Abstract
The application of high doses of mineral feed additives in the form of inorganic salts increases the growth performance of animals, but at the same, due to their low bioavailability, can contaminate the environment. Therefore, there is a need to find a replacement of administering high doses of minerals with an equally effective alternative. The application of lower doses of metal-containing nanoparticles with the same effect on animal production could be a potential solution. In the present review, zinc, silver, copper, gold, selenium, and calcium nanoparticles are discussed as potential feed additives for animals. Production of nanoparticles under laboratory conditions using traditional chemical and physical methods as well as green and sustainable methods – biosynthesis has been described. Special attention has been paid to the biological properties of nanoparticles, as well as their effect on animal health and performance. Nano-minerals supplemented to animal feed (poultry, pigs, ruminants, rabbits) acting as growth-promoting, immune-stimulating and antimicrobial agents have been highlighted. Metal nanoparticles are known to exert a positive effect on animal performance, productivity, carcass traits through blood homeostasis maintenance, intestinal microflora, oxidative damage prevention, enhancement of immune responses, etc. Metal-containing nanoparticles can also be a solution for nutrient deficiencies in animals (higher bioavailability and absorption) and can enrich animal products with microelements like meat, milk, or eggs. Metal-containing nanoparticles are proposed to partially replace inorganic salts as feed additives. However, issues related to their potential toxicity and safety to livestock animals, poultry, humans, and the environment should be carefully investigated.
1. Introduction
The inorganic salts, commonly used as feed additives exhibit poor bioavailability to animals, which is caused by ingredients that can inhibit absorption of micro- and macroelements (Scott et al. Citation2018; Matuszewski et al. Citation2020a; Alagawany and Abd El-Hack Citation2021; Górniak et al. Citation2022). Therefore, they may pose a threat to the environment through excretion of high mineral levels that can contaminate soil and aquatic environment (Mroczek-Sosnowska et al. Citation2015a, Citation2015b; Scott et al. Citation2018; Kociova et al. Citation2020; Matuszewski et al. Citation2020a; Cui et al. Citation2021; Szuba-Trznadel et al. Citation2021). It may be beneficial to apply solutions proposed by nanotechnology to minimize this negative effect on the environment, as well as to enhance health, performance and production of livestock animals and poultry.
Nowadays, nanobiotechnology is an emerging field in animal and veterinary sciences for a variety of practical applications such as therapeutic, diagnostic, and nutritional applications (Abd El-Hack et al. 2017; Prasad et al. Citation2018; Amlan and Lalhriatpuii Citation2020; El-Maddawy et al. Citation2022). Nanoparticles (NPs) of essential minerals, which size from 1 to 100 nm, could be used as an alternative to conventional forms of elements in animal diet (Mohamed et al. Citation2016; Swain et al. Citation2016; Scott et al. Citation2018; Abdollahi et al. Citation2020; Kociova et al. Citation2020; Szuba-Trznadel et al. Citation2021). It is assumed that much smaller doses of nanoparticles will be required to cover animal requirements for elements than bulk minerals (Vijayakumar and Balakrishnan Citation2014; Refaie et al. Citation2015; El Basuini et al. Citation2017; Scott et al. Citation2018; Youssef et al. Citation2019; Abdollahi et al. Citation2020; Szuba-Trznadel et al. Citation2021; Ouyang et al. Citation2021) and thus the environmental impact caused by the high concentration of inorganic salts will be alleviated (Vijayakumar and Balakrishnan Citation2014; Ouyang et al. Citation2021). Reduction in the quantity of minerals supplemented to animal diet could reduce the feed cost as well (Vijayakumar and Balakrishnan Citation2014). Additionally, nano-forms of elements can increase bioavailability to animals (Vijayakumar and Balakrishnan Citation2014; Hill and Li Citation2017; Youssef et al. Citation2019; Hidayat et al. Citation2021), due to their properties such as small size, good homogeneity, high surface area and physical reactivity (Grodzik and Sawosz Citation2006; Scott et al. Citation2018; Abdollahi et al. Citation2020; Amlan and Lalhriatpuii Citation2020; Ouyang et al. Citation2021). The biological properties of nanoparticles such as lesser dose, lower antagonism, greater absorption rate and better tissue distribution can also be beneficial to animals (Amlan and Lalhriatpuii Citation2020). It is well-known that nanoparticles have a great potential even at very low doses (Matuszewski et al. Citation2020a).
Nanoparticles in animal nutrition are explored for growth performance, feed utilization and health status (Abd El-Hack et al. 2017; Amlan and Lalhriatpuii Citation2020; Dawood et al. Citation2021). Nano-forms of micro- and macroelements most frequently increase the body weight, average daily gain, and improve the feed conversion ratio (FCR) (Bąkowski et al. Citation2018; Yusof et al. Citation2019). NPs are used to cover the animal's demand for elements, improve their productivity, ameliorate microbial profile and immune status, as well as reduce the risk of diseases. Nanoparticles are known for their antibacterial, antifungal, antiviral, antiprotozoal, antioxidative properties, etc. Silver, copper, selenium, and zinc nanoparticles can constitute alternative health and growth promoting additives to antibiotics (Sawosz et al. Citation2007; Pineda et al. Citation2012; Bąkowski et al. Citation2018; Kumar and Bhattacharya Citation2019; Yusof et al. Citation2019; Nabi et al. Citation2020; Sheiha et al. Citation2020; Hidayat et al. Citation2021; Morsy et al. Citation2021; Ouyang et al. Citation2021; El-Maddawy et al. Citation2022). Nano-copper and nano-zinc can also increase the activity of the superoxide dismutase enzyme, which constitutes a very important antioxidant defense against oxidative stress (Gonzales-Eguia et al. Citation2009; Refaie et al. Citation2015; Hidayat et al. Citation2021). Nano-selenium is also known to increase the activity of this enzyme (Kojouri et al. Citation2020), and improve the efficiency of the antioxidant system and prevent oxidative stress (Shi et al. Citation2011a; Sadeghian et al. Citation2012; El-Deep et al. Citation2016; Kojouri et al. Citation2020; Han et al. Citation2021).
However, it should be remembered that small size of nanoparticles might affect their toxicity by increasing the cellular uptake and translocation in the animal’s body (Gatoo et al. Citation2014; Scott et al. Citation2016). At the cellular level, nanoparticles can cause inflammation or even cell death (Bąkowski et al. Citation2018). Supplementation of nanoparticles to the animal diet can induce pathological changes in animal tissues, like liver, pancreas, kidney, small intestine, adrenal glands and brain (Bąkowski et al. Citation2018). Therefore, detailed research is still required to confirm the safety of application of metal-containing nanoparticles in animal nutrition, avoiding any harm to livestock, the environment, and human beings (Scott et al. Citation2018).
This comprehensive review aims to provide an up-to-date knowledge about the production of metal/metal oxides nanoparticles (zinc (Zn), silver (Ag), copper (Cu), gold (Au), selenium (Se), and calcium (Ca)) serving as feed additives and their application in the nutrition of various animal species (e.g., poultry, pigs, ruminants, and rabbits). Based on the literature mainly from the last decade, the effect of nano-minerals on livestock and poultry health, performance and production will be discussed. The potential harmful effect of metal/metal oxide nanoparticles on animals will also be highlighted.
2. Production of nanoparticles for animal research
Many minerals are used as nanoparticles in animal research. These include aluminium (Li et al. Citation2011), calcium (Matuszewski et al. Citation2020a; Abdelnour et al. Citation2021; Abo El-Maaty et al. Citation2021), copper (Gonzales-Eguia et al. Citation2009; Miroshnikov et al. Citation2015; Mroczek-Sosnowska et al. Citation2014, Citation2015a, Citation2015b, Citation2017; Refaie et al. Citation2015; Joshua et al. Citation2016; Ognik et al. Citation2016b; Scott et al. Citation2016; El Basuini et al. Citation2017; Tomaszewska et al. Citation2017; Scott et al. Citation2018; Aminullah et al. Citation2021; Morsy et al. Citation2021; Naz et al. Citation2021), gold (Sembratowicz et al. Citation2016; Sanati et al. Citation2019; Hassanen et al. Citation2020), iron (Pilaquinga et al. Citation2021), magnesium (Kesmati et al. Citation2016; Mazaheri et al. Citation2019; Abdelnour et al. Citation2021), nickel (Gong et al. Citation2016), selenium (Zhang et al. Citation2001, Citation2008; Shi et al. Citation2011a; Wu et al. Citation2011; Sadeghian et al. Citation2012; Xun et al. Citation2012; El-Deep et al. Citation2016; Joshua et al. Citation2016; Muralisankar et al. Citation2016; Yaghmaie et al. Citation2017; Gangadoo et al. Citation2018, Citation2020; Hassan et al. Citation2020; Kojouri et al. Citation2020; Nabi et al. Citation2020; Sheiha et al. Citation2020; Han et al. Citation2021; Rana, Citation2021), silver (Grodzik and Sawosz Citation2006; Sawosz et al. Citation2007; Fondevila et al. Citation2009; Fondevila, Citation2010; Ahmadi and Rahimi Citation2011; Pineda et al. Citation2012; Kout-Elkloub et al. Citation2015; Ognik et al. Citation2016a; Conine and Frost Citation2017; Bąkowski et al. Citation2018; Abdelsalam et al. Citation2019; Kumar and Bhattacharya Citation2019; Dung et al. Citation2020; Abdelnour et al. Citation2021; Awaad et al. Citation2021; Bidian et al. Citation2021; Niemiec et al. Citation2021), titanium (Li et al. Citation2011) and zinc (Joshua et al. Citation2016; Swain et al. Citation2016; Hassan et al. Citation2017; Olgun and Yildiz Citation2017; Bąkowski et al. Citation2018; Othman et al. Citation2018; Bakhshizadeh et al. Citation2019; Yusof et al. Citation2019; Abdollahi et al. Citation2020; Kociova et al. Citation2020; Cui et al. Citation2021; Eskandani et al. Citation2021; Hidayat et al. Citation2021; Mahmoud et al. Citation2021; Ouyang et al. Citation2021; Szuba-Trznadel et al. Citation2021; El-Maddawy et al. Citation2022) nanoparticles.
These nanoparticles can be synthesized in the laboratory or are commercially available. Nanoparticles can be produced using traditional chemical and physical methods, as well as green and sustainable method – biosynthesis with plant extracts or microorganisms (Gopi et al. Citation2017; Yusof et al. Citation2019; Abdelnour et al. Citation2021). The general scheme showing available NPs production methods is presented in .
There are several examples of laboratory-made mineral nanoparticles, which were tested as feed additives. They include the following types Ag (Ognik et al. Citation2016a; Abdelsalam et al. Citation2019; Dung et al. Citation2020; Kumar and Bhattacharya Citation2019; Awaad et al. Citation2021), Au (Sembratowicz et al. Citation2016; Hassanen et al. Citation2020), Ca (Abo El-Maaty et al. Citation2021), Cu (Gonzales-Eguia et al. Citation2009; Miroshnikov et al. Citation2015; Refaie et al. Citation2015; Joshua et al. Citation2016; Ognik et al. Citation2016b; Morsy et al. Citation2021), Se (Sadeghian et al. Citation2012; Joshua et al. Citation2016; Gangadoo et al. Citation2018; Gangadoo et al. Citation2020; Sheiha et al. Citation2020), and Zn (Joshua et al. Citation2016; Bakhshizadeh et al. Citation2019; Kociova et al. Citation2020; Hidayat et al. Citation2021; Mahmoud et al. Citation2021; Ouyang et al. Citation2021).
2.1. Physical methods
Physical methods include evaporation–condensation, chemical vapor deposition, laser ablation, electric arc discharge, ball milling–annealing, gas phase synthesis and physical vapor precipitation. The biggest advantages of these methods are the absence of solvent contamination and the maximal recovery of nanoparticles (Patra and Lalhriatpuii 2020; Abdelnour et al. Citation2021).
Gonzales-Eguia et al. (Citation2009) prepared copper nanoparticles using the wet polish method with a ball grinding machine. These nanoparticles were applied as a supplementation for weanling pigs. Miroshnikov et al. (Citation2015) produced copper nanoparticles for chicken by high temperature condensation. Furthermore, copper nanoparticles injected to chicken eggs were manufactured by a non-explosive high voltage method (Mroczek-Sosnowska et al. Citation2015a, Citation2015b, Citation2017; Scott et al. Citation2016). Joshua et al. (Citation2016) prepared copper nanoparticles using electrochemical method with copper rods as anode and cathode. Ognik et al. (Citation2016b) used copper nanoparticles prepared in a physical process – a non-explosive, high-current method for degradation of metals – as additives to water of chickens. Most recently, Aminullah et al. (Citation2021) used induction coupled plasma method to prepare copper nanoparticles that were used as a supplement for Swarnadhara breeder hens. Sembratowicz et al. (Citation2016) used in chicken feeding gold nanoparticles also produced by a non-explosive, high-current method for degradation of metals. Sawosz et al. (Citation2007) applied, in their research on quail, silver nanoparticles (added to the drinking water) produced by the solid–liquid phase discharge method. Pineda et al. (Citation2012) used in broiler chickens feed silver nanoparticles also produced by a non-explosive high voltage method (Polish Patent 3883399) from high purity metals and demineralized water. Mahmoud et al. (Citation2021) checked if zinc oxide (ZnO) nanoparticles, prepared by mechanical milling of a commercial ZnO with a planetary ball mill (Othman et al. Citation2018), can prevent multi-drug resistant Staphylococcus-induced footpad dermatitis in broilers. In summary, physical methods are most often used to produce copper nanoparticles that were mainly applied as feed additives for poultry.
2.2. Chemical methods
Chemical methods include chemical reduction, sol gel method and inert gas condensation. They allow achieving more effective and controlled bulk production of nanoparticles as compared to physical methods. The disadvantage is possible toxicity due to the use of hazardous chemicals during the synthesis (Patra and Lalhriatpuii 2020; Abdelnour et al. Citation2021).
Joshua et al. (Citation2016) used chemical synthesis with starch as a stabilizing agent to produce ZnO nanoparticles, the effect of which on post-hatch performance of broiler chickens was investigated. Most recently, zinc nanoparticles, used as a supplement to broiler chicken's diet, were synthesized using nano-chelating technology (Eskandani et al. Citation2021). Bakhshizadeh et al. (Citation2019) prepared zinc nanoparticles for dietary cows using the chemical co-precipitation method with zinc nitrate and sodium hydroxide. Kociova et al. (Citation2020) synthesized zinc phosphate-based nanoparticles for feeding of weaned piglets in two ways – the first one was from zinc nitrate and diammonium phosphate and the second one from zinc nitrate and tetrasodium pyrophosphate. Li et al. (Citation2021) used the sol–gel process to create ZnO quantum dots (ZnO QDs) with a size range of 3–6 nm and studied their antibacterial properties in vitro and in vivo (chickens).
Ognik et al. (Citation2016a) synthesized silver nanoparticles for chicken feeding by reducing silver ions with trisodium citrate at 100 °C. Abdelsalam et al. (Citation2019) prepared silver nanoparticles from sodium tricitrate aqueous solution and silver nitrate and the colloid form of nanoparticles was injected to rabbits. For the synthesis of silver nanoparticles for poultry, Kumar and Bhattacharya (Citation2019) used hydrothermal method with an autoclave (to the mixture of polyvinylpyrrolidone and sodium borohydride, aqueous solution of silver nitrate was added). Silver nanoparticles synthesized via chemical reduction method, using silver nitrate and sodium borohydride as a reducing agent, as well as chitosan solution as a stabilizer, were applied as antiviral agents against African swine fever virus (Dung et al. Citation2020). Awaad et al. (Citation2021) also used chemical reduction method, in which silver nitrate and aqueous solutions containing acetyl trimethyl ammonium bromide and hydrazine hydrate were applied to receive silver nanoparticles, supplemented to broiler chickens experimentally infected with Escherichia coli.
Refaie et al. (Citation2015) prepared copper nanoparticles from copper nitrate trihydrate and ethylene glycol and used them as a supplement for rabbits. Morsy et al. (Citation2021) synthesized copper oxide nanoparticles as chicken's feed additives via chemical precipitation method, using copper chloride dihydrate and sodium hydroxide solutions in ethanol. Chemical synthesis of copper oxide nanoparticles in the research of Naz et al. (Citation2021) was achieved by the reaction of copper acetate monohydrate with sodium hydroxide upon continuous stirring and heating. The aim of this work was to examine the copper NPs toxicity on rats and offspring. Muralisankar et al. (Citation2016) used chemical synthesis with copper chloride, sodium dodecyl benzenesulfonate and hydrazine hydrates to prepare copper nanoparticles, which were used as supplementation on freshwater prawn Macrobrachium rosenbergii post larvae.
Selenium nanoparticles were chemically produced from sodium selenite and glutathione by Zhang et al. (Citation2001) and later used as a chemo-preventive agent for mice (Zhang et al. Citation2008). Later, Sadeghian et al. (Citation2012) produced selenium nanoparticles from selenium oxide and ascorbic acid and used them as a feed additive for sheep. Joshua et al. (Citation2016) used chemical synthesis with selenium powder and sodium hydroxide to prepare nanoparticles for broiler chickens’ supplementation. Gangadoo et al. (Citation2018, Citation2020) synthesized selenium nanoparticles for poultry using chemical reduction with selenium tetrachloride, ascorbic acid as a reducing agent and polystyrene-4-sulfonate as a protecting agent. Recently, Hassan et al. (Citation2020) used sodium sulphate and selenium powder to produce selenium nanoparticles for broiler chickens exposed to heat stress. Selenium nanoparticles were also chemically synthesized by Kojouri et al. (Citation2020), who used ascorbic acid solution and aqueous solution of selenium oxide and checked their impact on neonatal lamb weight gain pattern. Sheiha et al. (Citation2020) used the selenium nanoparticles prepared by wet chemical approach using sodium selenite and l-cysteine as reagents in the feeding of growing rabbits reared under thermal stress.
Hassanen et al. (Citation2020) synthesized gold nanoparticles for broiler chickens by chemical reduction of gold chloride hydrate with tri-sodium citrate dehydrate under boiling conditions. Iron (II and III) oxide nanoparticles were synthesized using a co-precipitation method and were used to check their effect on fertility and iron bioaccumulation in Drosophila melanogaster (Pilaquinga et al. Citation2021). Based on the presented examples, chemical methods are much more often used for the synthesis of a wide range of nanoparticles (with Zn, Ag, Cu, Se, Au, Fe) than physical methods and these NPs have potential applications as feed additives in the nutrition of many animal species, such as chickens, cows, pigs, piglets, rabbits, sheep, etc.
2.3. Biological methods
Biological methods are currently gaining popularity because they are eco-friendly, efficient, easy, and less toxic than traditional methods. In this method, plant extracts, algal extracts, or microorganisms (bacteria, viruses, and fungi) are used as simple substitutes for chemical and physical processes (Eszenyi et al. Citation2011; Patra and Lalhriatpuii 2020; Abdelnour et al. Citation2021). The compounds in these extracts such as polyphenols, terpenoids, sugars, proteins, etc. act as reducing agents maintaining the minerals in a reduced state during biosynthesis (Gopi et al. Citation2017). The limitations for biological methods are maintaining the culture media and the culture conditions, the difficulty in nanoparticle's recovery, and the duration in the creation of nanoparticles (Abdelnour et al. Citation2021). These methods of synthesis are currently not as popular as physical or chemical, but their popularity has grown in the last few years.
For the biological synthesis of calcium carbonate nanoparticles, serving as a supplementation for aged laying hens, Abo El-Maaty et al. (Citation2021) used extract from brown macroalga Sargassum latifolium. Naz et al. (Citation2021) prepared copper oxide nanoparticles from Rhus punjabensis leaf extract and compared their toxicity during pregnancy and lactation in rats and offspring with chemically produced nanoparticles. El-Deep et al. (Citation2016) used biosynthesized selenium nanoparticles (with the use of bacteria strains like Lactobacillus casei, Streptococcus thermophilus, Bifidobacterium BB-12, Lactobacillus acidophilus (LA-5), and Lactobacillus helveticus (LH-B02)) in the feeding of broiler chickens. Sheiha et al. (Citation2020) also used lactic acid bacteria isolated from human breast milk to biosynthesize selenium nanoparticles. Bidian et al. (Citation2021) biosynthesized silver nanoparticles with the use of Viburnum opulus fruit extract to check their impact on the ultrastructure and cell death in the testis of offspring rats. Hidayat et al. (Citation2021) used guava leaf extract (Psidium guajava) to biosynthesize zinc nanoparticles that were used as a supplementation of broiler chicken’s diet. Despite many advantages of nanoparticles biosynthesis, the variability of organisms that are used for their direct synthesis, as well as the production of natural extracts rich in bioactive compounds serving as reducing/stabilizing agents of nanoparticles should be considered. Additionally, unlike physical and chemical methods used for nanoparticles production, large-scale research is lacking in NPs biosynthesis.
2.4. Commercially available nanoparticles
A wide range of nanoparticles, which could be used in animal studies, is accessible on the market as shown in numerous publications, for example Ag (Grodzik and Sawosz Citation2006; Pineda et al. Citation2012), Au (Sembratowicz et al. Citation2016), Cu (Mroczek-Sosnowska et al. Citation2015b; Mroczek-Sosnowska et al. Citation2017; Aminullah et al. Citation2021), Se (Shi et al. Citation2011a; Xun et al. Citation2012; Yaghmaie et al. Citation2017; Han et al. Citation2021), and Zn (Olgun and Yildiz, Citation2017; Abdollahi et al. Citation2020; Cui et al. Citation2021; Eskandani et al. Citation2021; Szuba-Trznadel et al. Citation2021; El-Maddawy et al. Citation2022). In many experiments on animals and poultry, nanoparticles were purchased to check their impact on performance, productivity, health, and disease prevention and control. Han et al. (Citation2021) used selenium nanoparticles as a supplementation for lactating dairy cows; Shi et al. (Citation2011a) and Xun et al. (Citation2012) used selenium nanoparticles to check their impact on feed digestibility, rumen fermentation and urinary purine derivatives in sheep; selenium nanoparticles were tested by Wu et al. (Citation2011) on cashmere goats; Yaghmaie et al. (Citation2017) used selenium nanoparticles in experiments on lambs; Grodzik and Sawosz (Citation2006) used, in their research on chicken embryo development, silver nanoparticles; Szuba-Trznadel et al. (Citation2021) checked the influence of zinc nanoparticles on growth performance and zinc status of weaned piglets; Abdollahi et al. (Citation2020) used ZnO nanoparticles in feeding experiments on young Holstein calves to examine performance, rumen fermentation, mineral status, and antioxidant activity; Ouyang et al. (Citation2021) used ZnO nanoparticles to evaluate their antimicrobial properties in weanling piglets and El-Maddawy et al. (Citation2022) used zinc oxide nanoparticles to investigate their anticoccidial efficacy in broiler chickens infected with mixed Eimeria species in an experimental setting.
3. Applications of nanoparticles as feed additives on animal and poultry performance and health
Different types of metal/metal oxide nanoparticles have already been tested as potential feed additives. presents the number of papers, according to the Web of Science database, on (a) type of nanoparticles used as feed additives for animals and (b) the species of animal supplemented with nanoparticles as feed additives. The most often tested are zinc, silver, and copper nanoparticles and among animal species the application of nanoparticles in poultry diet dominates (Mohapatra et al. Citation2014a,Citationb; Abd El-Hack et al. 2017).
Figure 2. The number of papers on (a) type of nanoparticles used as feed additives for animals and (b) the species of animal supplemented with nanoparticles as feed additives (Web of Science, September 9, 2021).
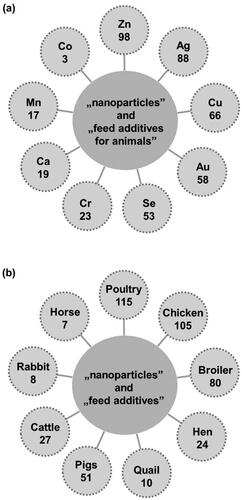
In the first step of testing metal nanoparticles as potential animal feed additives, many researchers conduct preliminary nano-toxicological studies to determine the impact of examined nanoparticles on cell cultures (Leng et al. Citation2020) or animal models, for example mice (Zhang et al. Citation2008; Kesmati et al. Citation2016), rats (Tomaszewska et al. Citation2017; Mazaheri et al. Citation2019; Sanati et al. Citation2019; Kociova et al. Citation2019; Bidian et al. Citation2021; Naz et al. Citation2021), worms (Ayech et al. Citation2020), insects like Drosophila melanogaster (Pilaquinga et al. Citation2021) or in the case of aquatic organisms on water flea, like Daphnia magna (Gong et al. Citation2016; Conine and Frost Citation2017), Ceriodaphnia dubia (Li et al. Citation2011) or fish (Gutierrez et al. Citation2021). The findings obtained for these organisms may be extrapolated on other living beings (Pilaquinga et al. Citation2021).
In this review we focus on the impact of the metal-containing nanoparticles on poultry, pigs, ruminants, and rabbits. The beneficial effects of nano-minerals on animals are generally illustrated in .
When using nanoparticles in animal nutrition, several aspects seem to be important. Metal/metal oxide nanoparticles used as feed additives can be supplemented directly to the feed or to the drinking water. In the case of poultry, in ovo administration of nanoparticles can be used as a method of nano-nutrition, supplying the embryos with an additional amount of nutrients (Pineda et al. Citation2012; Mroczek-Sosnowska et al. Citation2014, Citation2015b, Citation2017; Joshua et al. Citation2016; Saeed et al. Citation2019). It is hypothesized that in ovo supplementation of metal-containing nanoparticles allows for better utilization of microelements during embryo development than in the case of feed supplementation (Mroczek-Sosnowska et al. Citation2015b). Silver nanoparticles administered in ovo may also constitute potential antimicrobial growth-promoting supplement for poultry (Grodzik and Sawosz Citation2006; Pineda et al. Citation2012). Another method of administering metal-containing nanoparticles is parenteral injection – intramuscular (e.g., chicken – Miroshnikov et al. Citation2015) or subcutaneous (e.g., rabbits – Abdelsalam et al. Citation2019).
As seen in , in the feeding experiments, the effect of nanoparticles on animals is usually compared with organic or more frequently inorganic forms of elements (e.g., Gonzales-Eguia et al. Citation2009; Sadeghian et al. Citation2012; Xun et al. Citation2012; Mroczek-Sosnowska et al. Citation2015b; El-Deep et al. Citation2016; Olgun and Yildiz Citation2017; Yaghmaie et al. Citation2017; Gangadoo et al. Citation2018; Bakhshizadeh et al. Citation2019; Abdollahi et al. Citation2020; Aminullah et al. Citation2021; Eskandani et al. Citation2021; Hidayat et al. Citation2021; Ouyang et al. Citation2021; Szuba-Trznadel et al. Citation2021). For example, interesting results were presented by Sheiha et al. (Citation2020) who compared the effect of two types of selenium-nanoparticles – biologically or chemically-synthesized on rabbits exposed to thermal stress. Better effects in terms of growth, carcass characteristics, oxidative and inflammatory parameters were observed for biosynthesized NPs.
Table 1. Effects of nano-minerals on growth performance and productivity of different animal species (available literature from the last 10 years).
Metal/metal oxide nanoparticles supplemented to animal diet can improve not only animal performance and productivity such as meat, milk, and eggs production, but also ameliorate the quality of the animal-derived products (Hill and Li Citation2017; Mekonnen Citation2021). Usually, enrichment of animal products like meat or eggs with microelements is observed (e.g., Shi et al. Citation2011b; Hu et al. Citation2012; Mroczek-Sosnowska et al. Citation2014; Selim et al. Citation2015a; Sathyabama and Jagadeeswaran Citation2016; Eskandani et al. Citation2021). Nanoparticles can increase the bioavailability of nutrients to animals due to their nanoscale. Absorption of nutrients in the gut may be enhanced and can exert strengthened biological effects in the target tissues of animals (Bunglavan et al. Citation2014; Vijayakumar and Balakrishnan Citation2014; Hill and Li Citation2017; Youssef et al. Citation2019; Amlan and Lalhriatpuii Citation2020). Minerals in the nanoparticle form can pass through the stomach wall and into body cells faster than common inorganic salts with a larger particle size (Bunglavan et al. Citation2014). Hu et al. (Citation2012) showed that in the case of selenium, its nano-form transfer from the intestinal lumen to the body was significantly higher than for sodium selenite with significantly lower intestinal retention of nano-Se than for the inorganic form. Retention of nano-Se in the body was more efficient than sodium selenite. Metal-containing nanoparticles can be a solution for nutrient deficiencies in animals and can enrich animal products with microelements. Additionally, minerals in the form of nanoparticles are believed to reduce intestinal minerals antagonism and thus minimize their excretion and environmental pollution (Gopi et al. Citation2017).
Supplementation of nano-minerals can also reduce oxidative stress and improve serum oxidant status. The increase in activity of antioxidant enzymes such as glutathione peroxidase (GSH-Px), superoxidase dismutase (SOD), catalase (CAT), and total antioxidant activity (AOA) while decrease in malondialdehyde (MDA) is a common phenomenon, for example for SeNPs (Shi et al. Citation2011b; Hu et al. Citation2012; Aparna and Karunakaran Citation2016; Yaghmaie et al. Citation2017), ZnNPs (Ahmadi et al. Citation2014; Ibrahim et al. Citation2017; El-Katcha et al. Citation2018; El-Maddawy et al. Citation2022) and AgNPs (Abdelsalam et al. Citation2019). Antioxidant enzymes are responsible for elimination of reactive oxygen species, whereas MDA serves as an index of antioxidant status, is the end product of the oxidative stress (Shi et al. Citation2011b). Shokraneh et al. (Citation2020) showed that in ovo injection of nano-selenium (40 μg) and nano-zinc (500 μg) alleviated the negative effects of heat stress, induced by high eggshell temperature during late incubation, through boosting antioxidant activity and reducing oxidative stress (increased GSH-Px and SOD activity, while decreased corticosterone, cortisol, T4 and T3) in broiler hatchlings.
Metal/metal oxide nanoparticles are also known to be a potential alternative to antibiotics (Hill and Li Citation2017; Swain and Prusty Citation2021a). Examples of the antimicrobial effects of nano-minerals on different animal species are presented in . Zinc, silver, and selenium nanoparticles are mainly examined as antimicrobial agent. The best known are silver nanoparticles, which exhibit a broad spectrum of antimicrobial properties, for example against Escherichia coli, Staphylococcus aureus, Salmonella typhimurium, Salmonella pullorum, Klebsiella, Pseudomonas, and yeast (Kim et al. Citation2007; Wahab et al. Citation2010; Mekonnen Citation2021; Li et al. Citation2021), in addition to fungi (Aspergillus flavus, A. niger and A. ochraceus) and produced mycotoxins (Hassan et al. Citation2013; Swain and Prusty Citation2021a), as well as Eimeria species in rabbits and chickens (Chauke and Siebrits Citation2012; Abd El Megid et al. Citation2018; El-Maddawy et al. Citation2022). Generation of the free radicals or reactive oxygen species by Ag nanoparticles can inhibit microbial growth as their interaction with bacterial cells may lead to breaking down the bacterial cell wall (Kim et al. Citation2007).
Table 2. Ameliorative and antimicrobial effects of nano-minerals on different animal species – in vitro and in vivo studies.
4. Metal nanoparticles in the nutrition of particular animal species
4.1. Zinc (Zn) nanoparticles
The significance of zinc in animal feeding, its bioavailability (absorption) in the body, properties of Zn nanoparticles, their effect on biological systems/animal performance (growth, milk production), rumen fermentation, immunity, reproduction, antibacterial activity, and toxicity were described in detail in the reviews of Swain et al. (Citation2016), Bąkowski et al. (Citation2018), Yusof et al. (Citation2019), Amlan and Lalhriatpuii (Citation2020), and Abdelnour et al. (Citation2021). Zinc oxide nanoparticles (ZnONPs) have good prospects for the use as one of the new antibiotics (Yusof et al. Citation2019; Ouyang et al. Citation2021) and anticoccidial alternatives (El-Maddawy et al. Citation2022). The nano-zinc can also increase the activity of the superoxide dismutase enzyme, which constitutes a very important antioxidant defense against oxidative stress (Gonzales-Eguia et al. Citation2009; Refaie et al. Citation2015; Hidayat et al. Citation2021). Examples of zinc nanoparticles applications in various species of animal's nutrition are presented below.
4.1.1. Poultry
Eskandani et al. (Citation2021) compared the effect of different Zn sources (control – ZnSO4, organic – amino acid complex and Zn NPs at doses 30, 50, 70 and 90 mg/kg of diet) in the diet of broiler chickens on performance, carcass characteristics, humoral immunity, meat quality and Zn content in meat. Improvement of these parameters was observed for ZnNPs applied at a dose of 70 mg/kg of diet. Hidayat et al. (Citation2021) tested several doses of ZnNPs (45, 90, 135 and 180 mg/kg) on broilers. The addition of zinc nanoparticles up to 90 mg/kg had a positive effect on performance, antioxidant activity, and reduction in population of pathogenic intestinal bacteria (E. coli and Salmonella sp.). In addition, they exerted antifungal activity against toxigenic moulds in feed systems (Aspergillus flavus, A. ochraceus and A. niger) (Hassan et al. Citation2013). The weight of lymphoid organs in broiler chickens increased as well as humoral immunity was improved by supplementation of nano-Zn (0.06 mg/kg diet) similarly to that of 15 mg/kg diet of organic Zn (Sahoo et al. Citation2014). Hafez et al. (Citation2020) reported an enhancement of IgY production and cellular immunity (total lymphocyte count, macrophages, phagocytic activity, and index) in broiler chickens by ZnONPs supplementation compared to ZnO. Nano-form of zinc (20, 40, 60 and 80 μg/egg), in ovo supplemented in fertile broiler eggs, had no harmful effect on embryo development, did not influence the hatchability and can be used to improve the post-hatch performance of broilers (Joshua et al. Citation2016).
Supplementation of zinc in nano-form into the diet of turkey hens in the dose covering 10% of the demand (10 mg/kg of diet) maintained homeostasis in turkey muscles, as indicated by the activity of the aminopeptidases (alanyl, leucyl, and arginyl) (Jóźwik et al. Citation2018).
Abbasi et al. (Citation2017) evaluated dose–response of ZnONPs supplementation (25, 50, 75 and 100 mg/kg of diet) to Japanese quails from 10 to 40 days of age. Growth performance was increased significantly in zinc supplemented birds between 20 to 30 days. The optimal ZnONPs levels for body weight gain of Japanese quails were 90, 70 and 59 mg/kg of diet for birds 10–20 days old, 20–30 days old, and 30–40 days old, respectively. The relative weights of testes and thigh were increased significantly by increasing dietary Zn levels. Among doses of biological nano-zinc (0.1, 0.2, 0.3 and 0.4 g/kg diet) administered to growing Japanese quails, ZnNPs at doses of 0.1–0.3 g/kg diet resulted in an increase in the activity of ALT, AST, LDH, superoxide dismutase, glutathione peroxidase, and concentrations of malondialdehyde, and immunoglobulins G and M (IgG and IgM), as well as an increase in beneficial microbial populations. The total cholesterol, high-density lipoprotein, and low-density lipoprotein concentrations are the only among liver profile parameters influenced by ZnNPs. A significant improvement in body weight, weight gain, feed intake, and feed conversion ratio were observed by 0.2 g/kg of ZnNPs supplementation (Reda et al. Citation2021). Nano- or micro-particles of ZnO were supplied to Japanese quails from 47 to 75 days of age with doses of 49, 74, 99, and 124 mg zinc per kg/diet to investigate their effects on performance, fertility, hatchability, and egg quality characteristics (Abbasi et al. Citation2022). Quails fed diets supplement with ZnO showed significant improvement of the eggshell thickness regardless of particle size, as well as higher egg weight and eggshell surface as compared with ZnO-non-supplemented birds. The maximum egg production percentage was achieved when 67 or 72 mg/kg of dietary zinc was supplied from nano- and micro-ZnO, respectively, in addition to a considerable enhancement of fertility, which was shown by nano-ZnO supplementation. Hence, nano-ZnO could decrease the zinc requirement in laying Japanese quail (Abbasi et al. Citation2022).
Zinc in the diet was shown to be effective in maintaining laying bird performance, whereas Zn-glycine was found to be responsible for superior eggshell quality (Olgun and Yildiz Citation2017). However, in comparison to the Zn-sulphate and Zn-glycine forms, supplementing feed of laying hens with zinc nanoparticles (50, 75, and 100 mg/kg of diet) had a negative effect on eggshell thickness and bone mechanical characteristics while increasing egg weight (Olgun and Yildiz Citation2017). Despite this, Abedini et al. (Citation2018) found that laying hens fed 40 and 80 mg Zn/kg of feed as ZnONPs had improved eggshell thickness and strength, as well as egg mass. Even, dietary Zn source either inorganic, organic, or nano-Zn had no effect on egg mass and eggshell quality (Cufadar et al. Citation2020). Moreover, nano-Zn supplementation may aid in egg quality preservation through increasing of Zn content in egg yolk (Alagawany et al. Citation2018).
Dietary ZnONPs have shown to be beneficial in ameliorating the negative effects of heat stress (Ramiah et al. Citation2019; Shokraneh et al. Citation2020). Akhavan-Salamat and Ghasemi (Citation2019) compared three sources of dietary Zn (Zn oxide, Zn-Methionine (Zn-Met), and Zn oxide nanoparticles – ZnONPs) and three doses of supplemental Zn (low: 20 mg/kg, adequate: 40 mg/kg, and high: 80 mg/kg of diet) to broiler chickens reared under heat stress beginning from 14 to 42 days. Dietary Zn had no influence on growth performance induced by Zn content, but total and IgM antibody titers against sheep red blood cells (SRBC) were increased significantly by high-Zn level. The average daily weight gain from 0 to 42 days, relative spleen weight, and serum superoxide dismutase activity in the Zn-Met and ZnONP supplemented groups were significantly greater than those of Zn oxide treatment. Moreover, overall FCR and serum malondialdehyde concentration were decreased by dietary supplementation of Zn-Met and ZnONPs. They concluded that the supplementation of Zn in the forms of Zn-Met and ZnONPs at a dose of 40 mg/kg of diet could beneficially affect the growth performance, immune response, and antioxidant status of broiler chickens reared under high temperatures.
Moreover, zinc nanoparticles have shown antibacterial activity. Mahmoud et al. (Citation2021) indicated that dietary supplementation of 10, 20, 30, or 40 mg/kg of diet by ZnONPs prevented multidrug resistant Staphylococcus aureus-induced footpad dermatitis and ameliorated the negative effects on behavior (standing and walking), performance (daily body weight gain, feed conversion ratio) and welfare changes in broilers. In addition to the significantly higher latency to lie time (LLT), lower serum cortisol concentration was observed only for groups with ZnONPs doses − 30 and 40 mg/kg of diet and non-infected groups in comparison with the S. aureus group. Additionally, zinc oxide nanoparticles showed an anticoccidial effect in broiler chickens experimentally infected with a mixture of Eimeria maxima, E. acervulina, E. mivati, and E. tenella and appeared as effective as diclazuril, a chemical anticoccidial drug, through evaluation of growth performance, parasitological and hematological parameters, as well as antioxidant activity (El-Maddawy et al. Citation2022).
4.1.2. Pigs
Ouyang et al. (Citation2021) showed that porous nanoparticles of ZnO (500 and 1000 mg/kg of diet) had better anti-diarrhoea and growth promoting effect when compared with ordinary-size ZnO (3000 mg/kg of diet). There were no significant differences in average daily feed intake or average daily gain of piglets among the tested groups. Supplementation of ZnO nanoparticles to the diet of weaned piglets (500, 1000 and 2000 mg/kg of diet) significantly increased their weight gain when compared with inorganic ZnO. Feces of piglets fed with ZnONPs contained lower number of the total aerobic bacteria and coliform counts than ZnO groups (Kociova et al. Citation2020). The application of Zn nanoparticles in pig's diet can be one of the strategies for preventing diarrhea, which is common in weaned piglets. Szuba-Trznadel et al. (Citation2021) showed that low levels of ZnO nanoparticles (150 mg/kg of diet) can exhibit a similar antidiarrheal action as high therapeutic doses of ZnO (from 1000 to 4000 mg/kg of diet). Additionally, average daily gain in the group with ZnONPs was significantly higher than in the group with inorganic zinc supplied as ZnSO4. In the work of Cui et al. (Citation2021), nano-zinc (200, 300, 400, 500 mg/kg of diet) had a positive effect on piglets – especially 500 mg/kg of diet – improved intestinal antioxidant capacity (increase in the superoxide dismutase activity, total antioxidant capacity of duodenum and ileum, catalase in the jejunum and ileum, copper–zinc superoxide dismutase and glutathione peroxidase activities in the ileum and reduction in the malondialdehyde content in the jejunum), enhanced intestinal immunity (immunoglobulins concentration), improved intestinal morphology of duodenum, jejunum and ileum and reduced zinc content in liver and feces. But zinc nanoparticles had no significant effect on growth performance (Cui et al. Citation2021).
4.1.3. Ruminants
The effect of nano-zinc on growth performance, feed digestibility, milk yield parameters, antioxidant status and health, serum antioxidant parameters, immune response and serum/milk composition and reproduction of ruminants was described in detail by Abdelnour et al. (Citation2021). Many authors showed the promising positive effects of zinc nanoparticles on animal performance (Cui et al. Citation2021; Eskandani et al. Citation2021). In the research of Abdollahi et al. (Citation2020), pre- and post-weaning calves obtained in the diet zinc in the common form – ZnO and as high-surface ZnO nanoparticles. Calves receiving ZnONPs showed higher level of the post-weaning dry matter intake, nutrient digestibility, blood hematocrit and blood Zn concentration than calves supplemented with common ZnO. Additionally, both Zn forms positively affected the concentration of rumen volatile fatty acids, decreased rumen ammonia–N concentration, increased the blood total antioxidant capacity, and lowered the incidence of pneumonia and diarrhea in calves (Abdollahi et al. Citation2020). Bakhshizadeh et al. (Citation2019) compared the effect of three different zinc sources (control diet without zinc supplementation, zinc oxide, zinc glycine and nano-zinc) at a dose of 60 mg/kg of diet on dairy cows. There were no differences between examined groups in dry matter intake, milk yield, body weight, biochemical and hematological parameters (with exception of blood urea nitrogen concentration, which was lower in the zinc glycine and nano-zinc group than in the zinc oxide and the control group). Zinc supplementation in the form of zinc nanoparticles increased the superoxide dismutase activity and plasma Zn concentration when compared with ZnO and control group. As such, nano- and organic Zn sources in the diet of dairy cows were more suitable feed additives than inorganic Zn.
Zinc oxide nanoparticles were also proposed to control aflatoxin B1 contamination caused by fungi belonging to the genera of Aspergillus (A. flavus, A. ochraceus, and A. niger) in cattle feed – yellow corn (Hassan et al. Citation2017). Among tested concentrations − 0.025, 0.05, 0.1, 0.2, 0.3 and 0.4 mg/kg of feed, degradation of aflatoxin required high doses of ZnONPs − 0.3 mg/kg of feed. However, frequent addition of nanoparticles to feeding may result in toxicity to animals, therefore in this research the combination of ZnONPs (0.05 mg/kg of feed) and ozone fumigation for 10 minutes was applied to achieve the same degradation effect.
Hozyen et al. (Citation2019) prepared two forms of synthesized ZnONPs (sonochemically synthesized capped ZnONPs were compared with aggregated uncapped ZnONPs) and tested them at different concentrations for their antimicrobial effects in vitro against Staphylococcus aureus, Escherichia coli and Klebsiella pneumoniae isolated from milk samples of affected cows with clinical mastitis. Capped dispersed ZnONPs showed higher antibacterial activity against S. aureus, E. coli, and K. pneumoniae than aggregated uncapped ZnONPs at same concentrations. They observed that the inhibition zone for two forms of ZnONPs was concentration dependent. Additionally, Gram-positive S. aureus showed higher resistance to both ZnONPs than Gram-negative E. coli and K. pneumoniae.
Swain et al. (Citation2021b) evaluated the effects of nano-zinc (ZnNPs, dose 25 or 50 mg/kg diet) and inorganic zinc (dose of 50 mg/kg diet) on immune status, and hormone profiles (T3, T4 and Insulin-like growth factor 1 (IGF-1) in goats through 90 days feeding trial. ZnNPs supplementation by 25 mg/kg of diet had a similar improved effect on immunity and IGF-1 serum level compared with inorganic Zn at 50 mg/kg dose without changes of thyroid hormones profile, while 50 mg of ZnNPs/kg diet showed the highest humoral and cell-mediated immunity.
4.2. Silver (Ag) nanoparticles
The effect of silver nanoparticles on animal productive performance, blood parameters, immune system, their accumulation in animals’ organs and intestinal microbiome was summarized in the reviews of Fondevila (Citation2010), Gangadoo et al. (Citation2016), Bąkowski et al. (Citation2018), etc. Since silver compounds are known for their antimicrobial properties, silver nanoparticles are considered as a potential antimicrobial feed additive (Grodzik and Sawosz Citation2006; Sawosz et al. Citation2007; Pineda et al. Citation2012; Ognik et al. Citation2016a; Kumar and Bhattacharya Citation2019; Youssef et al. Citation2019; Awaad et al. Citation2021; Niemiec et al. Citation2021). Silver nanoparticles show inhibitory effects on various species of bacteria, including Escherichia coli and Staphylococcus aureus (Sawosz et al. Citation2007; Pineda et al. Citation2012; Kumar and Bhattacharya Citation2019), Salmonella, Streptococcus, and total mesophilic bacteria (Dobrzanski et al. Citation2010) and can be used in a variety of animal species.
4.2.1. Poultry
Most of the experiments concerning AgNPs testing in poultry are related to their antimicrobial properties and effect on the microbial profile. In the work of Awaad et al. (Citation2021), 1-day old broiler chickens infected with Escherichia coli O78 were fed with the diet supplemented with AgNPs at doses 4, 6 and 8 mg/kg of diet. The treatment of colisepticemic chickens with the lowest dose improved productive performance (weekly individual body weight, feed intake and feed conversion ratio), reduced gross and histopathological lesion scores and virulence genes expression (Awaad et al. Citation2021). Ognik et al. (Citation2016a) showed that silver nanoparticles hydrocolloid and lipid-coated nanosilver hydrocolloids at a dose of 5 mg per kg body weight per day, tested on broiler chickens increased the total number of aerobic mesophilic bacteria and decreased the number of Coli group bacteria. Supplementation of silver nanoparticle had no effect on growth performance and jejunum morphology. Kumar and Bhattacharya (Citation2019) added silver nanoparticles to drinking water (50 mg/L) of chickens. AgNPs revealed antibacterial activity against Escherichia coli, significantly reduced the poultry mortality rate, increased significantly feed intake and body weight but had no significant effect on feed conversion ratio. Kout-Elkloub et al. (Citation2015) indicated that broilers fed with diet supplemented with AgNPs had decreased number of E. coli as compared to the control and had no effect on microflora represented by Lactobacillus. Among tested doses of AgNPs (2, 4, 6, 8 and 10 mg/kg of diet) in the diet of broilers, the dose of 4 mg/kg of diet caused the greatest body weight gain, the highest final body weight and the best feed conversion ratio among the tested groups. Level of nano-silver had no significant impact on overall feed intake. AgNPs decreased serum total lipids and significantly increased total serum antioxidant capacity in all treatments. Ahmadi and Kurdestany (Citation2010) supplemented silver nanoparticles to the drinking water of male broiler chickens at doses 5, 15 and 25 mg/L. This additive had no effect on performance. Weight of bursa was reduced when compared with the control group and the highest decrease was observed for the highest AnNPs dose. Nano-silver increased catalase and glutathione peroxidase activity and malondialdehyde content and decreased superoxidase dismutase activity. Silver nanoparticles added to drinking water of Japanese quails significantly increased the number of bacteria (Lactobacillus spp., Leuconostoc lactis, Actinomyces naeslundii), had no effect on Enterococcus faecium population, the number of E. coli and other Enterobacteriaceae. Additionally, AgNPs had no negative effect on enterocytes of duodenal villi (Sawosz et al. Citation2007). Salem et al. (Citation2021) studied the antimicrobial activity of silver nanoparticles in broiler chickens experimentally infected with C. perfringens associated necrotic enteritis (NE). 30 µg/bird of AgNPs was administered to chickens for 5 successive days post infection via crop gavage. AgNPs reduced the severity of clinical signs, mortalities, and pathological lesions in the intestine and liver as well as C. perfringens' colonization in the intestine and ceca in infected birds. However, their residues were detected in the birds’ muscles. Grodzik and Sawosz (Citation2006) showed that silver nanoparticles, injected into fertilized chicken eggs, did not influence the development of chicken embryos, but decreased the number and size of lymph follicles in the bursa of Fabricius. Further investigation on the application of nano-silver in the commercial poultry production, could lead to the development of feeding strategies for chickens aiming at reducing the use of antibiotics as growth promoters (Pineda et al. Citation2012).
Nevertheless, literature data also indicate the unfavorable effects of supplementing the diet of poultry with silver nanoparticles. Ahmadi and Rahimi (Citation2011) tested colloidal nano-silver added to drinking water of broilers at doses of 4, 8 and 12 mg/L. This nano-element had a negative effect on body weight, feed intake and feed conversion rate when compared with the control group. AgNPs decreased growth performance and economic traits in broilers. Similar negative effect was obtained by 50 mg/L in drinking water for broiler chickens in addition to impaired immune functions (Vadalasetty et al. Citation2018). 15 mg of AgNPs/L did not show either a significant growth promoting effect or conclusive coccidiostatic activity (Chauke and Siebrits Citation2012). Moreover, nano-silver accumulated in breast muscle, liver, femur and feces. In the work of Pineda et al. (Citation2012), silver nanoparticles were injected into fertile eggs, as well as added to drinking water during the post-hatch period. Such treatment reduced feed intake and body weight, decreased lactose-negative enterobacteria and lactic acid bacteria in the cecum but had no effect on the bacterial populations in the ileum and concentrations of IgG and IgM in plasma.
4.2.2. Pigs
Silver nanoparticles, supplied at doses 20 and 40 mg/kg of weanling pigs feed, increased linearly their daily growth with the increasing dose of metallic Ag, and reduced ileal concentration of coliforms, whereas lactobacilli remained unaffected. Feed intake was higher for a lower dose of AgNPs (Fondevila et al. Citation2009). Interesting properties of nano-silver were demonstrated in the study of Dung et al. (Citation2020). Silver nanoparticles exhibited antiviral ability against African swine fever virus, which is the cause of a highly contagious and fatal disease in domestic swine. Nano-silver at a dose of 25 mg/L significantly reduced the microbial contamination in the pig house and constitutes a promising disinfectant.
4.2.3. Sheep and goat
Farouk et al. (Citation2020) investigated in vitro antibacterial activity of silver nanoparticles against multidrug-resistant (MDR) Salmonella isolates recovered from diarrhoeic sheep and goats as well as assessed their in vivo treatment efficacy in mice against S. enteritidis. 4% and 3.6% of sheep and goats, respectively, were infected with MDR Salmonella isolates and all of these isolates were affected efficiently with AgNPS with MIC (minimum inhibitory concentration) of ≤0.02–0.313 μg/mL and MBC (minimum bactericidal concentration) of 0.078–1.250 μg/mL. In vivo, AgNPs reduced the number of viable S. enteritidis in feces of infected mice, that completely stopped between the 4th and 6th day of treatment, as well as AgNPs suppressed inflammatory reaction caused by S. enteritidis.
4.2.4. Rabbits
Abdelsalam et al. (Citation2019) tested subcutaneous injections of silver nanoparticles (0.5 and 1.0 mg AgNPs/kg body weight) twice a week for four consecutive weeks. Administered AgNPs had no significant effect on feed intake, feed conversion ratio and average daily gain. Rabbits supplemented with 0.5 mg of AgNPs showed lower concentration of total cholesterol and triglycerides in plasma than the control group, the lowest total antioxidant capacity and the highest concentration of malondialdehyde and glutathione peroxidase activity. Additionally, the accumulation of silver in blood plasma and meat increased with the increasing dose of AgNPs.
4.3. Copper (Cu) nanoparticles
Cu is an essential trace element in animals and an essential cofactor for several cellular enzymes. It can promote participation in hematopoiesis, bone formation, enhancement of immunity, increase in resistance to foreign pathogens and antioxidant capacity (Gangadoo et al. Citation2016). Furthermore, copper is involved in various physiological and biochemical processes (Gangadoo et al. Citation2016; Scott et al. Citation2018). The importance of Cu in the animal diet, physicochemical and biological properties of CuNPs (nutritional and physiological characteristics, antibacterial activity, immunological and toxicological effects) were described in reviews, for example by Scott et al. (Citation2018), Amlan and Lalhriatpuii (Citation2020). Copper nanoparticles supplemented to animal feed act as a growth promoter, immune stimulant, and antibacterial and antifungal agent (Morsy et al. Citation2021).
4.3.1. Poultry
Copper nanoparticles are being studied in many aspects in poultry nutrition. Aminullah et al. (Citation2021) compared the effect of different forms of copper supplemented to the diet of Swarnadhara breeder hens. It was found that the commonly used inorganic form of Cu (CuSO4) can be replaced with 50% of organic (Cu proteinate) or 25% of nano-form without negative impact on hens’ productivity, hatchability, progeny, and egg quality. Miroshnikov et al. (Citation2015) administered copper nanoparticles to broiler chickens by single intramuscular injection. Quick growth stimulation and metabolic changes were observed. Copper and protein concentration in serum was changed only 7 and 21 days after injection. Ognik et al. (Citation2016b) evaluated the effect of copper nanoparticles supplementation on the intestinal absorption of calcium, iron and zinc. Oral administration of copper nanoparticles (5, 10 and 15 mg/L) to Ross 308 chickens led to the accumulation of this element in the intestinal walls. For a dose of 15 mg/L of nano-copper, the concentration of Cu increased in plasma. It is supposed that copper accumulated in intestines can reduce calcium and zinc absorption but has no effect on iron absorption.
For poultry, in ovo application of copper nanoparticles has shown promising results. Mroczek-Sosnowska et al. (Citation2015b) demonstrated that in ovo administration of copper nanoparticles (0.3 mL of 50 mg/L) had a positive effect on broiler chickens’ performance (e.g., body weight) as compared to the control group. Group with nano-copper had significantly lower feed conversion rate and mortality and higher percentage of breast and leg muscles in the carcass when compared with the control group. Mroczek-Sosnowska et al. (Citation2017) evaluated nano-copper as an agent to minimize the problem of weak bones in broiler chickens, which is caused by a rapid body weight gain and imbalance between the increase in muscle mass and bone mass. Injection in ovo of copper nanoparticles at the beginning of embryogenesis resulted in a femoral bone, which was characterized by a higher weight and volume and by significantly greater resistance to fractures as compared to the control group. Additionally, in ovo application of copper nanoparticles at the stage of chicken embryo development poseses no threat of excessive copper accumulation in poultry organs like liver and spleen and muscles on the age at slaughter (Mroczek-Sosnowska et al. Citation2014).
Copper nanoparticles can also have a negative effect on poultry. Morsy et al. (Citation2021) found that CuO nanoparticles supplemented to the diet of broiler chickens caused severe pathological alterations of muscle and different edible organs (muscle, heart, liver, spleen, and kidneys) which was correlated with increasing Cu dose from low − 5 mg/kg to high dose − 15 mg/kg body weight. DNA fragmentation was caused due to oxidative stress. No effect on growth performance and immune status was observed. Additionally, decreases in antibody titer of Avian Influenza and New Castle viruses was noted, which can lead to decreased disease resistance.
4.3.2. Pigs
Gonzales-Eguia et al. (Citation2009) confirmed beneficial effects of copper nanoparticles (50 mg/kg) on weaned piglets, which improved microelement bioavailability and reduced its fecal excretion, increased crude fat digestibility, and enhanced growth performance as compared to the control group supplemented with CuSO4. Additionally, nano-copper supplementation increased the level of serum IgG, γ-globulin, and total globulin protein, as well as superoxide dismutase activity.
4.3.3. Rabbits
Copper nanoparticles supplemented to the diet of rabbits, improved feed conversion ratio, final body weight, performance index, increased activity of superoxide dismutase enzyme, enhanced intestinal microbiota (increase in the total bacterial count, whereas decrease in the population of ureolytic bacteria, Escherichia coli and Clostridium spp.) and increased hemoglobin content. Supplementation of CuNPs had no significant effect on Cu concentration in plasma and muscles, but the content of copper in liver and feces was significantly higher than in the control group (Refaie et al. Citation2015).
4.4. Gold (Au) nanoparticles
Gold nanoparticles are known to be used for various biomedical applications, such as biosensors, photothermal therapy, and drug delivery (Sembratowicz et al. Citation2016). There is little research into the use of gold nanoparticles in the veterinary medicine and livestock production, which is relatively innovative (Gangadoo et al. Citation2016; Hassanen et al. Citation2020). In the veterinary science, gold nanoparticles-based diagnostics is used for the enhanced detection of major pathogens and toxins in poultry and livestock (e.g., bacterial diseases like anthrax caused by Bacillus anthracis, brucellosis caused by Brucella spp., a viral disease – Newcastle disease, aflatoxicosis caused by aflatoxins produced by Aspergillus spp.) (Manhas et al. Citation2021).
The first experiments concern the use of gold nanoparticles in poultry nutrition. Sembratowicz et al. (Citation2016) showed that gold nanoparticles supplied to drinking water of Ross 308 chickens at doses of 5, 10 and 15 mg/L were accumulated in the intestinal walls in a dose- and time-dependent manner. In vitro experiments also indicated that nano-gold present in the jejunum had a negative influence on the iron, calcium and potassium absorption. This may be the result of inhibition of the activity of transport proteins, blocking ion channels, or causing inflammation of the intestines leading to destruction of the intestinal villi. Hassanen et al. (Citation2020) tested gold nanoparticles (5 and 15 mg/L of drinking water) on 90 one-day-old mixbred Cobb broiler chicken to evaluate their impact on growth performance, antioxidant levels and immune defense. Lower NPs dose had a positive effect on growth performance (average body weight and the feed conversion ratio) without any significant difference in immunological parameters, oxidative stress damage, pro-inflammatory cytokines, DNA fragmentation assay and histopathological organizations in some organs such as spleen, liver, bursa of fabricius, thymus, as compared with the control group.
4.5. Selenium (Se) nanoparticles
Selenium is known to play an important role in the antioxidant status of animals – it prevents oxidative stress (Sadeghian et al. Citation2012; Kojouri et al. Citation2020; Nabi et al. Citation2020; Han et al. Citation2021). Feed supplementation with selenium can improve not only the efficiency of the antioxidant system, but also can enhance disease resistance and nutritional quality of the livestock-based products (Amlan and Lalhriatpuii Citation2020; Shi et al. Citation2011a). Nano-selenium as a feed additive is mainly tested in poultry and ruminants. SeNPs administered to the poultry diet can enhance growth performance – feed conversion ratio, nutrient digestibility, growth rate, productivity, egg production, improve eggs and meat quality, increase microbial activity and gut microbial environment, and exert an antimicrobial activity (Nabi et al. Citation2020; Rana, Citation2021; Abdel-Moneim et al. Citation2022). In ruminants, nano-selenium can increase rumen fermentation and feed digestibility, enhance the body growth and weight gain, reduce oxidative stress and improve serum antioxidant enzymes (phospholipid hydroperoxide glutathione peroxidase, superoxide dismutase and catalase), improve the concentration of Se in serum, and maintain the animal’s reproductive physiology (Abdelnour et al. Citation2021). The role of selenium nanoparticles in animal feeding was described in detail by many researchers, for example Gangadoo et al. (Citation2016), Amlan and Lalhriatpuii (Citation2020), Nabi et al. (Citation2020), Rana (Citation2021), etc.
4.5.1. Poultry
Selenium supplementation to poultry diet increases its antioxidative and immune properties (El-Deep et al. Citation2016; Gangadoo et al. Citation2020; Nabi et al. Citation2020). Gangadoo et al. (Citation2018) demonstrated that selenium nanoparticles at a dose of 0.9 mg/kg of diet (among tested 0.3, 0.9 and 1.5 mg/kg) exhibited the best performance, improved gut health by increasing the population of beneficial bacteria, such as Lactobacillus and Faecalibacterium prausnitzii and short-chain fatty acids. El-Deep et al. (Citation2016) showed that the addition of 0.3 mg of nano-selenium per kg of broiler chickens diet kept under high ambient temperature alleviated its negative effects such as decrease in body weight gain, feed intake, feed conversion ratio, weight of breast muscle and abdominal fat. Reduction in the content of malondialdehyde in liver and breast muscle was observed and increase in the Se and vitamin E content in breast muscle in the nano-selenium group. Selenium retention in liver and muscle was increased in a dose-dependent manner by dietary intake of nano-Se (0.3, 05, 1, and 2 mg/kg diet), but did not affect growth performance. However, meat quality, immune function, and oxidation resistance were improved by nano-Se levels ranging from 0.3 to 1 mg/kg diet (Cai et al. Citation2012).
The impact of in ovo injection of 5 or 10 ppb of SeNPs were explored on physiological responses, immunological status and growth performance of broiler chickens post hatching that were later supplemented or not with 10 ppb SeNPs/kg ration for 5 weeks (Ibrahim et al. Citation2020). The tested parameters increased for both examined routes of SeNPs delivery. The in ovo injection of SeNPs, along with their diet supplementation had improved body weight, body weight gain and feed conversion ratio. In addition, both delivery routes decreased serum triglycerides and malondialdehyde content, while high-density lipoprotein cholesterol and glutathione content were reduced, whereas glutathione reductase activity and immunoglobulin status were increased.
Dietary supplementation of growing quail with 0.2, 0.4, and 0.6 g/kg of diet with SeNPs increased body weight gain, and feed conversion ratio, while feed intake decreased compared to the control group with the highest effect recorded for 0.4 g/kg of diet. They significantly improved the plasma lipid profile, antioxidant enzymes activity, immunoglobulin G values, blood biochemistry, and bacterial environment of the intestine of quail (Alagawany et al. Citation2021).
Layer chickens group treated with nano-selenium (0.3 mg/kg – among tested doses 0.075, 0.15, 0.3 and 0.6 mg/kg of diet) increased relative weight gain and final body weight as compared to sodium selenite. Nano-Se treated groups had also higher content of Se in breast muscle and liver, increased activity of antioxidant enzymes – glutathione peroxidase, erythrocyte catalase and superoxide dismutase, increased serum biochemical (e.g., glucose, total protein, albumin, globulin) and hematological parameters (hemoglobin, Total Erythrocyte Count (TEC), Packed Cell Volume (PCV)) and finally increased cellular and humoral immunity (Mohapatra et al. Citation2014c). In addition to the ability of SeNPs to scavenge 2,2′-azino-bis(3-ethylbenzothiazoline-6-sulfonic acid) (ABTS) and 2,2-diphenyl-1-picrylhydrazyl (DPPH) radicals in a dose-dependent manner in a trial performed by Abdel-Moneim et al. (Citation2022), they also possess considerable antimicrobial activity against Gram-positive, Gram-negative bacteria, Candida sp. and Aspergillus sp.
Feeding experiments on layer birds performed by Mohapatra et al. (Citation2014b) showed that nano-selenium at a dose of 0.3 mg/kg of diet significantly increased Se content in breast muscle, liver, pancreas and feathers as compared to inorganic form – sodium selenite. Additionally, nano-selenium improved body weight, feed consumption ratio, antioxidant status (activity of glutathione peroxidase, erythrocyte catalase and superoxide dismutase), as well as cellular and humoral immunity. In comparison to sodium selenite, Meng et al. (Citation2019) found that supplementing laying hens with nano-Se at 0.3 mg/kg of diet increased egg Se concentration and showed better retention in the body than the former. Qu et al. (2017) observed that SeNPs provided effective antioxidative protection against toxicity of laying hens with deoxynivalenol (DON). The supplementation of DON-contaminated basal diet (10 mg/kg DON) with 0.5 mg/kg SeNPs was able to improve glutathione peroxidase activity, total antioxidant status and egg production rate as well as decreased rate of soft-shelled or cracked eggs by increasing blood calcium level.
4.5.2. Ruminants
Selenium nanoparticles can be also supplemented to the diet of sheep. Nano-selenium administered to the sheep diet, increased the resistance and defensive ability of the intrinsic immunity, as well as the neutrophil counts. Moreover, selenium nanoparticles showed better antioxidative effect than sodium selenite and were found to be less toxic and more bioactive than selenite (Sadeghian et al. Citation2012). In the work of Shi et al. (Citation2011a), supplementation of nano-selenium to the basal diet of sheep improved feed utilization and rumen fermentation. The nutrient digestibility was higher for SeNPs supplemented at a dose of 0.3, 3 g/kg of diet than in the control group but lower for the dose of 6 g/kg of diet. With the increase of nano-selenium in the diet, ruminal volatile fatty acids concentration also increased. Nano-Se is also known to stimulate rumen microbial activity and enzyme activity. Supplementation of 4 g of nano-selenium (4 mg Se)/kg of diet in the work of Xun et al. (Citation2012) decreased ruminal pH, ammonia N concentration and increased the total ruminal volatile fatty acids. Additionally, nano-selenium improved ruminal fermentation and increased feed conversion efficiency when compared to yeast–selenium.
Kojouri et al. (Citation2020) tested nano-selenium in the diet of newborn lambs. SeNPs at a dose of 0.1 mg/kg of diet showed a significantly higher concentration of selenium in serum than for the control group, whereas the concentration of copper and zinc significantly decreased. SeNPs supplementation significantly improved the weight gain of lambs and increased the superoxide dismutase activity. The same effect was observed in the work of Yaghmaie et al. (Citation2017) – nano-selenium (0.10 mg/kg of diet) reduced the oxidative stress (enhanced activity of blood glutathione peroxidase) and increased the lamb's weight gain. Selenium compound had no effects on Cu, Zn and Fe concentration in blood.
Han et al. (Citation2021) showed that selenium nanoparticles supplemented to the diet of Holstein cows increased the concentration of Se in milk and activity of milk glutathione peroxidase when compared with sodium selenite, but there was no effect of selenium form on dry matter intake, as well as milk yield and composition.
4.5.3. Rabbits
Sheiha et al. (Citation2020) compared the effect of biologically and chemically synthesized selenium nanoparticles (25 and 50 mg/kg diets) on growth (daily body weight gain, and feed intake), carcass characteristics, oxidative (malondialdehyde, superoxide dismutase, glutathione, and catalase) and inflammatory parameters (interleukin 4, and interferon-gamma) of rabbits exposed to the thermal stress. Better results were observed for nano-silver produced by biological method. Additionally, these nanoparticles showed strong in vitro antibacterial activity against Bacillus cereus, Listeria monocytogenes, Staphylococcus aureus, Escherichia coli, Salmonella typhimurium, Klebsiella pneumoniae and Pseudomonas aeruginosa.
4.6. Calcium (Ca) nanoparticles
Calcium is a key element in the skeletal mineralization process (Matuszewski et al. Citation2020a). Reviews of Amlan and Lalhriatpuii (Citation2020) and Matuszewski et al. (Citation2020a) present examples on the effect of CaNPs on performance, immunity, and other biological traits in poultry. Since phosphorus is expensive among the mineral sources for poultry (broiler chickens), several studies proposed to replace its requirement as dicalcium phosphate by nano-form of calcium phosphate. Results from feeding experiments (body weight gain, and feed conversion ratio) showed that 50% dose of calcium phosphate nanoparticles can be used instead of the conventional 100% dose of dicalcium phosphate (Vijayakumar and Balakrishnan Citation2014; Samanta et al. Citation2019). Similarly, growth promoting effect was obtained by 1.75, 1.31, and 0.88% levels of calcium phosphate (Hassan et al. Citation2016), 0.12% levels of calcium phosphate-nanoparticles or supplementation of 2 to 10% of hydroxyapatite nanoparticles (Sohair et al. Citation2017) comparing to the control birds fed with conventional dicalcium phosphate (DCP). Nano-dicalcium phosphate supplemented to the diet of broilers, increased bone parameters such as tibia ash, Ca and P content when compared with conventional dicalcium phosphate (Mohamed et al. Citation2016).
Abo El-Maaty et al. (Citation2021) showed that biosynthesized with marine seaweed – Sargassum latifolium extract calcium nanoparticles had a positive effect on egg and shell weight of laying hens, increased the level of serum Ca and P when compared with the control group. Addition of Ca carbonate nanoparticles to drinking water (1 g/L) of layers produced more eggshell strength and freshness indices (Wang et al. Citation2017). Neverthless, the egg production rate and blood Ca level were decreased by replacing 4.03% of Ca carbonate by 2.02%, 1.01%, 0.25% Ca carbonate nanoparticles that may be attributed to too much reduction in the Ca level in the layer hen's diet (Ganjigohari et al. Citation2018).
4.7. Chromium (Cr) nanoparticles
In broilers exposed to heat stress, the favourable effects of chromium (III) picolinate nanoparticles (NCrPic) applied at doses 500, 1000, and 1500 ppb were explored on growth performance, stress-related hormonal changes, serum levels of different immune biomarkers, and IFN-gene expression (Hamidi et al. Citation2022). Accordig to findings of Hamidi and his co-authors, lower body weight, daily weight gain, daily feed intake due to heat stress, and feed conversion ratio were all significantly improved by supplementation of NCrPic. NCrPic significantly lowered stress-elevated cortisol and immunoglobulin concentrations as well as upregulated the upregulation of IFN-expression. Similarly, in quail broilers, supplementation of nano-Cr at 800 µg/kg diet was effective in reducing the negative effects of physiological stress induced by dexamethazone. The performance and hematological parameters were not affected with nanoparticles-chromium, but they increased meat oxidative stability in stressed birds by reducing malondialdehyde concentration in the thigh muscle (Yarmohammadi et al. Citation2020). Neither the growth performance of birds nor the rate of egg production was affected by supplementation of chromium nanoparticles. However, the meat and egg quality, as well as tissue retention of different minerals was improved by their adminstration (Sirirat et al. Citation2013; Sathyabama and Jagadeeswaran Citation2016; Sathyabama et al. Citation2017). The conflicting results obtained from studies conducted on farm animals may be due to the use of different quantities and forms of Cr, as well as to differences in the means and time of use, age and species of the birds (Farag et al. Citation2017; Ognik et al. Citation2020).
4.8. Manganese (Mn) nanoparticles
Manganese in animal and poultry is responsible for proper bone formation and several biochemical processes (Matuszewski et al. Citation2020b). This micronutrient is required as a cofactor and activator of many enzymes, like galactosyltransferase, glutamine synthetase, agmatinase, arginase, pyruvate carboxylase, and superoxide dismutase to ensure appropriate growth and development (Avila et al. Citation2013). After nano-MnSO4 supplementation, tibia bone parameters such as tibia length, volume, breaking strength, diameter, and bone weight have been improved (Patra and Lalhriatpuii 2020). Jankowski et al. (Citation2019) explored how different quantities of manganese (10, 50, and 100 mg/kg diet), either in the form of manganese oxide (MnO) or manganese nanoparticles (NPs-Mn2O3), affected growth performance, absorption, and accumulation of Mn, Zn, and Cu, antioxidant and immunological status in growing turkeys. The turkeys' development performance was not affected by reducing the amount of Mn in the feed from 100 to 50 or even 10 mg/kg, or by replacing MnO with NPs-Mn2O3. Interestingly, replacing MnO with NPs-Mn2O3 enhanced ileal Mn digestibility and reduced Cu buildup in the liver and breast muscle. Similar doses were used by Ognik et al. (Citation2019) – they showed that regardless the dosage of Mn in turkey's diets, either the form of manganese – MnO or NPs-Mn2O3, Mn induced lipid oxidation reactions to the greatest extent. NPs-Mn2O3 reduced protein nitration more than MnO. They also concluded that the reduction of the Mn level, regardless of the form used, showed negative effect regarding the antioxidant activity that may be weakened and consequently induce oxidative processes in the cell. In broiler chickens, the use of NanoMn2O3 had no negative impact on development and growth, but reduced the excretion of Mn (Matuszewski et al. Citation2020b).
5. Concerns about the use of nanoparticles in animal nutrition
As shown in the present review, the supplementation of metal-containing nanoparticles in animal diets could be a promising option in the future. Encouraging results from recent studies on animals are the driving force for further investigations (Scott et al. Citation2018). But the potential hazard of a long-term application of nanoparticles as feed additives and their toxicological data are still unknown. Before nanoparticles can be recommended in animal nutrition, their toxicity and safety margins should be evaluated in animals tested for a long time (Amlan and Lalhriatpuii Citation2020). The small size of nanoparticles can affect their toxicity through easier cellular uptake, translocation in the animal’s body and retention (Gatoo et al. Citation2014; Exbrayat et al. Citation2015; Muralisankar et al. Citation2016; Conine and Frost Citation2017; Hill and Li Citation2017; Scott et al. Citation2018; Kumar and Bhattacharya Citation2019; Youssef et al. Citation2019; Gangadoo et al. Citation2020; Bidian et al. Citation2021). For example, in vitro findings showed that silver nanoparticles cause apoptosis and oxidative stress in cow granulosa cells, as well as alter the pattern of steroid hormone synthesis, as a result AgNPs may decrease the function and viability of ovarian cells (CitationTabandeh et al. 2022). Another main concern is the accumulation of these nanoparticles in the internal organs of treated animals (e.g., kidneys, liver), as well as in animal-derived products such as meat (Fondevila et al. Citation2009; Abdelsalam et al. Citation2019; Kumar and Bhattacharya Citation2019; Naz et al. Citation2021), which may be toxic for human beings (Exbrayat et al. Citation2015; Abdelsalam et al. Citation2019). Feeding animals with metallic/metal oxide nanoparticles requires understanding their mechanism of action to ensure safety for animals as well as further food quality assurance tests to confirm its safety for people (Hill and Li Citation2017; Hassanen et al. Citation2020; Awaad et al. Citation2021). A great amount of research is still required to support the safety of metal-containing nanoparticles application in animal nutrition, avoiding any harm to livestock, the environment, and human beings (Exbrayat et al. Citation2015; Scott et al. Citation2018).
6. Conclusions
In the present review it was shown that metal-containing nanoparticles, produced by physical, chemical, or biological methods can be used as beneficial feed additives. The supplementation of animal diet with elements such as copper, silver, zinc, gold, selenium, chromium, or calcium in nano-form has a positive effect on livestock and poultry performance, productivity, and health (especially antioxidant status and immunity). Nano-elements also proved to have antimicrobial activities against viral, bacterial (including drug resistant bacterial strains), parasitic, and fungal pathogens as well as effects on mycotoxins. They are proposed as an alternative to antibiotic growth promoters. Due to the inhibition of pathogens, nanoparticles are responsible for the intestinal health improvement. One of the main advantages of nanoparticles is their small size, thanks to which smaller doses can be administered in the animal's diet instead of bulk inorganic salts. At the same time, it is possible to prevent the excessive accumulation of these metals in waste, which results in the reduction of environmental damage. Although nanoparticles have many advantages there are also some obstacles in using them in animals. Small size of the nano-scale elements relates to their higher toxicity. Accumulation of metal-containing nanoparticles can induce cell death or can cause pathological changes and damage to the whole organism. Therefore, further very careful and precise toxicity studies are necessary before determining an appropriate, safe dose of dietary supplementation for livestock.
Acknowledgments
This work was funded by a grant entitled: “Eco-friendly technologies for the management of seaweed biomass for products useful for sustainable agriculture and biosorbents used for the removal of heavy metal ions from the environment” (No. 2019/33/B/NZ9/01844) from the National Science Centre in Poland. All the authors acknowledge and thank their respective Institutes and Universities.
Funding
This compilation is a review article written by its authors and required no substantial funding to be stated.
Disclosure statement
All authors declare that there exist no commercial or financial relationships that could, in any way, lead to a potential conflict of interest.
References
- Abbas HS, Abou Baker DH, Ahmed EA. 2021. Cytotoxicity and antimicrobial efficiency of selenium nanoparticles biosynthesized by Spirulina platensis. Arch Microbiol. 203(2):523–532.
- Abbasi M, Dastar B, Afzali N, Shargh MS, Hashemi SR. 2017. Zinc requirements of Japanese quails (Coturnix coturnix japonica) by assessing dose-evaluating response of zinc oxide nano-particle supplementation. Poult Sci J. 5(2):131–143.
- Abbasi M, Dastar B, Afzali N, Shargh MS, Hashemi SR. 2022. The effects of nano and micro particle size of zinc oxide on performance, fertility, hatchability, and egg quality characteristics in laying Japanese quail. Biol Trace Element Res. 200:2338–2348.
- Abd El Megid ADA, Khaled M, Emam MA, Adel A. 2018. Biochemical role of zinc oxide and propolis nanoparticles in protection rabbits against coccidiosis. Benha Vet Med J. 34(1):314–328.
- Abdel-Moneim EA, El-Saadony MT, Shehata AM, Saad AM, Aldhumri SA, Ouda SM, Mesalam NM. 2022. Antioxidant and antimicrobial activities of Spirulina platensis extracts and biogenic selenium nanoparticles against selected pathogenic bacteria and fungi. Saudi J Biol Sci. 29(2):1197–1209
- Abdelnour SA, Alagawany M, Hashem NM, Farag MR, Alghamdi ES, Hassan FU, Bilal RM, Elnesr SS, Dawood MAO, Nagadi SA, et al. 2021. Nanominerals: fabrication methods, benefits and hazards, and their applications in ruminants with special reference to selenium and zinc nanoparticles. Animals. 11(7):1916.
- Abdelsalam M, Al-Homidan I, Ebeid T, Abou-Emera O, Mostafa M, Abd El-Razik M, Shehab-El-Deen M, Abdel Ghani S, Fathi M. 2019. Effect of silver nanoparticle administration on productive performance, blood parameters, antioxidative status, and silver residues in growing rabbits under hot climate. Animals. 9(10):845.
- Abdel-Wareth AAA, Hussein KRA, Ismail ZSH, Lohakare J. 2022. Effects of zinc oxide nanoparticles on the performance of broiler chickens under hot climatic conditions. Biol Trace Elem Res. doi: 10.1007/s12011-022-03095-9.
- Abdollahi M, Rezaei J, Fazaeli H. 2020. Performance, rumen fermentation, blood minerals, leukocyte and antioxidant capacity of young Holstein calves receiving high-surface ZnO instead of common ZnO. Arch Anim Nutr. 74(3):189–205.
- Abedini M, Shariatmadari F, Karimi Torshizi MA, Ahmadi H. 2018. Effects of zinc oxide nanoparticles on the egg quality, immune response, zinc retention, and blood parameters of laying hens in the late phase of production. J Anim Physiol Anim Nutr (Berl). Jun;102(3):736–745. doi: 10.1111/jpn.12871. Epub 2018 Feb 28. PMID: 29493020.
- Abo El-Maaty HA, El-Khateeb AY, Al-Khalaifah H, El. Hamed EA, Hamed S, El-Said EA, Mahrose KM, Metwally K, Mansour AM. 2021. Effects of ecofriendly synthesized calcium nanoparticles with biocompatible Sargassum latifolium algae extract supplementation on egg quality and scanning electron microscopy images of the eggshell of aged laying hens. Poult Sci. 100(2):675–684.
- Ahmadi F, Ebrahimnezgad Y, Maheri N. 2014. The effects of dietary zinc oxide nanoparticles on the antioxidant state and serum enzyme activity in broiler chickens during starter stage. Int J Biosci. 3:29–35.
- Ahmadi F, Kurdestany AH. 2010. The impact of silver nano particles on growth performance, lymphoid organs and oxidative stress indicators in broiler chicks. Glob Vet. 5(6):366–370.
- Ahmadi F, Rahimi F. 2011. The effect of different levels of nano silver on performance and retention of silver in edible tissues of broilers. World Appl Sci J. 12:1–4.
- Ahmadi M, Ahmadian A, Seidavi AR. 2018. Effect of different levels of nano-selenium on performance, blood parameters, immunity and carcass characteristics of broiler chickens. Poult Sci J. 6(1):99–108.
- Akhavan-Salamat H, Ghasemi H. 2019. Effect of different sources and contents of zinc on growth performance, carcass characteristics, humoral immunity and antioxidant status of broiler chickens exposed to high environmental temperatures. Livestock Sci. 223:76–83.
- Alagawany M, Farag MR, Dhama K, Patra A. 2018. Nutritional significance and health benefits of designer eggs. World’s Poult Sci J. 74(2):317–330.
- Alagawany M, Qattan SYA, Attia YA, El-Saadony MT, Elnesr SS, Mahmoud MA, Madkour MA, El-Hack ME, Reda FM. 2021. Use of chemical nano-selenium as an antibacterial and antifungal agent in quail diets and its effect on growth, carcasses, antioxidant, immunity and caecal microbes. Animals. 11(11):3027.
- Alagawany M, Abd El-Hack ME. 2021. Natural feed additives used in the poultry industry. Singapore: Bentham Science Publishers Pte. Ltd.
- Ali S, Masood S, Zaneb H, Faseeh-ur-Rehman H, Masood S, Khan MUR, Tahir SK. 2017. Supplementation of zinc oxide nanoparticles has beneficial effects on intestinal morphology in broiler chicken. Pak Vet J. 37(3):335–339.
- Aminullah N, Prabhu TM, Naik J, Suresh BN, Indresh HC. 2021. Performance of Swarnadhara breeder hens supplemented with reduced levels of different copper forms. Vet World. 14(5):1371–1379.
- Amlan P, Lalhriatpuii M. 2020. Progress and prospect of essential mineral nanoparticles in poultry nutrition and feeding – a review. Biol Trace Elem Res. 197(1):233–253.
- Aparna N, Karunakaran R, Parthiban M, Department of Animal Nutrition, Madras Veterinary College, Tamil Nadu Veterinary and Animal Sciences University, Chennai – 600007, Tamil Nadu, India. 2017. Effect of selenium nano particles on glutathione peroxidase mRNA gene expression in broiler chicken. Indian J Sci Technol. 10(32):1–5.
- Aparna N, Karunakaran R. 2016. Effect of selenium nanoparticles supplementation on oxidation resistance of broiler chicken. Indian J Sci Technol. 9(S1):1–5.
- Avila DS, Puntel RL, Aschner M. 2013. Manganese in health and disease. Met Ions Life Sci. 13:199–227.
- Awaad MHH, Moustafa KM, Zoulfakar SA, Elhalawany MS, Mohammed FF, El-Refay RM, Morsy EA. 2021. The role of silver nanoparticles in the reluctance of colisepticemia in broiler chickens. J Appl Poult Res. 30(2):100155.
- Ayech A, Josende ME, Ventura-Lim J, Ruas C, Gelesky MA, Ale A, Cazenave J, Galdopórpora JM, Desimon MF, Duart M, et al. 2020. Toxicity evaluation of nanocrystalline silver-impregnated coated dressing on the life cycle of worm Caenorhabditis elegans. Ecotoxicol Environ Saf. 197:110570.
- Badawi M, Ali M, Behairy A. 2017. Effects of zinc sources supplementation on performance of broiler chickens. J Am Sci. 13(7):35–43.
- Bagheri M, Golchin-Gelehdooni S, Mohamadi M, Tabidian A. 2015. Comparative effects of nano, mineral and organic selenium on growth performance, immunity responses and total antioxidant activity in broiler chickens. Int J Biol Pharm Allied Sci. 4:583–595.
- Bakhshizadeh S, Mirzaei Aghjehgheshlagh F, Taghizadeh A, Seifdavati J, Navidshad B. 2019. Effect of zinc sources on milk yield, milk composition and plasma concentration of metabolites in dairy cows. SA J Anim Sci. 49(5):884–891.
- Bąkowski M, Kiczorowska B, Samolińska W, Klebaniuk R, Lipiec A. 2018. Silver and zinc nanoparticles in animal nutrition – a review. Ann Anim Sci. 18(4):879–898.
- Bidian C, Filip GA, David L, Florea A, Moldovan B, Robu DP, Olteanu D, Radu T, Clichici S, Mitrea D, et al. 2021. The impact of silver nanoparticles phytosynthesized with Viburnum opulus L. extract on the ultrastrastructure and cell death in the testis of offspring rats. Food Chem Toxicol. 150:112053.
- Bunglavan SJ, Garg AK, Das RS, Sameer S. 2014. Use of nanoparticles as feed additives to improve digestion and absorption in livestock. Livestock Res Int. 2(3):36–47.
- Cai SJ, Wu CX, Gong LM, Song T, Wu H, Zhang LY. 2012. Effects of nanoselenium on performance, meat quality, immune function, oxidation resistance, and tissue selenium content in broilers. Poult Sci. 91(10):2532–2539.
- Chauke N, Siebrits FK. 2012. Evaluation of silver nanoparticles as a possible coccidiostat in broiler production. Peer reviewed paper: Proc. 44th Congress of the South African Society for Animal Science. South Afr J Anim Sci. 42:493–497.
- Conine AL, Frost PC. 2017. Variable toxicity of silver nanoparticles to Daphnia magna: effects of algal particles and animal nutrition. Ecotoxicology. 26(1):118–126.
- Cufadar Y, Göçmen R, Kanbur G, Yıldırım B. 2020. Effects of dietary different levels of nano, organic and inorganic zinc sources on performance, eggshell quality, bone mechanical parameters and mineral contents of the tibia, liver, serum and excreta in laying hens. Biol Trace Elem Res. 193(1):241–251.
- Cui Y, Tian Z, Lu H, Deng D, Liu Z, Rong T, Yu M, Ma X. 2021. Zinc oxide nanoparticles improve gut health and reduce faecal zinc excretion in piglets. Livestock Sci. 251:104610.
- Dawood MAO, Basuini MFE, Yilmaz S, Abdel-Latif HMR, Kari ZA, Abdul Razab MKA, Ahmed HA, Alagawany M, Gewaily MS. 2021. Selenium nanoparticles as a natural antioxidant and metabolic regulator in aquaculture: a review. Antioxidants. 10(9):1364.
- Dobrzanski Z, Zygadlik K, Patkowska-Sokola B, Nowakowski P, Janczak M, Sobczak A, Bodkowski R. 2010. The effectiveness of nanosilver and mineral sorbents in the reduction of ammonia emissions from livestock manure. Przem Chem. 4:348–351 (in Polish).
- Dung TTN, Nam VN, Nhan TT, Ngoc TTB, Minh LQ, Nga BTT, Le VP, Quang DV. 2020. Silver nanoparticles as potential antiviral agents against African swine fever virus. Mater Res Expr. 6(12):1250g9.
- El Basuini MF, El-Hais AM, Dawood MAO, Abou-Zeid AE-S, El-Damrawy SZ, Khalafalla M-S, Koshio S, Ishikawa M, Dossou S. 2017. Effects of dietary copper nanoparticles and vitamin C supplementations on growth performance, immune response and stress resistance of red sea bream Pagrus major. Aquat Nutr. 2017:1–12.
- El-Deep MH, Ijiri D, Ebeid TA, Ohtsuka A. 2016. Effects of dietary nano-selenium supplementation on growth performance, antioxidative status, and immunity in broiler chickens under thermoneutral and high ambient temperature conditions. J Poult Sci. 53(4):274–283.
- El-Katcha MI, Soltan MA, Arafa MM, Kawarei ESR. 2018. Impact of dietary replacement of inorganic zinc by organic or nano sources on productive performance, immune response and some blood biochemical constituents of laying hens. Alex J Vet Sci. 59(1):48–59.
- Elkloub K, Moustafa ME, Ghazalah AA, Rehan A. 2015. Effect of dietary nanosilver on broiler performance. Int J Poultry Sci. 14(3):177–182.
- El-Maddawy ZK, El-sawy A-EF, Ashoura NR, Aboelenin SM, Soliman MM, Ellakany HF, Elbestawy AR, El-Shall NA. 2022. Use of zinc oxide nanoparticles as anticoccidial agents in broiler chickens along with its impact on growth performance, antioxidant status, and hematobiochemical profile. Life. 12(1):74.
- Eskandani M, Janmohammadi H, Mirghelenj SA, Ebrahimi M, Kalanaky S. 2021. Effects of zinc nanoparticles on growth performance, carcass characteristics, immunity, and meat quality of broiler chickens. Iran J Appl Anim Sci. 11(1):135–146.
- Eszenyi P, Sztrik A, Babka B, Prokisch J. 2011. Elemental, nano-sized (100-500 nm) selenium production by probiotic lactic acid bacteria. IJBBB. 1(2):148–152.
- Exbrayat JM, Moudilou EN, Lapied E. 2015. Harmful effects of nanoparticles on animals. J Nanotechnol. 2015:1–10.
- Farag MR, Alagawany M, Abd El-Hac ME, Arif M, Ayasan T, Dhama K, Patra A, Karthik K. 2017. Role of chromium in poultry nutrition and health: beneficial applications and toxic effects. Int J Pharmacol. 13(7):907–915.
- Farouk MM, El-Molla A, Salib FA, Soliman YA, Shaalan M. 2020. The role of silver nanoparticles in a treatment approach for multidrug-resistant Salmonella species isolates. Int J Nanomed. 15:6993–7011.
- Fondevila M, Herrer R, Casallas MC, Abecia L, Ducha JJ. 2009. Silver nanoparticles as a potential antimicrobial additive for weaned pigs. Anim Feed Sci Technol. 150(3–4):259–269.
- Fondevila M. 2010. Potential use of silver nanoparticles as an additive in animal feeding. In Perez DP, editor. Silver nanoparticles. London, UK: IntechOpen; p. 325–334.
- Gangadoo S, Dinev I, Willson NL, Moore RJ, Chapman J, Stanley D. 2020. Nanoparticles of selenium as high bioavailable and non-toxic supplement alternatives for broiler chickens. Environ Sci Pollut Res Int. 27(14):16159–16166.
- Gangadoo S, Dinev I, Chapman J, Hughes RJ, Van TTH, Moore RJ, Stanley D. 2018. Selenium nanoparticles in poultry feed modify gut microbiota and increase abundance of Faecalibacterium prausnitzii. Appl Microbiol Biotechnol. 102(3):1455–1466.
- Gangadoo S, Stanley D, Hughes RJ, Moore RJ, Chapman J. 2016. Nanoparticles in feed: progress and prospects in poultry research. Trends Food Sci Technol. 58:115–126.
- Ganjigohari S, Ziaei N, Ramzani Ghara A, Tasharrofi S. 2018. Effects of nanocalcium carbonate on egg production performance and plasma calcium of laying hens. J Anim Physiol Anim Nutr. 102(1):e225–e232.
- Gatoo MA, Naseem S, Arfat MY, Mahmood Dar A, Qasim K, Zubair S. 2014. Physicochemical properties of nanomaterials: implication in associated toxic manifestations. Biomed Res Int. 2014:498420–498428.
- Gong N, Shao K, Li G, Sun Y. 2016. Acute and chronic toxicity of nickel oxide nanoparticles to Daphnia magna: the influence of algal enrichment. NanoImpact. 3–4:104–109.
- Gonzales-Eguia A, Fu C-M, Lu F-Y, Lien T-F. 2009. Effects of nanocopper on copper availability and nutrients digestibility, growth performance and serum traits of piglets. Livestock Sci. 126(1–3):122–129.
- Gopi M, Pearlin B, Kumar RD, Shanmathy M, Prabakar G. 2017. Role of nanoparticles in animal and poultry nutrition: modes of action and applications in formulating feed additives and food processing. Int J Pharmacol. 13(7):724–731.
- Górniak W, Popiela E, Szuba-Trznadel A, Konkol D, Korczyński M. 2022. Smart feed additives for livestock. In: Chojnacka K, Saeid A, editors. Smart agrochemicals for sustainable agriculture, vol. 5. New York: Academic Press; p. 103–138.
- Grodzik M, Sawosz E. 2006. The influence of silver nanoparticles on chicken embryo development and bursa of Fabricius morphology. J Anim Feed Sci. 15(Suppl. 1):111–114.
- Gutierrez MF, Ale A, Andrade V, Bacchetta C, Rossi A, Cazenave J. 2021. Metallic, metal oxide, and metalloid nanoparticles toxic effects on freshwater microcrustaceans: an update and basis for the use of new test species. Water Environ Res. 93(11):2505–2526.
- Hafez A, Hegazi SM, Bakr AA, Shishtawy HEL. 2017. Effect of zinc oxide nanoparticles on growth performance and absorptive capacity of the intestinal villi in broiler chickens. Life Sci J. 14(11):125–129.
- Hafez A, Nassef E, Fahmy M, Elsabagh M, Bakr A, Hegazi E. 2020. Impact of dietary nano-zinc oxide on immune response and antioxidant defense of broiler chickens. Environ Sci Pollut Res Int. 27(16):19108–19114.
- Hamidi O, Chamani M, Ghahri H, Sadeghi AA, Malekinejad H, Palangi V. 2022. Effects of supplemental chromium nanoparticles on IFN-γ expression of heat stress broilers. Biol Trace Elem Res. 200(1):339–347.
- Han L, Pang K, Fu T, Phillips CJC, Gao T. 2021. Nano-selenium supplementation increases selenoprotein (Sel) gene expression profiles and milk selenium concentration in lactating dairy cows. Biol Trace Elem Res. 199(1):113–119.
- Hassan AA, El-Mokhtar NM, El-Hamaky AM. 2017. Evaluation of the efficacy of ozone fumigation and zinc oxide nanoparticles in control of aflatoxins contamination in cattle feeds. Anim Health Res J. 5:165–180.
- Hassan AA, Howayda ME, Mahmoud HH. 2013. Effect of zinc oxide nanoparticles on the growth of mycotoxigenic mould. Stud Chem Process Technol. 1:66–74.
- Hassan H, Samy A, El-Sherbin AE, Mohamed MA, Abd-Elsame MO. 2016. Application of nano-dicalcium phosphate in broiler nutrition: performance and excreted calcium and phosphorus. Asian J Anim Vet Adv. 11(8):477–483.
- Hassan RA, Soliman ES, Hamad RT, El-Borady OM, Ali AA, Helal MS. 2020. Selenium and nano-selenium ameliorations in two breeds of broiler chickens exposed to heat stress. SA J Anim Sci. 50(2):215–232.
- Hassanen EI, Morsy EA, Hussien AM, Ibrahim MA, Farroh KY. 2020. The effect of different concentrations of gold nanoparticles on growth performance, toxicopathological and immunological parameters of broiler chickens. Biosci Rep. 40:BSR20194296.
- Hidayat C, Sumiati S, Jayanegara A, Wina E. 2021. Supplementation of dietary nano Zn-phytogenic on performance, antioxidant activity, and population of intestinal pathogenic bacteria in broiler chicken. Trop Anim Sci J. 44(1):90–99.
- Hill EK, Li J. 2017. Current and future prospects for nanotechnology in animal production. J Anim Sci Biotechnol. 8:26.
- Hozyen HF, Ibrahim ES, Khairy EA, El-Dek SI. 2019. Enhanced antibacterial activity of capped zinc oxide nanoparticles: a step towards the control of clinical bovine mastitis. Vet World. 12(8):1225–1232.
- Hu CH, Li YL, Xiong L, Zhang HM, Song J, Xia MS. 2012. Comparative effects of nano elemental selenium and sodium selenite on selenium retention in broiler chickens. Anim Feed Sci Technol. 177(3–4):204–210.
- Ibrahim D, Ali HA, El-Mandrawy SA. 2017. Effects of different zinc sources on performance, bio distribution of minerals and expression of genes related to metabolism of broiler chickens. Zag Vet J. 45(3):292–304.
- Ibrahim NS, Sabic EM, Wakwak MM, El-Wardany IE, El-Homosany YM, Mohammad NE-D. 2020. In-ovo and dietary supplementation of selenium nano-particles influence physiological responses, immunological status and performance of broiler chicks. J Anim Feed Sci. 29(1):46–58.
- Jankowski J, Ognik K, Stępniowsk A, Zduńczyk Z, Kozłowski K. 2019. The effect of the source and dose of manganese on the performance, digestibility and distribution of selected minerals, redox, and immune status of turkeys. Poultry Sci. 98(3):1379–1389.
- Jose N, Elangovan AV, Awachat VB, Shet D, Ghosh J, David CG. 2018. Response of in ovo administration of zinc on egg hatchability and immune response of commercial broiler chicken. J Anim Physiol Anim Nutr (Berl). 102(2):591–595.
- Joshua PP, Valli C, Balakrishnan V. 2016. Effect of in ovo supplementation of nano forms of zinc, copper, and selenium on post-hatch performance of broiler chicken. Vet World. 9(3):287–294.
- Jóźwik A, Marchewka J, Strzałkowska N, Horbańczuk J, Szumacher-Strabel M, Cieślak A, Lipińska-Palka P, Józefiak D, Kamińska A, Atanasov A. 2018. The effect of different levels of Cu, Zn and Mn nanoparticles in hen Turkey diet on the activity of aminopeptidases. Molecules. 23(5):1150.
- Kazaz S, Hafez M. 2020. Evaluation of copper nanoparticles and copper sulfate effect on immune status, behavior, and productive performance of broilers. J Adv Vet Anim Res. 7(1):16–25.
- Kesmati M, Konani M, Torabi M, Khajehpour L. 2016. Magnesium oxide nanoparticles reduce anxiety induced by morphine withdrawal in adult male mice. Physiol Pharmacol. 20:197–205.
- Kim JS, Kuk E, Yu KN, Kim JH, Park SJ, Lee HJ, Kim SH, Park YK, Park YH, Hwang CY, et al. 2007. Antimicrobial effects of silver nanoparticles. Nanomedicine. 3(1):95–101.
- Kociova S, Dolezelikova K, Horky P, Skalickova S, Baholet D, Bozdechova L, Vaclavkova E, Belkova J, Nevrkla P, Skladanka J, et al. 2020. Zinc phosphate-based nanoparticles as alternatives to zinc oxide in diet of weaned piglets. J Anim Sci Biotechnol. 11(1):59.
- Kociova S, Valeckova V, Bytesnikova Z, Horky P, Kopel P, Adam V, Smerkova K. 2019. In vivo antibacterial study of zinc phosphate-based nanoparticles. NANOCON 2018 – Conference Proceedings, 10th Anniversary International Conference on Nanomaterials – Research and Application; p. 511–516.
- Kojouri G, Arbabi F, Mohebbi A. 2020. The effects of selenium nanoparticles (SeNPs) on oxidant and antioxidant activities and neonatal lamb weight gain pattern. Comp Clin Pathol. 29(2):369–374.
- Konieczka P, Szkopek D, Kinsner M, Fotschki B, Juśkiewicz J, Banach J. 2020. Cannabis-derived cannabidiol and nanoselenium improve gut barrier function and affect bacterial enzyme activity in chickens subjected to C. perfringens challenge. Vet Res. 51(1):141.
- Kumar I, Bhattacharya J. 2019. Assessment of the role of silver nanoparticles in reducing poultry mortality, risk and economic benefits. Appl Nanosci. 9(6):1293–1307.
- Leng D, Li Y, Zhu J, Liang R, Zhang C, Zhou Y, Li M, Wang Y, Rong D, Wu D, et al. 2020. The antibiofilm activity and mechanism of nanosilver- and nanozinc-incorporated mesoporous calcium-silicate nanoparticles. Int J Nanomed. 15:3921–3936.
- Li M, Czymmek KJ, Huang CP. 2011. Responses of Ceriodaphnia dubia to TiO2 and Al2O3 nanoparticles: a dynamic nano-toxicity assessment of energy budget distribution. J Hazard Mater. 187(1–3):502–508.
- Li Y, Xie S, Xu D, Shu G, Wang X. 2021. Antibacterial activity of ZnO quantum dots and its protective effects of chicks infected with Salmonella pullorum. Nanotechnology. 32(50):505104.
- Mahmoud UT, Darwish MHA, Ali FAZ, Amen OA, Mahmoud MAM, Ahmed OB, El-Reda GA, Osman MA, Othman AA, Abushahba MFN, et al. 2021. Zinc oxide nanoparticles prevent multidrug resistant Staphylococcus-induced footpad dermatitis in broilers. Avian Pathol. 50(3):214–226.
- Manhas PK, Quintela IA, Wu VCH. 2021. Enhanced detection of major pathogens and toxins in poultry and livestock with zoonotic risks using nanomaterials-based diagnostics. Front Vet Sci. 8:673718.
- Matuszewski A, Łukasiewicz M, Niemiec J. 2020a. Calcium and phosphorus and their nanoparticle forms in poultry nutrition. World's Poult Sci J. 76(2):328–345.
- Matuszewski A, Łukasiewicz M, Łozicki A, Niemiec J, Zielińska-Górska M, Scott A, Chwalibog A, Sawosz E. 2020b. The effect of manganese oxide nanoparticles on chicken growth and manganese content in excreta. Anim Feed Sci Technol. 268:114597.
- Mazaheri N, Naghsh N, Karimi A, Salavati H. 2019. In vivo toxicity investigation of magnesium oxide nanoparticles in rat for environmental and biomedical applications. Iran J Biotech. 17(1):e1543–9.
- Mekonnen G. 2021. Review on application of nanotechnology in animal health and production. J Nanomed Nanotech. 12:559.
- Meng T, Liu Y, Xie C, Zhang B, Huang Y, Zhang Y, Yao Y, Huang R, Wu X. 2019. Effects of different selenium sources on laying performance, egg selenium concentration, and antioxidant capacity in laying hens. Biol Trace Elem Res. 189(2):548–555.
- Miroshnikov S, Yausheva E, Sizova E, Miroshnikova E. 2015. Comparative assessment of effect of copper nano- and microparticles in chicken. Orient J Chem. 31(4):2327–2336.
- Mohamed MA, Hassan H, Samy A, Abd-Elsame MO, El-Sherbin AE. 2016. Carcass characteristics and bone measurements of broilers fed nano dicalcium phosphate containing diets. Asian J Anim Vet Adv. 11(8):484–490.
- Mohammadi F, Ahmadi F, Andi AM. 2015. Effect of zinc oxide nanoparticles on carcass parameters, relative weight of digestive and lymphoid organs of broiler fed wet diet during the starter period. Int J Biosci. 6(2):389–394.
- Mohapatra P, Swain RK, Mishra SK, Behera T, Swain P, Mishra SS, Behura NC, Sabat SC, Sethy K, Dhama K, et al. 2014c. Effects of dietary nano-Se on tissue Se deposition antioxidant status and immune functions in layer chicks. Int J Pharm. 10(3):160–167.
- Mohapatra P, Swain RK, Mishra SK, Behera T, Swain P, Behura NC, Sahoo G, Sethy K, Bhol BP, Dhama K. 2014b. Effects of dietary nano-selenium supplementation on the performance of layer grower birds. Asian J Anim Vet Adv. 9(10):641–652.
- Mohapatra P, Swain RK, Mishra SK, Behera T, Swain P, Mishra SS, Behura NC, Sabat SC, Sethy K, Dhama K, et al. 2014a. Effects of dietary nano-selenium on tissue selenium deposition, antioxidant status and immune functions in layer chicks. Int J Pharmacol. 10(3):160–167.
- Morsy EA, Hussien AM, Ibrahim MA, Farroh KY, Hassanen EI. 2021. Cytotoxicity and genotoxicity of copper oxide nanoparticles in chickens. Biol Trace Elem Res.199(12):4731–4745.
- Mroczek-Sosnowska N, Łukasiewicz M, Adamek D, Kamaszewski M, Niemiec J, Wnuk-Gnich A, Scott A, Chwalibog A, Sawosz E. 2017. Effect of copper nanoparticles administered in ovo on the activity of proliferating cells and on the resistance of femoral bones in broiler chickens. Arch Anim Nutr. 71(4):327–332.
- Mroczek-Sosnowska N, Łukasiewicz M, Wnuk A, Sawosz E, Niemiec J, Skot A, Jaworski S, Chwalibog A. 2015b. In ovo administration of copper nanoparticles and copper sulfate positively influences chicken performance: effect of Cu on chicken performance. J Sci Food Agric. 96(9):3058–3062.
- Mroczek-Sosnowska N, Łukasiewicz M, Wnuk A, Sawosz E, Niemiec J. 2014. Effect of copper nanoparticles and copper sulfate administered in ovo on copper content in breast muscle, liver and spleen of broiler chickens. Ann Warsaw Univ Life Sci – SGGW Anim Sci. 53:135–142.
- Mroczek-Sosnowska N, Sawosz E, Vadalasetty K, Łukasiewicz M, Niemiec J, Wierzbicki M, Kutwin M, Jaworski S, Chwalibog A. 2015a. Nanoparticles of copper stimulate angiogenesis at systemic and molecular level. Int J Mol Sci. 16(3):4838–4849.
- Muralisankar T, Saravana Bhavan P, Radhakrishnan S, Seenivasan C, Srinivasan V. 2016. The effect of copper nanoparticles supplementation on freshwater prawn Macrobrachium rosenbergii post larvae. J Trace Elem Med Biol. 34:39–49.
- Nabi F, Arain MA, Hassan F, Umar M, Rajput N, Alagawany M, Syed SF, Soomro J, Somroo F, Liu J. 2020. Nutraceutical role of selenium nanoparticles in poultry nutrition: a review. World's Poult Sci J. 76(3):459–471.
- Naz S, Nasir B, Ali H, Zia M. 2021. Comparative toxicity of green and chemically synthesized CuO NPs during pregnancy and lactation in rats and offspring: part I – hepatotoxicity. Chemosphere. 266:128945.
- Niemiec T, Łozicki A, Pietrasik R, Pawęta S, Rygało-Galewska A, Matusiewicz M, Zglińska K. 2021. Impact of Ag nanoparticles (AgNPs) and multimicrobial preparation (EM) on the carcass, mineral, and fatty acid composition of Cornu aspersum aspersum snails. Animals. 11(7):1926.
- Ognik K, Kozłowski K, Stępniowska A, Szlązak R, Tutaj K, Zduńczyk Z, Jankowski J. 2019. The effect of manganese nanoparticles on performance, redox reactions and epigenetic changes in turkey tissues. Animal. 13(6):1137–1144.
- Ognik K, Sembratowicz I, Cholewińska E, Wlazło Ł, Nowakowicz-Dębek B, Szlązak R, Tutaj K. 2016a. The effect of chemically-synthesized silver nanoparticles on performance and the histology and microbiological profile of the jejunum in chickens. Ann Anim Sci. 16(2):439–450.
- Ognik K, Stępniowska A, Cholewińska E, Kozłowski K. 2016b. The effect of administration of copper nanoparticles to chickens in drinking water on estimated intestinal absorption of iron, zinc, and calcium. Poult Sci. 95(9):2045–2051.
- Ognik K, Drażbo A, Stępniowska A, Kozłowski K, Listos P, Jankowski J. 2020. The effect of chromium nanoparticles and chromium picolinate in broiler chicken diet on the performance, redox status and tissue histology. Anim Feed Sci Technol. 259:114326.
- Olgun O, Yildiz AÖ. 2017. Effects of dietary supplementation of inorganic, organic or nano zinc forms on performance, eggshell quality, and bone characteristics in laying hens. Ann Anim Sci. 17(2):463–476.
- Othman AA, Osman MA, Abd-Elrahim AG. 2018. The effect of milling time on structural, optical and photoluminescence properties of ZnO nanocrystals. Optik. 156:161–168.
- Ouyang Z, Ren P, Zheng D, Huang L, Wei T, Yang C, Kong X, Yin Y, He S, He Q. 2021. Hydrothermal synthesis of a new porous zinc oxide and its antimicrobial evaluation in weanling piglets. Livestock Sci. 248:104499.
- Pathak SS, Reddy KV, Prasoon S. 2016. Influence of different sources of zinc on growth performance of dual purpose chicken. J Bio Innov. 5:663–672.
- Pilaquinga F, Cardenas S, Vela D, Jara E, Morey J, Gutiérrez-Coronado JL, Debut A, de las Nievas Piña M. 2021. Fertility and iron bioaccumulation in Drosophila melanogaster fed with magnetite nanoparticles using a validated method. Molecules. 26(9):2808.
- Pineda L, Sawosz E, Lauridsen C, Engberg RM, Elnif J, Hotowy A, Sawosz F, Chwalibog A. 2012. Inluence of in ovo injection and subsequent provision of silver nanoparticles on growth performance, microbial profile, and immune status of broiler chickens. Open Access Anim Physiol. 4:1–8.
- Prasad M, Lambe UP, Brar B, Shah I, Manimegalai J, Ranjan K, Rao R, Kumar S, Mahant S, Khurana SK, et al. 2018. Nanotherapeutics: an insight into healthcare and multi-dimensional applications in medical sector of the modern world. Biomed Pharmacother. 97:1521–1537.
- Prasoon S, Jayanaik Malathi V, Nagaraj CS, Narayanaswamy HD. 2018. Effects of dietary supplementation of inorganic, organic and nano selenium on meat production and meat quality parameters of a dual purpose crossbred chicken. Int J Agr Sci. 10(15):6788–6792.
- Qu W, Yang J, Sun Z, Zhang R, Zhou F, Zhang K, Xia Y, Huang K, Miao D. 2017 Effect of Selenium Nanoparticles on Anti- Oxidative Level, Egg Production and Quality and Blood Parameter of Laying Hens Exposed to Deoxynivalenol J Anim Res Nutr. 2:1. doi: 10.21767/2572-5459.100021
- Ramiah SK, Atta Awad E, Mookiah S, Idrus Z. 2019. Effects of zinc oxide nanoparticles on growth performance and concentrations of malondialdehyde, zinc in tissues, and corticosterone in broiler chickens under heat stress conditions. Poult Sci. 98(9):3828–3838.
- Rana T. 2021. Nano-selenium on reproduction and immunocompetence: an emerging progress and prospect in the productivity of poultry research. Trop Anim Health Prod. 53(2):324.
- Reda FM, El-Saadony MT, El-Rayes TK, Attia AI, El-Sayed SAA, Ahmed SYA, Madkour M, Alagawany M. 2021. Use of biological nano zinc as a feed additive in quail nutrition: biosynthesis, antimicrobial activity and its effect on growth, feed utilisation, blood metabolites and intestinal microbiota. Italian J Anim Sci. 20(1):324–335.
- Refaie A, Ghazal M, Barakat S, Morsy W, Meshreky S, Younan G, Eisa W. 2015. Nano-copper as a new growth promoter in the diet of growing New Zealand white rabbits. Egypt J Rabbit Sci. 25(1):39–57.
- Sadeghian S, Kojouri GA, Mohebbi A. 2012. Nanoparticles of selenium as species with stronger physiological effects in sheep in comparison with sodium selenite. Biol Trace Elem Res. 146(3):302–308.
- Saeed M, Babazadeh D, Naveed M, Alagawany M, Abd El-Hack ME, Arain MA, Tiwari R, Sachan S, Karthik K, Dhama K, et al. 2019. In ovo delivery of various biological supplements, vaccines and drugs in poultry: current knowledge. J Sci Food Agric. 99(8):3727–3739.
- Sagar DP, Mandal AB, Akbar N, Dinani OP. 2018. Effect of different levels and sources of zinc on growth performance and immunity of broiler chicken during summer. Int J Curr Microbiol App Sci. 7(05):459–471.
- Sahoo A, Swain RK, Mishra SK. 2014. Effect of inorganic, organic and nano zinc supplemented diets on bioavailability and immunity status of broilers. Int J Adv Res. 2(11):828–837.
- Salem HM, Ismael E, Shaalan M. 2021. Evaluation of the effects of silver nanoparticles against experimentally induced necrotic enteritis in broiler chickens. Int J Nanomed. 16:6783–6796.
- Samanta G, Mishra SK, Behura NC, Sahoo G, Behera K, Swain RK, Sethy K, Biswal S, Sahoo N. 2019. Studies on utilization of calcium phosphate nano particles as source of phosphorus in broilers. Anim Nutr Feed Tech. 19(1):77–88.
- Sanati M, Khodagholi F, Aminyavari S, Ghasemi F, Gholami M, Kebriaeezadeh A, Sabzevari O, Hajipour MJ, Imani M, Mahmoudi M, et al. 2019. Impact of gold nanoparticles on amyloid β-induced Alzheimer's disease in a rat animal model: involvement of STIM proteins . ACS Chem Neurosci. 10(5):2299–2309.
- Sarvestani S, Rezvani MR, Zamiri MJ, Shekarforoush S, Atashi H, Mosleh N. 2016. The effect of nanocopper and mannan oligosaccharide supplementation on nutrient digestibility and performance in broiler chickens. J Vet Res. 71:153–161.
- Sathyabama T, Jagadeeswaran A. 2016. Effect of chromium supplementation on performance, mineral retention and tissue mineral accumulation in layer chickens. J Anim Res. 6(6):989–994.
- Sathyabama T, Nanjappan K, Jagadeeswaran A, Kirubakaran A. 2017. Effect of chromium supplementation on external egg quality characteristic of layer chickens. Indian Vet J. 94:9–10.
- Sawosz E, Binek M, Grodzik M, Zielińska M, Sysa P, Szmidt M, Niemiec T, Chwalibog A. 2007. Influence of hydrocolloidal silver nanoparticles on gastrointestinal microflora and morphology of enterocytes of quails. Arch Anim Nutr. 61(6):444–451.
- Scott A, Vadalasetty KP, Sawosz E, Łukasiewicz M, Vadalasetty RKP, Jaworski S, Chwalibog A. 2016. Effect of copper nanoparticles and copper sulphate on metabolic rate and development of broiler embryos. Anim Feed Sci Technol. 220:151–158.
- Scott A, Vadalasetty KP, Chwalibog A, Sawosz E. 2018. Copper nanoparticles as an alternative feed additive in poultry diet: a review. Nanotechnol Rev. 7(1):69–93.
- Selim NA, Radwan NL, Youssef SF, Salah Eldin TA, A, Elwafa S. 2015a. Effect of inclusion inorganic, organic or nano selenium forms in broiler diets on: growth performance, carcass and meat characteristics. Int J Poultry Sci. 14(3):135–143.
- Selim NA, Radwan NL, Youssef SF, Salah Eldin TA, A, Elwafa S. 2015b. Effect of inclusion inorganic, organic or nano selenium forms in broiler diets on: physiological, immunological and toxicity statuses of broiler chicks. Int J Poultry Sci. 14(3):144–155.
- Sembratowicz I, Ognik K, Stępniowska A. 2016. An evaluation of in vitro intestinal absorption of iron, calcium and potassium in chickens receiving gold nanoparticles. Br Poult Sci. 57(4):559–565.
- Sheiha AM, Abdelnour SA, Abd El-Hack ME, Khafaga AF, Metwally KA, Ajarem JS, Maodaa SN, Allam AA, El-Saadony MT. 2020. Effects of dietary biological or chemical-synthesized nano-selenium supplementation on growing rabbits exposed to thermal stress. Animals. 10(3):430.
- Shi L, Xun W, Yue W, Zhang C, Ren Y, Liu Q, Wang Q, Shi L. 2011a. Effect of elemental nano-selenium on feed digestibility, rumen fermentation, and purine derivatives in sheep. Anim Feed Sci Technol. 163(2–4):136–142.
- Shi L, Xun W, Yue W, Zhang C, Ren Y, Shi L, Wang Q, Yang R, Lei F. 2011b. Effect of sodium selenite, Se-yeast and nano-elemental selenium on growth performance, Se concentration and antioxidant status in growing male goats. Small Rum Res. 96(1):49–52.
- Shokraneh M, Sadeghi AA, Mousavi SN, Esmaeilkhanian S, Chamani M. 2020. Effects of in ovo injection of nano-selenium and nano-zinc oxide and high eggshell temperature during late incubation on antioxidant activity, thyroid and glucocorticoid hormones and some blood metabolites in broiler hatchlings. Acta Sci Anim Sci. 42:e46029.
- Sirirat N, Lu J, Hung T, Lien T. 2013. Effect of different levels of nanoparticles chromium picolinate supplementation on performance, egg quality, mineral retention, and tissues minerals accumulation in layer chickens. J Agric Sci. (Toronto). 5:150–159.
- Sohair AA, El-Manylawi MA, Bakr M, Ali AA. 2017. Use of nano-calcium and phosphorus in broiler feeding. Egypt Poult Sci J. 37:637–650.
- Swain PS, Rao SBN, Rajendran D, Dominic G, Selvaraju S. 2016. Nano zinc, an alternative to conventional zinc as animal feed supplement: a review. Anim Nutr. 2(3):134–141.
- Swain PS, Prusty S, Nageswara SB, Rajendran D, Patra AK. 2021a. Essential nanominerals and other nanomaterials in poultry nutrition and production. In Patra AK, editor. Advances in poultry nutrition research. London: IntechOpen; Available from: https://www.intechopen.com/chapters/75137. (accessed 2 February 2022).
- Swain PS, Rao SBN, Rajendran D, Krishnamoorthy P, Monda S, Pal D, Selvaraju S. 2021b. Nano zinc supplementation in goat (Capra hircus) ration improves immunity, serum zinc profile and IGF-1 hormones without affecting thyroid hormones. J Anim Physiol Anim Nutr. 105(4):621–629.
- Szuba-Trznadel A, Rząsa A, Hikawczuk T, Fuchs B. 2021. Effect of zinc source and level on growth performance and zinc status of weaned piglets. Animals. 11(7):2030.
- Tabandeh MR, Samie KA, Mobarakeh ES, Khadem MD, Jozaie S. 2022. Silver nanoparticles induce oxidative stress, apoptosis and impaired steroidogenesis in ovarian granulosa cells of cattle. Anim Reprod Sci. 236:106908.
- Tomaszewska E, Muszyński S, Ognik K, Dobrowolski P, Kwiecień M, Juśkiewicz J, Chocyk D, Świetlicki M, Blicharski T, Gładyszewska B. 2017. Comparison of the effect of dietary copper nanoparticles with copper (II) salt on bone geometric and structural parameters as well as material characteristics in a rat model. J Trace Elem Med Biol. 42:103–110.
- Vadalasetty KP, Lauridsen C, Engberg RM, Vadalasetty R, Kutwin M, Chwalibog A, Sawosz E. 2018. Influence of silver nanoparticles on growth and health of broiler chickens after infection with Campylobacter jejuni. BMC Vet Res. 14(1):1. doi: 10.1186/s12917-017-1323-x.
- Vijayakumar MP, Balakrishnan V. 2014. Effect of calcium phosphate nanoparticles supplementation on growth performance of broiler chicken. IJST. 7(8):1149–1154.
- Wahab R, Mishra A, Yun SI, Kim YS, Shin HS. 2010. Antibacterial activity of ZnO nanoparticles prepared via non-hydrolytic solution route. Appl Microbiol Biotechnol. 87(5):1917–1925.
- Wang MJ, Chen Huang JT Huang Jw, Chen SE. 2017. Evaluation of the toxicity of nano-calcium carbonates and their effects on antiheat stress in laying hens. J Chin Soc Anim Sci. 46:223–234.
- Wernicki A, Puchalski A, Urban-Chmiel R, Dec M. 2014. Antimicrobial properties of gold, silver, copper and platinum nanoparticles against selected microorganisms isolated from cases of mastitis in cattle. Med Weter. 70(9):564–567.
- Wu X, Yao J, Yang Z, Yue W, Ren Y, Zhang C, Liu X, Wang H, Zhao X, Yuan S, et al. 2011. Improved fetal hair follicle development by maternal supplement of selenium at nano size (Nano-Se). Livestock Sci. 142(1–3):270–275.
- Xun W, Shi L, Yue W, Zhang C, Ren Y, Liu Q. 2012. Effect of high-dose nano-selenium and selenium-yeast on feed digestibility, rumen fermentation, and purine derivatives in sheep. Biol Trace Elem Res. 150(1–3):130–136.
- Yaghmaie P, Ramin A, Asri-Rezaei S, Zamani A. 2017. Evaluation of glutathione peroxidase activity, trace minerals and weight gain following administration of selenium compounds in lambs. Vet Res Forum. 8(2):133–137.
- Yarmohammadi AB, Sharifi Sd, Mohammadi-Sangcheshmeh A. 2020. Efficacy of dietary supplementation of nanoparticles-chromium, chromium methionine and zinc-proteinate, on performance of Japanese quail under physiological stress. Italian J Anim Sci. 19:1123–1134.
- Youssef FS, El-Banna HA, Elzorba HY, Galal AM. 2019. Application of some nanoparticles in the field of veterinary medicine. Int J Vet Sci Med. 7(1):78–93.
- Yusof HM, Mohamad R, Zaidan UH, Rahman NAA. 2019. Microbial synthesis of zinc oxide nanoparticles and their potential application as an antimicrobial agent and a feed supplement in animal industry: a review. J Anim Sci Biotechnol. 10(57).doi: 10.1186/s40104-019-0368-z
- Zhang J, Wang X, Xu T. 2008. Elemental selenium at nano size (Nano-Se) as a potential chemopreventive agent with reduced risk of selenium toxicity: comparison with se-methylselenocysteine in mice. Toxicol Sci. 101(1):22–31.
- Zhang JS, Gao XY, Zhang LD, Bao YP. 2001. Biological effects of a nano red elemental selenium. Biofactors. 15(1):27–38.