ABSTRACT
Relative afferent pupillary defects (RAPD) may be detected in patients with occipital lobe lesions. However, no previous report has used an objective technique to record the abnormal pupillary light reflex in such cases. Therefore, we measured the pupillary light reflex objectively in 15 patients with homonymous visual field defects (HVFD) due to occipital stroke using a new pupillometer. This study detected significantly smaller and slower pupillary light reflexes in the contralateral eyes than in the other eyes, which is equivalent to the presence of RAPD in patients with HVFDs caused by retrogeniculate lesions using an objective technique. Our results confirmed those of the previous reports using the swinging flashlight test more objectively.
Introduction
Wernicke reported that the pupillary afferent fibre pathway departs from the visual pathway at the optic tract.Citation1 The presence of a contralateral relative afferent pupillary defect (RAPD) on the lesion side is well known in homonymous visual field defects (HVFDs) patients with optic tract lesions; the proposed pathogenesis is based on the greater nasal photoreceptor density and a ratio of crossed to uncrossed fibres in the chiasm of 53:47.Citation2
On the other hand, Wilhelm and associates reported that contralateral RAPD was detected in some of the patients with HVFDs caused by lesions not involving the optic tract.Citation3,Citation4 These phenomena could be explained by the notion that the pupillomotor response depends upon or is influenced by the occipital cortex.Citation3–Citation5 However, convincing anatomical evidence for this interpretation is lacking. Alternatively, such RAPD may be caused by a lesion of intercalated neurons between the visual pathways and the pupillomotor centres in the midbrain. This assumption has been strengthened by several case reports in which contralateral RAPD was found in patients without visual field defects due to a lesion between the visual pathways and pretectum.Citation6,7
In recent years, retrograde trans-synaptic degeneration (RTSD) in the patients with HVFDs has reported that the thickness of the inner layer of the retina is thinned,Citation8,Citation9 but the relationship with pupillary light reflex is not clarified. If retinal ganglion cells are damaged due to RTSD, there could be corresponding pupillary defects. However, in a case report of a patient with right occipital lesion, while optical coherence tomography (OCT) revealed inner retinal thinning corresponding to the homonymous visual field loss, no RAPD was found to be present.Citation10
In the previous studies of pupillary light reflex in patients of HVFDs due to geniculate or retrogeniculate lesions, the contralateral RAPD was measured by the swinging flashlight test (SFT) with a neutral density (ND) filter.Citation3,Citation4 The reported occurrence of the RAPD appears to be definitely more than a chance. However, it may be difficult to detect a small contralateral RAPD due to a difference in the crossed and uncrossed fibres during a subjective evaluation.
Recently, a new commercially available pupillometer, RAPDx® (Konan Medical USA, Inc., Irvine, CA), became available. This pupillometer measures the pupillary light reflex by alternating the stimulation of both eyes and can calculate two kinds of RAPD scores from the pupillary constriction amplitude and latency.Citation11–Citation16 The pupillometer keeps a fixed duration, interval and intensity of the stimulation and can therefore measure a stable pupil light reflex. By using this pupillometer, it may be possible to quantitatively detect a small RAPD arising from the difference in crossed and uncrossed retinal nerve fibres. We herein objectively examined whether the asymmetry of the pupil light reflex in terms of the amplitude and latency using the pupillometer is detectable, as a group, in patients with HVFDs due to a stroke affecting the occipital lobe.
Material and methods
Subjects
We performed a retrospective cross-sectional study investigating consecutive patients with HVFDs and control subjects at the Kawasaki Medical School Hospital from November 2012 to August 2014. These studies were approved by Research Ethics Committee of Kawasaki Medical School and Hospital. Informed consent for examinations was obtained from all patients. This study adhered to the tenets of the Declaration of Helsinki.
We included patients with HVFDs due to stroke of the occipital lobe. The patients with HVFDs underwent an examination of the visual acuity and visual field, magnetic resonance imaging (MRI), a slit lamp examination and an ocular fundus examination. We checked that the affected area did not involve the lateral geniculate nucleus (LGN) using the MRI. We excluded patients with unilateral cataracts, retinal disease, glaucoma, or optic nerve disease. We also enrolled subjects without any eye disease other than refractive error as controls.
Fifteen patients with HVFDs due to stroke of the occipital lobe were enrolled in this study ( and ). Thirty-two normal subjects (seven male and 25 female; mean age 23.1 ± 6.0 years old; range 6–36 years old) were enrolled in this study.
Table 1. Detailed profile and results of patients with HVFDs.
Table 2. The clinical characteristics of the 15 patients with HVFDs.
Pupil recordings
We used a pupillometer, the RAPDx® (Konan Medical USA, Inc., Irvine, CA), with the intensity of the stimulus light set at 384 cd/mCitation2, the stimulus colour was white, and the area of stimulation was 30° of the visual field. The duration of stimulation was 0.2 seconds, and the presentation interval of the stimulation was 2.1 seconds. The stimulation was presented each of 18 times alternately to both eyes. The first two stimuli were excluded from the analysis, and 16 of 18 stimuli were analysed. The background illuminance was 0.01 cd/mCitation2. The amplitude was defined by the pre-stimulus pupil diameter minus the minimum pupil diameter, divided by the pre-stimulus pupil diameter. The pre-stimulus pupil diameter were determined by averaging pupil sizes during 300 ms before the onset of stimulation. The RAPD score of amplitude was defined by 10*log10 (mean amplitude of direct and consensual responses by left eye stimulation/mean amplitude of direct and consensual responses by right eye stimulation) using a built-in software of pupillometer. The latency was defined by the interval between the stimulus onset and the time when pupil velocity had reached 50% of the peak velocity of constriction. The RAPD score of latency was defined as 10*log10 (mean latency of direct and consensual responses by the right eye stimulation/mean latency of direct and consensual responses by left eye stimulation). The RAPD score was then represented by a median value of the 16 measurements. Thus, positive RAPD score shows the RAPD of right eye, and negative RAPD scores the RAPD of left eye. We inverted the sign of the RAPD score of the patients with left HVFDs to facilitate the interpretation of the results. In this study, data from patients with excessive eye blinking were excluded.
Statistical analysis
The statistical analyses were performed using the SPSS 22 software program (SPSS, IBM, Tokyo, Japan). The differences between patients with HVFDs and control subjects were compared by the two-sample Student’s t-test. In addition, the relationship between the RAPD scores and the interval of time from the stroke to examination was examined using Pearson’s correlation coefficient and linear regression. A statistically significant difference was defined by a value of p < 5%.
Results
The mean RAPD score of amplitude was 0.13 ± 0.24 (range from −0.22 to 0.63) in the eye contralateral to the brain lesions in patients with HVFDs, which was significantly higher than that of the control subjects (mean 0.01 ± 0.09, range from −0.20 to 0.21) (p = 0.02; , left). Also, the mean RAPD score of latency was 0.09 ± 0.10 (range from −0.07 to 0.28) in patients with HVFDs, which was significantly higher than that of the control subjects (mean 0.00 ± 0.12 log unit, range from −0.20 to 0.30) (p = 0.01) (, right). The correlation coefficient was R = 0.21 (p = 0.45) between the RAPD score of amplitude and the interval of time from the stroke to examination, and was R = 0.59 (p = 0.02) between the RAPD score of latency and the interval of time. ()
Figure 1. Box plot of the relative afferent pupillary defect (RAPD) scores in the patients with homonymous visual field defects (HVFD) and control groups. Upper horizontal line of box = mean plus one standard deviation (SD); Lower horizontal line of box = mean minus one SD; horizontal bar within box = mean; Upper horizontal bar outside box = maximum; Lower horizontal bar outside box = minimum. Circle = each measured value. RAPD scores of amplitude of the HVFDs (the sign of scores of the patients with left HVFDs were inverted to merge the data, n = 15) group were significantly higher in the eye contralateral to the brain lesion than those in the control group (n = 32) (p < 0.05). Also, the RAPD scores of latency were significantly higher in the eye contralateral to the brain lesion than those of the controls (p < 0.01). * p < 0.05, ** p < 0.01.
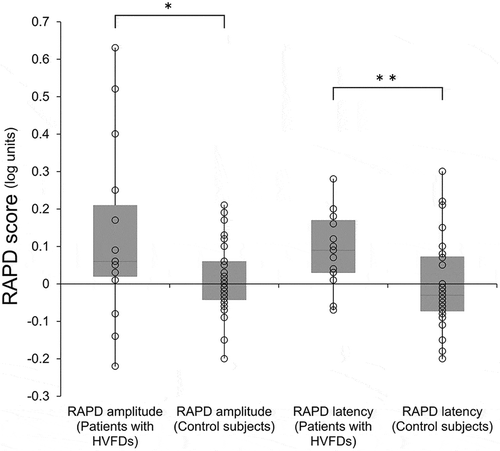
Figure 2. The relationship between the RAPD scores and the interval of time from the stroke to examination of RAPD.
The upper scatter plot shows the relationship between the RAPD score of amplitude (the sign of scores of the patients with left HVFDs was inverted to merge them with those of the right HVFDs, n = 15) and interval of time from the stroke to examination. R = 0.21 (p = 0.45). The lower scatter plot shows the relationship between the RAPD score of latency (the sign of scores of the patients with left HVFDs was inverted to merge them with those of the right HVFDs, n = 15) and interval of time from the stroke to examination. R = 0.59 (p = 0.02).
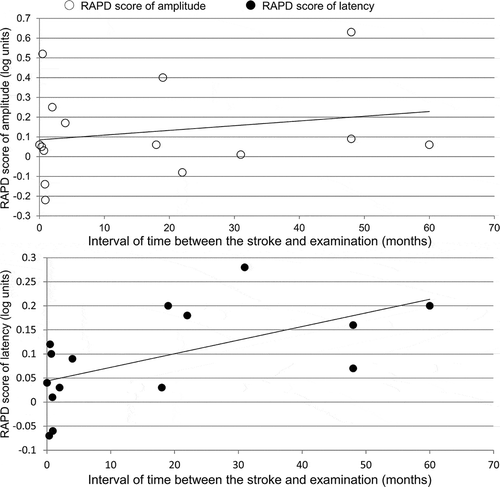
Discussion
The RAPD scores of amplitude and latency determined using this new method detected a small but statistically significant RAPD in the patients with HVFDs due to a stroke affecting the occipital lobe in this study. The RAPD score of latency and the interval of time from the stroke to examination of RAPD showed a weak relationship.
The pupillary light reflex pathway has been explained by Wernicke’s model of direct retinal-pretectal interaction. The model was supported by the presence of continued pupil responses in patients with cortical blindness.Citation17 However, several authors have indicated that there are pupillary abnormalities consistent with the visual field defects (i.e. pupillary hemihypokinesia or hemiakinesia) in patients with occipital hemianopia.Citation18–Citation21
The RAPD in congenital retrogeniculate hemianopia has been considered to be caused by RTSD.Citation22,Citation23 There was a weak but significant correlation between only the RAPD score of latency and interval of time elapsed after the stroke. This result seems to support the notion that RAPD progressively increased with the development of RTSD. Recently, it has been reported that retinal thinning possibly due to RTSD is present in humans, as determined using OCT in patients with HVFDs due to lesions not involving the optic tract and LGN.Citation8–Citation10,Citation24,Citation25 In our previous study, we reported that the inner retina corresponding to the blind visual fields demonstrated thinning within a few years after the onset of occipital damage using spectral-domain OCT.Citation9 Some of the patients who developed a post-geniculate lesion within 1 year of evaluation did not show inner macular thinning by OCT.Citation26 Meier et al. found that the retinal nerve fibre layer of a patient with HVFD due to an occipital lobe abscess was thinned but no RAPD was noted after 2 years.Citation10 Conversely, small RAPD due to HVFDs has been detected immediately after stroke, when no retinal atrophy should be present.Citation4 Therefore, such a discrepancy between the OCT and pupillary findings suggest that a major contribution of RTSD to the abnormal light reflex demonstrated here is considered to be unlikely. In the present study, the median time interval between lesion onset and the measurement of the RAPD was about 4 months and 8 of 15 patients with HVFDs were examined within 1 year of onset, RTSD of retinal ganglion cells should have been incomplete at the time of our pupillary examination. Thus, the interval of time elapsed after the stroke was relatively short in this study, and it would be necessary to study the effect of RTSD on RAPD scores from a long-term follow-up of these patients.
Wilhelm et al. showed that 5 of 9 patients with geniculate lesions and 11 of 34 patients with retrogeniculate lesions had an RAPD greater than 0.3 log units and contralateral to the lesion.Citation3 This RAPD in homonymous hemianopia did not occur in lesions farther than 18 mm from the LGN.Citation3 Papageorgiou et al. reported that an RAPD greater than 0.3 log units and contralateral to the affected hemisphere was observed in 10 of 23 patients with HVFDs. Their MRI analysis has suggested that a region including the course of the optic radiation at its early beginning in the temporal white matter is involved in RAPD.Citation4 Therefore, it seems that an RAPD appears when this particular region near the LGN is damaged. The relationship between the area Papageorgiou et al. demonstratedCitation4 and the responsible lesion for normal vision with RAPDCitation6,7 remains unclear.
Although clinical standards for the normal threshold of RAPD score by SFT with ND filter is 0.3 log units, Kawasaki et al. demonstrated that RAPD of 0.3 log units could be present in normal eyes.Citation27 In previous studies of RAPD in patients with HVFDs, since the RAPD score of 0.3 log units was used for distinguishing between the presence and absence of RAPD, RAPDs smaller than 0.3 log units had not been evaluated.Citation3,Citation4 It was reported that there was a significant correlation between RAPD score by SFT using ND filter and RAPD score by RAPDx®,Citation28 and the normal value of RAPDx® in normal subjects was determined to be ≤0.2 log units.Citation16 While such a threshold should be useful in the clinical settings, this pupillometer has an advantage that a small RAPD can be measured because it is directly calculated from the amplitude and latency of the pupillary constriction obtained, unlike the conventional quantification by SFT using ND filter. The cause of RAPD in HVFDs is attributable to the difference in the ratio between crossed and non-crossed fibres and the resulting RAPD is considered to be fairly small. It is possible that the naso-temporal asymmetry of retina and visual fields may produce RAPD of less than 0.2 or 0.3 log units. Goto et al. have recently reported that the RAPD score of amplitude measured using RAPDx® of patients with optic tract syndrome was 0.49 ± 0.18 log units.Citation29 The RAPD score of the stroke of occipital lobe seems to be smaller than that of optic tract syndrome, and quantitative measurement by RAPDx® may be clinically useful for predicting the location of lesions causing HVFDs.
On the other hand, three of patients with HVFDs (Patients 6, 12, and 15) showed small RAPDs, in terms of the RAPD score of amplitude, on the ipsilateral eye of the geniculate or retrogeniculate lesion side. The previous studies showing RAPDs contralateral to the brain lesion found no patient with an RAPD ipsilateral to the brain damage.Citation3,Citation4 We objectively measured the pupillary light reflex, while the previous reports used SFT with ND filter, which may be inherently complicated by the bias towards an RAPD on the expected side by the investigator.
We are aware that there are limitations to our study. Firstly, there was a significant difference in the mean age between the patients with HVFDs and the control subjects. However, Satou et al. reported that the RAPD score of amplitude and latency measured RAPDx® did not differ significantly among the different age groups.Citation16 Therefore, we consider that the difference in the age between the groups does not seem to explain our findings. Secondly, we did not take the size or location of brain lesions into consideration in our analysis. A further study to classify the patients with HVFDs according to the results of neuroimaging would be necessary.
In conclusion, we detected significantly smaller and slower pupillary light reflex in the eyes contralateral to the side of the brain lesion in patients with a stroke affecting the occipital lobe using an objective pupillometer-based method.
Declaration of interest
The authors report no conflicts of interest. The authors alone are responsible for the content and writing of the paper.
Funding
This work was supported by JSPS KAKENHI, Grant Number 26462703.
Additional information
Funding
References
- Wernicke C. Über hemianopische pupillenreaktion. Fortschr Med 1883;1:49–53.
- Bell RA, Thompson HS. Relative afferent pupillary defect in optic tract hemianopias. Am J Ophthalmol 1978;85:538–540. doi:10.1016/S0002-9394(14)75251-1.
- Wilhelm H, Wilhelm B, Petersen D, Schmidt U, Schiefer U. Relative afferent pupillary defects in patients with geniculate and retrogeniculate lesions. Neuro-Ophthalmol 1996;16:219–224. doi:10.3109/01658109609044629.
- Papageorgiou E, Ticini LF, Hardiess G, Schaeffel F, Wiethoelter H, Mallot HA, Bahlo S, Wilhelm B, Vonthein R, Schiefer U, Karnath HO. The pupillary light reflex pathway: cytoarchitectonic probabilistic maps in hemianopic patients. Neurology 2008;70:956–963. doi:10.1212/01.wnl.0000305962.93520.ed.
- Wilhelm BJ, Wilhelm H, Moro S, Barbur JL. Pupil response components: studies in patients with Parinaud’s syndrome. Brain 2002;125:2296–2307. doi:10.1093/brain/awf232.
- Ellis CJ. Afferent pupillary defect in pineal region tumour. J Neurol Neurosurg Psychiatry 1984;47:739–741. doi:10.1136/jnnp.47.7.739.
- Papageorgiou E, Wermund T, Wilhelm H. Pupil perimetry demonstrates hemifield pupillary hypokinesia in a patient with a pretectal lesion causing a relative afferent pupil defect but no visual field loss. J Neuroophthalmol 2009;29:33–36. doi:10.1097/WNO.0b013e318198cb8c.
- Jindahra P, Petrie A, Plant GT. The time course of retrograde trans-synaptic degeneration following occipital lobe damage in humans. Brain 2012;135:534–541. doi:10.1093/brain/awr324.
- Yamashita T, Miki A, Iguchi Y, Kimura K, Maeda F, Kiryu J. Reduced retinal ganglion cell complex thickness in patients with posterior cerebral artery infarction detected using spectral-domain optical coherence tomography. Jpn J Ophthalmol 2012;56:502–510. doi:10.1007/s10384-012-0146-3.
- Meier PG, Maeder P, Kardon RH, Borruat FX. Homonymous ganglion cell layer thinning after isolated occipital lesion: macular OCT demonstrates transsynaptic retrograde retinal degeneration. J Neuroophthalmol 2015;35:112–116.
- Chang DS, Boland MV, Arora KS, Supakontanasan W, Chen BB, Friedman DS. Symmetry of the pupillary light reflex and its relationship to retinal nerve fiber layer thickness and visual field defect. Invest Ophthalmol Vis Sci 2013;54:5596–5601. doi:10.1167/iovs.13-12142.
- Ozeki N, Yuki K, Shiba D, Tsubota K. Pupillographic evaluation of relative afferent pupillary defect in glaucoma patients. Br J Ophthalmol 2013;97:1538–1542. doi:10.1136/bjophthalmol-2013-303825.
- Tatham AJ, Meira-Freitas D, Weinreb RN, Zangwill LM, Medeiros FA. Detecting glaucoma using automated pupillography. Ophthalmology 2014;121:1185–1193. doi:10.1016/j.ophtha.2013.12.015.
- Chang DS, Arora KS, Boland MV, Supakontanasan W, Friedman DS. Development and validation of an associative model for the detection of glaucoma using pupillography. Am J Ophthalmol 2013;156:1285–1296. doi:10.1016/j.ajo.2013.07.026.
- Takizawa G, Miki A, Maeda F, Goto K, Araki S, Ieki Y, Kiryu J, Yaoeda K. Association between a relative afferent pupillary defect using pupillography and inner retinal atrophy in optic nerve disease. Clin Ophthalmol 2015;9:1895–1903. doi:10.2147/OPTH.S91278.
- Satou T, Goseki T, Asakawa K, Ishikawa H, Shimizu K. Effects of age and sex on values obtained by RAPDx® pupillometer, and determined the standard values for detecting relative afferent pupillary defect. Transl Vis Sci Technol 2016;5:18. doi:10.1167/tvst.5.2.18.
- Brindley GS, Gautier-Smith PC, Lewin W. Cortical blindness and the functions of the non-geniculate fibres of the optic tracts. J Neurol Neurosurg Psychiatry 1969;32:259–2647. doi:10.1136/jnnp.32.4.259.
- Cibis GW, Campos EC, Aulhorn E. Pupillary hemiakinesia in suprageniculate lesions. Arch Ophthalmol 1975;93:1322–1327. doi:10.1001/archopht.1975.01010020954004.
- Hamann KU, Hellner KA, Müller-Jensen A, Zschocke S. Videopupillographic and VER investigations in patients with congenital and acquired lesions of the optic radiation. Ophthalmologica 1979;178:348–356. doi:10.1159/000308846.
- Kardon RH. Pupil perimetry. Curr Opin Ophthalmol 1992;3:565–570. doi:10.1097/00055735-199210000-00002.
- Yoshitomi T, Matsui T, Tanakadate A, Ishikawa S. Comparison of thresholdvisual perimetry and objective pupil perimetry in clinical patients. J Neuroophthalmol 1999;19:89–99. doi:10.1097/00041327-199906000-00003.
- Tychsen L, Hoyt WF. Occipital lobe dysplasia: magnetic resonance findings in two cases of isolated congenital hemianopia. Arch Ophthalmol 1985;103:680–682. doi:10.1001/archopht.1985.01050050072021.
- Tychsen L, Hoyt WF. Relative afferent pupillary defect in congenital occipital hemianopia. Am J Ophthalmol 1985;100:345–346. doi:10.1016/0002-9394(85)90818-9.
- Goto K, Miki A, Yamashita T, Araki S, Takizawa G, Nakagawa M, Ieki Y, Kiryu J. Sectoral analysis of the retinal nerve fiber layer thinning and its association with visual field loss in homonymous hemianopia caused by post-geniculate lesions using spectral-domain optical coherence tomography. Graefes Arch Clin Exp Ophthalmol 2016;254:745–756. doi:10.1007/s00417-015-3181-1.
- Yamashita T, Miki A, Goto K, Araki S, Takizawa G, Ieki Y, Kiryu J, Tabuchi A, Iguchi Y, Kimura K, Yagita Y. Retinal ganglion cell atrophy in homonymous hemianopia due to acquired occipital lesions observed using cirrus High-Definition-OCT. J Ophthalmol 2016;2016:2394957. doi:10.1155/2016/2394957.
- Mitchell JR, Oliveira C, Tsiouris AJ, Dinkin MJ. Corresponding ganglion cell atrophy in patients with postgeniculate homonymous visual field loss. J Neuroophthalmol 2015;35:353–359. doi:10.1097/WNO.0000000000000268.
- Kawasaki A, Moore P, Kardon RH. Long-term fluctuation of relative afferent pupillary defect in subjects with normal visual function. Am J Ophthalmol 1996;122:875–882. doi:10.1016/S0002-9394(14)70385-X.
- Cohen LM, Rosenberg MA, Tanna AP, Volpe NJ. A novel computerized portable pupillometer detects and quantifies relative afferent pupillary defects. Curr Eye Res 2015;40:1120–1127. doi:10.3109/02713683.2014.980007.
- Goto K, Miki A, Yamashita T, Araki S, Takizawa G, Mizukawa K, Ieki Y, Kiryu J. Quantitative analysis of macular inner retinal layer using swept-source optical coherence tomography in patients with optic tract syndrome. J Opthalmol 2017;2017:3596587.