Abstract
The expression of colonization factors by gut bacteria, the growth rate of gut bacteria, and the rate of plasmid exchange by gut bacteria indicate that biofilms are a normal component of bacterial growth in the large bowel. Further, in vitro experiments demonstrate that growth of normal enteric bacteria in biofilms can be facilitated by secretory IgA (SIgA) and by mucins, 2 major components of the gut milieu. However, biofilms have not been previously observed in the normal gut. In this study, bacterial colonies characteristic of biofilms were observed by electron microscopy in normal rat, baboon, and human gut by electron microscopy. Confirming these results, acridine orange staining of flash-frozen tissues revealed biofilms in the mucus lining along normal gut epithelium. Immunofluorescenct microscopy supported this finding and demonstrated an association between IgA and the biofilms. These findings provide direct evidence that biofilms are present and may play an important role in the commensal relationship between enteric bacteria and their hosts. Hematoxylin and eosin staining of formalin-fixed tissues resulted in dissociation of the luminal contents from the epithelium, suggesting that the association between biofilms and the gut epithelium is sensitive to some conditions used to preserve tissue for histologic evaluation.
As early as 1936 it was established that bacteria can exist in both a bound, sessile state, now recognized as a biofilm, and in a free-floating or swimming condition, known as the planktonic state [Citation[1]]. Later research affirmed not just the ubiquity of biofilms, but the many advantages that this strategy holds for bacteria [Citation[2–6]]. The cumulative insight gained from years of research is that biofilms reflect the most common mode of steady-state bacterial growth while planktonic growth is characteristic of microbial dissemination [Citation[7]].
Prior work on the molecules expressed by normal gut bacteria has led investigators to postulate that biofilms should be found as a part of the normal gut flora [Citation[8–12]]. Further, the general tendency of microbes to form biofilms has led Hooper and Gordon to postulate the same [Citation[13]]. Hooper and Gordon [Citation[13]], in a discussion of factors that direct establishment and maintenance of a spatially diversified gut microflora, speculated that nutrient exchange in the gut might occur through biofilms. The rationale behind this speculation was the potential usefulness of biofilms in the complex bacteria–bacteria and bacteria–host interactions that take place in the gut. Licht et al. performed a study describing the plasmid transfer rates in biofilms and in the gut [Citation[14]] and found that the transfer rate was consistent with that of biofilms but not with planktonic growth. The finding that biofilms can be mediated by secretory IgA (SIgA) and mucins in vitro [Citation[15]] is consistent with the hypothesis that the formation of biofilms in vivo is a standard mode of bacterial growth in the gut.
Given the expected presence of biofilms in the large bowel, it is curious that biofilms have not been previously identified in the normal gut. Although a number of authors all speculate that biofilms are present in the gut, they consistently acknowledge the lack of direct observations of biofilms in the gut [Citation[8–12]].
In this study, tissues from primates and from rodents were examined by electron microscopy to determine whether biofilms were in fact present on the colonic epithelium. Additional studies were performed by light microscopy to confirm and extend observations made by electron microscopy. This work provides new insight into the nature of the host–microbial interactions and the importance of biofilms in gut biology.
MATERIALS AND METHODS
Tissue Procurement
For procurement of tissues for light microscopy, samples were obtained from several sources. From rats (n = 9), 2- to 3-cm lengths of gut were harvested from live, anesthetized animals. Baboon samples (n = 2) were obtained from animals immediately following euthanasia. Baboons were euthanized following unrelated experiments involving the lung. All experiments involving animals were approved by the Duke University Animal Care and Use Committee. Anonymous human appendix samples (n = 5) were obtained from tissue that was discarded during the normal course of a kidney–pancreas transplant. Following procurement of rat tissue, an embedding medium for frozen tissue specimens (Tissue-Tek OCT compound, Sakura Finetechnical, Tokyo, Japan) was injected into the gut lumen by flushing gently in the same direction as normal fecal stream. This technique created a column of preservation agent that displaced the bulk stook from the lumen without disrupting the biofilm layer. Bulk stool was removed from sections of human appendix, and the sections were placed in the OCT compound so that the luminal side of the sample, with remaining fecal material, was face up and covered with the OCT compound. All samples (rat and human) were then flash-frozen in liquid nitrogen and sectioned on a cryostat. These techniques proved to be reliable methods for removing the bulk stool while preserving biofilms. Tissues from baboons were not submitted to this procedure because of the possibility of pathogen transmission from unfixed baboon tissue. Baboon tissues were only used in studies involving fixation and electron microscopy (see below).
Tissue Staining
Four-micrometer cryosections were prepared from frozen biopsies and mounted on microscope slides. Mounted tissue sections were stored at −80°C until needed. For immunohistochemistry, slides were air-dried at room temperature and fixed with 10% formalin in phosphate-buffered saline, pH 7.4, for 10 min at room temperature and rinsed with phosphate–buffered saline, pH 7.2. Rat IgA was detected using affinity-isolated, goat anti-rat IgA (ICN, Aurora, OH). Since SIgA in the rat is rapidly and efficiently transported from serum into the bile and excreted into the bowel, leaving the serum deficient in rat SIgA[Citation[16–18]], anti-rat IgA antibodies were absorbed with rat sera to remove any cross reactivity with other immunoglobulin. Human IgA was detected by using affinity isolated, goat anti-human IgA (α- chain specific; Sigma, St. Louis, MO). Goat antibody was detected by using affinity-isolated, fluorescein- conjugated donkey F(ab′)2 anti-goat IgG (ICN), which shows no reactivity to rat or human serum proteins. Slides were coverslipped with 50 to 100 µL of Vectashield mounting medium with 1.5 µg/mL DAPI (Vector Laboratories, Burlingame, CA). To determine whether staining was specific for the primary antibody, slides not treated with primary antibody were incubated with the fluorescein-conjugated anti-goat antibody and evaluated. All such controls were found to be negative, indicating that the staining was specific for the primary antibody.
To visualize bacterial colonies and their surrounding matrices, slides were fixed in 95% ethanol, gradually hydrated to distilled water, rinsed with 1% acetic acid for 6 s and then washed twice in phosphate-buffered saline, pH 7.4. Slides were incubated with 0.1% acridine orange (Sigma) in 67 mM phosphate buffer, pH 6.0, for 3 min, washed with phosphate buffer for 1 min and differentiated in 100 mM CaCl2 for 30 s. Slides were coverslipped with Vectashield mounting medium.
Electron Microscopy
Biopsies for electron microscopy were obtained from rats (n = 2), baboons (n = 2), or humans (n = 2) and were fixed in 4% glutaraldehyde in 0.1 M cacodylate buffer with 3.4% sucrose, pH 7.4. Two to 10 biopsies (average 4.7) were obtained from each individual. After fixation, samples were washed 3 times for 10 min each in cacodylate buffer and postfixed for 1 h in 1% osmium, buffered with 0.1 M cacodylate. After one 10-min wash in cacodylate buffer and two 10-min washes in 0.11 M veronal acetate buffer, pH 7.2, tissues were en bloc stained for 1 h with veronal acetate-buffered 1% uranyl acetate. They were then dehydrated in a graded series of alcohols (35%, 70%, 95%, and 2 × 100%), followed by 2 changes of propylene oxide. They were infiltrated with propylene oxide/resin mixtures and embedded in Poly/Bed 812 resin, (Polysciences, Warrington, PA). Finally, sections were cut with a diamond knife on a Reichert-Jung Ultracut E ultramicrotome and poststained with aqueous uranyl acetate and lead citrate. They were examined by using a Philips EM-300 or EM-400 electron microscope. As excepted (see Discussion section), the glycocalyx of the epithelium and the associated adherent mucus layer were detached from the epithelium in many fields. However, some fields were identified within each block in which the glycocalyx was not detached from the epithelium, and the photos shown in the Results section are typical of those.
RESULTS
One feature of biofilm growth is that the bacteria within the biofilm can grow in distinct layers, the bacteria on the top of the biofilm being different from those on the inner layers of the biofilm [Citation[19]]. Consistent with this feature of a biofilm, bacteria adjacent to the luminal surface of the gut epithelium of a normal baboon were found by electron microscopy to be present in distinct layers, with smaller bacteria occupying the area near the mucosal surface and larger bacteria being in the outer layer of the bacterial colony (, B).
Fig. 1 Bacterial colonies adjacent to the normal gut epithelium of baboon (A–C), human (D), and rat (E) as observed by transmission electron microscopy. Biopsies from normal animals and from humans were fixed with glutardehyde, osmium, and uranyl acetate; embedded in epoxy resin; ultrathin sectioned; post-stained with uranyl acetate and lead citrate; and examined using an electron microscope. Both small, slender rod-shaped and large, ovoid, and thick rod-shaped bacteria were observed in the cecum of the baboon (A–C), whereas only larger bacteria were observed in the appendix of the human and the cecum of the rat. A matrix, or amorphous material surrounding the bacteria, was identified in all fields. Results are representative of 2 baboons, 2 humans, and 2 rats. The bar represents 2 μm.
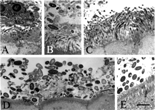
Another characteristic feature of biofilms is the presence of a porous material, termed an extracellular matrix, that surrounds the bacteria and comprises 50–90% of the total biofilm volume [Citation[6], Citation[19]]. Such extracellular matrices were found by electron microscopy to surround the bacteria along the gut epithelium of normal baboons (), of humans (), and of normal rats (.
The orientation of microorganisms with respect to flow is another common feature of biofilms. Use of a rapid flash-freezing technique in the absence of chemical fixative and staining of sections with acridine orange (see Methods) revealed dense colonies of bacteria, 2–30 µm thick and adjacent to the gut epithelium of the large bowel in all rats examined. The contour of the colonies followed the contours of the epithelium (), and bacteria were oriented parallel to the wall of the bowel.The colonies were more common in the cecum, ascending colon, and transverse colon than in the descending colon and were almost completely absent in the small bowel. The bacteria could be found oriented parallel to the bowel and perpendicular to the plane of longitudinal sections () but not of transverse sections (, F). This indicated that the bacteria were oriented parallel to the direction of flow, consistent with the expected properties of biofilms. Of note is that bacteria in colonies adjacent to the gut epithelium in the cecum appeared to be randomly oriented to a greater extent than bacteria adjacent to the epithelium of the ascending and transverse colon. Further, bacterial colonies adjacent to the epithelium of the human appendix were not oriented in any particular direction (. These observations might be expected, given the lower rate of flow in the cecum and appendix and given that the orientation of bacteria within biofilms is dependent on the flow of the system. In contrast to the appearance of flash-frozen tissues, tissues either washed with saline prior to flash freezing or tissues fixed in 10% formalin showed no material in contact with the gut epithelium (not shown), suggesting that the luminal contents had separated from the mucosal surface during the procedure.
Fig. 2 Bacterial colonies adjacent to the normal rat and human gut epithelium. Rat colon (A–F) or human appendix (G) was flushed with OCT preservative, Flash-frozen, cut, stained with acridine orange and examined by fluorescent microscopy. Longitudinal (parallel to flow of bowel contents) sections (A–D) and transverse (perpendicular to flow of bowel contents) sections (E and F) were cut. Longitudinal sections of the ascending colon (A and B) and the transverse colon (C and D) are shown at 2 different magnifications. Transverse sections of the ascending colon (E) and transverse colon (F) are also shown. The human appendix (G) was sectioned longitudinally. Results are representative of 9 rats and 5 humans. The bars represent 30 μm (A, C, and G) and 15 μm (B and D–F).
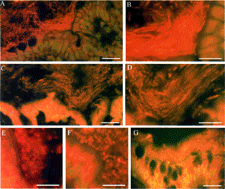
To determine whether SIgA was associated with biofilms, we used the same rapid freezing technique without chemical fixatives described above and evaluated the presence of SIgA in normal rat gut and in human appendix. Secretory IgA was identified in rat bowel using goat anti-rat IgA and a fluorescein-labeled anti-goat IgG. By using serial sections stained with acridine orange to identify biofilms, it was found that the SIgA staining was consistently high in biofilms adjacent to rat gut epithelium (, C). Staining of human appendix by using goat anti-human IgA and a fluorescein-labeled anti-goat IgG also revealed IgA associated with bacterial biofilms along the luminal surface of the human epithelium (, D). Bacteria within the biofilms of the rat gut appeared less organized using the fluorescenated anti-IgA stain than with an acridine orange stain, perhaps because the staining technique disrupted the biofilm to a greater extent. In addition, bacteria in the rat and in the human gut could not be as clearly visualized using the fluorescent anti-IgA stain as with the acridine orange stain, perhaps because the SIgA is associated with the matrix surrounding the bacteria as well as the bacteria themselves. Nevertheless, the findings do demonstrate that SIgA is associated with the biofilms lining the gut epithelium of the normal rat and the human. The observation that biofilms growing along the adherent mucus layer of the normal gut epithelium are associated with SIgA casts doubt on the conventional thinking that the primary function of SIgA involves aggregation of planktonic bacteria for the sole purpose of eliminating those bacteria from the gut.
Fig. 3 Association of IgA with biofilms adjacent gut epithelium. Samples were submerged in OCT, flash-frozen, sectioned, and stained with goat anti-rat IgA or goat anti-human IgA followed by a fluorescein labeled anti-goat IgG. Sections were counterstained with the nucleic acid-specific stain DAPI. The presence of IgA (indicated by green fluorescence, A and B) and nucleic acid (indicated by blue fluorescence, C and D) was evaluated by using a fluorescence microscope. One section from the rat cecum (A and C) and one section from the human appendix (B and D) are shown. The portion of the sections photographed was kept constant so that the regions in (A) and (B) correspond exactly to the regions in (C) and (D), respectively. Biofilms were characterized by heavy staining for IgA (green fluorescence) and moderate staining for nucleic acid (blue fluorescence), whereas the nuclei of the gut epithelium were characterized by heavy staining for nucleic acid but not for IgA. The bar represents 15 μm.
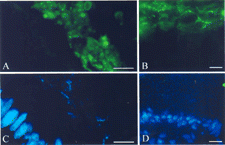
DISCUSSION
While a large number of micrographs of bacteria in the gut have been published, the bacteria are generally disordered and lack the features characteristic of a biofilm. One exception to this is the series of electron micrographs published by Banwell and colleagues [Citation[8]], who identified biofilms in the small and large bowel of rats fed phytohemagglutinin lectins. The lectins induce a pathological state characterized by maladsorption of a variety of nutrients. Biofilms in the small bowel apparently reflected abnormal growth of microorganisms in the presence of the lectin. However, lectins that crosslink cell surface sugars, such as phytohemagglutinins, are known to stabilize cell surface coats during fixation processes [Citation[20]]. Thus, it remains unknown whether Banwell's observations of biofilms in the large bowel were “abnormal” or whether the presence of the lectin may have improved preservation of “normal” biofilms during preparation of the samples for electron microscopy. The present study would suggest the latter.
There are some technical hurdles that might account for the lack of prior observations concerning biofilms in the normal gut. Our experience taught us that some of the techniques commonly used for preparing gut tissue for staining, such as washing with saline or fixation with aldehydes, tend to disrupt biofilms from the gut surface. This might be because the glycocalyx, or cell coats, of both the bacteria and the epithelial cells where the biofilm is anchored are easily disrupted by chemical fixatives and by other nonphysiologic solutions [Citation[20–22]].
The evidence presented herein that biofilms are found in the gut is consistent with a considerable amount of previously published work. For example, the gut mucosa must provide a barrier to invasion by pathogens and, at the same time, remain highly permeable to allow the efficient exchange of nutrients across its surface. Consistent with these requirements, biofilms are known to be both porous, allowing nutrients to pass through, and barrier-like, excluding some larger molecules and other microorganisms. Further, unlike planktonic bacteria, biofilms are presumably unable to transverse the epithelial barrier, thus providing a potentially less invasive type of bacterial growth in the gut. There are a number of other examples of prior work supporting indirectly the idea that biofilms are present in the gut. Growth of enteric bacteria, like the growth of bacteria in previously characterized biofilms, is steady state, involves colonization factors, is typical of symbiotic relationships, is relatively slow, and is relatively resistant to antibiotics. Thus, the present findings, corroborated by previous work, lead to the conclusion that biofilms are a normal component of the gut milieu. Given this conclusion, the fundamental mechanisms underlying host–microbial relationships in the gut require reexamination. For example, the possibility that the immune system facilitates the growth of microorganisms in biofilms merits consideration.
We thank David Howell for helpful discussion, Hai Fan Lin for the use of his microscope. Susan Hester for help with electron microscopy, and Julie A. Fuller for tissue staining. This work was supported in part by the Fannie E. Rippel Foundation and by grant P30 DK34987 from the National Institutes of Health.
REFERENCES
- Zobell CE, Anderson DQ.. Observations on the multiplication of bacteria in different volumes of stored seawater and the influence of oxygen tension and solid surfaces. Biol Bull Woods Hole. 1936; 71: 324–42
- Costerton JW.. Introduction to biofilm. Int J Antimicrob Agents. 1999; 11: 217–221, discussion. 237–239. [PUBMED], [INFOTRIEVE], [CSA], [CROSSREF]
- Costerton JW.. Overview of microbial biofilms. J Indust Microbiol. 1995; 15: 137–140. [CSA], [CROSSREF]
- Costerton JW, Cheng KJ, Geesey GG, et al, Bacterial biofilms in nature and disease. Annu Rev Microbiol. 1987; 41: 435–464. [PUBMED], [INFOTRIEVE], [CROSSREF]
- Costerton JW, Geesey GG. Cheng K-J. How bacteria stick. Sci Ame. 1978; 238: 86–95
- Costerton JW, Lewandowski Z, Caldwell DE, Korber DR, Lappin-Scott HM.. Microbial biofilms. Annu Rev Microbiol. 1995; 49: 711–745. [PUBMED], [INFOTRIEVE], [CSA], [CROSSREF]
- Lappin-Scott HM, Costerton JW.. Bacterial biofilms and surface fouling. Biofouling. 1989; 1: 323–342. [CSA]
- Banwell JG, Howard R, Cooper D, Costerton JW.. Intestinal microbial flora after feeding phytohemagglutinin lectins (Phaseolus vulgaris) to rats. Appl Environ Microbiol. 1985; 50: 68–80. [PUBMED], [INFOTRIEVE], [CSA]
- Banwell JG, Howard R, Kabir I, Costerton JW.. Bacterial overgrowth by indigenous microflora in the phytohemagglutinin- fed rat. Can J Microbiol. 1988; 34: 1009–1013. [PUBMED], [INFOTRIEVE], [CSA]
- Macfarlane S, McBain AJ, Macfarlane GT.. Consequences of biofilm and sessile growth in large intestine. Advances in Dental Research. 1997; 11: 59–68. [PUBMED], [INFOTRIEVE], [CSA]
- Cassels FJ, Wolf MK.. Colonization factors of diarrheagenic E. coli and their intestinal receptors. Journal of Industrial Microbiology. 1995; 15: 214–226. [PUBMED], [INFOTRIEVE], [CSA], [CROSSREF]
- Macfarlane GT. Macfarlane S. 2000. Growth of mucin degrading bacteria in biofilms. Methods in Molecular Biology. 2000; 43: 9–452
- Hooper LV, Gordon JI.. Commensal host-bacterial relationships in the gut. Science. 2001; 292: 1115–1118. [PUBMED], [INFOTRIEVE], [CROSSREF]
- Licht TR, Christensen BB, Krogfelt KA, Molin S.. Plasmid transfer in the animal intestine and other dynamic bacterial populations: the role of community structure and environment. Microbiology. 1999; 145: 2615–2622. [PUBMED], [INFOTRIEVE]
- Bollinger RR, Everett ML, Palestrant D, Love SD, Lin SS, Parker W.. Human secretory immunoglobulin A may contribute to biofilm formation in the gut. Immunology. 2003; 109: 580–587. [PUBMED], [INFOTRIEVE], [CROSSREF]
- Jackson GD, Lemaitre-Coelho I, Vaerman JP, Bazin H, Beckers A.. Rapid disappearance from serum of intravenously injected rat myeloma IgA and its secretion into bile. Eur J Immunol. 1978; 8: 123–126. [PUBMED], [INFOTRIEVE]
- Lemaitre-Coelho I, Jackson GD, Vaerman JP.. High levels of secretory IgA and free secretory component in the serum of rats with bile duct obstruction. J Exp Med. 1978; 147: 934–939. [PUBMED], [INFOTRIEVE], [CROSSREF]
- Lemaitre-Coelho I, Jackson GD, Vaerman JP.. Rat bile as a convenient source of secretory IgA and free secretory component. Eur J Immunol. 1977; 7: 588–590. [PUBMED], [INFOTRIEVE]
- Lawrence JR, Korber DR, Hoyle BD, Costerton JW, Caldwell DE.. Optical sectioning of microbial biofilms. J Bacteriol. 1991; 173: 6558–6567. [PUBMED], [INFOTRIEVE]
- Luft JH.. The structure and properties of the cell surface coat. Int Rev Cytol. 1976; 45: 291–382. [PUBMED], [INFOTRIEVE]
- Allen A, Pearson JP.. The gastrointestinal adherent mucous gel barrier. Methods Mol Biol. 2000; 125: 57–64. [PUBMED], [INFOTRIEVE]
- Fassel TA, Edmiston CE, Jr.. Bacterial biofilms: strategies for preparing glycocalyx for electron microscopy. Methods Enzymol. 1999; 310: 194–203. [PUBMED], [INFOTRIEVE]