Abstract
Ion channels remain the primary target of most of the small molecule insecticides. This review examines how the subunit composition of heterologously expressed receptors determines their insecticide-specific pharmacology and how the pharmacology of expressed receptors differs from those found in the insect nervous system. We find that the insecticide-specific pharmacology of some receptors, like that containing subunits of the Rdl encoded GABA receptor, can be reconstituted with very few of the naturally occurring subunits expressed. In contrast, workers have struggled even to express functional insect nicotinic acetylcholine receptors (nAChRs), and work has therefore often relied upon the expression of vertebrate receptor subunits in their place. We also examine the extent to which insecticide-resistance-associated mutations, such as those in the para encoded voltage-gated sodium channel, can reveal details of insecticide-binding sites and mode of action. In particular, we examine whether mutations are present in the insecticide-binding site and/or at sites that allosterically affect the drug preferred conformation of the receptor. We also discuss the ryanodine receptor as a target for the recently developed diamides. Finally, we examine the lethality of the genes encoding these receptor subunits and discuss how this might determine the degree of conservation of the resistance-associated mutations found.
Introduction
The target sites and secondary effects of neuroactive insecticides have recently been reviewed (Casida & Durkin, Citation2013). The current review therefore updates work on ion channels as targets (Bloomquist, Citation1996) and examines the role of Drosophila genetics in the cloning of the genes that encode them. Five ion channels within the insect nervous system remain the primary targets for the development of small molecule insecticides. These are, first, the γ-aminobutyric acid (GABA) receptor containing subunits encoded by the Resistance to dieldrin gene or Rdl (the site of action of cyclodienes and fipronil), second, the glutamate-gated chloride channel, third, the insect nicotinic acetylcholine receptor or nAChR (neonicotinoids and spinosyns), fourth, the voltage-gated sodium channel encoded by homologs of the Drosophila gene para (DDT and pyrethroids) and fifth the insect ryanodine receptor (ryanodine and the diamides). Here, we will review the discovery and cloning of the first receptor subunits genes. We will examine the historical role that Drosophila genetics has played in the cloning of these important genes and compare their structure with those found in pest insects. We will then examine the extent to which the reconstitution of the full native pharmacology of these receptors is necessary in order to make valid inferences about insecticide-specific aspects of their pharmacology. Finally, we will examine the extent to which resistance-associated mutations in both Drosophila and pest insects have highlighted the binding sites and potential biophysical modes of action of the insecticides that target them. We will start our discussion with chloride ion channels gated either by GABA or glutamate.
The GABA-gated chloride channel or GABA receptor
Lessons from Drosophila
γ-aminobutyric acid or GABA, is the major inhibitory neurotransmitter in both vertebrates and invertebrates and acts to open the pentameric transmembrane chloride channel within the GABA receptor (Bloomquist, Citation1993). In vertebrates, GABA receptors are divided into GABAA and GABAB subtypes. The human brain GABAA receptor is composed of various combinations of α, β, or γ subunits, typically two α, two β and one γ subunit per pentamer, with each subunit type then conferring its own drug-specific pharmacology on the final native receptor. However, until the cloning of insect GABA receptors from Drosophila, little was known about the subunit composition or pharmacology of the equivalent receptors in insects. Two different approaches were originally taken to the cloning of Drosophila GABA receptors. The first was positional cloning of the Resistance to dieldrin or Rdl locus, which was isolated from field populations of D. melanogaster by screening with the cyclodiene insecticide dieldrin (ffrench-Constant, Rocheleau, Steichen, & Chalmers, Citation1993). Pharmacological work suggested that the cyclodiene insecticides (including dieldrin) interacted with insect GABA receptors and that picrotoxinin or ‘PTX’ had the same site of action and acts as a non-competitive antagonist (Bloomquist, Citation1993). The second approach was to use conserved amino acid sequences within the ion channel lining of GABA-gated chloride channels to design primers for use in the polymerase chain reaction or PCR (Henderson, Knipple, & Soderlund, Citation1994). Gratifyingly both approaches produced genes encoding GABA-gated chloride ion channels. The Rdl locus encodes the Rdl GABA receptor subunit and the same Rdl-containing sequence, termed LCCH1 (Henderson et al., Citation1994), was also recovered from the PCR-based approach. The PCR-based approach also recovered two other GABA receptor-like subunit encoding genes termed LCCH2 and LCCH3 (Henderson et al., Citation1994).
Heterologous expression and GABA receptor subunit composition in vivo
Vertebrate GABAA receptors are complex heteromultimers and co-expression of both α, β, or γ subunits together are necessary for heterologous expression in systems such as the Xenopus oocyte (Barnard Steichen, & Ode, Citation1987). It is therefore highly surprising that transcripts from the Rdl gene could be readily expressed as Rdl homomultimers in both Xenopus oocytes and insect cells (ffrench-Constant, Steichen, & Ode, Citation1993; Shotkoski et al., Citation1994). These Rdl homomultimers not only form highly functional GABA-gated chloride channels but currents from these channels can also be impeded by the application of either dieldrin (the insecticide used to recover the resistant mutant) or picrotoxinin (PTX) (ffrench-Constant, Steichen, & Ode, Citation1993). In this regard, insect GABA receptors are very different from their vertebrate counterparts and should therefore probably be considered as corresponding to their own unique receptor subtypes (Buckingham, Biggin, Sattelle, Brown, & Sattelle, Citation2005).
Subsequent analysis of single ion channels in patch clamped Drosophila neurons confirmed that most GABA-gated channels can be blocked by both dieldrin and PTX (Zhang et al., Citation1994). However, measurements of the conductance of these native channels confirmed that they could not correspond to Rdl homomultimers and therefore that other unidentified subunits must also be present in the native receptor (Zhang et al., Citation1995). Moreover, whilst the co-expression of LCCH3 with Rdl in heterologous systems adds bicuculine sensitivity to the pharmacology of the heterologously expressed receptor, again measurements of the single channel conductance of the Rdl-LCCH3 heteromultimer suggest that this is again not the native composition of most PTX sensitive GABA receptors in Drosophila (Zhang et al., Citation1995). In short, the precise subunit composition of native GABA receptors containing Rdl subunits remains far from clear.
Finally, adding further complexity to likely insect GABA receptor subunit diversity, both alternative splicing and predicted RNA editing have been shown for the subunits encoded by Rdl. The Rdl transcript shows alternative splicing at two locations where alternative exons of the same size are selected, termed ‘a’ or ‘b’ at position one and ‘c’ and ‘d’ at position two (ffrench-Constant & Rocheleau, Citation1993). Similarly, the Rdl transcript also shows several predicted sites for RNA editing and functional expression of transcripts altered by both alternative splicing and RNA editing shows that the combination of the two editing methods delivers an array of different subtypes with differing sensitivities to the agonist GABA (Jones et al., Citation2009). This combination of alternative splicing and RNA editing is a powerful method for obtaining numerous receptor subtypes from a single gene and is a theme we will be returning to, as we examine other ion channel targets.
Resistance-associated mutations in Rdl and insecticide binding
The Rdl-containing GABA receptor is the site of action of cylcodiene insecticides such as dieldrin, aldrin and endrin (Bloomquist, Citation1993). Whilst resistance to cyclodienes has accounted for a staggering number of reported cases of insecticide resistance historically (ffrench-Constant, Citation1994), as the cyclodienes have now been withdrawn, the Rdl-containing receptor is now much more important as the target site for the phenylpyrazoles, such as the now widely used fipronils. To this end it was important to find the resistance-associated mutation in Rdl both to examine its historical role in cyclodiene resistance and also its potential future role in conferring resistance to fipronil. Sequencing of several dieldrin-resistant strains of D. melanogaster showed that a single alanine (alanine301 in Drosophila), within the predicted ion channel lining of the receptor (at the start of transmembrane segment M2, ), could be replaced with either a serine or a glycine residue to make the Drosophila strains resistant to dieldrin. Replacement of this alanine within laboratory expressed Rdl homomultimers also confers dieldrin and PTX resistance on the associated GABA-gated chloride fluxes. Importantly, a susceptible Rdl mini-gene also rescues resistance in transformed D. melanogaster (Stilwell, Rocheleau, & ffrench-Constant, Citation1995) and correspondingly both A301S and A301G replacements (which are both semi-dominant) also confer resistance when expressed in vivo in transformed flies (Remnant et al., Citation2014). The observation that replacements of this alanine, either with a serine (addition of a hydroxyl group to the amino acid side chain) or a glycine (reduction of the side chain to a hydrogen group) can cause resistance, led to the hypothesis that it was the presence of this specific alanine at the base of the pore, close to the narrowest part of the ion channel () that promotes drug binding. To test this hypothesis, the detailed biophysics of the Rdl-containing receptor were examined and a model derived that suggests that dieldrin and PTX bind preferentially to the desensitized state of the insect GABA receptor. Further replacements of the key alanine residue not only directly alter the drug-binding site but also allosterically destabilize the drug preferred (desensitized) state of the receptor (Zhang et al., Citation1994). This model is supported by the direct electrophysiological observation that mutants where the critical alanine is replaced with serine or glycine spend less time in the desensitized state (Zhang et al., Citation1994). Replacements of alanine301 therefore play a unique dual role in promoting insecticide resistance.
Figure 1. (a) GABA and glutamate-gated chloride channels share similar structures with individual subunits comprising a large extracellular N-terminal domain (for ligand binding) and four transmembrane domains (M1–M4). The mature receptor (b) is a pentamer and gates a chloride-selective channel following GABA/Glu binding. The positions of mutations implicated in cyclodiene/fipronil resistance at the GABA-R (red circles) and avermectin resistance at the GluCl (yellow circles) are shown in (a) and the corresponding regions of the mature receptors highlighted in (b). Structural models of these binding sites (AVE, avermectin and FIP, fipronil) are shown in (c) (adapted from Casida and Durkin (Casida & Durkin, Citation2015).
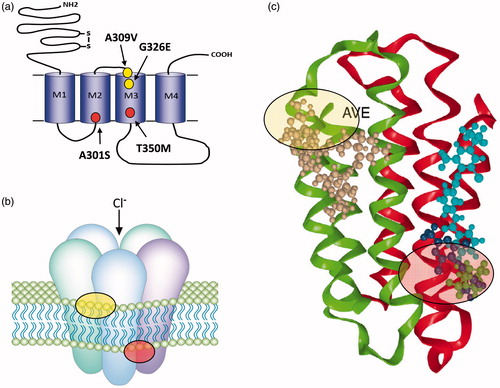
Further amino acid replacements associated with fipronil resistance
The critical importance of alanine301 has been confirmed by the recent finding of yet another replacement of the equivalent residue, in this case to asparagine (A301N, but called A2′N, where 2′ refers to the relative location of the residue within the second membrane spanning domain) in the fipronil-resistant planthopper Laodelphax striatellus (Nakao et al., Citation2011). Further amino acid replacements within Rdl, beyond replacements of alanine301 alone, have also been associated with resistance to fipronil. In a strain of D. simulans selected for fipronil resistance in the laboratory, both the original A301G replacement and a second replacement T350M in the third membrane spanning domain (M3) were found (Le Goff, Hamon, Berge, & Amichot, Citation2005). Functional expression of these two replacements together confirmed that T350M enhances the effect of A301G (Le Goff et al., Citation2005). Recent structure modelling studies have shown that both A301 and T350 map closely to the proposed cyclodiene/fipronil (NCA-1A) site within the channel pore () (Casida & Durkin, Citation2015; Remnant et al., Citation2014) suggesting that the mutations impact directly on binding to this site. Here, we therefore speculate that T350M may further destabilize the fipronil preferred desensitized state of Rdl and therefore increase resistance over and above that conferred by A301G alone. However, to our knowledge, this hypothesis currently remains to be tested. It is also interesting to note that the same amino acid replacement T345M also occurs at an equivalent position in the Rdl receptor subunit of Anopheles gambiae (Taylor-Wells, Brooke, Bermudez, & Jones, Citation2015). Finally, it is worth pointing out that the existing mutations do not appear to affect the binding and activity of two new classes of GABA receptor blockers, the isoxazolines and meta-diamides, which modelling studies suggest interact with different residues within the channel pore (Casida & Durkin, Citation2015) (). These compounds are currently being developed for control of animal health pests (e.g., ticks and fleas) but may also find subsequent applications in crop protection.
Fitness phenotypes and Rdl gene duplication
Finally, before leaving the insect GABA receptor it is worth discussing the likely negative fitness phenotypes associated with the Rdl gene itself. One often overlooked Rdl phenotype is that resistant mutants (R) in which alanine301 is replaced are also temperature sensitive paralytics (ffrench-Constant, Rocheleau, Steichen, & Chalmers, Citation1993). That is to say that when raised to elevated temperatures that mutant adult flies take longer to recover (fly away from the substrate) than their susceptible (S) wild-type counterparts. This temperature sensitive paralysis may be associated with a negative fitness phenotype for this resistance gene in the field. It is therefore extremely interesting to note the recent documentation of duplicated alleles of Rdl in D. melanogaster where both a resistant (R) and a susceptible (S) copy of the Rdl are found to be physically linked in tandem on the same allele (Remnant et al., Citation2013). This effectively ‘compound’ heterozygote (R + S), which would be expected to encode both susceptible and resistant subunits from the same allele, may therefore be an effective way of preserving the production of susceptible subunits even when the other allele was resistant (R + S/R).
The glutamate-gated chloride channel
Cloning and functional expression
Glutamate-gated chloride channels (GluCls) are members of the Cys-loop ligand-gated ion channel family that mediate inhibitory synaptic transmission in the nervous system of invertebrates (Wolstenholme, Citation2012). As they are exclusively found in invertebrates they represent excellent highly selective nematicidal, acaricidal and insecticidal targets and are the primary target of the macrocyclic lactones (avermectins and milbemycins). They may also play a secondary role in the insecticidal activity of fipronil (Zhao, Yeh, Salgado, & Narahashi, Citation2004). In contrast to nematodes and mites, the genomes of most insects contain a single GluCl gene with the subunit they encode assumed to assemble into homo-pentameric receptors (Dermauw et al., Citation2012; Jones & Sattelle, Citation2007; Knipple & Soderlund, Citation2010). The subunit composition of GluCls in non-insect arthropods, such as mites is unknown (Wolstenholme, Citation2012).
The first insect GluCl gene to be characterised was from Drosophila which when expressed in Xenopus oocytes produced a homomeric chloride channel gated by both glutamate and ivermectin (Cully, Paress, Liu, Schaeffer, & Arena, Citation1996).
Resistance-associated mutations within GluCl
The first target-site mutation in an insect GluCl was also first described in Drosophila in a strain with resistance to nodulisporic acid, a natural insecticidal product isolated from an endophytic fungus, and weak (threefold) cross-resistance to ivermectin (Kane et al., Citation2000). A combination of genetics and sequencing was used to identify a single mutation, P299S in the Drosophila GluCl channel of this strain that when expressed in Xenopus oocytes was found to reduce channel sensitivity to both compounds (Kane et al., Citation2000). The avermectin abamectin is also an effective miticide and two studies have reported mutations associated with resistance in the GluCl of T. urticae (Dermauw et al., Citation2012; Kwon, Yoon, Clark, & Lee, Citation2010), which in contrast to insects has six genes encoding GluCl (Dermauw et al., Citation2012). In the first study, a G323D mutation in TuGluCl-1 was associated with ∼20-fold resistance to abamectin in T. urticae and found to correlate with resistance in individual F2 progenies obtained by backcrossing (Kwon et al., Citation2010). A second study identified the same mutation in TuGluCl-1 in a T. urticae strain with ∼2000-fold resistance to abamectin, however, a novel substitution was also identified in the same strain, at an identical position (G326E), but in a different GluCl gene (Tu_GluCl3), suggesting these mutations may have an additive or synergistic effect in conferring resistance (Dermauw et al., Citation2012). Genetic association studies with two diagnostic doses of abamectin was used to link both mutations with resistance to lower abamectin concentrations, and the G326E substitution in Tu_GluCl3 with resistance to higher abamectin concentrations (Dermauw et al., Citation2012). Together these studies suggest that these two subunits might be the primary target-sites of abamectin in T. urticae and it would be interesting to explore if they coassemble to form a heteropentameric GluCl or form individual homopentameric receptors.
A point mutation associated with abamectin resistance has also been described in P. xylostella (Wang et al., Citation2016). In this case, A309V substitution at the N-terminus of the third transmembrane helix (M3) was associated with resistance in a field population introgressed into a susceptible strain (Wang et al., Citation2016). Homology modelling suggested that the A309V substitution modifies the abamectin-binding by an allosteric mechanism rather than eliminating a key binding contact (Wang et al., Citation2016). The modelling does however infer a more direct role for the G323/326 mutations identified in T. urticae since this residue within the M3 helix does show direct contact with ivermectin in the open state GluCl model developed by Wang et al. (Citation2016) ().
Alternative splicing and RNA editing of GluCl
Finally, perhaps to overcome the constraints of being encoded by a single gene, insect GluCls expand their coding capacity by mRNA splicing and editing (Jones & Sattelle, Citation2007; Knipple & Soderlund, Citation2010). A study on P. xylostella observed three naturally occurring transcript variants of PxGluCl in both abamectin resistant and susceptible strains (Liu et al., Citation2014). One of these, described as a ‘36-bp deletion’, represents a transcript with alternative exon 9c. Transcripts with this exon were observed at higher frequency in abamectin-resistant strains than susceptible strains and in vitro expression of this isoform revealed that GluCl channels with exon 9c are less sensitive to both abamectin and glutamate than transcripts containing exon 9a, the primary variant observed in P. xylostella (Kwon et al., Citation2010; Liu et al., Citation2014). The finding that alternative exon usage in insect GluCls can influence insecticide binding/sensitivity was further supported by recent work characterising the transcriptional patterns of the GluCl of A. gambiae (Meyers et al., Citation2015). A total of four isoforms were identified in this species that differ in their splicing of exons 3, 8 and 10 and result in differences in the N-terminal extracellular domain and intracellular loop region. Functional expression of two different isoforms, performed to examine their influence on modulating responses to the natural ligand and insecticides, revealed specific isoforms that were sensitive (AgGluCl-a1) and insensitive (AgGluCl-b) to ivermectin. These results suggest that residues encoded by alternative exons 3, 8 and 10, at least in part, define sensitivity to ivermectin and also suggest resistance to this insecticide in A. gambiae could arise simply through altered regulation of AgGluCl to favour ivermectin insensitive transcripts (Meyers et al., Citation2015).
The nicotinic acetylcholine receptor
Subunit composition and structure of the nAChR
nAChRs are cation permeable ion channels that mediate fast synaptic transmission in the central nervous system of both vertebrates and invertebrates, and at the neuromuscular junction of vertebrates. nAChRs are the primary target of two major insecticide classes, the neonicotinoids, which comprise seven major compounds with a market share of more than 25% of total global insecticide sales (Bass, Denholm, Williamson, & Nauen, Citation2015b), and the natural product-derived spinosyns (Sparks, Crouse, & Durst, Citation2001).
nAChRs are oligomers composed of five subunits arranged in combinations from a variety of different subunit subtypes. These subunits form multiple functional heteropentamers or homopentamers, which have distinct functional and pharmacological profiles (Millar, Citation2003). Like the ligand-gated chloride channels discussed in the previous section, nAChR subunits have a similar topology comprising an N-terminal extracellular region containing the ligand-binding site and four transmembrane segments (TM1 – TM4) that create the ion channel (). Acetylcholine (and neonicotinoids) bind at the interface of two subunits, each of which contribute 3 binding loops (A–C by an α subunit and D–F by an α or non-α neighbouring subunit) to the binding pocket (Corringer, Le Novere, & Changeux, Citation2000). The first insect nAChR subunit sequence was isolated from Drosophila using probes designed on sequences from electric rays (Torpedo spp.), a rich source of this receptor (Hermans-Borgmeyer et al., Citation1986). Later sequencing of the Drosophila genome revealed a relatively compact family of 10 genes (Dα1–Dα7 and Dβ1–Dβ3) encoding nAChR subunits (Adams et al., Citation2000), a number subsequently found to be representative of most other insects (Jones & Sattelle, Citation2010). Despite the reduction in size of this gene family in insects when compared to vertebrates further research has revealed that the transcriptomic diversity generated by certain insect nAChR genes can be greatly expanded by posttranscriptional modifications such as alternative splicing and RNA editing (Grauso, Reenan, Culetto, & Sattelle, Citation2002).
Figure 2. (a) nAChRs share a similar overall structure to the ligand-gated chloride channels with individual subunits containing four trans-membrane domains (M1–M4) and a large extracellular N-terminal domain. The mature receptor (b) is a pentamer of either identical or non-identical subunits and gates a cation-selective channel following acetylcholine binding to the extracellular domain. Neonicotinoids such as imidacloprid activate the channel by binding to the same site. The only field-confirmed case of target site resistance to these compounds involves mutation (red circle) of arginine 81 to threonine (R81T) in Myzus persicae β1 subunit that causes repulsion of imidacloprid binding at the α/β subunit interface as shown in (c) (taken from Bass et al. (Citation2015)).
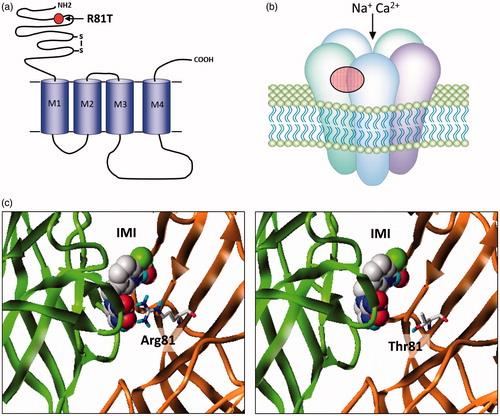
Functional expression of insect nAChR subunits and neonicotinoid binding
The subunit composition of human nAChR muscle-type receptors is well established (α12, β, γ, δ, or α12, β, ɛ, δ), and although neuronal subtypes are less well characterised various combinations of 12 different subunit subtypes (α2 − α10 and β2 − β4) have been defined (Millar, Citation2003). In contrast, much less is known about the composition of insect nAChRs, to a large degree a reflection of significant difficulties encountered in expressing insect-only subunits in heterologous systems (Millar & Lansdell, Citation2010). These issues have been somewhat circumvented by expressing insect nAChR subunits in combination with vertebrate subunits (such as vertebrate β2), or creating subunit chimeras containing ligand-binding domains of insect subunits (Millar & Lansdell, Citation2010). While these hybrid receptors are unlikely to fully reflect the native pharmacology of insect-only receptors they have proved useful to identify candidate neonicotinoid targets. For example, Lansdell and Millar demonstrated that Dα1, Dα2 and Dα3 form a high-affinity imidacloprid-binding site in Drosophila S2 cells when co-expressed with rat β2 subunits (Lansdell & Millar, Citation2000). Significantly, Dα3 (which showed highest affinity for imidacloprid) showed no specific binding of imidacloprid when co-expressed with alternative vertebrate non-α subunits such as rat neuronal β4, or muscle γ- or δ-subunits suggesting native Drosophila β-subunits also play an important role in defining the sensitivity of the nAChR to neonicotinoids.
Although the expression of hybrid receptors containing vertebrate β2 precludes the analysis of insect β receptors, mutagenesis of amino acid residues in important ligand-binding loops of vertebrate β receptors to make them more ‘insect-like’ has been shown to result in significant shifts in sensitivity to imidacloprid, further predicting the importance of insect β subunits in neonicotinoid binding (Shimomura et al., Citation2001, Citation2006). To further circumvent issues with functional expression in vitro, researchers have resorted to alternative approaches, such as immunohistochemistry, to characterise the subunit composition of insect nAChRs and their potential involvement in neonicotinoid binding. An excellent example is the combination of co-immunoprecipitation studies using subunit selective antisera and radioligand binding to native nAChR preparations of the brown planthopper, Nilaparvata lugens. These approaches provided evidence that the Nlα3, Nlα8 and Nlβ2 subunits of this species co-assemble in one complex to form a high affinity imidacloprid-binding site while Nlα1, Nlα2 and Nlβ1 assemble to form a lower affinity site (Li et al., Citation2010). More recent work expressing certain Drosophila α subunits (Dα5, Dα6 and Dα7) in combination with the molecular chaperone RIC-3 has successfully achieved the generation of functional homomeric and heteromeric insect only nAChRs, providing greater optimism that the composition and sensitivity of insect nAChRs to neonicotinoids may yet be determined using heterologous systems (Lansdell, Collins, Goodchild, & Millar, Citation2012; Watson et al., Citation2010).
The insect nAChR as a target for the spinosyns
In contrast to the neonicotinoids, the nAChR subtypes targeted by spinosyn insecticides are more clearly defined with this class of insecticides appearing to specifically target insect nAChR α6 subunits. Evidence for this, initially came from Drosophila, with a Dα6 knock-out mutant exhibiting >1000-fold resistance to spinosad (Perry, McKenzie, & Batterham, Citation2007). Subsequent studies on spinosad-resistant insect pests have provided substantial additional evidence that α6 is the primary target of spinosyns (see below). Very recently, the ease of genome engineering in Drosophila has been elegantly exploited in two studies to confirm both insect α6 nAChRs as the target of spinosad and localise the binding site of this insecticide (Perry, Somers, Yang, & Batterham, Citation2015; Somers, Nguyen, Lumb, Batterham, & Perry, Citation2015). In the first of these studies Perry et al. exploited two Drosophila mutants created by EMS mutagenesis, that are highly resistant to spinosad, to develop an in vivo rescue system (Perry et al., Citation2015). They then used this tool to demonstrate that while Dα1, Dα5 and Dα7 do not rescue the response to spinosad, four different isoforms of Dα6 and the α6 gene from three different pest insects are all able to rescue spinosad sensitivity. Insect α6 genes show a remarkable capacity to expand their transcriptional diversity through alternative splicing of two mutually exclusive exons (exon 3 and 8) and RNA A to I editing (Grauso et al., Citation2002). An important result of the study by Perry and co-workers was therefore the finding that expression of four Dα6 isoforms with different alternative exon 3 and exon 8 combinations resulted in significant difference in fly mortality to spinosad, despite the fact they were expressed in a uniform genetic background (Perry et al., Citation2015). This finding highlights the potential importance of alternative splicing in influencing the responsiveness of nAChRs to insecticides. In the second study, Somers et al. expressed two chimeric subunits, where the N-terminal half of Dα6 was fused to the C-terminal half of Dα7 (which exhibits >60% sequence similarity to Dα6) and vice versa, in a spinosad resistant, Dα6 null background to test their ability to rescue susceptibility to spinosad (Somers et al., Citation2015). Significantly, only subunits containing the C-terminal region of Dα6 were able to respond to spinosad, suggesting, in contrast to neonicotinoid insecticides, that this region is critical for spinosad binding.
Resistance-associated mutations and nAChR insecticide-binding sites
The molecular characterisation of spinosad and neonicotinoid resistance has shed light on the binding, and selectivity of these insecticides and the nAChR subunits that are important in these interactions. In the case of neonicotinoids, resistance in several insect species was initially attributed to metabolic mechanisms, and, to date, modification of the receptor (i.e. target-site resistance) has only been described in a handful of insect species. Prior to the development of ‘field-evolved’ neonicotinoid resistance, work on Drosophila provided valuable information on the nAChR subunit subtypes that may be important in forming the binding site of this insecticide class. EMS mutagenesis followed by selection with the neonicotinoid nitenpyram was used to identify four highly resistant lines with mutations in Dα1 and Dβ2 that confer resistance to this neonicotinoid (Perry, Heckel, McKenzie, & Batterham, Citation2008) and also other members of this class (Perry et al., Citation2012). This finding provided strong evidence that nAChRs containing either or both of these subunits form important sites of neonicotinoid action.
Target-site resistance to neonicotinoids was first described in an insect pest species in 2005 (Liu et al., Citation2005). Selection of a laboratory strain of the planthopper N. lugens with imidacloprid for 35 generations resulted in a strain with over 250-fold resistance compared to a susceptible reference. Radioligand binding experiments to whole body membrane preparations revealed a significantly lower level of [3H]imidacloprid-specific binding to preparations of the resistant strain and sequencing of N. lugens nAChR subunit genes identified a single point mutation at a conserved position (Y151S) in two nAChR subunits, Nlα1 and Nlα3. The causal effect of these mutations was examined by expression of hybrid nAChRs containing N. lugens α and rat β2 subunits, with the presence of Y151S associated with a substantial reduction in specific [3H]imidacloprid binding (Liu et al., Citation2005). The discovery of these mutations in the orthologs of Drosophila (Dα1 and Dα3), which have previously been shown to form a high-affinity binding site for imidacloprid (see above), provides additional evidence of their importance in neonicotinoid binding. Finally, the identification of the same mutation at homologous residues in two distinct nAChR α subunits is intriguing and it is unclear if Nlα1 and Nlα3 subunits contribute to different populations of receptors, conferring resistance to each, or if they occur together in a heteromeric receptor. It should be noted that this mutation combination, although able to confer strong resistance to neonicotinoids, may also carry an unacceptable fitness penalty as these mutations have never been reported in resistant field populations of N. lugens.
In the Green Peach aphid Myzus persicae, significant resistance to neonicotinoids used as aphicides has also taken a surprisingly long time to evolve with control compromising levels of resistance only described in a clone of M. persicae collected in 2009, nearly 20 years after the introduction of imidacloprid (Bass et al., Citation2011). Binding assays using radiolabelled imidacloprid to native membrane preparations revealed that the very high affinity site present in aphids, and indeed other hemipterans, was completely lost in the resistant clone and the remaining lower affinity site was also altered compared to susceptible clones resulting in a significant overall reduction in binding affinity to the nAChR. Sequencing of the previously characterised nAChR subunit genes from resistant and susceptible M. persicae clones revealed a point mutation in the nAChR β1 subunit of the resistant clone resulting in an arginine to threonine substitution or R81T (Slater, Paul, Andrews, Garbay, & Camblin, Citation2012) (). Remarkably, the amino acid at this position had been previously identified as a key determinant of the selectivity of neonicotinoids for insects, over vertebrates (Shimomura et al., Citation2006). The high sensitivity of insect nAChRs to neonicotinoids is thought to result, in part, from interactions between the distinctive electronegative pharmacophore (nitro or cyano group) of these insecticides and conserved positively charged residues in loop D (such as R81) of β subunits (Shimomura et al., Citation2006; Tomizawa & Casida, Citation2003, Citation2005). Indeed substituting the threonine residue in the chicken β2 subunit at this position with arginine or another basic residue greatly enhanced the affinity of heterologously expressed nAChRs (such as Drosophila Dα2/chicken β2 hybrids) for imidacloprid (Shimomura et al., Citation2006). The mutation identified in M. persicae therefore confers a ‘vertebrate-like’ quality to the β1 subunit of resistant aphids resulting in reduced sensitivity of the nAChR to neonicotinoids through the loss of direct electrostatic interactions of the electronegative pharmacophore with the basic arginine residue at this key position within loop D (). These results both provide further evidence of the importance of insect β1 subunits in forming the neonicotinoid-binding site and provide additional validation of the current models of neonicotinoid binding and selectivity for insect nAChRs. Unlike Y151S in planthoppers, the R81T mutation in M. persicae does not appear to carry a strong fitness penalty and is seen at high frequency in resistant aphid populations from peach growing regions of Southern Europe (Slater et al., Citation2012).
More recent work has suggested that quantitative changes in nAChR subunits may represent an alternative form of target-site resistance to neonicotinoids with resistance to imidacloprid in a laboratory-selected strain of the planthopper N. lugens associated with a reduction in both mRNA and protein levels of the nAChR subunit Nlα8 (Zhang et al., Citation2015). Functional validation of the observed down-regulation using RNA interference showed that knockdown of Nlα8 mRNA and protein levels causes a significant increase in imidacloprid resistance in N. lugens and demonstrated a decrease in Nlα8 expression is sufficient to confer resistance in vivo. As detailed above, previous work has indicated that Nlα8 co-assembles with Nlβ2 to form a high affinity imidacloprid-binding site. It is therefore significant that radioligand-binding assay on native membrane preparations of the resistant strain revealed that the affinity of this binding site was reduced over 10-fold compared to an earlier (and hence less resistant) generation of the same strain (Zhang et al., Citation2015).
In the case of spinosad resistance, target-site resistance appears to be the primary route of resistance evolution, likely because, in contrast to other conserved insecticide receptors, insect α6 subunits are not essential for viability and so can tolerate a variety of mutations even if they result in loss of function. In this regard a wide range of genetic alterations have been described that result in truncated non-functional protein (Baxter et al., Citation2010; Hsu et al., Citation2012; Rinkevich, Chen, Shelton, & Scott, Citation2010). In most of these cases, the mutations involved disrupt the normal pattern of alternative splicing of this gene. For example, an investigation of a spinosad-resistant strain of Diamondback moth, Plutella xylostella, collected in Hawaii, identified a mutation within the ninth intron splice junction of Pxα6 resulting in mis-splicing of transcripts and a predicted protein truncated between the third and fourth transmembrane domains (Baxter et al., Citation2010). More recently, spinosad resistance has been associated with two alternative point mutations that do not result in loss of function (Bao et al., Citation2014; Puinean, Lansdell, Collins, Bielza, & Millar, Citation2013; Silva et al., Citation2016; Somers et al., Citation2015). In the first case, the same non-synonymous point mutation resulting in a G275E substitution was identified in exon 9 of the α6 nAChR in three different pest insect species. An alternative amino acid replacement, P146S, was also recently associated with spinosad resistance in an EMS mutagenized line of Drosophila. As a result of recent advances in genome editing approaches the causality of this mutation and G275E were both demonstrated using the CRISPR/Cas9 system to edit the mutations into Drosophila and measure the effect of this on reduced sensitivity to spinosad (Somers et al., Citation2015; Zimmer et al., Citation2016). In contrast to mutations involving loss of the whole gene, specific amino acid replacements can also provide more specific information on the mode and site of insecticide binding. Homology modeling using the nematode glutamate-gated chloride channel structure predicts the G275E mutation to lie at the top of the third α-helical transmembrane domain of the nAChR α6 subunit, providing additional evidence that the transmembrane domain of α6 contains the spinosad-binding site (Puinean et al., Citation2013). In contrast, the P146S mutation is unlikely to reside in the spinosad-binding site and its close proximity to the conserved Cys-loop indicates an alternative mode of action potentially by impairing the gating of the receptor (Somers et al., Citation2015).
The Para containing voltage-gated sodium channel
Lessons from Drosophila
The screening of chemically induced Drosophila mutants for temperature sensitivity (Siddiqi & Benzer, Citation1976; Suzuki, Citation1970) has played an important role in isolating mutants of genes encoding important insecticide target sites. One such temperature sensitive paralytic mutant, termed paralytic (temperature sensitive) or parats, was isolated using a screen involving a shift of temperature from 22 °C to 29 °C upon which paralysis in parats flies is immediate but reversible (Suzuki, Grigliatti, & Williamson, Citation1971). The structural gene encoding the voltage-gated sodium channel was then cloned from the para locus via a chromosomal walk (Loughney, Kreber, & Ganetzky, Citation1989). The polypeptide encoded by this locus will here be referred to as ‘Para’ and the gene itself as ‘para’. The para locus was shown to be both large and complex, spanning over 60 kb of genomic DNA and with a minimum of 26 predicted exons, encoding numerous transcripts via alternative splicing (Loughney et al., Citation1989). Further studies in the Ganetzky laboratory showed that the para locus in fact has six different sites for alternative splicing and that at least 48 different splice forms could be generated (Thackeray & Ganetzky, Citation1994). The variety of para splice forms found differed markedly between embryos and adults suggesting that the adult nervous system may contain a very different complement of sodium channels (Thackeray & Ganetzky, Citation1994). This same pattern of alternative splicing is also conserved in D. virilis suggesting that this developmentally regulated variability does indeed have a functional role (Thackeray & Ganetzky, Citation1995). To add to this bewildering array of alternative splice forms, the Ganetzky laboratory subsequently showed that para also contains three sites where RNA editing occurs (Hanrahan, Palladino, Ganetzky, & Reenan, Citation2000). In the RNA editing process, post-transcriptional editing of pre-mRNAs, through the action of dsRNA adenosine deaminases, modifies adenosine (A) residues to inosine (I), which then alters the coding potential of modified transcripts. These three RNA editing sites were again developmentally regulated and again conserved in D. virilis, supporting their functional role (Hanrahan et al., Citation2000). At each position, a highly conserved region was found downstream of the RNA-editing site in the following intron, and this region matched the exonic-editing site (Hanrahan et al., Citation2000). This suggests a mechanism whereby the edited exon forms a base-paired secondary structure with the distant noncoding intronic sequence, in a similar manner to that seen in A-to-I RNA editing in vertebrate glutamate receptor subunits. More recently, the functional significance of both editing and splice variants has been investigated via the heterologous expression of a range of different Para variants (here alternatively termed DmNa(V)) in Xenopus oocytes (Olson, Liu, Nomura, Song, & Dong, Citation2008; Lin, Wright, Muraro, & Baines, Citation2009). These studies showed a wide range of voltage dependence in the gating properties of different DmNa(V) (Para) variants (Olson et al., Citation2008) and also differences in activation, inactivation and persistent current (Lin et al., Citation2009), proving that such variation does indeed have a meaningful biological role in generating insect sodium channel diversity. In summary, the para locus is large and complex and the variety of different mRNAs produced via a combination of both alternative splicing and RNA editing is large. Understanding the role of all of these different Para variants in the development and functioning of the insect nervous system therefore remains a major challenge.
Heterologous expression of Para
The Drosophila para sodium channel alpha subunit expresses alone when injected into Xenopus oocytes (Warmke et al., Citation1997). However, co-expression with the gene product of the temperature-induced paralysis, locus E (tipE), a putative accessory subunit, results in both elevated sodium currents and accelerated current decay (Feng, Deak, Chopra, & Hall, Citation1995; Warmke et al., Citation1997). The resulting Para/TipE sodium channels have both biophysical and pharmacological properties that are similar to native insect channels. However, they differ from the pharmacology of vertebrate sodium channels, most notably in their sensitivity to pyrethroid insecticides. Thus, the insect Para channel is over 100-fold more sensitive to pyrethroids than rat brain type IIA sodium channels (Warmke et al., Citation1997).
Resistance-associated mutations and insecticide binding
The voltage-gated sodium channel is the primary site of action of pyrethroid insecticides and in pest insects, such as the house fly, Musca domestica, and the German cockroach, Blattella germanica, pyrethroid resistance is associated with a locus originally termed knockdown resistance or kdr. The first evidence that the kdr locus might indeed encode a para-like channel came from observations of tight genetic linkage between kdr and molecular probes for the para-like gene in both house flies (Knipple et al., Citation1994; Williamson, Denholm, Bell, & Devonshire, Citation1993) and German cockroaches (Dong & Scott, Citation1994). Subsequently, sequencing of both the kdr and super-kdr alleles of the house fly showed that both mutants are associated with point mutations in domain II of the Para-like sodium channel (Williamson, Martinez-Torres, Hick, & Devonshire, Citation1996). Specifically, a leucine to phenylalanine replacement (L1014F) in the hydrophobic IIS6 transmembrane section is found in both kdr and super-kdr alleles and a second methionine to threonine replacement (M918T) within the intracellular IIS4-S5 loop is unique to super-kdr (Williamson et al., Citation1996). Similarly, an equivalent mutation (L1014F) was shown to be associated with kdr-like mutants of the German cockroach (Dong, Citation1997; Miyazaki, Ohyama, Dunlap, & Matsumura, Citation1996). These equivalent mutations in two different resistant insects suggested a binding site for pyrethroids at the intracellular mouth of the channel pore in a region thought to be important for channel inactivation (Williamson et al., Citation1996).
Interestingly, para-associated (kdr-like) pyrethroid resistance has not, to our knowledge, been isolated from field strains of D. melanogaster. Despite the fact that para is X-linked and therefore kdr-like resistance should be easy to indentify as it should be clearly sex linked. The absence of kdr-like (‘combined’ DDT and pyrethroid) resistance in field strains of Drosophila may be associated with the presence of the dominant DDT-R gene on chromosome II which confers resistance to DDT but ironically not to pyrethroids. However, we can still examine the effects of different point mutations on the insecticide sensitivity of the Drosophila Para channel as although the original parats mutants were isolated on the basis of their paralysis at high temperature, the same point mutations conferring temperature sensitivity also overlap with those conferring resistance to DDT and pyrethroids (Pittendrigh, Reenan, ffrench-Constant, & Ganetzky, Citation1997). Specifically, six out of 13 para mutants tested showed 10 to 30-fold resistance to DDT and the associated mutations were clustered into a few sites across the channel polypeptide (). First, within the intracellular loop between S4 and S5 in homology domains I and III, second, within the pore region of homology domain III and third, within S6 of homology domain III (Pittendrigh et al., Citation1997).
Figure 3. The voltage-gated sodium channel as a target for pyrethroids. (a) Diagram of the voltage gated sodium channel showing the four repeat domains (I-IV), each comprising six membrane spanning helices (S1-S6). The positions of resistance mutations that identify pyrethroid binding sites PyR1 (red circles) and PyR2 (blue circles) are highlighted. The proposed PyR1 (b) and PyR2 (c) sites are shown in more detail, taken from O'Reilly et al. (Citation2006) and Du et al. (Citation2013). Positions of the mutations originally identified in pyrethroid and DDT-resistant parats Drosophila strains are also shown (yellow circles).
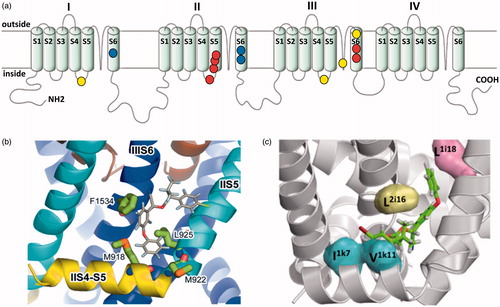
Numerous different pest species have now been shown to possess kdr-like mutations in their para homologous sodium channel genes (Davies, O'Reilly, Field, Wallace, & Williamson, Citation2008; Dong et al., Citation2014). However, the functionality of many of these mutations has not been tested via heterologous expression and mutagenesis. Current work on the mode of action of pyrethroids on the insect voltage-gated sodium channel is, however, now divided into two camps. Those that favour a single-binding site for pyrethroids (Davies et al., Citation2008; O'Reilly et al., Citation2006), as implicated by the original kdr and super-kdr mutations, and those that favour two different binding sites on the sodium channel polypeptide (). A single-binding site for fenvalerate and DDT has been predicted using a homology model of the house fly Para-like voltage gated sodium channel (O'Reilly et al., Citation2006). In this model, the sodium channel was modelled in the open conformation with the pyrethroid-binding site as a hydrophobic cavity formed by domain II of the S4-S5 linker and the IIS5 and IIS6 helices () (O'Reilly et al., Citation2006). Importantly, this binding site is predicted to be accessible to the lipid bilayer where the lipid-soluble insecticides are predicted to accumulate. Insecticide binding, and the consequent formation of contacts across different channel elements, is predicted to stabilize the open state of the channel. This stabilisation of the open state of the channel is consistent with the prolonged sodium tail currents induced by both pyrethroids and DDT (Vais et al., Citation2000). Conversely, in the closed state, repositioning of the domain II S4-S5 linker would disrupt this binding site and help explain why pyrethroids have a higher affinity for the open state of the sodium channel (O'Reilly et al., Citation2006). Importantly, some of the residues on the helices that form the putative-binding contacts are not conserved between vertebrates and invertebrates, which may help explain the 100-fold difference in pyrethroid potency discussed above. Similarly, additional binding contacts on the II S4-S5 linker (in the super-kdr region) may also explain the higher affinity binding of pyrethroids over DDT (O'Reilly et al., Citation2006) and why the potency of DDT is relatively unaffected by super-kdr (Usherwood et al., Citation2007). It should be noted that in this model the kdr site does not have a direct interaction with the pyrethroid molecule and mutations at this site are postulated to affect pyrethroid binding via an indirect (allosteric) impact that alters the conformation of the adjacent pyrethroid-binding site (Davies et al., Citation2008).
Evidence for a two binding site model comes from studies of kdr-like resistance in the Para-like sodium channel of the mosquitoes Aedes aegypti and A. gambiae. In these mosquitoes, two resistance associated mutations map to the same binding site predicted from the house fly model, termed pyrethroid receptor site or ‘PyR1’, located in domain interface II/III. However, three other resistance associated mutations apparently map to a second binding site on the receptor, termed pyrethroid receptor site 2 or ‘PyR2’, which is located in domain interface I/II () (Du et al., Citation2013). Further molecular modelling of the interaction between the mosquito sodium channel and deltamethrin suggests a possible additional binding site between the linker helix IL45 (domain I S4-S5 linker) and transmembrane helices IS5 and IIS6, with deltamethrin dibromoethenyl and diphenylether residues pointing in intra- and extra-cellular directions, respectively (Du, Nomura, Zhorov, & Dong, Citation2015). Finally, recent evidence suggests that pairs of mutations can work in concert to synergise pyrethroid resistance. Thus, a unique mutation, N1575Y in the cytoplasmic loop linking domains III and IV of the A. gambiae Para channel, confers no resistance on its own but synergises the effect of the previously identified L1014F replacement in IIS6 (Wang et al., Citation2015). Molecular modelling supports a mechanism whereby this second mutation (N1575Y) allosterically alters the PyR2-binding site via a small shift in IIS6 (Wang et al., Citation2015). It therefore appears that secondary mutations can accumulate within the resistant receptor causing allosteric changes in the mutant receptor, which accentuate the effect of the primary mutation. It will therefore be interesting to see if such effects can be proven for mutations in other receptors such as the secondary mutations seen around A301S in the Rdl-containing GABA receptor (see discussion above).
The calcium-activated calcium channel or ryanodine receptor
Structure of the ryanodine receptor
Ryanodine receptors (RyRs) are homotetrameric calcium release channels and are the largest known ion channels. RyRs mediate the controlled release of Ca2+ from intracellular stores to initiate a wide variety of cellular processes, not least excitation–contraction coupling in muscle tissues (Lanner, Georgiou, Joshi, & Hamilton, Citation2010). Mammals possess three RyR isoforms (RyR1–3) each derived from a separate gene that are localized in different tissues. In contrast insect RyRs are encoded by a single gene with an ORF of >15,000 bp (Sattelle, Cordova, & Cheek, Citation2008). RyRs are the target of ryanodine, a plant-derived alkaloid and natural insecticide, and the synthetic diamide insecticides, currently comprising the phthalic diamide flubendiamide and anthranilic diamides chlorantraniliprole and cyantraniliprole. The first insect RyR gene to be sequence characterised was from Drosophila, and was identified using a cDNA probe of the rabbit skeletal muscle RyR, with the receptor showing less than 50% sequence identity to mammalian isoforms (Takeshima et al., Citation1994). Early attempts at functional expression of the Drosophila receptor in Chinese hamster ovary cells were only partially successful (Xu, Bhat, Nishi, Takeshima, & Ma, Citation2000), however, subsequent expression of this receptor in insect Sf9 cells was much more robust, and sufficient to characterize the action of anthranilic diamides and to generate a stable cell line (Cordova et al., Citation2006). This system was further exploited to identify regions of the RyR that are critical for diamide binding (Tao et al., Citation2013). Replacement of a 46 amino acid segment of the Drosophila C-terminus with that from the plant parasitic nematode, Meloidogyne incognita, which is insensitive to anthranilic diamides, resulted in a functional RyR which lacked sensitivity to these compounds suggesting this region is critical to diamide sensitivity in insect RyRs (Tao et al., Citation2013). Beyond Drosophila, functional expression of the RyR of the model lepidopteran, Bombyx mori, has identified additional putative sites of diamide binding (Kato et al., Citation2009). HEK cells expressing modified B. mori RyRs with an N-terminal deletion (residues183–290) or where the 20% C-terminus (amino acids 4111–5084) was replaced with the rabbit RyR2 counterpart sequence, failed to produce Ca2+ mobilization in the presence of flubendiamide suggesting these two regions are essential for diamide sensitivity (Kato et al., Citation2009).
Insecticide resistance and insecticide-binding sites
Use of radiolabelled diamides and ryanodine on native muscle membrane preparations of the house fly suggested that the major anthranilic and phthalic diamide insecticides bind to insect RyRs at different allosterically coupled sites (Isaacs, Qi, Sarpong, & Casida, Citation2012). This finding was subsequently called into question by the result of work elucidating the molecular basis of diamide resistance in the diamondback moth, P. xylostella (Troczka et al., Citation2012). This species is notorious both as a pest of cruciferous crops and also for its ability to rapidly evolve resistance to insecticides used for control. Remarkably, reports of resistance in P. xylostella to diamides emerged just a few years after their introduction (Troczka et al., Citation2012; Wang & Wu, Citation2012). Sequencing of the RyR from strains collected from the Philippines and Thailand with over 200-fold resistance to both chlorantraniliprole and flubendiamide revealed different non-synonymous mutations in each of the resistant strains that in both cases lead to the same glycine to glutamic acid substitution (G4946E) in the transmembrane spanning region of the RyR () (Troczka et al., Citation2012). Subsequent radioligand-binding studies with P. xyostella thoracic microsomal membrane preparations provided direct evidence of the role of the G4946E mutation on diamide-specific binding with the binding of the tritiated flubendiamide derivative [3H]PAD1 profoundly reduced in preparations of the resistant strain (Steinbach et al., Citation2015). Finally, the expression of the P. xylostella RyR in Sf9 cells demonstrated that the sensitivity of heterologously expressed receptors to flubendiamide and chlorantraniliprole is dramatically reduced in cells stably expressing the G4946E-modified RyR, providing final unequivocal validation that the G4946E RyR mutation impairs diamide insecticide binding (Troczka et al., Citation2015).
Figure 4. Structure of the insect ryanodine receptor and the location of resistance associated mutations. (a) Topology of the C-terminal domain of the insect RyR showing the six membrane spanning helices (TM1–6) with the positions of the two resistance mutations (I4790M & G4946E) that map to this region of the channel highlighted. (b) Shows a full model of the insect RyR based on the recently reported structure of a rabbit RyR, with the membrane domain (boxed) further magnified in (c) to show the close proximity of the resistance-associated mutations (adapted from (Steinbach et al., Citation2015)).
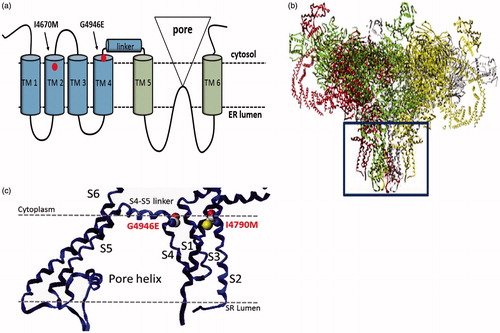
Sequencing of a second chlorantraniliprole-resistant field population of P. xylostella, this time from China, identified three novel amino acid substitutions, E1338D, Q4594L and I4790M at high frequency in addition to the G4946E mutation, which was observed at lower frequency (20%) (Guo, Liang, Zhou, & Gao, Citation2014). Crossing the resistant strain with a susceptible strain, and subsequent analysis of the frequency of these mutations in a subset of the F2 generation that had survived a diagnostic dose of chlorantraniliprole, showed an increase in frequency of all three novel mutations compared to an untreated subset of the F2 population. Less convincing were the results of fluorescent probe-based assays, which largely failed to provide compelling evidence of the functional significance for the novel mutations due to the low specificity of the diamide-like probe employed.
More recent homology modelling of the P. xylostella RyR based on a cryo-EM structure of rabbit RyR1 has been used to unambiguously locate two of the four mutations (G4946E, I4790M) in close proximity to each other in the voltage sensor domain () (Steinbach et al., Citation2015). The observation that G4946E is located in trans-membrane helix S4 close to the S4–S5 linker domain thought to be involved in the modulation of the voltage sensor suggests that the wild-type residue in this position may act as a glycine hinge at the interface between the S4 helix and the S4-S5 linker. The mutation to glutamic acid observed in resistant strains is therefore likely to have a significant impact on movement of the S5 and S6 helices that control channel gating, and hence a direct knock on effect on binding of diamide insecticides to the receptor. Interestingly, the I4790M occurs in helix S2 opposite and in close proximity to G4946E and together these two residues may define the diamide-binding site on the receptor () (Steinbach et al., Citation2015; Troczka et al., Citation2015). Interestingly I4790 is conserved in Lepidoptera, with all other insects and arachnids exhibiting alternative amino acids at this position suggesting this residue may in part underlie the species-specific differences exhibited in flubendiamide and chlorantraniliprole binding at the RyR (see below).
Contrary to the conclusions drawn from the work on the house fly (Isaacs et al., Citation2012), the observed cross-resistance of resistant strains of P. xylostella to anthranilic and phthalic diamides, and the demonstration that the G4946E mutation results in insensitivity to both classes suggests that they share a common binding site. Further comparison of diamide binding to thoracic muscle preparations of Musca domestica and Heliothis virescens using [3H] ryanodine [3H]chlorantraniliprole and [3H]flubendiamide reconciled these two studies by providing evidence that there may be more than one type of diamide site in insects and species-specific differences in diamide binding at the receptor (Qi, Luemmen, Nauen, & Casida, Citation2014). In the housefly, Musca domestica, binding data suggest [3H]Chlo, [3H]Ry and [3H]Flu bind at three distinct sites, however, in the caterpillar pest, Heliothis, anthranilic and phthalic diamide insecticides displace each other suggesting they bind at the same or closely coupled sites (Qi et al., Citation2014).
Conclusions and future directions
This brief over-view of the five major ion channel targets for current small molecule insecticides has attempted to emphasize how our knowledge of these important receptors in pest insects has been enriched by studies in the genetic model Drosophila. Whilst studies of insecticide-resistance-associated mutations have identified not only the receptor subunits to which the insecticides bind but also their likely binding sites on those subunits. Importantly, however, studies of insecticide binding have also reinforced important lessons about receptor biophysics. Thus, it is clear that many insecticides (such as the cyclodienes and pyrethroids) bind to specific biophysical forms of their receptors (the cyclodienes binding preferentially to the desensitized state of the insect GABA receptor for example). Critically therefore, insecticide-resistance-associated mutations can either be directly within the insecticide-binding site and/or they can alter the stability of the drug preferred conformation of the receptor. This begins to explain how mutations within the drug-binding site can be synergized by distant mutations that act allosterically on the drug preferred conformation of the receptor. It will therefore be important in the future to examine the growing number of target site mutations in the light of this dual role hypothesis.
Acknowledgements
This article is a review for a special issue of the Journal of Neurogenetics to honour the lifetime achievements of Barry Ganetzky from the University of Wisconsin-Madison.
Disclosure statement
The authors report no conflicts of interest. The authors alone are responsible for the content and writing of this article.
References
- Adams, M.D., Celniker, S.E., Holt, R.A., Evans, C.A., Gocayne, J.D., Amanatides, P.G., …. Craig Venter, J. (2000). The genome sequence of Drosophila melanogaster. Science, 287, 2185–2195. doi:10.1126/science.287.5461.2185.
- Bao, W.X., Narai, Y., Nakano, A., Kaneda, T., Murai, T., & Sonoda, S. (2014). Spinosad resistance of melon thrips, Thrips palmi, is conferred by G275E mutation in α6 subunit of nicotinic acetylcholine receptor and cytochrome P450 detoxification. Pesticide Biochemistry and Physiology, 112, 51–55. doi:10.1016/j.pestbp.2014.04.013.
- Barnard, E.A., Bilbe, G., Houamed, K., Moss, S.J., Van Renterghem, C., & Smart, T.G. (1987). Functional expression in the Xenopus oocyte of messenger ribonucleic acids encoding brain neurotransmitter receptors: Further characterisation of the implanted GABA receptor. Neuropharmacology, 26, 837–844. doi:10.1016/0028-3908(87)90060-8.
- Bass, C., Denholm, I., Williamson, M.S., & Nauen, R. (2015). The global status of insect resistance to neonicotinoid insecticides. Pesticide Biochemistry and Physiology, 121, 78–87. doi:10.1016/j.pestbp.2015.04.004.
- Bass, C., Puinean, A.M., Andrews, M., Cutler, P., Daniels, M., Elias, J., …Slater, Ret al. (2011). Mutation of a nicotinic acetylcholine receptor β subunit is associated with resistance to neonicotinoid insecticides in the aphid Myzus persicae. BMC Neuroscience, 12, 51. doi:10.1186/1471-2202-12-51.
- Baxter, S.W., Chen, M., Dawson, A., Zhao, J.Z., Vogel, H., Shelton, A.M., … Jiggins, C.D. (2010). Mis-spliced transcripts of nicotinic acetylcholine receptor alpha6 are associated with field evolved spinosad resistance in Plutella xylostella (L.). PLoS Genetics, 6, e1000802. doi:10.1371/journal.pgen.1000802.
- Bloomquist, J.R. (1993). Toxicology, mode of action and target site-mediated resistance to insecticides acting on chloride channels. Comparative Biochemistry and Physiology. C, Comparative Pharmacology and Toxicology, 106, 301–314. doi:10.1016/0742-8413(93)90138-B.
- Bloomquist, J.R. (1996). Ion channels as targets for insecticides. Annual Review of Entomology, 41, 163–190. doi:10.1146/annurev.en.41.010196.001115.
- Buckingham, S.D., Biggin, P.C., Sattelle, B.M., Brown, L.A., & Sattelle, D.B. (2005). Insect GABA receptors: splicing, editing, and targeting by antiparasitics and insecticides. Molecular Pharmacology, 68, 942–951. doi:10.1124/mol.105.015313.
- Casida, J.E., & Durkin, K.A. (2013). Neuroactive insecticides: targets, selectivity, resistance, and secondary effects. Annual Review of Entomology, 58, 99–117. doi:10.1146/annurev-ento-120811-153645.
- Casida, J.E. & Durkin, K.A. (2015). Novel GABA receptor pesticide targets. Pesticide Biochemistry and Physiology, 121, 22–30. doi:10.1016/j.pestbp.2014.11.006.
- Cordova, D., Benner, E.A., Sacher, M.D., Rauh, J.J., Sopa, J.S., Lahm, G.P., …Tao, Y. (2006). Anthranilic diamides: A new class of insecticides with a novel mode of action, ryanodine receptor activation. Pesticide Biochemistry and Physiology, 84, 196–214. doi:10.1016/j.pestbp.2005.07.005.
- Corringer, P.J., Le Novere, N., & Changeux, J.P. (2000). Nicotinic receptors at the amino acid level. Annual Review of Pharmacology and Toxicology, 40, 431–458. doi:10.1146/annurev.pharmtox.40.1.431.
- Cully, D.F., Paress, P.S., Liu, K.K., Schaeffer, J.M., & Arena, J.P. (1996). Identification of a Drosophila melanogaster glutamate-gated chloride channel sensitive to the antiparasitic agent avermectin. Journal of Biological Chemistry, 271, 20187–20191. doi:10.1074/jbc.271.33.20187.
- Davies, T.G.E., O'Reilly, A.O., Field, L.M., Wallace, B., & Williamson, M.S. (2008). Knockdown resistance to DDT and pyrethroids: from target-site mutations to molecular modelling. Pest Management Science, 64, 1126–1130. doi:10.1002/ps.1617.
- Dermauw, W., Ilias, A., Riga, M., Tsagkarakou, A., Grbic, M., Tirry, L., … Vontas, J. (2012). The cys-loop ligand-gated ion channel gene family of Tetranychus urticae: Implications for acaricide toxicology and a novel mutation associated with abamectin resistance. Insect Biochemistry and Molecular Biology, 42, 455–465. doi:10.1016/j.ibmb.2012.03.002.
- Dong, K. (1997). A single amino acid change in the para sodium channel protein is associated with knockdown-resistance (kdr) to pyrethroid insecticides in German cockroach. Insect Biochemistry and Molecular Biology, 27, 93–100. doi:10.1016/S0965-1748(96)00082-3.
- Dong, K., Du, Y., Rinkevich, F., Nomura, Y., Xu, P., Wang, L., … Zhorov, B.S. (2014). Molecular biology of insect sodium channels and pyrethroid resistance. Insect Biochemistry and Molecular Biology, 50, 1–17. doi:10.1016/j.ibmb.2014.03.012.
- Dong, K. & Scott, J.G. (1994). Linkage of kdr-type resistance and the para-homologous sodium channel gene in German cockroaches (Blattella germanica). Insect Biochemistry and Molecular Biology, 24, 647–654. doi:10.1016/0965-1748(94)90051-5.
- Du, Y., Nomura, Y., Satar, G., Hu, Z., Nauen, R., He, S.Y., … Dong, K. (2013). Molecular evidence for dual pyrethroid-receptor sites on a mosquito sodium channel. Proceedings of the National Academy of Sciences of the United States of America, 110, 11785–11790. doi:10.1073/pnas.1305118110.
- Du, Y., Nomura, Y., Zhorov, B.S., & Dong, K. (2015). Rotational symmetry of two pyrethroid receptor sites in the mosquito sodium channel. Molecular Pharmacology, 88, 273–280. doi:10.1124/mol.115.098707.
- Feng, G., Deak, P., Chopra, M., & Hall, L.M. (1995). Cloning and functional analysis of TipE, a novel membrane protein that enhances Drosophila para sodium channel function. Cell, 82, 1001–1011. doi:10.1016/0092-8674(95)90279-1.
- ffrench-Constant, R.H. (1994). The molecular and population genetics of cyclodiene insecticide resistance. Insect Biochemistry and Molecular Biology, 24, 335–345. doi:10.1016/0965-1748(94)90026-4.
- ffrench-Constant, R.H. & Rocheleau, T.A. (1993). Drosophila gamma-aminobutyric acid receptor gene Rdl shows extensive alternative splicing. Journal of Neurochemistry, 60, 2323–2326. doi:10.1111/j.1471-4159.1993.tb03523.x.
- ffrench-Constant, R.H., Rocheleau, T.A., Steichen, J.C., & Chalmers, A.E. (1993). A point mutation in a Drosophila GABA receptor confers insecticide resistance. Nature, 363, 449–451. doi:10.1038/363449a0.
- ffrench-Constant, R.H., Steichen, J.C., & Ode, P.J. (1993). Cyclodiene insecticide resistance in Drosophila-melanogaster (Meigen) is associated with a temperature-sensitive phenotype. Pesticide Biochemistry and Physiology, 46, 73–77. doi:10.1006/pest.1993.1038.
- Grauso, M., Reenan, R.A., Culetto, E., & Sattelle, D.B. (2002). Novel putative nicotinic acetylcholine receptor subunit genes, Dα5, Dα6 and Dα7, in Drosophila melanogaster identify a new and highly conserved target of adenosine deaminase acting on RNA-mediated A-to-I pre-mRNA editing. Genetics, 160, 1519–1533.
- Guo, L., Liang, P., Zhou, X., & Gao, X. (2014). Novel mutations and mutation combinations of ryanodine receptor in a chlorantraniliprole resistant population of Plutella xylostella (L.). Scientific Reports, 4, 6924. doi:10.1038/srep06924.
- Hanrahan, C.J., Palladino, M.J., Ganetzky, B., & Reenan, R.A. (2000). RNA editing of the Drosophila para Na(+) channel transcript. Evolutionary conservation and developmental regulation. Genetics, 155, 1149–1160.
- Henderson, J.E., Knipple, D.C., & Soderlund, D.M. (1994). PCR-based homology probing reveals a family of GABA receptor-like genes in Drosophila melanogaster. Insect Biochemistry and Molecular Biology, 24, 363–371. doi:10.1016/0965-1748(94)90029-9.
- Hermans-Borgmeyer, I., Zopf, D., Ryseck, R.P., Hovemann, B., Betz, H., & Gundelfinger, E.D. (1986). Primary structure of a developmentally regulated nicotinic acetylcholine receptor protein from Drosophila. The EMBO Journal, 5, 1503–1508.
- Hsu, J.C., Feng, H.T., Wu, W.J., Geib, S.M., Mao, C.H., & Vontas, J. (2012). Truncated transcripts of nicotinic acetylcholine subunit gene Bdα6 are associated with spinosad resistance in Bactrocera dorsalis. Insect Biochemistry and Molecular Biology, 42, 806–815. doi:10.1016/j.ibmb.2012.07.010.
- Isaacs, A.K., Qi, S., Sarpong, R., & Casida, J.E. (2012). Insect ryanodine receptor: Distinct but coupled insecticide binding sites for [N-(C3)H3] chlorantraniliprole, flubendiamide, and [3H] ryanodine. Chemical Research in Toxicology, 25, 1571–1573. doi:10.1021/tx300326m.
- Jones, A.K., Buckingham, S.D., Papadaki, M., Yokota, M., Sattelle, B.M., Matsuda, K., & Sattelle, D.B. (2009). Splice-variant- and stage-specific RNA editing of the Drosophila GABA receptor modulates agonist potency. The Journal of Neuroscience: The Official Journal of the Society for Neuroscience, 29, 4287–4292. doi:10.1523/JNEUROSCI.5251-08.2009.
- Jones, A.K., & Sattelle, D.B. (2007). The cys-loop ligand-gated ion channel gene superfamily of the red flour beetle, Tribolium castaneum. BMC Genomics, 8, 327. doi:10.1186/1471-2164-8-327.
- Jones, A.K. & Sattelle, D.B. (2010). Diversity of insect nicotinic acetylcholine receptor subunits. Advances in Experimental Medicine and Biology, 683, 25–43. doi:10.1007/978-1-4419-6445-8_3.
- Kane, N.S., Hirschberg, B., Qian, S., Hunt, D., Thomas, B., Bronchu, R., …. Cully, D.F. (2000). Drug-resistant Drosophila indicate glutamate-gated chloride channels are targets for the antiparasitics nodulisporic acid and ivermectin. Proceedings of the National Academy of Sciences of the United States of America, 97, 13949–13954. doi:10.1073/pnas.240464697.
- Kato, K., Kiyonaka, S., Sawaguchi, Y., Tohnishi, M., Masaki, T., Yasokawa, N., …. Mori, Y. (2009). Molecular characterization of flubendiamide sensitivity in the lepidopterous ryanodine receptor Ca2+ release channel. Biochemistry, 48, 10342–10352. doi:10.1021/bi900866s.
- Knipple, D.C., Doyle, K.E., Marsella-Herrick, P.A., & Soderlund, D.M. (1994). Tight genetic linkage between the KDR insecticide resistance trait and a voltage-sensitive sodium channel gene in the house fly. Proceedings of the National Academy of Sciences of the United States of America, 91, 2483–2487. doi:10.1073/pnas.91.7.2483.
- Knipple, D.C. & Soderlund, D.M. (2010). The ligand-gated chloride channel gene family of Drosophila melanogaster. Pestcide Biochemistry and Physiology, 97, 140–148. doi:10.1016/j.pestbp.2009.09.002.
- Kwon, D.H., Yoon, K.S., Clark, J.M., & Lee, S.H. (2010). A point mutation in a glutamate-gated chloride channel confers abamectin resistance in the two-spotted spider mite, Tetranychus urticae Koch. Insect Molecular Biology, 19, 583–591. doi:10.1111/j.1365-2583.2010.01017.x.
- Lanner, J.T., Georgiou, D.K., Joshi, A.D., & Hamilton, S.L. (2010). Ryanodine receptors: Structure, expression, molecular details, and function in calcium release. Cold Spring Harbor Perspectives in Biology, 2, a003996. doi:10.1101/cshperspect.a003996.
- Lansdell, S.J., Collins, T., Goodchild, J., & Millar, N.S. (2012). The Drosophila nicotinic acetylcholine receptor subunits Dα5 and Dα7 form functional homomeric and heteromeric ion channels. BMC Neuroscience, 13, 73. doi: 10.1186/1471-2202-13-73.
- Lansdell, S.J. & Millar, N.S. (2000). The influence of nicotinic receptor subunit composition upon agonist, alpha-bungarotoxin and insecticide (imidacloprid) binding affinity. Neuropharmacology, 39, 671–679. doi:10.1016/S0028-3908(99)00170-7.
- Le Goff, G., Hamon, A., Berge, J.B., & Amichot, M. (2005). Resistance to fipronil in Drosophila simulans: influence of two point mutations in the RDL GABA receptor subunit. Journal of Neurochemistry, 92, 1295–1305. doi:10.1111/j.1471-4159.2004.02922.x.
- Li, J., Shao, Y., Ding, Z., Bao, H., Liu, Z., Han, Z., & Millar, N.S. (2010). Native subunit composition of two insect nicotinic receptor subtypes with differing affinities for the insecticide imidacloprid. Insect Biochemistry and Molecular Biology, 40, 17–22. doi:10.1016/j.ibmb.2009.12.003.
- Lin, W.H., Wright, D.E., Muraro, N.I., & Baines, R.A. (2009). Alternative splicing in the voltage-gated sodium channel DmNav regulates activation, inactivation, and persistent current. Journal of Neurophysiology, 102, 1994–2006. doi:10.1152/jn.00613.2009.
- Liu, F., Shi, X., Liang, Y., Wu, Q., Xu, B., Xie, W., … Liu, N. (2014). A 36-bp deletion in the alpha subunit of glutamate-gated chloride channel contributes to abamectin resistance in Plutella xylostella. Entomologia Experimentalis et Applicata, 153, 85–92. doi:10.1111/eea.12232.
- Liu, Z.W., Williamson, M.S., Lansdell, S.J., Denholm, I., Han, Z.J., & Millar, N.S. (2005). A nicotinic acetylcholine receptor mutation conferring target-site resistance to imidacloprid in Nilaparvata lugens (brown planthopper). Proceedings of the National Academy of Sciences of the United States of America, 102, 8420–8425. doi:10.1073/pnas.0502901102.
- Loughney, K., Kreber, R., & Ganetzky, B. (1989). Molecular analysis of the para locus, a sodium channel gene in Drosophila. Cell, 58, 1143–1154. doi:10.1016/0092-8674(89)90512-6.
- Meyers, J.I., Gray, M., Kuklinski, W., Johnson, L.B., Snow, C.D., Black, W.C., … Foy, B.D. (2015). Characterization of the target of ivermectin, the glutamate-gated chloride channel, from Anopheles gambiae. Journal of Experimental Biology, 218, 1478–1486. doi:10.1242/jeb.118570.
- Millar, N.S. (2003). Assembly and subunit diversity of nicotinic acetylcholine receptors. Biochemical Society Transactions, 31, 869–874. doi:10.1042/bst0310869.
- Millar, N.S. & Lansdell, S.J. (2010). Characterisation of insect nicotinic acetylcholine receptors by heterologous expression. Advances in Experimental Medicine and Biology, 683, 65–73.
- Miyazaki, M., Ohyama, K., Dunlap, D.Y., & Matsumura, F. (1996). Cloning and sequencing of the para-type sodium channel gene from susceptible and kdr-resistant German cockroaches (Blattella germanica) and house fly (Musca domestica). Molecular & General Genetics: MGG, 252, 61–68. doi:10.1007/s004389670007.
- Nakao, T., Kawase, A., Kinoshita, A., Abe, R., Hama, M., Kawahara, N., & Hirase, K. (2011). The A2'N mutation of the RDL gamma-aminobutyric acid receptor conferring fipronil resistance in Laodelphax striatellus (Hemiptera: Delphacidae). Journal of Economic Entomology, 104, 646–652. doi:10.1603/EC10391.
- O'Reilly, A.O., Khambay, B.P., Williamson, M.S., Field, L.M., Wallace, B.A., & Davies, T.G.E. (2006). Modelling insecticide-binding sites in the voltage-gated sodium channel. The Biochemical Journal, 396, 255–263. doi:10.1042/BJ20051925.
- Olson, R.O., Liu, Z., Nomura, Y., Song, W., & Dong, K. (2008). Molecular and functional characterization of voltage-gated sodium channel variants from Drosophila melanogaster. Insect Biochemistry and Molecular Biology, 38, 604–610. doi:10.1016/j.ibmb.2008.01.003.
- Perry, T., Chan, J.Q., Batterham, P., Watson, G.B., Geng, C., & Sparks, T.C. (2012). Effects of mutations in Drosophila nicotinic acetylcholine receptor subunits on sensitivity to insecticides targeting nicotinic acetylcholine receptors. Pesticide Biochemistry and Physiology, 102, 56–60. doi:10.1016/j.pestbp.2011.10.010.
- Perry, T., Heckel, D.G., McKenzie, J.A., & Batterham, P. (2008). Mutations in Dα1 or Dβ2 nicotinic acetylcholine receptor subunits can confer resistance to neonicotinoids in Drosophila melanogaster Insect Biochemistry and Molecular Biology, 38, 520–528. doi:10.1016/j.ibmb.2007.12.007.
- Perry, T., McKenzie, J.A., & Batterham, P. (2007). A knockout strain of Drosophila melanogaster confers a high level of resistance to spinosad. Insect Biochemistry and Molecular Biology, 37, 184–188. doi:10.1016/j.ibmb.2006.11.009.
- Perry, T., Somers, J., Yang, Y.T., & Batterham, P. (2015). Expression of insect α6-like nicotinic acetylcholine receptors in Drosophila melanogaster highlights a high level of conservation of the receptor:spinosyn interaction. Insect Biochemistry and Molecular Biology, 64, 106–115. doi:10.1016/j.ibmb.2015.01.017.
- Pittendrigh, B., Reenan, R., ffrench-Constant, R.H., & Ganetzky, B. (1997). Point mutations in the Drosophila sodium channel gene para associated with resistance to DDT and pyrethroid insecticides. Molecular & General Genetics: MGG, 256, 602–610. doi:10.1007/s004380050608.
- Puinean, A.M., Lansdell, S.J., Collins, T., Bielza, P., & Millar, N.S. (2013). A nicotinic acetylcholine receptor transmembrane point mutation (G275E) associated with resistance to spinosad in Frankliniella occidentalis. Journal of Neurochemistry, 124, 590–601. doi:10.1111/jnc.12029.
- Qi, S., Luemmen, P., Nauen, R., & Casida, J.E. (2014). Diamide insecticide target site specificity in the Heliothis and Musca ryanodine receptors relative to toxicity. Journal of Agricultural and Food Chemistry, 62, 4077–4082. doi:10.1021/jf501236h.
- Remnant, E.J., Good, R.T., Schmidt, J.M., Lumb, C., Robin, C., Daborn, P.J., & Batterham, P. (2013). Gene duplication in the major insecticide target site, RDL, in Drosophila melanogaster. Proceedings of the National Academy of Sciences of the United States of America, 110, 14705–14710. doi:10.1073/pnas.1311341110.
- Remnant, E.J., Morton, C.J., Daborn, P.J., Lumb, C., Yang, Y.T., Ng, H.L., … Batterham, P. (2014). The role of Rdl in resistance to phenylpyrazoles in Drosophila melanogaster. Insect Biochemistry and Molecular Biology, 54, 11–21. doi:10.1016/j.ibmb.2014.08.008.
- Rinkevich, F.D., Chen, M., Shelton, A.M., & Scott, J.G. (2010). Transcripts of the nicotinic acetylcholine receptor subunit gene Pxylα6 with premature stop codons are associated with spinosad resistance in diamondback moth, Plutella xylostella. Invertebrate Neuroscience, 10, 25–33. doi:10.1007/s10158-010-0102-1.
- Sattelle, D.B., Cordova, D., & Cheek, T.R. (2008). Insect ryanodine receptors: Molecular targets for novel pest control chemicals. Invertebrate Neuroscience, 8, 107–119. doi:10.1007/s10158-008-0076-4.
- Shimomura, M., Okuda, H., Matsuda, K., Komai, K., Akamatsu, M., & Sattelle, D.B. (2001). Effects of mutations of a glutamine residue in loop D of the alpha7 nicotinic acetylcholine receptor on agonist profiles for neonicotinoid insecticides and related ligands. British Journal of Pharmacology, 137, 162–169. doi:10.1038/sj.bjp.0704848.
- Shimomura, M., Yokota, M., Ihara, M., Akamatsu, M., Sattelle, D.B., & Matsuda, K. (2006). Role in the selectivity of neonicotinoids of insect-specific basic residues in loop D of the nicotinic acetylcholine receptor agonist binding site. Molecular Pharmacology, 70, 1255–1263. doi:10.1124/mol.106.026815.
- Shotkoski, F., Lee, H.J., Zhang, H.G., Jackson, M.B., & ffrench-Constant, R.H. (1994). Functional expression of insecticide-resistant GABA receptors from the mosquito Aedes aegypti. Insect Molecular Biology, 3, 283–287. doi:10.1111/j.1365-2583.1994.tb00179.x.
- Siddiqi, O., & Benzer, S. (1976). Neurophysiological defects in temperature-sensitive paralytic mutants of Drosophila melanogaster. Proceedings of the National Academy of Sciences of the United States of America, 73, 3253–3257. doi:10.1073/pnas.73.9.3253.
- Silva, W.M., Berger, M., Bass, C., Williamson, M., Moura, D.M., Ribeiro, L.M., & Siqueira, H.A. (2016). Mutation (G275E) of the nicotinic acetylcholine receptor α6 suresistance to spinosyns in Tuta absoluta (Meyrick) (Lepidoptera: Gelechiidae). Pesticide Biochemistry and Physiology, 131, 1–8. doi:10.1016/j.pestbp.2016.02.006.
- Slater, R., Paul, V.L., Andrews, M., Garbay, M., & Camblin, P. (2012). Identifying the presence of neonicotinoid resistant peach-potato aphid (Myzus persicae) in the peach-growing regions of southern France and northern Spain. Pest Management Science, 68, 634–638. doi:10.1002/ps.2307.
- Somers, J., Nguyen, J., Lumb, C., Batterham, P., & Perry, T. (2015). In vivo functional analysis of the Drosophila melanogaster nicotinic acetylcholine receptor Dα6 using the insecticide spinosad. Insect Biochemistry and Molecular Biology, 64, 116–127. doi:10.1016/j.ibmb.2015.01.018.
- Sparks, T.C., Crouse, G.D., & Durst, G. (2001). Natural products as insecticides: the biology, biochemistry and quantitative structure-activity relationships of spinosyns and spinosoids. Pest Management Science, 57, 896–905. doi:10.1002/ps.358.
- Steinbach, D., Gutbrod, O., Luemmen, P., Matthiesen, S., Schorn, C., & Nauen, R. (2015). Geographic spread, genetics and functional characteristics of ryanodine receptor based target-site resistance to diamide insecticides in diamondback moth, Plutella Xylostella. Insect Biochemistry and Molecular Biology, 63, 14–22. doi:10.1016/j.ibmb.2015.05.001.
- Stilwell, G.E., Rocheleau, T., & ffrench-Constant, R.H. (1995). GABA receptor minigene rescues insecticide resistance phenotypes in Drosophila. Journal of Molecular Biology, 253, 223–227. doi:10.1006/jmbi.1995.0547.
- Suzuki, D.T. (1970). Temperature-sensitive mutations in Drosophila melanogaster. Science, 170, 695–706. doi:10.1126/science.170.3959.695.
- Suzuki, D.T., Grigliatti, T., & Williamson, R. (1971). Temperature-sensitive mutations in Drosophila melanogaster. VII. A mutation (para-ts) causing reversible adult paralysis. Proceedings of the National Academy of Sciences of the United States of America, 68, 890–893. doi:10.1073/pnas.68.5.890.
- Takeshima, H., Nishi, M., Iwabe, N., Miyata, T., Hosoya, T., Masai, I., & Hotta, Y. (1994). Isolation and characterization of a gene for a ryanodine receptor/calcium release channel in Drosophila melanogaster. FEBS Lett, 337, 81–87. doi:10.1016/0014-5793(94)80634-9.
- Tao, Y., Gutteridge, S., Benner, E.A., Wu, L., Rhoades, D.F., Sacher, M.D., … Cordova, D. (2013). Identification of a critical region in the Drosophila ryanodine receptor that confers sensitivity to diamide insecticides. Insect Biochemistry and Molecular Biology, 43, 820–828. doi:10.1016/j.ibmb.2013.06.006.
- Taylor-Wells, J., Brooke, B.D., Bermudez, I., & Jones, A.K. (2015). The neonicotinoid imidacloprid, and the pyrethroid deltamethrin, are antagonists of the insect Rdl GABA receptor. Journal of Neurochemistry, 135, 705–713. doi:10.1111/jnc.13290.
- Thackeray, J.R. & Ganetzky, B. (1994). Developmentally regulated alternative splicing generates a complex array of Drosophila para sodium channel isoforms. The Journal of Neuroscience: The Official Journal of the Society for Neuroscience, 14, 2569–2578.
- Thackeray, J.R. & Ganetzky, B. (1995). Conserved alternative splicing patterns and splicing signals in the Drosophila sodium channel gene para. Genetics, 141, 203–214.
- Tomizawa, M. & Casida, J.E. (2003). Selective toxicity of neonicotinoids attributable to specificity of insect and mammalian nicotinic receptors. Annual Review of Entomology, 48, 339–364. doi:10.1146/annurev.ento.48.091801.112731.
- Tomizawa, M. & Casida, J.E. (2005). Neonicotinoid insecticide toxicology: Mechanisms of selective action. Annual Review of Pharmacology and Toxicology, 45, 247–268. doi:10.1146/annurev.pharmtox.45.120403.095930.
- Troczka, B., Zimmer, C.T., Elias, J., Schorn, C., Bass, C., Davies, T.G.E., …. Nauen, R. (2012). Resistance to diamide insecticides in diamondback moth, Plutella xylostella (Lepidoptera: Plutellidae) is associated with a mutation in the membrane-spanning domain of the ryanodine receptor. Insect Biochemistry and Molecular Biology, 42, 873–880. doi:10.1016/j.ibmb.2012.09.001.
- Troczka, B.J., Williams, A.J., Williamson, M.S., Field, L.M., Luemmen, P., & Davies, T.G.E. (2015). Stable expression and functional characterisation of the diamondback moth ryanodine receptor G4946E variant conferring resistance to diamide insecticides. Scientific Reports, 5, 14680. doi:10.1038/srep14680.
- Usherwood, P.N., Davies, T.G.E., Mellor, I.R., O'Reilly, A.O., Peng, F., Vais, H., … Williamson, M.S. (2007). Mutations in DIIS5 and the DIIS4-S5 linker of Drosophila melanogaster sodium channel define binding domains for pyrethroids and DDT. FEBS Letters, 581, 5485–5492. doi:10.1016/j.febslet.2007.10.057.
- Vais, H., Williamson, M.S., Goodson, S.J., Devonshire, A.L., Warmke, J.W., Usherwood, P.N., & Cohen, C.J. (2000). Activation of Drosophila sodium channels promotes modification by deltamethrin. Reductions in affinity caused by knock-down resistance mutations. The Journal of General Physiology, 115, 305–318. doi:10.1085/jgp.115.3.305.
- Wang, L., Nomura, Y., Du, Y., Liu, N., Zhorov, B.S., & Dong, K. (2015). A mutation in the intracellular loop III/IV of mosquito sodium channel synergizes the effect of mutations in helix IIS6 on pyrethroid resistance. Molecular Pharmacology, 87, 421–429. doi:10.1124/mol.114.094730.
- Wang, X., Wang, R., Yang, Y., Wu, S., O'Reilly, A.O., & Wu, Y. (2016). A point mutation in the glutamate-gated chloride channel of Plutella xylostella is associated with resistance to abamectin. Insect Molecular Biology, 25, 116–125. doi:10.1111/imb.12204.
- Wang, X. & Wu, Y. (2012). High levels of resistance to chlorantraniliprole evolved in field populations of Plutella Xylostella. Journal of Economic Entomology, 105, 1019–1023. doi:10.1603/EC12059.
- Warmke, J.W., Reenan, R.A., Wang, P., Qian, S., Arena, J.P., Wang, J., …. Cohen, C.J. (1997). Functional expression of Drosophila para sodium channels. Modulation by the membrane protein TipE and toxin pharmacology. The Journal of General Physiology, 110, 119–133. doi:10.1085/jgp.110.2.119.
- Watson, G.B., Chouinard, S.W., Cook, K.R., Geng, C., Gifford, J.M., Gustafson, G.D., … Stillwell, G.E. (2010). A spinosyn-sensitive Drosophila melanogaster nicotinic acetylcholine receptor identified through chemically induced target site resistance, resistance gene identification, and heterologous expression. Insect Biochemistry and Molecular Biology, 40, 376–384. doi:10.1016/j.ibmb.2009.11.004.
- Williamson, M.S., Denholm, I., Bell, C.A., & Devonshire, A.L. (1993). Knockdown resistance (kdr) to DDT and pyrethroid insecticides maps to a sodium channel gene locus in the housefly (Musca domestica). Molecular & General Genetics: MGG, 240, 17–22. doi:10.1007/BF00276878.
- Williamson, M.S., Martinez-Torres, D., Hick, C.A., & Devonshire, A.L. (1996). Identification of mutations in the housefly para-type sodium channel gene associated with knockdown resistance (kdr) to pyrethroid insecticides. Molecular & General Genetics: MGG, 252, 51–60. doi:10.1007/BF02173204.
- Wolstenholme, A.J. (2012). Glutamate-gated chloride channels. Journal of Biological Chemistry, 287, 40232–40238. doi:10.1074/jbc.R112.406280.
- Xu, X.H., Bhat, M.B., Nishi, M., Takeshima, H., & Ma, J.J. (2000). Molecular cloning of cDNA encoding a Drosophila ryanodine receptor and functional studies of the carboxyl-terminal calcium release channel. Biophysical Journal, 78, 1270–1281. doi:10.1016/S0006-3495(00)76683-5.
- Zhang, H.G., ffrench-Constant, R.H., & Jackson, M.B. (1994). A unique amino acid of the Drosophila GABA receptor with influence on drug sensitivity by two mechanisms. The Journal of Physiology, 479, 65–75. doi:10.1113/jphysiol.1994.sp020278.
- Zhang, H.G., Lee, H.J., Rocheleau, T., ffrench-Constant, R.H., & Jackson, M.B. (1995). Subunit composition determines picrotoxin and bicuculline sensitivity of Drosophila gamma-aminobutyric acid receptors. Molecular Pharmacology, 48, 835–840.
- Zhang, Y., Wang, X., Yang, B., Hu, Y., Huang, L., Bass, C., & Liu, Z. (2015). Reduction in mRNA and protein expression of a nicotinic acetylcholine receptor α8 subunit is associated with resistance to imidacloprid in the brown planthopper, Nilaparvata lugens. Journal of Neurochemistry, 135, 686–694. doi:10.1111/jnc.13281.
- Zhao, X.L., Yeh, J.Z., Salgado, V.L., & Narahashi, T. (2004). Fipronil is a potent open channel blocker of glutamate-activated chloride channels in cockroach neurons. Journal of Pharmacology and Experimental Therapeutics, 310, 192–201. doi:10.1124/jpet.104.065516.
- Zimmer, C.T., Garrood, W.T., Puinean, A.M., Eckel-Zimmer, M., Williamson, M.S., Davies, T.G.E., & Bass, C. (2016). A CRISPR/Cas9 mediated point mutation in the alpha 6 subunit of the nicotinic acetylcholine receptor confers resistance to spinosad in Drosophila melanogaster. Insect Biochemistry and Molecular Biology, 73, 62–69. doi:10.1016/j.ibmb.2016.04.007.