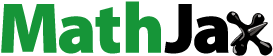
Abstract
Purpose. Behind armor blunt trauma (BABT) is a non-penetrating injury caused by the rapid deformation of body armor, by a projectile, which may in extreme circumstances cause death. The understanding of the mechanisms is still low, in relation to what is needed for safety threshold levels. High velocity projectile BABT causes immediate and severe hypoxia by increased venous admixture (Q’s/Q’t), but it is not known whether the level of hypoxia correlates to the kinetic energy (Ek) of the projectile.
Materials and Methods. We constructed a 65 mm BABT-simulator to measure the Ek absorbed by the thorax. The simulator was validated to 7.62 mm high velocity BABT (swine with removed organs) for 7.62 mm (n = 7) and 65 mm (n = 12). Physiological measurements during 60 minutes were performed in 40 anesthetized swine in groups control (n = 9), 7.62 mm (n = 7), 65 mm weight variation (n = 24), 65 mm speed variation (n = 12, included in the weight variation group). New calculations were done for a previously studied group of 7.62 mm with backing (n = 9).
Results. 65 mm BABT simulation and 7.62 mm BABT had similar back-face signatures (24 mm), and maximum thoracic impression speed (24-34 m/s). Back-face signatures correlated linearly to Ek (R2=0.20). Rib fractures had a 50% likelihood at back-face signature 23.0 mm (95% CI 18.5 to 29.0 mm, area under ROC curve 0.93). Ek correlated linearly to pO2 (R2=0.34, p = 0.0026) and venous admixture (R2=0.37, p = 0.0046). The extrapolated Ek at 5 minutes for pO2=0 kPa was 587 J and for venous admixture = 100% 574 J.
Conclusions. Hypoxia and venous admixture correlated linearly to Ek, allowing for a calculated predicted lethal Ek to ≥574 J, which should be verified in survival studies. Lethality predictions from lung physiology is an alternative to clay impressions and may facilitate the development of ballistic safety equipment and new BABT safety criteria.
Supplemental data for this article is available online at https://doi.org/10.1080/01902148.2021.1950869 .
Introduction
Behind armor blunt trauma (BABT) is a non-penetrating injury caused by the rapid deformation of body armor by a projectile hit.Citation1 When the body armor inhibits the projectile from penetrating the thorax, shock waves propagate and kinetic energy (Ek) from the ballistic impact is transferred to the chest wall and to the internal organs, causing injury through rapid acceleration and deceleration of the tissue.Citation2 BABT may lead to cardiopulmonary failure and in extreme circumstances death.Citation1,Citation3,Citation4 Personal armors are specified to stop penetration from given types of ammunition. Since BABT injuries do exist, criteria for allowable back face movement should also be used. Especially for high energy rifle ammunition general standards are still lacking. There is also a concern that BABT could be a more important problem when newer protection materials with a lower mass (areal density) provide the same efficiency against penetration. Increased research efforts of injury mechanisms are therefore needed to meet the increased risk of severe pulmonary injury.Citation1,Citation3,Citation5 BABT is well described in gelatin models but the correlation with human physiology is low.Citation3,Citation6–8 We have shown that high velocity projectile BABT in swine results in an immediate and severe hypoxia,Citation9 which is attributed to a severe and transient decrease in ventilation perfusion mismatch (V’A/Q’) to <1 and increased venous admixture (Q’s/Q’t) in the exposed lung.Citation10 BABT injury mechanisms are complex and differ from other types of thoracic trauma, e.g. traffic accidents, and may include characteristics of primary blast injury.Citation1 It is not fully known how the lung injury correlates to the Ek of the projectile and the energy absorbed by the thorax. Measuring the absorbed Ek is methodologically challenging. Trauma by large objects impacting the thorax may result in elastic deformation which divert energy. Stopping a high energy bullet requires a hard armor plate. When the bullet hits the plate both the bullet and plate material is disintegrated, and part of the incoming energy transformed to heat. The total effect of the armor panel is therefore a reduction of kinetic energy, a high reduction of speed and a spread of force over a larger area. To be able to control and vary input force characteristics we constructed a BABT-simulator. The BABT-simulator was validated to inflict similar back-face signature and impression velocity, when fired directly at the naked chest as a high velocity projectile assault rifle 7.62 mm bullet impacting a ceramic plate armor placed on the thorax at 800 m/s.Citation8–10 Using the BABT-simulator, we investigated the relationship between impact parameters (Ek) and immediate and potentially life-threatening pulmonary consequences. Quantitative estimates of the effect of oxygenation and venous admixture (Q’s/Q’t) on gas exchange was measured in relation to the Ek absorbed by the thorax. We hypothesized that increased Ek would decrease pO2 and increase venous admixture (Q’s/Q’t) linearly.
Material and methods
The study was approved by, and conducted in accordance with, the Swedish regional ethics approval board for animal research (approval no A89-04, A 17-08, A 80-07, S-22-13). The animals were housed in an accredited animal facility for up to 5 days prior to the experiment and fed a standard diet with free access to tap water. The ambient room temperature was maintained at 21-22 °C with 12-hour light/12-hour darkness cycles. A total of 40 Swedish landrace swine (females or castrated males) with mean weight 69 (range 48-79) kg were divided into control (n = 9) and 7.62 mm (n = 7), 65 mm weight variation (n = 12 + 7 + 5 = 24). The 65 mm/58-gram weight variation group of twelve animals was divided into different speeds and also presented as the 65 mm speed variation (). At completion of the experiments, the animals were euthanized with 70 mL pentobarbital (100 mg/mL). In addition, we performed new calculations of lung function including venous admixture in relation to the energy transfer, in a group of identically anesthetized and instrumented swine (n = 9) which were previously included in a trauma attenuating backing report: 7.62 mm with backing.Citation11
Figure 1. List of experimental groups. The 65 mm/58-gram weight variation group (n = 12) was also divided into different speeds and in subsequently presented as 65 mm speed variation. 7.62 B means BABT with protective backing.
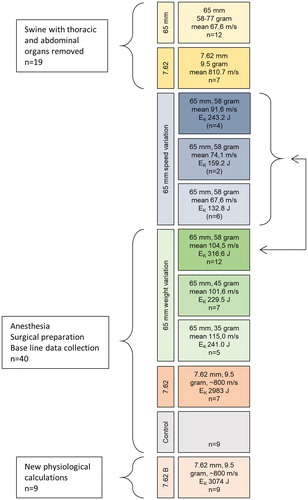
Preparation
The animals were sedated with 150 mg tiletamine/zolazepam (Zoletil 100 Vet) and 6 mg medetomidine (Domitor) before transport to the laboratory facility. Anesthesia was induced with pentobarbital 6 mg/kg, atropine 0.02 mg/kg and fentanyl 2.5 µg. Anesthesia was maintained with continuous infusion of ketamine 25 mg/kg/h (50 mg/mL Ketalar, Parke-Davis). Atropin 0.5 mg was administered to prevent mucus secretion. Animals were tracheotomized and mechanically ventilated during preparation in a volume-controlled mode with 21% O2 (Siemens Servo Ventilator 900 C, Siemens-Elema or Hamilton-C2, Hamilton medical). Respiratory rate (RR), tidal volume (VT), minute volume (MV) and peak airway pressure were monitored from the ventilator.
The left carotid artery was cannulated with a polyethylene catheter (Portex Ltd., Kent, England) through a cervical cut-down to measure mean arterial blood pressure (MAP). An optical pulmonary artery thermodilution catheter (Opticath, Abbott, 7.5 French Critical care systems) was inserted into the right external jugular vein for measurement of central venous pressure (CVP), mean pulmonary artery pressure (MPAP), cardiac output (CO), mixed venous saturation (SvO2) and body core temperature. Heart rate (HR), MAP, central venous pressure (CVP) and MPAP were measured and monitored by Biopac (Biopac systems) or Sirecust 960 (Siemens). CO, SvO2 and body core temperature were monitored using an Oximetrix 3 (Abbott Critical Care Systems, Abbot Laboratories). Blood samples were obtained from the arterial line for analysis of SaO2, PaO2, PaCO2, Na+, K+, Ca2+, pH, base excess (BE) (GEM 3000 analyzer, Instrumentation Laboratories), hemoglobin (Hemoglobin Photometer Electrolux, Mecatronic AB). After the preparation, animals were not manipulated for 30 minutes to enable for physiological steady state. Data were registered at baseline prior to BABT, and at 1, 5, 10, 15, 30, 45, 60, 90 and 120 minutes after BABT ().
BABT 7.62 mm against armor (indirect hit)
In the animals selected for 7.62 mm BABT, a focally severe pulmonary contusion was induced, as previously describedCitation8–10 by a gunshot perpendicular to a body armor panel fixed on the mid-lateral right thorax with two 3-cm wide girdles (). The armor protection consisted of a 300 × 255 mm ceramic ballistic plate (weight 3 Kg) and 14 additional 0.24 mm aramid layers placed behind the plate, (Åkers Krutbruk Protection Inc.). A Swedish Armed Forces AK4 assault rifle, a Swedish-made version of the Heckler & Koch G3A3 assault rifle, equipped with a laser aiming device (Diode laser type S1889, Melles Griot), was fixed to a gun-carriage and placed 10 m from the ballistic plate. Nato 7.62 mm x 51 mm bullets (M/94, Danish Ammunitions-Arsenalet) with a mean bullet velocity of 803 (range 800–806) m/s, measured by an optical shutter device (Chronograph Beta model, Shooting Chrony, Inc.) were fired. The target was costa 8, the laser aim was pointed to the center of the rib, and firing was synchronized with the end of inspiration. Airway suctioning was started after three minutes and was performed when required. Before the shot, the ventilator was disconnected. After the shot, the animals were reconnected to the ventilator with a positive end-expiratory pressure of 6 cm H2O and mandatory minute ventilation mode. In addition, we performed new calculations of venous admixture, and included a previously reported group, for comparison of the protective properties of trauma attenuating backing in relation to the energy transfer.Citation11
Figure 3. A. photo of the 7.62 mm assault rifle in its experimental rig. B. photo of ceramic armor plate placement. Red dot marks point of projectile impact. C. photo of imprint of the skin after BABT and imprint in the ceramic plate. D. photo of the 65 mm BABT-simulator in its experimental rig. E. high speed camera photo of 65 mm projectile impacting the thoracic wall. F. photo of thoracic wall in swine with removed internal organs, with hole for measuring of the inside chest wall movement. Red dot is the reflection of the laser measurement beam on the inside of the thoracic wall.
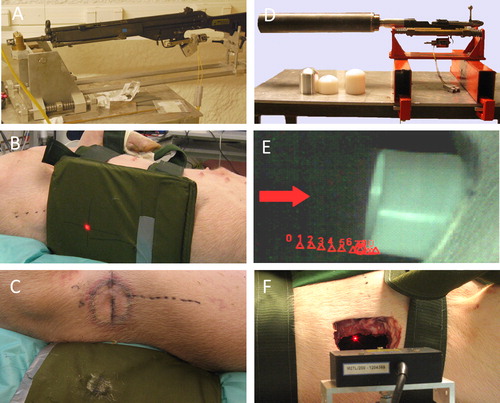
BABT-simulator 65 mm against skin (direct hit)
The BABT-simulator was a gun powder driven weapon. The gun powder was loaded in a Mauser-type cartridge case which shot 65 mm plastic projectiles. The amount of gun powder was calibrated for desired velocity, for each projectile weight. The pipe was smooth bore. Polyethylene projectiles weighing 35, 44 or 58 g were used at velocities 60 − 140 m/s. The frontal area (38.5 cm2) and shape of the projectile were intended to be similar to the back-face impression recorded in plastilina witness backing. The shooting distance was 1.5 m (). Before the shot, the ventilator was disconnected. After the shot, the animals were reconnected to the ventilator with a positive end-expiratory pressure of 6 cm H2O and mandatory minute ventilation mode.
Chest wall movement measurements (dead swine with organs removed)
19 swine were euthanized in a meat processing facility and had thoracic and abdominal organs removed prior to delivery to the lab. The swine were selected to groups 65 mm (n = 12) and 7.62 mm (n = 7), placed in supine position, and a 5 × 10 cm hole was opened, by cutting 1-2 ribs, in the chest wall opposite to the point of impact. BABT was inflicted on the right side of the chest wall and the monitoring hiatus was placed on the left chest wall. The inside of the chest wall was concave. Therefore, a reflex tape was fixed on the inside of the right chest wall at the point of impact by cyanoacrylate glue 6 × 6 mm, to enable optimal reflection of the laser measurements (). Each swine was subjected to 1-7 impacts for the measurements, until rib fractures occurred.
Synchronization of the high-speed video camera was performed by a trigger pulse from the velocity meter. A laser distance meter (ML M27L/200, MEL) measured the chest wall impression (the distance to the object) by a triangulation method. The high frequency pulsed laser beam was reflected from a point on the interior side of the chest wall. The change in direction of the reflection was measured by a positioning sensor (CCD-array). It was sensitive in the range 240 ± 100 mm. The weapon (assault rifle or BABT-simulator) targeted a point 5 cm behind and 5 cm above the inferior angle of the scapula. The reflection point for the distance meter was on pointed toward the inside of the thoracic wall at the expected point of impact. The 65 mm projectile velocity was measured with a conventional light curtain speedometer and the retardation, after contact with the chest wall, was recorded with a high-speed Kodak CCD camera taking 30 000 frames per second, mounted above the target area (video in supplemental online material) The 7.62 mm projectile velocity was measured by a Chrony chronograph Alpha Master. The distance between the photosensitive detectors was 1 foot (304.8 mm). Data collection was performed with aby Nicolet 12-bit Trancient recorder and Accura 100 Nicolet s/n: IDA 0200138, with a 50 kHz filter and Kodak high speed camera. The discreet signal analysis and script were performed by Matlab and the plugin “Signal Processing Toolbox”.
Calculations
The Ek of the projectiles was calculated from the Ek equation:
where V0 was the terminal speed of the projectile before impact and m was the mass of the projectile ().
Figure 4. Validation of the 65 mm BABT-simulator to 7.62 BABT. A-B. The back-face signature and max speed of the thoracic wall did not differ between 7.62 mm and the 65 mm BABT-simulator. C. the back-face signature of the BABT-simulator correlated to Ek. D-E. Logistic regression of rib fracture and back-face signature from the BABT-simulator. Rib fractures had a 50% likelihood at back-face signature 22.95 mm, area under ROC curve 0.93. F. Example of retardation of the 65 mm projectile on thoracic impact. Ek was calculated for the velocity just before impact.
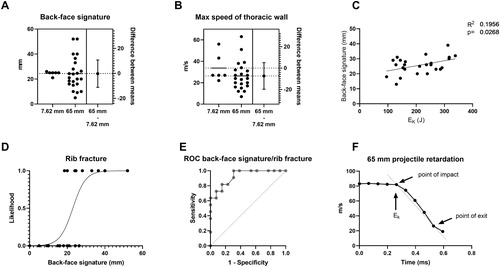
Venous admixture (Q’s/Q’t) was calculated by the shunt equation (Berggren equation):Citation12
where Q’s/Q’t = shunt fraction (shunt flow divided by total cardiac output), CcO2 = pulmonary end capillary O2 content, same as alveolar O2 content = SaO2 x Hb x 1.3. SaO2 was assumed at 100% in the lungs. CaO2 = arterial O2 content (SaO2 x Hb x 1.3), CvO2 = mixed venous O2 content (SvO2 x Hb x 1.3). V’A/Q’ = alveolar minute ventilation/cardiac output. Units: CcO2/CaO2/CvO2 = mL/L, Hb = g/L, SaO2 = %.
Statistical analyses
Statistical analyses were done using GraphPad Prism version 9.0.0 (GraphPad Software). Baseline values were collected at 0 hours. The primary outcome was venous admixture (Q’s/Q’t). Comparisons of back-face signatures and the max speed of the thoracic wall for 7.62 mm and 65 mm were analyzed by a non-inferiority design, and estimation plots were performed from unpaired t-tests. The back-face signature was fitted with linear regression with predictor Ek. Rib fracture and Ek were fitted with simple logistic regression. All temporal data sets were analyzed with a mixed effects model, fixed effects (type III) and Tukeýs multiple comparisons test. The physiological response 5 minutes after impact of the 65 mm BABT-simulator (the peak of response) was fitted with linear regression with predictor Ek. Plotted bars display the standard error of the mean. p < 0.05 was considered significant.
Results
Validation of the BABT-simulator
The BABT-simulator was validated to BABT from 7.62 mm bullets impacting a ceramic ballistic plate armor by two characteristics: back-face signature (maximal impression distance) of the armor and max speed of the thoracic wall on dead pigs. Both factors were measured by laser. The back-face signature mean was 24.5 mm for 7.62 and 24.3 mm for the 65 mm BABT-simulator (difference between means ± SEM −0.18 ± 5.3, 95% confidence interval −11.1 to 10.7) (). The max impression speed mean was 33.7 m/s for 7.62 and 26.3 m/s for the 65 mm BABT-simulator (difference between means ± SEM −7.35 ± 6.1, 95% confidence interval −19.8 to 5.1) (). The 95% confidence interval was visualized in estimation plots, where the 95% confidence interval was displayed. The confidence intervals for differences included zero, indicating that the mean of back-face signature and the mean of max speed were not statistically different (non-inferiority approach) between 7.62 mm BABT and the 65 mm BABT-simulator.
BABT-simulator calculations of physiological effect
Using the 65 mm BABT-simulator, the back-face signature correlated to Ek of the 65 mm projectile (R2 0.1956, p = 0.0268) (). Rib fractures were confirmed by manual palpation, and detected with a 50% likelihood at back-face signature 23.0 mm (95% CI of likelihood 18.5 to 29.0 mm) (), area under the receiver operating characteristic (ROC) curve 0.9266, 95% confidence interval 0.8277 to 1.000, p = 0.0004, equation: log odds = −6.703 + 0.2921*X (). Ek of the 65 mm projectiles were calculated just before impacting the thoracic wall (m/s) and time (ms), an illustration of which is given in .
Physiological responses to graded BABT
pO2 displayed similar responses after 7.62 mm BABT and 65 mm BABT-simulator. Differentiating velocity and weight of the 65 mm projectile caused a graded physiological response. There was an inverse linear correlation between pO2 and Ek (R2 0.3447, p = 0.0026) (). The regression equation of pO2 on Ek was Y = −0.02007*X + 11.79. By calculation, the criterion pO2 was 0 kPa when the predictor Ek was 587 J.
Figure 5. Physiological effects of graded BABT. A-D. pO2 displayed a similar effect profile after high velocity BABT by 7.62 mm assault rifle and BABT-simulator. Differentiating velocity and speed of the 65 mm projectile caused a graded physiological response. E-H. SvO2 displayed a similar effect profile after high velocity BABT by 7.62 mm assault rifle and BABT-simulator. I-L. MAP displayed a similar effect profile after high velocity BABT by 7.62 mm assault rifle and BABT-simulator. M-P. MPAP displayed a similar effect profile after high velocity BABT by 7.62 mm assault rifle and BABT-simulator. Q-T. Q’s/Q’t displayed a similar effect profile after high velocity BABT by 7.62 mm assault rifle and BABT-simulator. *p<.05, **p<.01, ***p<.005.
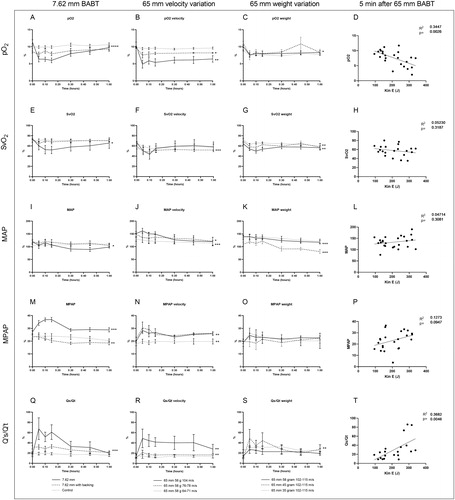
SvO2 displayed similar responses after 7.62 mm BABT and 65 mm BABT-simulator. Differentiating velocity and weight of the 65 mm projectile caused a graded physiological response. There was no linear correlation between SvO2 and Ek (R2 0.0523, p = 0.3187) ().
MAP displayed similar responses after 7.62 mm BABT and 65 mm BABT-simulator. Differentiating velocity and weight of the 65 mm projectile caused a graded physiological response. There was no linear correlation between MAP and Ek (R2 0.04714, p = 0.3081) ().
MPAP displayed similar responses after 7.62 mm BABT and 65 mm BABT-simulator. Differentiating velocity and weight of the 65 mm projectile caused a graded physiological response. There was no linear correlation between MPAP and Ek (R2 0.1273, p = 0.0947) ().
Q’s/Q’t displayed similar responses after 7.62 mm BABT and 65 mm BABT-simulator. Differentiating velocity and weight of the 65 mm projectile caused a graded physiological response. There was a linear correlation between Q’s/Q’t and Ek (R2 0.3682, p = 0.0046) (). The regression equation of Q’s/Q’t on Ek was Y = 0.1930*X − 10.72. By calculation, the criterion Q’s/Q’t was 100% when the predictor Ek was 574 J.
Discussion
In this study we show that Ek transferred to and absorbed by the thorax correlated to pulmonary gas exchange impairments. To defeat a bullet, the body armor must dissipate the Ek of the bullet. The energy is dissipated by deformation of the armor, deformation or fragmentation of the bullet, and deformation of the underlying body wall. Although a bullet can be effectively stopped from penetrating the body, the energy transfer has the potential to cause serious injury or even death.
The most used safety standard for body armor BABT prevention in both civilian and military applications is based on a maximum back-face signature of 44-mm in clay (Roma Plastilina 1). It is based on a relationship between goat-thorax deformation, when covered with armor and exposed to pistol shots, and probability of lethality.Citation13 The standard was meant to be preliminary at the time of conception in the 1970s, and not to have the widespread use it has today. The NIJ (National Institute of Justice, USA) BABT standard has also been used in applications for which it was never intended nor validated.Citation13 Examples of unintended use include the assessment of hard-body armor, body armor for small individuals, and impacts with rounds with much higher speed and kinetic energy than the pistol ammunition used in the original investigation. Impacted hard-armor plates load the torso differently than soft body armor, which could create different injury patterns in BABT situations.Citation13 Therefore, to enable improved predictions of safety, current research on BABT injuries should investigate the physiologic consequences of high velocity BABT and hard plate armors.
We have shown that BABT injuries can extend to the underlying organs as a result of the rapid acceleration and localized deformation of the thoracic wall and damage the lungsCitation11 and the heart.Citation14 High velocity projectile BABT caused an immediate and severe hypoxia in swine which peaked at 5 minutes.Citation9 The hypoxia was related to a severe and transiently increased venous admixture (Q’s/Q’t) in the exposed lung,Citation10 and we hypothesized that the gas exchange would deteriorate in relation to Ek. Measuring the absorbed Ek is methodologically challenging, and investigations of porcine chest trauma by bolt guns have not assessed graded injury.Citation15,Citation16
We therefore constructed a BABT-simulator, a device that shot 65 mm plastic projectiles, which allowed for measurements of Ek transfer and adjustable energy levels. To validate the BABT-simulator to comparable effects as real high projectile velocity BABT, we calibrated the speed and size of the projectiles to create a similar back-face signature (impression distance) of 24 mm, and maximum impression speed of the thoracic wall of 24-34 m/s, as high velocity projectile BABT from a 7.62 mm bullet impacting a ceramic plate armor at 800 m/s.Citation8–10 The back-face signature correlated linearly to the Ek with a low R2=0.20. The low R2 suggests that a back-face signature threshold alone may be inadequate when assessing lethality of high velocity BABT.
The energy levels delivered by the BABT-simulator caused rib fractures with a high correlation to back-face signature and a 50% likelihood at back-face signature 23.0 mm (area under the ROC curve 0.93), which was an indication of adequate trauma to the thoracic wall. Hence, reaching the 44 mm threshold of back-face signature would result in a high likelihood of rib fractures (95% CI of likelihood 18.5 to 29.0 mm). The main loading modes during impacts to ribs from thoracic trauma are local bending and shearing. Motion responses such as displacement and velocity, and internal forces are not good correlates in high-speed impacts.Citation17
We then assessed the physiological consequences of graded BABT. We have shown that most consequences, if survivable, are reversible within one hour, which is why the observation time was set to 60 minutes.Citation10 We specifically assessed markers of pulmonary gas exchange and circulation at 5 minutes: pO2, SvO2, MAP, MPAP and venous admixture (Q’s/Q’t). In our experience, these physiological measurements are affected the most by BABT, and it is likely that lethality from high velocity BABT is a consequence of severe gas exchange impairments at 5 minutes.Citation10 Venous admixture (Q’s/Q’t) and pO2 correlated linearly to the Ek and were described as linear functions. From these functions, we predicted a lethal Ek transferred to the thorax. pO2 of 0 kPa and venous admixture (Q’s/Q’t) of 100% would likely result in death, as pulmonary changes occurred for 20-30 minutes before subsiding. The Ek required for pO2 = 0 kPa and venous admixture (Q’s/Q’t) = 100% were 587 J and 574 J respectively. We suggest that these energy levels may be associated with limits of survivability, although verification requires survival studies. Consequently, body armor development for high velocity BABT may consider safety margins below these limits.
It is possible that trauma attenuating backings decrease the pulmonary impairments. Trauma attenuating backing decreases the size of the lung contusion, prevents from hemoptysis and reduces transient peak pressures in the thorax by 91%.Citation11 We therefore performed new calculations of venous admixture and pulmonary function, in a group of pigs exposed to 7.62 mm BABT with trauma attenuating backing.Citation11 This allowed for comparisons of the protective properties of trauma attenuating backing in relation to the energy transfer. The protective properties of trauma attenuating backing could be verified in this study by decreased venous admixture (Q’s/Q’t).
Some limitations need to be discussed. First, the observation time was limited to 60 minutes. The study was designed to determine acute lung function impairments that cause immediate respiratory failure, which is an important aspect of potential treatment targets to prevent death in prehospital or tactical environments. Respiratory mechanics are affected early after pulmonary contusion.Citation18 Future studies may include longer observation times to investigate delayed effects of BABT trauma, which may be characterized by lung hemorrhage, alveolar collapse, pulmonary edema and an inflammatory responseCitation18–20 but were beyond the scope of this investigation. Second, the similar size and anatomy of swine organs and human organs allow the model to be particularly beneficial for translational research. For the field of respiratory medicine, the similarities between swine and human lungs give porcine models the potential of advancing translational medicine. However, interspecies differences in lung function, such as decreased pulmonary compliance in swine, may limit extrapolation to humans with BABT.Citation21,Citation22 Third, while the energy calculations represent the first predictions of lethality from BABT based on lung physiology, the findings need to be verified in survival studies. Fourth, it is possible that multiple shots may cause cumulative damage to the rib, which may confound the likelihood for rib fracture in relation to the back-face signature, an aspect which should be explored in future studies.
The pulmonary consequences by BABT display unique features compared to experimental pulmonary contusion. BABT-specific models are therefore needed for adequate assessments of injuries related to protective equipment. Body armors validated for handgun- and grenade fragment protection are still used, where high velocity weapons are a realistic threat, and few reports include data of energy levels equal to- or higher than 7.62 mm rifle bullets.Citation3 Light body armors may not provide enough protection from the kinetic energy transferred from a high energy projectile and the development and increased use of light-weight body armors may increase injuries.Citation4 Body armor designs and injury assessments should therefore include relevant BABT-models to encompass the severe injury spectrum from high velocity projectile BABT. The BABT-simulator allowed for improved assessments of the physiological response of BABT. The establishment of lethality predictions from lung physiology as an alternative to clay impressions may facilitate the development of body armor, to meet the requirements of future ballistic safety equipment and the development of new BABT safety criteria.
Conclusions
A BABT-simulator was constructed which allowed for graded BABT. The Ek transferred to the thorax correlated to pulmonary gas exchange impairments. pO2 and venous admixture (Q’s/Q’t) correlated linearly to Ek and predicted lethal Ek from BABT to ≥574 J, which should be verified in survival studies.
Supplemental_online_material_BABT_kinE.mp4
Download MP4 Video (837.9 KB)Acknowledgements
The study was funded by the Swedish defense. We would like to thank Lars-Gunnar Olsson for excellent technical assistance.
Disclosure statement
No potential competing interest was reported by the authors.
Data availability statement
The data that support the findings of this study are available from the corresponding author, [MG], upon reasonable request.
References
- Cannon L. Behind armour blunt trauma-an emerging problem. J R Army Med Corps. 2001;147(1):87–96. doi:https://doi.org/10.1136/jramc-147-01-09.
- Liden E, Berlin R, Janzon B, Schantz B, Seeman T. Some observations relating to behind-body armour blunt trauma effects caused by ballistic impact. J Trauma. 1988;28(1 Suppl):S145–S8.
- Carr DJ, Horsfall I, Malbon C. Is behind armour blunt trauma a real threat to users of body armour? A systematic review. J R Army Med Corps. 2016;162(1):8–11. doi:https://doi.org/10.1136/jramc-2013-000161.
- Gryth D, Rocksen D, Persson JK, et al. Severe lung contusion and death after high-velocity behind-armor blunt trauma: relation to protection level. Mil Med. 2007;172(10):1110–1116. doi:https://doi.org/10.7205/milmed.172.10.1110.
- Wilhelm M, Bir C. Injuries to law enforcement officers: the backface signature injury. Forensic Sci Int. 2008;174(1):6–11. doi:https://doi.org/10.1016/j.forsciint.2007.02.028.
- Luo S, Xu C, Wang S, Wen Y. Transient pressure wave in the behind armor blunt trauma: experimental and computational investigation. Comput Methods Biomech Biomed Eng. 2017;20(3):308–318. doi:https://doi.org/10.1080/10255842.2016.1228908.
- Wen Y, Xu C, Wang S, Batra RC. Analysis of behind the armor ballistic trauma. J Mech Behav Biomed Mater. 2015;45:11–21. doi:https://doi.org/10.1016/j.jmbbm.2015.01.010.
- Rocksen D, Gryth D, Druid H, Gustavsson J, Arborelius UP. Pathophysiological effects and changes in potassium, ionised calcium, glucose and haemoglobin early after severe blunt chest trauma. Injury. 2012;43(5):632–637. doi:https://doi.org/10.1016/j.injury.2010.10.002.
- Gunther M, Sonden A, Gustavsson J, Arborelius UP, Rocksen D. Feasibility of pleural and perilesional subcutaneous microdialysis to assess porcine experimental pulmonary contusion. Exp Lung Res. 2020:46(5):117–127. doi:https://doi.org/10.1080/01902148.2020.1742252.
- Rocksén D, Arborelius UP, Gustavsson J, Günther M. Severe, transient pulmonary ventilation-perfusion mismatch in the lung after porcine high velocity projectile behind armor blunt trauma. Exp Lung Res. 2020;46(8):271–282. doi:https://doi.org/10.1080/01902148.2020.1797246.
- Sonden A, Rocksen D, Riddez L, et al. Trauma attenuating backing improves protection against behind armor blunt trauma. J Trauma. 2009;67(6):1191–1199. doi:https://doi.org/10.1097/TA.0b013e3181a5b0e1.
- Sven B. The oxygen deficit of arterial blood caused by non-ventilating parts of the lung. Acta Physiol Scand. 1942;11(4).
- Hanlon E, Gillich P. Origin of the 44-mm behind-armor blunt trauma standard. Mil Med. 2012;177(3):333–339. doi:https://doi.org/10.7205/MILMED-D-11-00303.
- Kunz SN, Arborelius UP, Gryth D, et al. Cardiac changes after simulated behind armor blunt trauma or impact of nonlethal kinetic projectile ammunition. J Trauma. 2011;71(5):1134–1143. doi:https://doi.org/10.1097/TA.0b013e318232b079.
- Batchinsky AI, Jordan BS, Necsoiu C, Dubick MA, Cancio LC. Dynamic changes in shunt and ventilation-perfusion mismatch following experimental pulmonary contusion. Shock. 2010;33(4):419–425. doi:https://doi.org/10.1097/SHK.0b013e3181b8bcd9.
- Batchinsky AI, Weiss WB, Jordan BS, Dick EJ, Jr., Cancelada DA, Cancio LC. Ventilation-perfusion relationships following experimental pulmonary contusion. J Appl Physiol (1985)). 2007;103(3):895–902. doi:https://doi.org/10.1152/japplphysiol.00563.2006.
- Shen W, Niu Y, Stuhmiller JH. Biomechanically based criteria for rib fractures induced by high-speed impact. J Trauma. 2005;58(3):538–545. doi:https://doi.org/10.1097/01.ta.0000158802.75760.f9.
- Raghavendran K, Notter RH, Davidson BA, Helinski JD, Kunkel SL, Knight PR. Lung contusion: inflammatory mechanisms and interaction with other injuries. Shock. 2009;32(2):122–130. doi:https://doi.org/10.1097/SHK.0b013e31819c385c.
- Simon B, Ebert J, Bokhari F, et al. Management of pulmonary contusion and flail chest: an Eastern Association for the Surgery of Trauma practice management guideline. J Trauma Acute Care Surg. 2012;73(5):S351–S361. doi:https://doi.org/10.1097/TA.0b013e31827019fd.
- Ware LB. Pathophysiology of acute lung injury and the acute respiratory distress syndrome. Semin Respir Crit Care Med. 2006;27(4):337–349. doi:https://doi.org/10.1055/s-2006-948288.
- Woolcock AJ, Macklem PT. Mechanical factors influencing collateral ventilation in human, dog, and pig lungs. J Appl Physiol. 1971;30(1):99–115. doi:https://doi.org/10.1152/jappl.1971.30.1.99.
- Judge EP, Hughes JML, Egan JJ, Maguire M, Molloy EL, O’Dea S. Anatomy and bronchoscopy of the porcine lung. Am J Respir Cell Mol Biol. 2014;51(3):334–343. doi:https://doi.org/10.1165/rcmb.2013-0453TR.