Abstract
This study assessed the effect of surface conditioning methods and ceramic primers on the repair strength of resin-composite to zirconia-veneering ceramic with different defect sizes. Zirconia (IPS e.max ZirCAD) (n = 30) and veneering ceramic (Creation) (n = 30) alone acted as control groups. In the 1st part of the study, zirconia was silica-coated (SC) and treated with 3 different ceramic primers (Monobond Plus + Helibond (MBP), Clearfil Ceramic Primer Plus (CCP), Z-Prime Plus (ZPP)). Feldspathic ceramic was etched with 9.6% hydrofluoric acid (HF) and primed. After repairing with resin-composite (Empress Esthetic) and testing for shear bond strength (SBS), the best performing adhesive system (MBP) for both substrates (N = 60, n = 10 per group) was tested for defect sizes of (a) 3.2, (b) 4, (c) 5 mm with zirconia (diameter: 1.7 mm) being in the center (N = 60, n = 10 per group). Half of the specimens were repaired employing HFSC and the other half with SCHF. The specimens were stored in distilled water (37 °C, 24 h) and thermocycled (5–55 °C, 5000 cycles). The resin-substrate interface was loaded under shear force (1 mm/min). Data (MPa) were analyzed using two-way ANOVA and Tukey’s tests (α = 0.05). In the 1st study, MBP resulted in significantly higher SBS (MPa) for both zirconia and veneering ceramic (Zirconia: 14.2 ± 3.6; Veneer: 11.0 ± 3.7), compared to those of CCP (Zirconia: 9.4 ± 2.2; Veneer: 7.5 ± 3.4) and ZPP (Zirconia: 5.0 ± 0.9; Veneer: 5.9 ± 2.1) (p < 0.01). Neither the size of the defect (p > 0.05) nor repair sequence showed a significant effect (p > 0.05) on the repair bond strength with mainly mixed type with cohesive fracture in the veneering ceramic.
Introduction
During the last few decades, the demand for metal-free, long-span, stable and aesthetically sufficient materials to replace metal-ceramic fixed dental prosthesis (FDP) has increased. One such material fulfilling these requirements is zirconium dioxide (hereafter: zirconia). Not only the higher price for gold alloy but also the possibility to produce FDPs overnight, utilizing Computer Aided Design/Computer Aided Manufacturing (CADCAM) workflow has increased the production of FDPs made of zirconia as a framework that is later veneered with a glassy matrix ceramic or as monolithic restoration. Zirconia ceramic is highly biocompatible and due to its high flexural strength (up to 1200 MPa) and fracture toughness (up to 8 MPa*m1/2), this material is indicated for long-span FDPs [Citation1,Citation2]. In the early versions, zirconia presented very low translucency with unfavourable refractive index, and therefore insufficient optical characteristics. Unfortunately, although zirconia can be made more translucent by increasing sintering temperature and decreasing particle size, such attempts resulted in lower flexural strength [Citation3]. Consequently, in situations demanding high aesthetics, there is still a need for veneering the zirconia framework in anterior or posterior FDPs.
Currently, the veneering ceramic demonstrating the best optical properties is feldspar ceramic, which has high translucency but low flexural strength with approximately 120 MPa depending on the brand. However, fusing these two materials with different physical properties may lead to technical complications. A recent clinical study reporting on such veneered zirconia FDPs up to ten years using United States Public Health Services (USPHS) criteria [Citation4] showed a technical complication rate of extended chipping in 17.5% of the cases followed by small chipping in 10.5%, leading to a total chipping failure rate of 28% [Citation4]. Another review with an approximate mean observation period of 3 years also reported similar results with 24% chipping rate of zirconia FDPs [Citation5].
According to the USPHS criteria, chipping failures are graded in severity [Citation6]. While minor chipping failures (score Bravo) are recommended to be polished, chippings into the framework (score Delta), needs to be replaced which is a costly option for the patients. On the other hand, failures rated with score Charlie where cohesive failure is observed solely in the feldspathic with and without zirconia framework exposure could be repaired [Citation7].
A number of surface conditioning methods have been suggested for intraoral repair actions [Citation8]. Feldspar as a silicate-based ceramic is acid sensitive and can be therefore conditioned using 5–9.6% hydrofluoric acid gel that selectively dissolves the glassy phase and creates micro-porosities favouring micromechanical retention. Additional silane coupling agent application based on 3-methacryloxypropyl trimethoxysilane coupling agent (γ-MPS), enhances the chemical adhesion between the glass ceramic and the repair resin composite [Citation9–13]. These steps improve the resin hydrophilicity on the ceramic surface increasing the physico-chemical interactions between the resin and the ceramic [Citation9,Citation13].
In contrast to feldspar, due to the lack of silica phase on its composition, zirconia is inert for etching. For this reason, such ceramics need to be roughened to achieve micromechanical retention of the resin [Citation14,Citation15]. The optimal surface conditioning method for zirconia ceramic has been reported to be air-borne particle abrasion, with or without silica-coated particles followed by silanization or primer application [Citation14–16].
When repairing mixed surfaces as in the case of veneering ceramic and metal or zirconia, since both surfaces require a separate surface conditioning method, carry-over effect cannot be avoided in repair scenarios in clinical practice. In one study, comparing conditioning methods for mixed surfaces showed that first etching and then air abrading with silica-coated alumina particles results in the higher shear bond strength to veneer ceramic and zirconia [Citation17]. This study, however, assessed only one defect size ratio, namely 3 mm of zirconia with 2 mm feldspar, and thus cannot extrapolate to chippings with other ratios of exposed zirconia ceramic. Furthermore, bifunctional coupling agents or ceramic primers today not only contain MPS but also 10-methacryloyloxydecyl dihydrogenphosphate (10-MDP) monomers which could be beneficial especially when glass-ceramic/zirconia assemblies are to be repaired creating siloxane bonds onto both substrates [Citation14,Citation15].
The objectives of this study therefore were to evaluate the adhesion potential of resin composite to glass ceramic and zirconia after using bifunctional coupling agents/primers and to investigate the best performing primer on the repair of small to larger ratios of veneering ceramic-zirconia substrates in two sequences and evaluate the failure types. The null hypotheses tested were that neither the repair sequence nor the size of exposed zirconia surface would affect the repair bond strength.
Materials and methods
The brands, chemical compositions and manufacturers and of the materials used in this study are listed in . Distribution of experimental groups based on the substrate types, primers tested and the sequence of experimental procedures are presented in .
Figure 1. Experimental sequence and allocation of groups depending on the silanization method and surface conditioning sequence employed for repair.
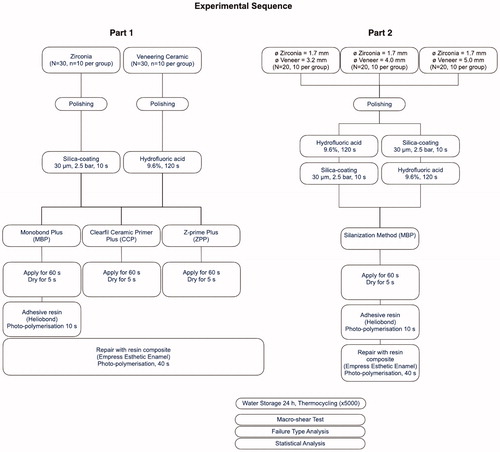
Figure 2. Representative digital microscopy photos of the failure types after debonding. (a) Score 0 = Adhesive failure with no resin composite remnants left on zirconia (Z), veneering ceramic (V) or Z + V; (b) Score 1 = Mixed 1: Cohesive fracture in the veneering ceramic and ≥1/2 resin composite left adhered on the Z, V or Z + V; (c) Score 2= Mixed 2, ≥ 1/3 resin composite left adhered on Z, V or Z + V.
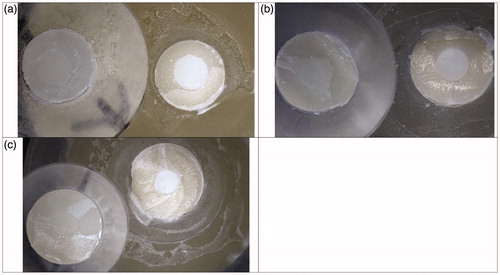
Table 1. The brands, chemical compositions, manufacturers, and batch numbers of the main materials used in this study.
Specimen preparation-Part 1
Zirconia (diameter: 5 mm; thickness: 2 mm) (N = 30; n = 10 per subgroup) (IPS e.max ZirCAD, Ivoclar Vivadent, Schaan, Liechtenstein) and feldspathic ceramic specimens (diameter: 5 mm; thickness: 2 mm) (N = 30; n = 10 per subgroup) (Creation Willi Geller International GmbH, Meiningen, Austria) were fabricated according each manufacturer’s instructions. They were initially embedded in plastic moulds (Scandiquick, Scandia, Hagen, Germany) with auto-polymerized polymethylmethacrylate using an acrylic resin (Duralay, Reliance, Illinois, USA) with their bonding surfaces exposed [Citation18].
The specimens were wet ground finished using silicon carbide papers in (#1000-grit) at low speed for 30 s each (English Abrasives Ltd., London, United Kingdom) followed by 10 µm diamond paste and then cleaned ultrasonically (Vitasonic, VITA Zahnfabrik, Bad Säckingen, Germany) in distilled water for 10 min.
Surface conditioning methods
Silica-coating (SC): The zirconia specimens were air-abraded with 30 µm aluminum oxide particles coated with silica (CoJet Sand, 3 M ESPE, St. Paul, MN, USA) at 2.5 bar pressure from a distance of approximately 10 mm from the surface, in a circular motion for 15 s using an intraoral air-abrasion device (Dento-Prep, RØNVIG, Daugaard, Denmark).
Hydrofluoric acid etching (HF): The surfaces of feldspathic veneering ceramic were etched with 9.6% hydrofluoric acid for 120 s (Ultradent, South Jordan, UT, USA) and rinsed for 60 s with distilled water.
All specimens were ultrasonically cleaned (Vitasonic) in distilled water for 10 min and randomly assigned to one of the three ceramic primers: Monobond Plus (Ivoclar Vivadent, Schaan, Liechtenstein-MBP), Clearfil Ceramic Primer Plus (CCP) and Z-prime Plus (ZPP). Application modes according to each manufacturer are listed in . One coat of ceramic primer was applied with disposable micro brushes, waited for its reaction for 60 s and air blown with oil free air. Only in group MBP, one coat of adhesive resin (Heliobond, Ivoclar Vivadent) was applied, air-thinned and photo-polymerized for 10 s prior to direct resin composite application according to the manufacturer’s instructions.
One calibrated operator (R.S.) carried out adhesive procedures throughout the experiments. Translucent polyethylene moulds (height: 4 mm, inner diameter: 2.9 mm) (DuPont, Saint-Gobain, France) were stabilized on the ceramic specimens in a custom-made device. The mould was filled with the direct resin composite (Empress Esthetic Enamel, Ivoclar Vivadent) with a hand instrument in one increment up to 3 mm and photo-polymerized (Bluephase, Ivoclar Vivadent) for 40 s from 5 directions from a distance of 2 mm [Citation18]. Light intensity was assumed to be higher than 1200 mW/cm2, verified by a radiometer after every 10 specimens (Model 100, Kerr, Orange, CA, USA). Oxygen inhibiting gel (Oxyguard, Kuraray) was applied at the bonded margins and rinsed with copious water after 1 min.
The specimens were kept dry at 37 °C for 24 h in dark incubator and then subjected to thermocycling for 5000 cycles between 5 and 55 °C in distilled water (Haake DC 10, Thermo Haake, Karlsruhe, Germany). The dwelling time at each temperature was 30 s and the transfer time from one bath to the other was 10 s.
Specimen preparation-Part 2
In this part of the experiments, zirconia specimens were fabricated (diameter: 1.7 mm; thickness: 2 mm) (N = 60) and veneered with feldspar ceramic according to the manufacturer’s instructions as in Part 1, using a split metal mould resulting in three thicknesses yielding to a diameter of 3.4, 4.2 and 5.2 mm, respectively (N = 60, 20 per subgroup). In this manner, zirconia (diameter: 1.7 mm) remained in the center of the veneered specimen, simulating the chipping scenario with exposed zirconia. This allowed a bonding area of core/veneer ratio of 1.7/3.2, 1.7/4 and 1.7/5 mm of defect sizes, respectively.
The zirconia-veneer ceramic assemblies were embedded in plastic moulds with auto-polymerized polymethylmethacrylate using an acrylic resin with the bonding surfaces exposed, ground finished using silicon carbide papers and cleaned ultrasonically as described in Part 1.
Surface conditioning methods
The specimens in each defect size ratio (zirconia/veneer) were randomly assigned to two conditioning sequences:
Half of the specimens were repaired first by conditioning the zirconia and then the veneering ceramic (Sequence 1-SCHF), and the other half in the reverse order (Sequence 2-HFSC) simulating the typical clinic repair situation.
Custom-made translucent polyethylene moulds (height: 4 mm, diameters: 3.2, 4 and 5 mm) (Heinz Baumgartner Werkzeugbau and Feinmechanik AG, Urdorf, Switzerland) were stabilized on the zirconia-veneer ceramic assemblies in a custom-made device. Repair procedures were carried out as described in Part 1with the same materials. After repair procedures, repair surface areas of 8, 12.6, 19.6 mm2 were achieved for the three defect sizes, respectively.
Based on the results of the experiments in Part 1, MBP method resulted in significantly higher results than those of other ceramic primers. Therefore, only this method was used after the surface conditioning procedures in Part 2.
The specimens were kept dry at 37 °C for 24 h in dark and then subjected to thermocycling for 5000 cycles between 5 and 55 °C in distilled water (Haake DC 10). The dwelling time at each temperature was 30 s and the transfer time from one bath to the other was 10 s as described in Part 1.
Macro-shear bond test
For the macro-shear bond test (MSB), specimens were mounted in the jig of the Universal Testing Machine (Zwick ROELL Z2.5 MA 18-1-3/7, Ulm, Germany) and shear force was applied using a shearing blade to the adhesive interface until failure occurred. The load was applied to the adhesive interface as close as possible to the surface of the substrate at a crosshead speed of 1 mm/min and the stress-strain curve was analyzed with the software program (TestXpert, Zwick ROELL, Ulm, Germany). The maximum load (N) was converted into megapascal (MPa) by dividing it by the bonding surface area of the resin cements.
Microscopic examination and failure analysis
After adhesion tests, debonded specimen surfaces were examined in order to analyze the failure types using an optical microscope (Stemi 2000-C, Carl Zeiss, Gottingen, Germany) at ×40 magnification. Failure types were classified for zirconia (Z), veneering ceramic (V) and the both (Z + V) as follows: (a) Score 0 = Adhesive failure with no resin composite remnants left on Z, V or Z + V; (b) Score 1 = Mixed 1: Cohesive fracture in the veneering ceramic and ≥1/2 resin composite left adhered on the Z, V or Z + V; (c) Score 2 = Mixed 2, ≥1/3 resin composite left adhered on Z, V or Z + V.
Statistical analysis
According to the two-group Satterthwaite t-test (SPSS Software V.20, Chicago, IL, USA) with a 0.05 two-sided significance level, a sample size of 10 in each experimental group was calculated to provide more than 80% power to detect a difference of 2 MPa between mean values. This assumes that the mean ± standard deviation ranges between 1 and 4 using a two-group Satterthwaite t-test with a 0.05 two-sided significance level. Data were analyzed using statistical softwares (Statistica 8.0, StatSoft, Inc., Tulsa, OK, USA and SPSS 11.0, SPSS Inc., Chicago, IL, USA). Kolmogorov-Smirnov and Shapiro-Wilk tests were used to test normal distribution of the data. For the data of Part 1, the means of each group were analyzed using a two-way analysis of variance (2-way ANOVA) and Tukey’s post hoc tests with the shear bond strength (MPa) as the dependent variable and substrate types (2 levels: Zirconia, Veneering ceramic), ceramic primer type (3 levels: MBP, CCP, ZPP) as the independent factors. For the data of Part 2, the means of each group were again analyzed using a separate 2-way ANOVA and Tukey’s post hoc tests with the shear bond strength (MPa) as the dependent variable and defect size of zirconia/veneer (3 levels: 1.7/3.2, 1.7/4 and 1.7/5 mm), conditioning sequence (2 levels: SCHF, HFSC) as the independent factors. The p values less than 0.05 were considered to be statistically significant in all tests.
Results
No spontaneous debonding occurred during water storage and thermocycling in all experiments.
Part 1
While ceramic primer systems (p < 0.01) showed a significant effect, substrate type (p > 0.05) did not significantly affect the bond results. Interaction terms were not significant (p > 0.05).
MBP resulted in significantly higher bond strength (MPa) for both zirconia and veneering ceramic (Zirconia: 14.2 ± 3.6; Veneer: 11.0 ± 3.7), compared to those of CCP (Zirconia: 9.4 ± 2.2; Veneer: 7.5 ± 3.4) and ZZP (Zirconia: 5.0 ± 0.9; Veneer: 5.9 ± 2.1) (p < 0.01) (). CCP and ZZP did not show a significant difference for both of the substrates tested (p > 0.05).
Table 2. The mean bond strength values (Mean ± standard deviations) (MPa) of macro-shear repair bond strength, minimum, maximum values at 95% confidence interval (CI).
All specimens in this part presented Score 1 (100%) for veneering ceramic and Score 0 (100%) for zirconia. Ceramic for MBS.
Part 2
Neither the size of the defect for repair (p > 0.05) nor the repair sequence showed a significant effect (p > 0.05) on the bond strength results. Interaction terms were also not significant (p > 0.05).
In the case of SCHF surface conditioning sequence, resulted in mean bond strength of 20.1 ± 3.6, 18.2 ± 4.8, 15.8 ± 4.2 in descending order for repair defects of 1.7/5, 1.7/4 1.7/3.2, respectively (p > 0.05) (). On the other hand, HFSC surface conditioning sequence resulted in the highest bond strength with size: 20.6 ± 8.3 followed by 18.6 ± 3.1 and16.4 ± 3.7 in descending order for repair defects of 1.7/3.2, 1.7/5, 1.7/4, respectively (p > 0.05).
Table 3. The mean bond strength values (Mean ± standard deviations) (MPa) of macro-shear repair bond strength, minimum, maximum values at 95% confidence interval (CI).
Failure types were the predominantly mixed type where cohesive fracture in the veneering ceramic was observed that was accompanied with resin composite left adhered on either on zirconia or both zirconia and veneering ceramic ().
Table 4. Frequency of failure types as a function of repaired defect size and sequence of surface conditioning method.
Discussion
This study was undertaken firstly to evaluate the adhesion of resin composite to feldspathic glass ceramic and zirconia after using bifunctional ceramic primers for repair purposes and then to investigate the best performing ceramic primer on repair of small to larger ratios of zirconia/veneering ceramic substrate combinations in two surface conditioning sequences. Based on the results of this study, since neither the repair sequence nor the size of exposed zirconia surface showed a significant effect on the repair bond strength, the null hypotheses could be rejected.
In order to measure the bond strength values between an adhesives and restorative material substrates, a number of adhesion test methods have been suggested such as macro-, micro-shear, macro- or micro-tensile tests. In any adhesion test method, however, it is crucial that the bonding interface should be the most stressed region [Citation19,Citation20]. Conventional tensile tests also present some limitations, such as the difficulty of specimen alignment and the tendency for heterogeneous stress distribution at the adhesive interface. On the other hand, when specimens are aligned correctly, the micro-tensile test shows more homogeneous distribution of stress, and thereby more sensitive comparison or evaluation of bond performances [Citation21]. However, small deviations in specimen alignment in the jig may increase the bond strength due to shear component introduced during deboning bonded joints [Citation21]. Shear tests have also limitations for the development of non-homogeneous stress distributions at the bonded interface, inducing either underestimation or misinterpretation of the results, as the failure often starts in one of the substrates and not solely at the adhesive zone [Citation21]. Overall, adhesion-related studies in dentistry, bonded surface areas range from 3 mm2 to 1 mm2 in macro- and micro-test methods, respectively [Citation21]. Due to the reduced bonded area and more homogeneous distribution of stresses, micro-test methods are suggested in adhesion tests [Citation19,Citation20].
In this study, due to the nature of the clinical problems related to the chipping of ceramic reconstructions, two substrates were considered as substrates to be repaired. Typically, chipping of veneered zirconia FDPs could either occur cohesively only within the feldspathic veneering ceramic, or with some degree of zirconia framework exposure. In the case of monolithic zirconia FDPs where no veneering is present, chipping could take place cohesively within the zirconia only. For this reason, the ceramic primers and surface conditioning methods were tested initially on individual substrates followed by the combination. Studies on adhesion tests to two substrates simultaneously are limited in the literature [Citation17,Citation22,Citation23] although, in fact, this type of test has more translational meaning for the clinical repair scenarios.
In both the first and second part of the study, the surface conditioning method employed on feldspathic ceramic was HF etching which is still the most widely accepted method in order to selectively dissolve the glassy matrix and thereby achieve micromechanical retention of the subsequent resin material, be it luting resin or repair resin. Likewise, the consensus based on systematic reviews and meta-analysis suggest the use of airborne particle abrasion in order to roughen the zirconia surface [Citation14,Citation15]. During air-abrasion, since the energy available for crack propagation is in part dissipated in the damaged area shaped by the monoclinic zirconia, transformation toughening occurs in zirconia [Citation24]. This mechanism could be influenced by several factors such as shape, size and location of grains, presence of defects and/or oxygen vacancies in the structure, type and amount of stabilizing oxides, manufacturing methods, applied stresses and temperature variation [Citation24]. Unfortunately to date, air-abrasion protocols could not be substituted with the use of ceramic primers only [Citation15,Citation18].
In principle, MPS silanes with general chemical formula X-(CH2) Si-(OR), where X is the monomeric end and R is the alkyl group(s) of the coupling agent MPS, are able to provide chemical bonding with organic surfaces such as resin materials and silica-based ceramics [Citation11]. MPS silanes present molecules that react with water, forming silanol groups (–Si–OH) from the methacryloxy groups (–Si–O–CH3). Silanol groups react to form a siloxane network (–Si–O–Si–O–) with the silica oxide of feldspathic ceramics [Citation11,Citation16]. The monomeric ends of silane then react with methacrylate groups of the resin material. Thus, the bonding process between ceramic surface and resin material occurs through the chemical process between silica oxides and silane bonding agent. In the case of zirconia, however, additional chemical adhesion could best be achieved using ceramic primers based on phosphate ester monomer, 10-MDP, 4-methacryloxyethyl trimellitic anhydride (4-META), thiophosphoric acid methacrylate (MEPS) that can chemically react with oxides on zirconia [Citation14,Citation15]. However, all these ceramic primers still do require air-abrasion in order to achieve a clean surface prior to their application according to the manufacturers.
The presence of MPS in the MDP containing primers are expected to co-polymerize and create siloxane bonds on both feldspathic and zirconia ceramics. In this study, the ceramic primer MBP with a mixture of alcohol solution of silane methacrylate, phosphoric acid methacrylate and sulphide methacrylate in its chemical composition resulted in significantly higher bond strength on both substrates separately in the first part of the study compared to those of CCP and ZPP. Besides the presence of alcohol, CCP contains both MPS and MDP whereas ZPP contains only MDP in addition to bis-GMA monomer. However, material-resin bond is vulnerable to degradation especially in accelerated aging conditions, resulting in decrease in the results obtained in dry conditions [Citation18].
The shear bond strength results (MPa) obtained for zirconia with MBP with 14.2 ± 3.6 for zirconia and 11.0 ± 3.6 for veneer were similar to a previous study where macro-shear test was used on adhesion to zirconia (11.9–13.8 MPa) [Citation18] and slightly lower than another study (17.4) where micro-tensile test was employed on veneering ceramic [Citation25]. In some studies, adhesion to feldspathic ceramic was reported to be higher with MPS silane coupling agent [Citation16,Citation25] compared to this study but due to hydrolysis such results decrease [Citation26,Citation27]. When water molecules penetrate into the bonded interfaces, physical bonds are destroyed yielding to detachment of the resin-based materials [Citation26,Citation27]. In this study, the bond strength data were obtained after ×5000 hydrothermal aging in order to test the worse-case scenario. The bond strength results are almost half the less after aging compared to non-aged conditions according to a previous study [Citation18].
The other aspect between the differences in the bond strength of ceramic primers could be attributed to the solvent evaporation. In this regard, solvent evaporation represents a factor affecting the coupling potential of silanes [Citation28]. Even though a small amount of solvent may help silane wetting, incomplete evaporation may impair adhesion. Water, alcohol, acetone, acetic acid and other by-products that remain on the surface, if not completely expelled by drying procedure, may remain as hydrogen-bonded to the OH-rich sites. This may decrease the number of bond sites available for reacting with silane, thus compromising the final degree of siloxane bond formation [Citation28]. Accordingly, heat treatment process was suggested to evaporate the solvent and volatile by-products from the silane reaction, catalyzing and completing the condensation reactions both with the substrate and within the silane coating [Citation28]. In this study, no heat treatment was applied to the silane layer in any of the groups and reaction duration was limited to 60 s followed by 5 s of drying. Prolonged reaction time may increase the condensation reaction which needs to be further investigated.
The originality of this study was to focus on the effect of the size of the area to be repaired and while doing this incorporating the two substrates at the same time in the bonded area. The results indicated that increasing the presence of etchable feldspathic ceramic around zirconia did not result in increase in bond strength. This could be due to the fact that MBP ceramic primer was equally efficient on both substrates which was also the finding of the first part of this study. The other reason could be due to the better sealing of the margins with the etched feldspathic ceramic with the repair resin that probably compensated loss of adhesion from zirconia surface. Nevertheless, the chosen ceramic primer from the first part of the study effectively functioned on both substrates regardless of the size of the repaired area. These findings are also supported by the type of failures in that the incidence of adhesive failures were more frequent on zirconia surface. On the other hand, the incidence of mixed type of failures with cohesive fracture in the veneering ceramic along with ≥1/2 resin composite left adhered on the veneering ceramic (Score 1) was more common in the veneering ceramic part. Cohesive failures in the substrate is an indication that adhesion achieved exceeded the cohesive strength of the substrate material while cohesive failure in the repair resin indicates that the bond strength at the repaired interface exceeded that of the cohesive strength of the repair composite which could be interpreted as reliable adhesion and durable bond strength.
The interest of the study was to study the possible carry-over effect of individual surface conditioning methods on both substrates and possible consequences on the adhesion results. In previous studies, such an effect was observed when either silica-coating or hydrofluoric etching was performed in changing orders for alumina-veneer, amalgam-tooth or tooth-composite repairs [Citation17,Citation22,Citation23]. In this study, the change in surface conditioning sequence (SCHF vs HFSC), independent of the repaired defect size did not affect the bond strength data. It has to be noted that in the previous studies, the duration of air-abrasion was limited to 5 s where in this study it was 15 s. Prolonged duration of up to 20 s of air-abrasion was suggested for the zirconia surface in order to achieve increased silica deposition on the surface [Citation29]. This long duration, however, could damage the neighbouring substrate, increase the monoclinical phase in zirconia [Citation30,Citation31] and therefore was not chosen in this study. Nonetheless, from a clinical perspective completing air-abrasion and washing and rinsing the surface would eliminate sand particles from the surface and would allow for a better working environment to continue with HF etching of the veneering ceramic and could further be recommended. Most likely, HF application also dissolved the loose silica particles and thereby the repair sequence did not carry-over an effect on the two substrates.
Since chipping is a phenomenon related to anatomical support of the framework, thermal expansion coefficient or simply degradation of veneering ceramics, intraoral repair options could eliminate costly replacement procedures. Clinical studies should report on the longevity of such repair applications with the proposed protocols.
Conclusions
From this study, the following could be concluded:
Adhesion of repair resin confined to only zirconia or feldspathic ceramic was significantly higher with Monobond Plus, compared to those of other ceramic primers tested where zirconia was initially silica-coated and veneering feldspathic ceramic was etched with hydrofluoric acid.
Monobond Plus with a mixture of alcohol solution of silane methacrylate, phosphoric acid methacrylate and sulphide methacrylate in its chemical composition resulted in significantly higher repair bond strength on both zirconia and veneering ceramic compared to Clearfil Ceramic Primer Plus that contains both MPS and MDP and Z-Prime Plus that contains only MDP in addition to bis-GMA monomer.
The increase in the size of the veneering ceramic circular around the exposed zirconia from 3.2 to 5 mm did not increase the repair bond strength results.
The sequence of surface conditioning starting with zirconia with silica-coating and then application of hydrofluoric acid etching of veneering ceramic or reverse did not affect repair bond strength.
Cohesive failure in zirconia was not observed in any of the specimens regardless of the conditioning method and the ceramic primer used.
Clinical relevance
When repairing veneered zirconia reconstructions, after etching veneering feldspathic ceramic with hydrofluoric acid and silica-coating zirconia, both surfaces should receive ceramic primer containing silane methacrylate, phosphoric acid methacrylate and sulphide methacrylate (Monobond Plus) and coated with adhesive resin for durable intraoral repair.
Acknowledgments
The authors acknowledge Mr. A. Trottmann, University of Zürich, Center for Dental and Oral Medicine, Zürich, Switzerland, for his assistance with the specimen preparations, Ivoclar Vivadent, Schaan, Liechtenstein and Bisco Inc, Schaumburg, USA for the generous provision of the materials. We extend our gratitude to Miss. R. van Sluijs for her assistance with the statistical analysis.
Disclosure statement
The authors did not have any commercial interest in any of the materials used in this study.
References
- Guazzato M, Albakry M, Ringer SP, et al. Strength, fracture toughness and microstructure of a selection of all-ceramic materials. Part II. Zirconia-based dental ceramics. Dent Mater. 2004;20(5):449–456.
- Marinis A, Aquilino SA, Lund PS, et al. Fracture toughness of yttria-stabilized zirconia sintered in conventional and microwave ovens. J Prosthet Dent. 2013;109(3):165–171.
- Zhang Y, Lawn BR. Evaluating dental zirconia. Dent Mater. 2019;35(1):15–23.
- Ioannidis A, Bindl A. Clinical prospective evaluation of zirconia-based three-unit posterior fixed dental prostheses: up-to ten-year results. J Dent. 2016;47:80–85.
- Heintze SD, Rousson V. Survival of zirconia- and metal-supported fixed dental prostheses: a systematic review. Int J Prosthodont. 2010;23(6):493–502.
- Ryge G, Snyder M. Evaluating the clinical quality of restorations. J Am Dent Assoc. 1973;87(2):369–377.
- Agingu C, Zhang CY, Jiang NW, et al. Intraoral repair of chipped or fractured veneered zirconia crowns and fixed dental prosthesis: clinical guidelines based on literature review. J Adhes Sci Technol. 2018;32(15):1711–1723.
- Loomans B, Özcan M. Intraoral repair of direct and indirect restorations: procedures and guidelines. Oper Dent. 2016;41(S7):S68–S78.
- Lu R, Harcourt JK, Tyas MJ, et al. An investigation of the composite resin/porcelain interface. Aust Dent J. 1992;37(1):12–19.
- Spohr AM, Sobrinho LC, Sinhoreti MA, et al. Influence of surface conditions and silane agent on the bond of resin to IPS Empress 2 ceramic. Int J Prosthodont. 2003;16:277–282.
- Matinlinna JP, Lassila LV, Vallittu PK. The effect of three silane coupling agents and their blends with a cross-linker silane on bonding a bis-GMA resin to silicatized titanium (a novel silane system). J Dent. 2006;34(10):740–746.
- Özcan M, Barbosa SH, Melo RM, et al. Effect of surface conditioning methods on the microtensile bond strength of resin composite to composite after aging conditions. Dent Mater. 2007;23(10):1276–1282.
- Papia E, Larsson C, Du Toit M, et al. Bonding between oxide ceramics and adhesive cement systems: a systematic review. J Biomed Mater Res B Appl Biomater. 2014;102(2):395–413.
- Inokoshi M, De Munck J, Minakuchi S, et al. Meta-analysis of bonding effectiveness to zirconia ceramics. J Dent Res. 2014;93(4):329–334.
- Özcan M, Bernasconi M. Adhesion to zirconia used for dental restorations: a systematic review and meta-analysis. J Adhes Dent. 2015;17(1):7–26.
- Hooshmand T, Matinlinna JP, Keshvad A, et al. Bond strength of a dental leucite-based glass ceramic to a resin cement using different silanecoupling agents. J Mech Behav Biomed Mater. 2013;17:327–332.
- Özcan M, Valandro LF, Pereira SM, et al. Effect of surface conditioning modalities on the repair bond strength of resin composite to the zirconia core/veneering ceramic complex. J Adhes Dent. 2013;15:207–210.
- Rebholz-Zaribaf N, Özcan M. Adhesion to zirconia as a function of primers/silane coupling agents, luting cement types, aging and test methods. J Adhes Sci Technol. 2017;13:1408–1421.
- Della Bona A, Van Noort R. Shear vs. tensile bond strength of resin composite bonded to ceramic. J Dent Res. 1995;74(9):1591–1596.
- Betamar N, Cardew G, Van Noort R. Influence of specimen designs on the microtensile bond strength to dentin. J Adhes Dent. 2007;9(2):159–168.
- Bracher L, Özcan M. Adhesion of resin composite to enamel and dentin: a methodological assessment. J Adhes Sci Technol. 2018;32(3):258–271.
- Özcan M, Schoonbeek G, Gökçe B, et al. Bond strength comparison of amalgam repair protocols using resin composite in situations with and without dentin exposure. Oper Dent. 2010;35(6):655–662.
- Saracoglu A, Özcan M, Kumbuloglu O, et al. Adhesion of resin composite to hydrofluoric acid-exposed enamel and dentin in repair protocols. Oper Dent. 2011;36(5):545–553.
- Chevalier J, Deville S, Münch E, et al. Critical effect of cubic phase on aging in 3mol% yttria-stabilized zirconia ceramics for hip replacement prosthesis. Biomaterials. 2004;25(24):5539–5545.
- Brentel AS, Özcan M, Valandro LF, et al. Microtensile bond strength of a resin cement to feldpathic ceramic after different etching and silanization regimens in dry and aged conditions. Dent Mater. 2007;23(11):1323–1331.
- Ishida H, Koenig JL. Effect of hydrolysis and drying on the siloxane bonds of a silane coupling agent deposited on E-glass fibers. J Polym Sci B: Polym Phys. 1980;18:233–237.
- Larson TD. The uses of silane and surface treatment in bonding. Northwest Dent. 2006;85(3):27–30.
- Queiroz JR, Benetti P, Özcan M, et al. Surface characterization of feldspathic ceramic using ATR FT-IR and ellipsometry after various silanization protocols. Dent Mater. 2012;28(2):189–196.
- Özcan M, Raadschelders J, Vallittu P, et al. Effect of particle deposition parameters on silica coating of zirconia using a chairside air-abrasion device. J Adhes Dent. 2013;15:211–214.
- Souza RO, Valandro LF, Melo RM, et al. Air-particle abrasion on zirconia ceramic using different protocols: effects on biaxial flexural strength after cyclic loading, phase transformation and surface topography. J Mech Behav Biomed Mater. 2013;26:155–163.
- Abi-Rached FO, Martins SB, Campos JA, et al. Evaluation of roughness, wettability, and morphology of an yttria-stabilized tetragonal zirconia polycrystal ceramic after different airborne-particle abrasion protocols. J Prosthet Dent. 2014;112(6):1385–1391.