenic tomatoes. Biotechnology (NY). 13:1484–1487.
- McGettigan JP, David F, Figueiredo MD, Minke J, Mebatsion T, Schnell MJ. 2014. Safety and serological response to a matrix gene-deleted rabies virus-based vaccine vector in dogs. Vaccine. 32(15):1716–1719.
- McKay N, Wallis I. 2005. Rabies: a review of UK management. Emerg Med J. 22:316–321.
- McKimmie CS, Johnson N, Fooks AR, Fazakerley JK. 2005. Viruses selectively upregulate Toll-like receptors in the central nervous system. Biochem Biophys Res Commun. 336:925–933.
- Mebatsion T, Weiland F, Conzelmann KK. 1999. Matrix protein of rabies virus is responsible for the assembly and budding of bullet-shaped particles and interacts with the transmembrane spike glycoprotein G. J Virol. 73:242–250.
- Megret F, Prehaud C, Lafage M, Moreau P, Rouas-Freiss N, Carosella ED, Lafon M. 2007. Modulation of HLA-G and HLA-E expression in human neuronal cells after rabies virus or herpes virus simplex type 1 infections. Hum Immunol. 68(4):294–302.
- Mehta S, Roy S, Mukherjee S, Yadav N, Patel N, Chowdhary A. 2015. Exogenous interferon prolongs survival of rabies infected mice. Virusdisease. 26(3):163–169.
- Meltzer MI, Rupprecht CE. 1998. Review of the economics of the prevention and control of rabies. Part 2: Rabies in dogs, livestock and wildlife. Pharmacoeconomics. 14:481–498.
- Ménager P, Roux P, Mégret F, Bourgeois JP, Le Sourd AM, Danckaert A, Lafage M, Préhaud C, Lafon M. 2009. Toll-like receptor 3 (TLR3) plays a major role in the formation of rabies virus Negri bodies. PLoS Pathog. 5(2):e1000315.
- Meng S, Lv W, Wu J, Wang J, Xu G, Yan J. 2013. Comparison of three strategies for sequencing rabies viral G gene. J Appl Virol. 2:1.
- Meslin FX. 2005. Rabies as a traveler's risk, especially in high-endemicity areas. J Travel Med. 12(1):S30–S40.
- Meuth SG, Simon OJ, Grimm A, Melzer N, Herrmann AM, Spitzer P, Landgraf P, Wiendl H. 2008. CNS inflammation and neuronal degeneration is aggravated by impaired CD200-CD200R-mediated macrophage silencing. J Neuroimmunol. 194(1–2):62–69.
- Mills D. 2013. Intradermal pre-exposure rabies vaccination. Promises and pitfalls. Travel Med Inf Dis. 11(6):335–336.
- Moeschler S, Locher S, Conzelmann KK, Krämer B, Zimmer G. 2016. Quantification of lyssavirus-neutralizing antibodies using vesicular stomatitis virus pseudotype particles. Viruses. 8(9): pii: E254.
- Monaco F, Franchi PM, Lelli R. 2006. Studies on an inactivated vaccine against rabies virus in domestic animals. Dev Biol (Basel). 125:233–239.
- Montaner AD, De Nichilo A, Rodriguez JM, Hernando-Insua A, Fló J, Lopez RA, Sierra V, Paolazzi C, Larghi O, Horn DL, et al. 2012. IMT504: A new and potent adjuvant for rabies vaccines permitting significant dose sparing. World J Vaccines. 2:182.
- Mori T, Morimoto K. 2014. Rabies virus glycoprotein variants display different patterns in rabies monosynaptic tracing. Front Neuroanat. 7:47.
- Morimoto K, Hooper DC, Spitsin S, Koprowski H, Dietzschold B. 1999. Pathogenicity ofdifferent rabiesvirus variants inversely correlates with apoptosis and rabies virus glycoprotein expression in infected primary neuron cultures. J Virol. 73:510–518.
- Morimoto K, Patel M, Corisdeo S, Hooper DC, Fu ZF, Rupprecht CE, Koprowski H, Dietzschold B. 1996. Characterization of a unique variant of bat rabies virus responsible for newly emerging human cases in North America. Proc Natl Acad Sci USA. 93(11):5653–5658.
- Mshelbwala PP, Ogunkoya AB, Maikai BV. 2013. Detection of rabies antigen in the saliva and brains of apparently healthy dogs slaughtered for human consumption and its public health implications in Abia State, Nigeria. ISRN Vet Sci. 2013:468043.
- Mudur G. 2005. Foreign visitors to India are unaware of rabies risk. Br Med J. 331:255.
- Muller TF, Schuster P, Vos AC, Selhorst T, Wenzel UD, Neubert AM. 2001. Effect of maternal immunity on the immune response to oral vaccination against rabies in young foxes. Am J Vet Res. 62:1154–1158.
- Muzzini E, Aparicio G. 2013. Urban growth and spatial transition in Nepal: an initial assessment. Washington (DC): World Bank Publications.
- Nadin D, Sheen M, Abdel Malik M, Elmgren L, Armstrong J, Wandler AI. 2000. A panel of monoclonal antibodies targeting the rabies virus phosphoprotein identifies a highly variable epitope of virus for sensitive strain discrimination. J Clin Microbiol. 38:1397–1403.
- Nadin-Davis SA, Abdel-Malik M, Armstrong J, Wandeler AI. 2002. Lyssavirus P gene characterization provides insights into the phylogeny of the genus and identifies structural similarities and diversity within the encoded phosphoprotein. Virology. 298:286–305.
- Nadin-Davis SA, Fehlner-Gardiner C. 2008. Lyssaviruses-current trends. Adv Virus Res. 71:207–250.
- Naze F, Suin V, Lamoral S, Francart A, Brochier B, Roels S, Mast J, Kalai M, Van Gucht S. 2013. Infectivity of rabies virus-exposed macrophages. Microb Infect. 15(2):115–125.
- Nel LH, Rupprecht CE. 2007. Emergence of lyssaviruses in the Old World: the case of Africa. Curr Top Microbiol Immunol. 315:161–193.
- Ngoepe CE, Sabeta C, Nel L. 2009. The spread of canine rabies into Free State province of South Africa: a molecular epidemiological characterization. Virus Res. 142(1–2):175–180.
- Nguyen AK, Nguyen DV, Ngo GC, Nguyen TT, Inoue S, Yamada A, Dinh XK, Nguyen DV, Phan TX, Pham BQ, et al. 2011. Molecular epidemiology of rabies virus in Vietnam (2006–2009). Jpn J Infect Dis. 64(5):391–396.
- Nigg AJ, Walker PL. 2009. Overview, prevention, and treatment of rabies. Pharmacotherapy. 29:1182–1195.
- Nishizono A, Yamada K, Khawplod P, Shiota S, Perera D, Matsumoto T, Wimalaratne O, Mitui MT, Ahmed K. 2012. Evaluation of an improved rapid neutralizing antibody detection test (RAPINA) for qualitative and semiquantitative detection of rabies neutralizing antibody in humans and dogs. Vaccine. 30(26):3891–3896.
- Niu X, Tang L, Tseggai T, Guo Y, Fu ZF. 2013. Wild-type rabies virus phosphoprotein is associated with viral sensitivity to type I interferon treatment. Arch Virol. 158(11):2297–2305.
- Nokireki T, Nevalainen M, Sihvonen L, Gadd T. 2015. Adverse reactions from consumption of oral rabies vaccine baits in dogs in Finland. Acta Vet Scand. 58(1):53.
- Norton JE Jr, Lytle AG, Shen S, Tzvetkov EP, Dorfmeier CL, McGettigan JP. 2014. ICAM-1-based rabies virus vaccine shows increased infection and activation of primary murine B cells in vitro and enhanced antibody titers in-vivo. PLos One. 9(1):e87098.
- Nyberg M, Kulonen K, Neuvonen E, Ek-Kommonen C, Nuorgam M, Westerling B. 1992. An epidemic of sylvatic rabies in Finland-descriptive epidemiology and results of oral vaccination. Acta Vet Scand. 33:43–57.
- Ohara S, Sato S, Oyama K, Tsutsui KI, Iijima T. 2013. Rabies virus vector transgene expression level and cytotoxicity improvement induced by deletion of glycoprotein gene. PloS One. 8(11):e80245.
- OIE 2009. Manual of diagnostic tests and vaccines for terrestrial animals 2009. Chapter 2. 1. 13. Rabies, 1:1–28.
- Otolorin GR, Aiyedun JO, Mshelbwala PP, Ameh VO, Dzikwi AA, Dipeolu MA, Danjuma FA. 2015. A review on human deaths associated with rabies in Nigeria. J Vaccines Vaccin. 6:262.
- Pandey P, Shlim DR, Cave W, Springer MFB. 2002. Risk of possible exposure to rabies among tourists and foreign residents in Nepal. J Travel Med. 9(3):127–131.
- Park CH, Kondo M, Inoue S, Noguchi A, Oyamada T, Yoshikawa H, Yamada A. 2006. The histopathogenesis of paralytic rabies in six-week-old C57BL/6J mice following inoculation of the CVS-11 strain into the right triceps surae muscle. J Vet Med Sci. 68(6):589–595.
- Parola P, Gautret P. 2012. Rabies vaccination in travelers: a global perspective. J Travel Med. 19(6):395–395.
- Pawaiya RVS, Dhama K, Kapoor S, Mahendran M. 2010. Equine rabies. In: Mathew T, editor. Advances in medical and veterinary virology, immunology, and epidemiology – Vol. 7: tropical viral diseases of large domestic animals – Part 1. Philadelphia (PA): Xlibris Corporation Publisher; p. 200–223.
- Pawan JL. 1936. The transmission of paralytic rabies in Trinidad by the Vampire bat (Desmodus rotundus murinus Wagner, 1840). Ann Trop Med Parasitol. 30:101–130.
- Paweska JT, Blumberg LH, Liebenberg C, Hewlett RH, Grobbelaar AA, Leman PA, Croft JE, Nel LH, Nutt L, Swanepoel R. 2006. Fatal human infection with rabies-related Duvenhage virus, South Africa. Emerg Infect Dis. 12(12):1965–1967.
- Peltier DC, Simms A, Farmer JR, Miller DJ. 2010. Human neuronal cells possess functional cytoplasmic and TLR-mediated innate immune pathways influenced by 3 kinase signaling. J Immunol. 184(12):7010–7021.
- Permpalung N, Wongrakpanich S, Korpaisarn S, Tanratana P, Angsanakul J. 2013. Trend of human rabies prophylaxis in developing countries: Toward optimal rabies immunization. Vaccine. 31(38):4079–4083.
- Phares TW, Fabis MJ, Brimer CM, Kean RB, Hooper DC. 2007. A peroxynitrite-dependent pathway is responsible for blood-brain barrier permeability changes during a central nervous system inflammatory response: TNF-alpha is neither necessary nor sufficient. J Immunol. 178(11):7334–7343.
- Picard-Meyer E, Bruyere V, Barrat J, Tissot E, Barrat MJ, Cliquet F. 2004. Development of a hemi-nested RT-PCR method for the specific determination of European bat lyssa virus 1 comparison with other rabies diagnostic methods. Vaccine. 22:1921–1929.
- Piccinotti S, Kirchhausen T, Whelan SP. 2013. Uptake of rabies virus into epithelial cells by clathrin-mediated endocytosis depends upon actin. J Virol. 87(21):11637–11647.
- Podberscek AL. 2009. Good to pet and eat: The keeping and consuming of dogs and cats in South Korea. J Soc Issues. 65:615–632.
- Pounder D. 2003. Bat rabies. BMJ. 326(7392):726.
- Prehaud C, Lay S, Dietzschold B, Lafon M. 2003. Glycoprotein of nonpathogenic rabies viruses is a key determinant of human cell apoptosis. J Virol. 77:10537–10547.
- Préhaud C, Lay S, Dietzschold B, Lafon M. 2003. Glycoprotein of nonpathogenic rabies viruses is a key determinant of human cell apoptosis. J Virol. 77(19):10537–10547.
- Prosniak M, Hooper DC, Dietzschold B, Koprowski H. 2001. Effect of rabies virus infection on gene expression in mouse brain. Proc Natl Acad Sci USA. 98(5):2758–2766.
- Pulko V, Liu X, Krco CJ, Harris KJ, Frigola X, Kwon ED, Dong H. 2009. TLR3- stimulated dendritic cells up-regulate B7-H1 expression and influence the magnitude of CD8 T cell responses to tumor vaccination. J Immunol. 183(6):3634–3641.
- Pulmanausahakul R, Li J, Schnell MJ, Dietzschold B. 2008. The glycoprotein and the matrix protein of rabies virus affect pathogenicity by regulating viral replication and facilitating cell-to-cell spread. J Virol. 82:2330–2338.
- Radostits OM, Gay CC, Blood DC, Hinchcliff KW. 2000. Rabies. In: Veterinary medicine. 9th ed. Philadelphia (PA): W.B. Saunders Co. Ltd.; p. 1201–1208.
- Raux H, Flamand A, Blondel D. 2000. Interaction of the rabies virus P protein with the LC8 dynein light chain. J Virol. 74:10212–10216.
- Recuenco S, Blanton JD, Rupprecht CE. 2012. A spatial model to forecast raccoon rabies emergence. Vector Borne Zoonotic Dis. 12(2):126–137.
- Reddy GB, Singh R, Singh RP, Singh KP, Gupta PK, Mahadevan A, Shankar SK, Ramakrishnan MA, Verma R. 2011. Molecular characterization of Indian rabies virus isolates by partial sequencing of nucleoprotein (N) and phosphoprotein (P) genes. Virus Genes. 43(1):13–17.
- Reetz J, Schilow WF, Pahlke C, Anduleit M. 1990. Immunohistochemical detection of rabies antigen. Monatshefte-fur-Veterinarmedizin. 45:85–87.
- Robardet E, Picard-Meyer E, Andrieu S, Servat A, Cliquet F. 2011. International interlaboratory trials on rabies diagnosis: an overview of results and variation in reference diagnosis techniques (fluorescent antibody test, rabies tissue culture infection test, mouse inoculation test) and molecular biology techniques. J Virol Methods. 177(1):15–25.
- Rojas-Anaya E, Loza-Rubio E, Olivera-Flores MT, Gomez-Lim M. 2009. Expression of rabies virus G protein in carrots (Daucus carota). Transgenic Res. 18:911–919.
- Rose VL. 1999. CDC issues revised guidelines for the prevention of humanrabies. Am Fam Physician. 59:2007–2013.
- Rossiter JP, Hsu L, Jackson AC. 2009. Selective vulnerability of dorsal root ganglia neurons in experimental rabies after peripheral inoculation of CVS-11 in adult mice. Acta Neuropathol. 118(2):249–259.
- Roy A, Hooper DC. 2007. Lethal silver-haired bat rabies virus infection can be prevented by opening the blood-brain barrier. J Virol. 81(15):7993–7998.
- Rudd RJ, Trimarchi CV. 1989. Development and evaluation of an in-vitro virus isolation procedure as a replacement for the mouse inoculation test in rabies diagnosis. J Clin Microbiol. 27:2522–2528.
- Rupert WH. 2002. China's taste for the exotic. BBC News. Available from: http://news.bbc.co.uk/2/hi/programmes/from_our_own_correspondent/2074073.stm, accessed on 17/10/2013.
- Rupprecht CE, Barrett J, Briggs D, Cliquet F, Fooks AR, Lumlertdacha B, Meslin FX, Muler T, Nel LH, Schneider C, et al. 2008. Can rabies be eradicated? Dev Biol (Basel). 131:95–121.
- Rupprecht CE, Gibbons RV. 2004. Clinical practice. Prophylaxis against rabies. N Engl J Med. 351(25):2626–2635.
- Rupprecht CE, Hanlon CA, Hemachudha T. 2002. Rabies re-examined. Lancet Infect Dis. 2:327–343.
- Rupprecht CE, Hanlon CA, Slate D. 2006. Control and prevention of rabies in animals: paradigm shifts. Dev Biol (Basel). 125:103–111.
- Rupprecht CE, Smith JS. 1994. Raccoon rabies: the re-emergence of an epizootic in a densely populated area. Semester Virol. 5:155.
- Rupprecht CE, Turmelle A, Kuzmin IV. 2011. A perspective on lyssavirus emergence and perpetuation. Curr Opin Virol. 1:662–670.
- Sacramento D, Bourhy H, Tordo N. 1991. PCR technique as an alternative method for diagnosis and molecular epidemiology of rabies virus. Mol Cell Probes. 5:229–240.
- Sajjanar B, Saxena S, Bisht D, Singh AK, Manjunatha Reddy GB, Singh R, Singh RP, Kumar S. 2016. Effect of nicotinic acetylcholine receptor alpha 1 (nAChRα1) peptides on rabies virus infection in neuronal cells. Neuropeptides. 57:59–64.
- Schneider LG, Cox JH. 1983. Ein Feldversuch zur oralen Immunisierung von Füchsen gegen die Tollwut in der Bundesrepublik Deutschland. I. Unschädlichkeit, Wirksamkeit und Stabilität der Vakzine SAD B19. Tierarztl Umsch. 38:315–324.
- Schneider MC, Santos-Burgoa C, Aron J, Munoz B, Ruiz-Velazco S, Uieda W. 1996. Potential force of infection of human rabies transmitted by vampire bats in the Amazonian region of Brazil. Am J Trop Med Hyg. 55:680–684.
- Schnell MJ, McGettigan JP, Wirblich C, Papaneri A. 2010. The cell biology of rabies virus: using stealth to reach the brain. Nature Rev Microbiol. 8:51–61.
- Schnell MJ, Mebatsion T, Conzelmann KK. 1994. Infectious rabies viruses from cloned cDNA. EMBO J. 13:4195–4203.
- Schreiner B, Mitsdoerffer M, Kieseier BC, Chen L, Hartung HP, Weller M, Wiendl H. 2004. Interferon-beta enhances monocyte and dendritic cell expression of B7-H1 (PD-L1), a strong inhibitor of autologous T-cell activation: relevance for the immune modulatory effect in multiple sclerosis. J Neuroimmunol. 155(1–2):172–182.
- Scott CA, Rossiter JP, Andrew RD, Jackson AC. 2008. Structural abnormalities in neurons are sufficient to explain the clinical disease and fatal outcome of experimental rabies in yellow fluorescent protein-expressing transgenic mice. J Virol. 82(1):513–521.
- Sehgal S, Bhatia R. 1985. Rabies: current status and proposed control programme in India. New Delhi: National Institute of Communicable Diseases.
- Seimenis A. 2008. The rabies situation in the Middle East. Dev Biol (Basel). 131:43–53.
- Senba K, Matsumoto T, Yamada K, Shiota S, Iha H, Date Y, Ohtsubo M, Nishizono A. 2013. Passive carriage of rabies virus by dendritic cells. SpringerPlus. 2(1):1–12.
- Seneschall C, Luna-Farro M. 2013. Controlling rabies through a multidisciplinary, public health system in Trujillo, La Libertad, Peru. Pathog Glob Health. 107(7):361–366.
- Servat A, Picard-Meyer E, Robardet E, Muzniece Z, Kylli Must K, Cliquet F. 2012. Evaluation of a rapid immunochromatographic diagnostic test for the detection of rabies from brain material of European mammals. Biologicals. 40:61–66.
- Shah MAA, Khan SU, Ali Z, Yang H, Liu K, Mao L. 2014. Applications of nanoparticles for DNA based rabies vaccine. J Nanosci Nanotechnol. 14(1):881–891.
- Shankar BP. 2009. Advances in diagnosis of rabies. Vet World. 2(2):74–77.
- Shashen’ ko AS, Buchukuri DV, Kovalev NA, Machitidze Ts Z. 1990. Enzyme treatment of brain samples fixed in formalin and glycerine in immunofluorescent diagnosis of rabies. Veterinarnaya-Nauka-Proizvodstvu. 28:34–36.
- Shepherd J. 2012. All about Indonesian dog meat. Buzz Feed Staff. Available from: http://www.buzzfeed.com/expresident/all-about-indonesian-dog-meat, Accessed on 17/04/2013.
- Shimatsu T, Shinozaki H, Kimitsuki K, Shiwa N, Manalo DL, Perez RC, Dilig JE, Yamada K, Boonsriroj H, Inoue S, et al. 2016. Localization of the rabies virus antigen in Merkel cells in the follicle-sinus complexes of muzzle skins of rabid dogs. J Virol Methods. 237:40–46.
- Shimazaki Y, Gamoh K, Senda M, Itoh O, Makie H. 2000. Characterization of monoclonal antibodies against rabies. Annual report of the National Veterinary Assay Laboratory. 37:13–16.
- Shivasharanappa N, Singh R, Singh KP, Singh RP, Madhu B. 2014. Rabies virus induces expression of TLR-3 and its associated cytokines in Swiss-albino mice. J Vet Sci Photon. 115:384–394.
- Shuangshoti S, Thepa N, Phukpattaranont P, Jittmittraphap A, Intarut N, Tepsumethanon V, Wacharapluesadee S, Thorner PS, Hemachudha T. 2013. Reduced viral burden in paralytic compared to furious canine rabies is associated with prominent inflammation at the brainstem level. BMC Vet Res. 9:31.
- Shultz S, Baral HS, Charman S, Cunningham AA, Das D, Ghalsasi GR, Goudar MS, Green RE, Jones A, Nighot P, et al. 2004. Diclofenac poisoning is widespread in declining vulture populations across the Indian subcontinent. Proc Biol Sci. 271(6):S458–S460.
- Shwiff S, Hampson K, Anderson A. 2013. Potential economic benefits of eliminating canine rabies. Antiviral Res. 98(2):352–356.
- Simmons FJ. 1994. Eat not this flesh: food avoidances from prehistory to present. 2nd ed. Madison (WI): University of Wisconsin Press; p. 229–240.
- Singh R, Shukla DC, Khanna PN, Singh KP, Mehrotra ML. 1995. An outbreak or rabies in cattle and buffaloes in Uttar Pradesh. Indian J Anim Sci. 65:166–168.
- Skrzynecki E, Januszewska M. 1996. Extinction of non-specific fluorescence in brain impression slides in animals suspected of rabies. Medycyna Weterynaryjna. 52:38–40.
- Smith JS. 1996. New aspects of rabies with emphasis on epidemiology, diagnosis, and prevention of the disease in the United State. Clin Microbiol Rev. 9:166–176.
- Smith JS, Mccelland CL, Reid FL, Baer GM. 1982. Dual role of the immune response in street rabies virus infection of mice. Infect Immun. 35:213–221.
- Smith SJ, Yager AP, Baer MG. 1996. Rapid fluorescent focus inhibition test for determining rabies virus neutralizing antibodies. In: Meslin FX, Kaplan MM, Koprowski H, editors. Laboratory techniques in rabies. 4th ed. Geneva: WHO; p. 181–191.
- Smith TG, Ellison JA, Ma X, Kuzmina N, Carson WC, Rupprecht CE. 2013. An electrochemiluminescence assay for analysis of rabies virus glycoprotein content in rabies vaccines. Vaccine. 31(33):3333–3338.
- Sommereyns C, Paul S, Staeheli P, Michiels T. 2008. IFN-lambda (IFN-lambda) is expressed in a tissue-dependent fashion and primarily acts on epithelial cells in vivo. PLoS Pathog. 4(3):e1000017.
- Song Y, Hou J, Qiao B, Li Y, Xu Y, Duan M, Guan Z, Zhang M, Sun L. 2013. Street rabies virus causes dendritic injury and F-actin depolymerization in the hippocampus. J Gen Virol. 94(2):276–283.
- Sonwane AA, Dahiya SS, Saini M, Chaturvedi VK, Singh RP, Gupta PK. 2012. Inhibition of rabies virus multiplication by siRNA delivered through adenoviral vector in vitro in BHK-21 cells in vivo in mice. Res Vet Sci. 93(1):498–503.
- Srinivasan A, Burton EC, Kuehnert MJ, Rupprecht C, Sutker WL, Ksiazek TG, Paddock CD, Guarner J, Shieh WJ, Goldsmith C, et al. 2005. Transmission of rabies virus from an organ donor to four transplant recipients. N Engl J Med. 352(11):1103–1111.
- Stading BR, Osorio JE, Velasco-Villa A, Smotherman M, Kingstad-Bakke B, Rocke TE. 2016. Infectivity of attenuated poxvirus vaccine vectors and immunogenicity of a raccoonpox vectored rabiesvaccine in the Brazilian free-tailed bat (Tadarida brasiliensis). Vaccine. 34(44):5352–5358.
- Stantic-Paylinic M. 2005. Public health concerns in bat rabies across Europe. Euro Surveill. 10(11):217–220.
- Steck F, Wandeler A, Bichsel P, Capt S, Hafliger U, Schneider L. 1982. Oral immunization of foxes against rabies. Laboratory and field studies. Comp Immunol Microbiol Infect Dis. 5:165–171.
- Steele JH, Fernandez PJ. 1991. History of rabies and global aspects. In: Baer GM editor. The Natural History of Rabies. 2nd edn. Boca Raton (FL): CRC Press; p. 1–26.
- Streicker DG, Altizer SM, Velasco-Villa A, Rupprecht CE. 2012. Variable evolutionary routes to host establishment across repeated rabies virus host shifts among bats. Proc Natl Acad Sci. 109(48):19715–19720.
- Streicker DG, Franka R, Jackson FR, Rupprecht CE. 2013. Anthropogenic roost switching and rabies virus dynamics in house-roosting big brown bats. Vector-Borne Zoonot Dis. 13(7):498–504.
- Sudarshan MK, Mahendra BJ, Madhusudana SN, Ashwath Narayana DH, Rahman A, Rao NSN, Meslin FX, Lobo D, Ravikumar K, Gangaboraiah . 2006a. An epidemiological study of animal bites in India: results of a WHO sponsored national multi-centric rabies survey. J Commun Dis. 38(1):32–39.
- Sudarshan MK, Mahendra BJ, Madhusudana SN, Rahman SA, Ashwathnarayana DH. 2006b. An assessment of rabies free status of the Island of Andaman, Nicobar and Lakshadweep: results of the WHO sponsored national multicentric rabies survey. Indian J Public Health. 50(1):11–14.
- Sugiyama M, Ito N. 2007. Control of rabies: Epidemiology of rabies in Asia and development of new-generation vaccines for rabies. Comp Immunol Microbiol Infect Dis. 30:273–286.
- Suin V, Nazé F, Francart A, Lamoral S, De Craeye S, Kalai M, Van Gucht S. 2014. A two-step lyssavirus real-time polymerase chain reaction using degenerate primers with superior sensitivity to the fluorescent antigen test. Biomed Res Int. 2014:256175.
- Suja MS, Mahadevan A, Madhusudana SN, Shankar SK. 2011. Role of apoptosis in rabies viral encephalitis: a comparative study in mice, canine, and human brain with a review of literature. Patholog Res Int. 10:374286.
- Sukathida U, Wanwarang H, Narisara A, Pongsak U. 2001. A radical form of nitric oxide suppresses RNA synthesis of rabies virus. Virus Res. 81:125–132.
- Suraweera W, Morris SK, Kumar R, Warrell DA, Warrell MJ, Jha P. 2012. Deaths from symptomatically identifiable furious rabies in India: A nationally representative mortality survey. PLoS Negl Trop Dis. 6(10):e1847.
- Takayama N. 2005. Clinical feature of human rabies. Nippon Rinsho. 63:2175–2179.
- Tan GS, Preuss MA, Williams JC, Schnell MJ. 2007. The dynein light chain 8 binding motif of rabies virus phosphoprotein promotes efficient viral transcription. Proc Natl Acad Sci USA. 104:7229–7234.
- Tang SC, Arumugam TV, Xu X, Cheng A, Mughal MR, Jo DG, Lathia JD, Siler DA, Chigurupati S, Ouyang X, et al. 2007. Pivotal role for neuronal Toll-like receptors in ischemic brain injury and functional deficits. Proc Natl Acad Sci USA. 104(34):13798–13803.
- Tang SC, Lathia JD, Selvaraj PK, Jo DG, Mughal MR, Cheng A, Siler DA, Markesbery WR, Arumugam TV, Mattson MP. 2008. Toll-like receptor- mediates neuronal apoptosis induced by amyloid beta-peptide and the membrane lipid peroxidation product 4-hydroxynonenal. Exp Neurol. 213(1):114–121.
- Tang X, Luo M, Zhang S, Fooks AR, Hu R, Tu C. 2005. Pivotal role of dogs in rabies transmission, China. Emerg Infect Dis. 11(12):1970–1972.
- Tao C, Li G. 2014. A rapid one-step immunochromatographic test strip for rabies detection using canine serum samples. Lett Appl Microbiol. 59(2):247–251.
- Tekki IS, Okewole PA, Ekong SP, Kumbish PR, Shamaki D, Ahmed MS. 2013. Control of rabies and rabies related viruses in Nigeria: Perspectives from the National Veterinary Research Institute, Vom, Nigeria. RIWA Journal. 1:102.
- Tenzin , Ward MP. 2012. Review of rabies epidemiology and control in South, South East and East Asia: past, present and prospects for elimination. Zoonoses Public Health. 59(7):451–467.
- Tepsumethanon V, Lumbertdacha B, Mitmoonpitak C, Veera T, Boonlert L, Channarong M. 1997. The sensitivity of fluorescent antibody testing on samples taken frombrain stem, cerebellum, cerebrum and hippocampus. Thai J Vet Med. 27:335–340.
- Thanomsridetchai N, Singhto N, Tepsumethanon V, Shuangshoti S, Wacharapluesadee S, Sinchaikul S, Chen ST, Hemachudha T, Thongboonkerd V. 2011. Comprehensive proteome analysis of hippocampus, brainstem, and spinal cord from paralytic and furious dogs naturally infected with rabies. J Proteome Res. 10:4911–4924.
- Thoulouze MI, Lafage M, Schachner M, Hartmann U, Cremer H, Lafon M. 1998. The neural cell adhesion molecule is a receptor for rabies virus. J Virol. 72:7181–7190.
- Thoulouze MI, Lafage M, Yuste VJ, Kroemer G, Susin SA, Israel N, Lafon M. 2003. Apoptosis inversely correlates with rabies virus neurotropism. Ann N Y Acad Sci. 1010:598–603.
- Ting JP, Willingham SB, Bergstralh DT. 2008. NLRs at the intersection of cell death and immunity. Nat Rev Immunol. 8:372–379.
- Tobiume M, Sato Y, Katano H, Nakajima N, Tanaka K, Noguchi A, Inoue S, Hasegawa H, Iwasa Y, Tanaka J, et al. 2009. Rabies virus dissemination in neural tissues of autopsy cases due to rabies imported into Japan from the Philippines: Immunohistochemistry. Pathol Int. 59(8):555–566.
- Tordo N, Poch O, Ermine A, Keith G. 1986. Primary structure of leader RNA and nucleoprotein genes of the rabies virus genome: segmented homology with VSV. Nucleic Acids Res. 14:2671–2683.
- Tsiang H, Porte S, Ambroise DJ, Derer M, Koenig J. 1986. Infection of cultured rat myotubes and neurons from the spinal cord by rabies virus. J Neuropathol Exp Neurol. 45:28–42.
- Tsunoda I, Tanaka T, Saijoh Y, Fujinami RS. 2007. Targeting inflammatory demyelinating lesions to sites of Wallerian degeneration. Am J Pathol. 171(5):1563–1575.
- Tuffereau C, Benejean J, Blondel D, Kieffer B, Flamand A. 1998. Low affinity nerve-growth factor receptor (P75NTR) can serve as a receptor for rabies virus. EMBO J. 17:7250–7259.
- Tuffereau C, Schmidt K, Langevin C, Lafay F, Dechant G, Koltzenburg M. 2007. The glycoprotein receptor p75NTR is not essential for rabies virus infection. J Virol. 81:13622–13630.
- Turki I, Hammami A, Kharmachi H, Mohamed Mousli M. 2014. Engineering of a recombinant trivalent single-chain variable fragment antibody directed against rabies virus glycoprotein G with improved neutralizing potency. Mole Immunol. 57:66–73.
- Ubol S, Sukwattanapan C, Maneerat Y. 2001. Inducible nitric oxide synthase inhibition delays death of rabies virus-infected mice. J Med Microbiol. 50:238–242.
- Udow SJ, Marrie RA, Jackson AC. 2013. Clinical features of dog- and bat-acquired rabies in humans. Clin Infect Dis. 57(5):689–696.
- Ugolini G. 1995. Specificity of rabies virus as a transneuronal tracer of motor networks: transfer from hypoglossal motoneurons to connected second-order and higher order central nervous system cell groups. J Comp Neurol. 356(3):457–480.
- Ugolini G. 2010. Advances in viral transneuronal tracing. J Neurosci Methods. 194(1):2–20.
- Uieda W, Harmani NM, Silva MM. 1995. Rabies in insectivorous bats (Molossidae) of Southeastern Brazil. Rev Saúde Publ. 29:393–397.
- Ullas P, Desai A, Madhusudana S. 2012. Rabies DNA vaccines: current status and future. World J Vaccines. 2:36–45.
- Van der Poel WH, Lina PH, Kramps JA. 2006. Public health awareness of emerging zoonotic viruses of bats: a European perspective. Vector Borne Zoonot Dis. 6:315–324.
- van Dolleweerd CJ, Teh AY, Banyard AC, Both L, Lotter-Stark HC, Tsekoa T, Phahladira B, Shumba W, Sabeta CT, Gruber C, et al. 2014. Engineering, expression in transgenic plants and characterisation of E559, a rabies virus-neutralising monoclonal antibody. J Infect Dis. 210(2):200–208.
- Vanrompay D, Harkinezhad T, Van de Walle M, Beeckman D, Van Droogenbroeck C, Verminnen K, Leten R, Martel A, Cauwerts K. 2007. Chlamydophila psittaci infections from pet birds to humans. Emerg Infect Dis. 13:1108–1110.
- Velasco-Villa A, Gomez-Sierra M, Hernandez-Rodriguez G, Juarez-Islas V, Melendez-Felix A, Vargas-Pino F, Velázquez-Monroy O, Flisser A. 2002. Antigenic diversity and distribution of rabies virus in Mexico. J Clin Microbiol. 40:951–958.
- Venugopal AK, Ghantasala SS, Selvan LD, Mahadevan A, Renuse S, Kumar P, Pawar H, Sahasrabhuddhe NA, Suja MS, Ramachandra YL, et al. 2013. Quantitative proteomics for identifying biomarkers for Rabies. Clin Proteomics. 10:3.
- Verginelli F, Capelli C, Coia V, Musiani M, Falchetti M, Ottini L, Palmirotta R, Tagliacozzo A, De Grossi Mazzorin I, Mariani- Costantini R. 2005. Mitochondrial DNA from prehistoric canids highlights relationships between dogs and South-East European wolves. Mol Biol Evol. 22:2541–2551.
- Verma R, Khanna P, Prinja S, Rajput M. 2011. Intra-dermal administration of rabies vaccines in developing countries: at an affordable cost. Human Vaccine. 7:792–794.
- Vigilato MA, Clavijo A, Knobl T, Silva HM, Cosivi O, Schneider MC, Leanes LF, Belotto AJ, Espinal MA. 2013. Progress towards eliminating canine rabies: policies and perspectives from Latin America and the Caribbean. Philos Trans R Soc Lond B Biol Sci. 368(1623):20120143.
- Vos A, Neubert A, Aylan O, Schuster P, Pommerening E, Muller T, Chivatsi DC. 1999. An update on safety studies of SAD B19 rabies virus vaccine in target and non-target species. Epidemiol Infect. 123:165–175.
- Wacharapluesadee S, Phumesin P, Supavonwong P, Khawplod P, Intarut N, Hemachudha T. 2011. Comparative detection of rabies RNA by NASBA, real-time PCR and conventional PCR. J Virol Methods. 175(2):278–282.
- Wang L, Cao Y, Tang Q, Liang G. 2013. Role of the blood-brain barrier in rabies virus infection and protection. Protein Cell. 4(12):901–903.
- Wang ZW, Sarmento L, Wang Y, Li X, Dhingra V, Tseggai T, Jiang B, Fu ZF. 2005. Attenuated rabies virus activates, while pathogenic rabies virus evades, the host innate immune responses in the central nervous system. J Virol. 79:12554–12565.
- Warner CK, Whitfield SG, Fecadu M, Ho H. 1997. Procedure for reproducible detection of rabies virus antigen mRNA and genome in situ in formaline-fixed tissues. J Virol Methods. 67:5–12.
- Warner CK, Zaki SR, Shieh W-J, Whitfield SG, Smith JS, Orciari LA, Shaddock JH, Niezgoda M, Wright CW, Goldsmith CS, et al. 1999. Laboratory investigation of human deaths from vampire bat rabies in Peru. Am J Trop Med Hyg. 60:502–507.
- Warrell MJ, Warrell DA. 2004. Rabies and other lyssavirus diseases. Lancet. 363:959–969.
- Wasniewski M, Almeida I, Baur A, Bedekovic T, Boncea D, Chaves LB, David D, De Benedictis P, Dobrostana M, Giraud P, et al. 2016. First international collaborative study to evaluate rabies antibody detection method for use in monitoring the effectiveness of oral vaccination programmes in fox and raccoon dog in Europe. J Virol Methods. 238:77–85.
- Wasniewski M, Cliquet F. 2012. Evaluation of ELISA for detection of rabies antibodies in domestic carnivores. J Virol Methods. 179(1):166–175.
- Wasniewski M, Guiot AL, Schereffer JL, Tribout L, Mähar K, Cliquet F. 2013. Evaluation of an ELISA to detect rabies antibodies in orally vaccinated foxes and raccoon dogs sampled in the field. J Virol Methods. 187(2):264–270.
- Wasniewski M, Labbe A, Tribout L, Rieder J, Labadie A, Schereffer JL, Cliquet F. 2014. Evaluation of a rabies ELISA as an alternative method to sero-neutralization tests in the context of international trade of domestic carnivores. J Virol Methods. 195:211–220.
- Watanabe I, Yamada K, Aso A, Suda O, Matsumoto T, Yahiro T, Ahmed K, Nishizono A. 2012. Relationship between virus-neutralizing antibody levels and the number of rabies vaccinations: a prospective study of dogs in Japan. Jpn J Infect Dis. 66(1):17–21.
- Weant KA, Baker SN. 2013. Review of human rabies prophylaxis and treatment. Crit Care Nurs Clin North Am. 25(2):225–242.
- Weese JS. 2002. A review of equine zoonotic diseases: risks in veterinary medicine. Proc Annual Convention AAEP. 48:362–369.
- Weihe E, Bette M, Preuss MA, Faber M, Schafer MK, Rehnelt J, Schnell MJ, Dietzschold B. 2008. Role of virus-induced neuropeptides in the brain in the pathogenesis of rabies. Dev Biol (Basel). 131:73–81.
- Whitt MA, Buonocore L, Prehaud C, Rose JK. 1991. Membrane fusion activity, oligomerization, and assembly of the rabies virus glycoprotein. Virology. 185(2):681–688.
- WHO 1998. Regional strategy for elimination of rabies. Report of an informal consultation. New Delhi: World Health Organization, Regional Office for South-East Asia; p. 34.
- WHO. 2002. Rabies vaccines. Wkly Epidemiol Rec. 77:109–119.
- WHO 2005. World Health Organization Expert Committee on Rabies, First report. 2005. WHO Technical Report Series, 931. Geneva: WHO; p. 1–87.
- WHO. 2013. Expert consultation on rabies. Second report. WHO Tech. Rep. Ser., 982, p. 1–139.
- WHO. 2016. Rabies Bulletin Europe. Available from: http://www.who-rabies-bulletin.org [cited 2 November 2016].
- Wilde H, Hemachudha T, Wacharapluesadee S, Lumlertdacha B, Tepsumethanon V. 2013. Rabies in Asia: the classical zoonosis. In: One health: the human-animal-environment interfaces in emerging infectious diseases. Berlin: Springer; p. 185–203.
- Wilkins PA, Piero FD. 2007. Rabies. In: Sellon, DC and Long, MT, editors. Equine infectious diseases. Philadelphia (PA): Saunders, Elsevier Inc; Chapter 19, p. 185–191.
- Willoughby Jr RE, Tieves KS, Hoffman GM, Ghanayem NS, Amlie-Lefond CM, Schwabe MJ, et al. 2005. Survival after treatment of rabies with induction of coma. N Engl J Med. 352:2508–2514.
- Winkler WG, Bogel K. 1992. Control of rabies in wildlife. Sci Am. 266:86.
- Winkler WG, Fashinell TR, Leffingwell L, Howard P, Conomy JP. 1973. Airborne rabies transmission in a laboratory worker. J Am Med Assoc. 226:1219–1221.
- Wirblich C, Schnell MJ. 2011. Rabies virus (RV) glycoprotein expression levels are not critical for pathogenicity of RV. J Virol. 85(2):697–704.
- Woldehiwet Z. 2005. Clinical laboratory advances in the detection of rabies virus. Clinica Chimica Acta. 351:49–63.
- Wu X, Gong X, Foley HD, Schnell MJ, Fu ZF. 2002. Both viral transcription and replication are reduced when the rabies virus nucleoprotein is not phosphorylated. J Virol. 76(9):4153–4161.
- Wu X, Smith TG, Franka R, Wang M, Carson WC, Rupprecht CE. 2014. The feasibility of rabies virus-vectored immunocontraception in a mouse model. Trials Vaccinol. 3:11–18.
- Wunner WH. 1991. The chemical composition and molecular structure of rabies virus. In: Baer GM, editor. The natural history of rabies. Boca Raton (FL): CRC Press; p. 31–37.
- www.thehorse.com. 2008. Intervet's Equirab rabies vaccine: New equine rabies vaccine introduced: Edited Press Release July 10 2008, Article # 12253. Available from: http://www.thehorse.com/ViewArticle.aspx?ID=12253.
- Wyatt J. 2007. Rabies-Update on a global disease. Pediatr Infect Dis J. 26(4):351–352.
- Xiang Z, Ertl HCJ. 1995. Manipulation of the immune response to a plasmid-encoded viral antigen by co inoculation with plasmids expressing cytokines. Immunity. 2(2):129–135.
- Xiang ZQ, Gao GP, Reyes-Sandoval A, Li Y, Wilson JM, Ertl HC. 2003. Oral vaccination of mice with adenoviral vectors is not impaired by preexisting immunity to the vaccine carrier. J Virol. 77:10780–10789.
- Xiang ZQ, Greenberg L, Ertl HC, Rupprecht CE. 2014. Protection of non-human primates against rabies with an adenovirus recombinant vaccine. Virology. 450-451:243–249.
- Xu G. 1998. Rapid detection of rabies street virus antigen. Chinese J Zoonoses. 14:50–52.
- Xu G, Weber P, Hu Q, Xue H, Audry L, Li C, Wu J, Bourhy H. 2007. A simple sandwich ELISA (WELYSSA) for the detection of lyssavirus nucleocapsid in rabies suspected specimens using mouse monoclonal antibodies. Biol. 35(4):297–302.
- Xue XH, Zheng XX, Wang HL, Ma JZ, Li L, Gai WW, Wang TC, Yang ST, Xia XZ. 2014. An inactivated recombinant rabies CVS-11 virus expressing two copies of the glycoprotein elicits a higher level of neutralizing antibodies and provides better protection in mice. Virus Genes. 48(3):411–420.
- Yamada K, Noguchi K, Nishizono A. 2014. Efficient N-glycosylation at position 37, but not at position 146, in the street rabies virus glycoprotein reduces pathogenicity. Virus Res. 179:169–176.
- Yamaoka S, Ito N, Ohka S, Kaneda S, Nakamura H, Agari T, Masatani T, Nakagawa K, Okada K, Okadera K, et al. 2013. Involvement of the rabies virus phosphoprotein gene in neuroinvasiveness. J Virol. 87(22):12327–12338.
- Yang DK, Kim HH, Lee KW, Song JY. 2013. The present and future of rabies vaccine in animals. Clin Exp Vaccine Res. 2:19–25.
- Yang J, Hooper DC, Wunner WH, Koprowski H, Dietzschold B, Fu ZF. 1998. The specificity of rabies virus RNA encapsidation by nucleoprotein. Virology. 242:107–117.
- Yousaf MZ, Qasim M, Zia S, Khan Mu, Ashfaq UA, Khan S. 2012. Rabies molecular virology, diagnosis, prevention and treatment. Virol J. 9:50.
- Yu F, Zhang G, Zhong X, Han N, Song Y, Zhao L, Cui M, Rayner S, Fu ZF. 2014. Comparison of complete genome sequences of dog rabies viruses isolated from China and Mexico reveals key amino acid changes that may be associated with virus replication and virulence. Arch Virol. 159(7):1593–601.
- Yusibov V, Hooper DC, Stitsin SV, Fleysh N, Kean RB, Mikheeva T, Deka D, Karasev A, Cox S, Randall J, et al. 2002. Expression in plants and immunogenicity of plant virus-based experimental rabies vaccine. Vaccine. 20:3155–3164.
- Zavadova J, Svrcek S. 1994. Improvement in the laboratory diagnosis of rabies and the titration of rabies virus. Vet Med. 39:663–676.
- Zhang G, Wang H, Mahmood F, Fu ZF. 2013a. Rabies virus glycoprotein is an important determinant for the induction of innate immune responses and the pathogenic mechanisms. Vet Microbiol. 162(2):601–613.
- Zhang J, Wu X, Zan J, Wu Y, Ye C, Ruan X, Zhou J. 2013b. Cellular chaperonin CCTγ contributes to rabies virus replication during infection. J Virol. 87(13):7608–7621.
- Zhang S, Liu Y, Fooks AR, Zhang F, Hu R. 2008. Oral vaccination of dogs (Canis familiaris) with baits containing the recombinant rabies-canine adenovirus type-2 vaccine confers long-lasting immunity against rabies. Vaccine. 26:345–350.
- Zhao L, Toriumi H, Kuang Y, Chen H, Fu ZF. 2009. The roles of chemokines in rabies virus infection: overexpression may not always be beneficial. J Virol. 83:11808–11818.
- Zhou M, Zhang G, Ren G, Gnanadurai CW, Li Z, Chai Q, Yang Y, Leyson CM, Wu W, Cui M, et al. 2013. Recombinant rabies viruses expressing GM-CSF or flagellin are effective vaccines for both intramuscular and oral immunizations. PLoS One. 8(5):e63384.
- Zhu S, Guo C. 2016. Rabies control and treatment: From prophylaxis to strategies with curative potential. Viruses. 8(11): pii: E279.
- Zimmer K, Wiegand D, Manz D, Forest JW, Reincher M, Frese K. 1990. Evaluation of five different methods for routine diagnosis of rabies. J Vet Med B. 37:392–400.
- Zinsstag J. 2013. Towards a science of rabies elimination. Infect Dis Poverty. 2(1):22.