Abstract
A new quantitative biostratigraphical method, based on an ‘index of stratigraphical abundance’ (ISA), has been used to refine the stratigraphical range of 50 dinoflagellate cyst taxa. Most of these correspond to Quaternary representatives of Achomosphaera or Spiniferites. Some extinct taxa are also discussed when their morphology is close to that of recent species (e.g. Spiniferites lenzii, S. twistringiensis and S. pseudofurcatus), as well as some species possibly related to the motile Gonyaulax spinifera complex. The ISAs of S. mirabilis and S. hyperacanthus are similar, as well as for S. elongatus and S. sphaericum. Although initially presumed as indicating morphotypes of a same taxon, it seems rather that the ISAs similarites must be interpreted as an indication of close ecological preferences. The emergence of forms of Spiniferites with low to absent septa appears relatively late (compared to the range of the genus), but the first occurrence of intergonal processes is relatively early, indicating that it is probably a character deeply rooted into the generic genome. The previously calculated phylogeny of some Gonyaulax motile cells shows a different tree pattern than the stratigraphical first appearance succession of their presumed cyst equivalents.
1. Introduction
Spiniferites Mantell 1850 is one of the most geographically widespread and stratigraphically long-ranging dinoflagellate cyst genus (Hultberg and Malmgren Citation1995). This finding holds true both in the ancient (post Jurassic) and recent (Pliocene-Quaternary) series as shown by the numerous records of this genus, especially for the type species, Spiniferites ramosus (Ehrenberg Citation1837) Mantell 1854. Indeed, this taxon presents an exceptional case of stratigraphic longevity, as it is reported in the fossil record from the Upper Jurassic to Present, with a thriving distribution. With a stratigraphical longevity exceeding 140 Ma, Spiniferites ramosus is probably one of the longest living among the Quaternary dinoflagellate species, and perhaps among all species in the living realm.
The genus Spiniferites encompasses more than 100 species (Fensome and Williams Citation2004) among which 20 have at least been recorded in Quaternary strata. In connection with the “Quaternary Spiniferites Workshops” held in Montreal (2014) and Ostend (2015) (see the general Introduction of this volume), we herein focus primarily on Quaternary and Neogene species. Stratigraphical range charts were published for some Spiniferites species (e.g. Harland Citation1978; Sarjeant Citation1979), and the one of 55 Spiniferites species by Hultberg and Malmgren (Citation1995) remains a reference work, but over the last twenty years, new data were published and some new species have been erected. In addition, representations of species occurrences by a simple line between inception and extinction provide limited information.
To go beyond this method based on the only alternative presence/absence (i.e. occurrence), a new approach is here proposed based on an ‘index of stratigraphical abundance’ (ISA) calculated for various Spiniferites species. It is an occurrence probability of a taxon for each geological stage, which is the geochronological resolution selected here. On one hand, this allows to display the time interval in which a taxon is the most abundant relatively to other dinoflagellate cyst species, and secondly to compare the ISA’s variations between different taxa.
The motile equivalent of Spiniferites ramosus is not clear and might be attributed to Gonyaulax spinifera-type (Claparède and Lachmann Citation1857) Diesing (Citation1866) motile cells (e.g. Wall and Dale Citation1970; Lewis et al. Citation1999; Ellegaard et al. Citation2003; Rochon et al. Citation2009). Other types of cysts have been attributed to that motile complex, sometimes not belonging to the genus Spiniferites (e.g. Bitectatodinium tepikiense, Nematosphaeropsis labyrinthus, Tectatodinium pellitum; cf. ‘Heterospory’ in Head Citation1996; Lewis et al. Citation2001).
The present work is an opportunity to test whether dinoflagellate cyst taxa, which have been attributed to the motile Gonyaulax spinifera complex (Dodge Citation1989), present similarities in their 'index of stratigraphical abundance'. The study should also clarify the stratigraphical origin of the Spiniferites species still present in the modern seas.
2. Material and methods
2.1. The databases
In order to collect the occurrences of the selected taxa in the most exhaustive way possible, a very large number of records is needed. The PALYNODATA online database (Palynodata Inc. and White Citation2008) offers such an opportunity by providing a large number of species and references that list the geographical and stratigraphical local occurrences of each taxon. For the present work, a total of 2,279 publications from the organic-walled dinoflagellate cyst literature depicting 2,333 geological sequences (field sections or boreholes) was extracted from the PALYNODATA database. As the database has not been updated since 2006, it was completed with a personal database that contains data before and after 2006 for well-dated sequences, preferably with a dating not based on the dinoflagellate cysts themselves. It indexes occurrences of 1,585 organic-walled dinoflagellate cyst species from 214 additional publications (396 geological sequences) of global dinoflagellate cyst literature.
The merging of the two databases lead to 2,729 worldwide geological sequences from the Carnian (Upper Triassic) to today. Among them, 1,671 geological sequences provided species of Spiniferites or of the Gonyaulax spinifera complex. No stratigraphical synthesis (e.g. Sarjeant Citation1979; Powell Citation1992; Williams et al. Citation1993; Hultberg and Malmgren Citation1995) was integrated in the merged database, in order to not duplicate the data already included.
The final database therefore has two dimensions, with: the 46 geological stages, from the Carnian to the Holocene, in columns, and the taxa in rows. When a paper deals with several sites, each site was considered independently.
The occurrence of the taxon is 0 if it was not observed in the sequence and 1 if it has been recorded. The value of 0.5 is sometimes used when the dating is uncertain or imprecise (e.g. Senonian or Lower Miocene). The species referred as 'cf.' were not taken into account.
2.2. The index of stratigraphical abundance (ISA)
An ‘index of stratigraphical abundance’ (ISA) has been developed to get more information from the biostratigraphical records, allowing to go beyond the establishment of the stratigraphical range of a taxon by determining its oldest and youngest occurrences.
The ISA allows to not only to determine the stratigraphical range of a taxon as indicated by its earliest and latest occurrences but also to obtain a full occurrence probability distribution.
The ISA was calculated on the basis of the number of geological sequences in which a taxon was recorded during a time interval (= ‘nOcc’ for number of occurrences) versus the total number of sequences documented in the database (= ‘nSeq’ for total number of geological sequences) for the same time interval:
ISA = nOcc/nSeq
For each taxon, an ISA was calculated for each geological stage considering its worldwide occurrences. If necessary, the geographical area of the record can be restricted to an oceanic basin, to a (paleo)hemisphere, etc. Overall, the ISA can be considered as an estimate for the occurrence probability of a taxon during each geological stage. For example, 157 sequences of the database span the Holocene. Among these sequences, 66 record Spiniferites ramosus. The ISA of Spiniferites ramosus for the Holocene is 66/157 = 0.42. That means that in a global stratigraphical point of view, the probability to encounter a specimen of this species in a Holocene sequence is about 42%, implying that an ISA value cannot exceed 1. The database used here includes 2,729 different geological sequences and 1,585 organic-walled dinoflagellate cyst species. To calculate ISA, it is first needed to determine the number of sequences documented in the database for each geological stage (the time interval chosen here). Since the database from which it is calculated is substantial, featuring on average over a hundred publications for each geological stage (from 11 sequences for the Carnian to 308 for the Albian, cf. ), the ISA constitutes a significant estimator.
Table 1. Number of records for each species and each geological stage as registered in our database. The total number of sequences considered is indicated in the fourth line for each geological stage.
3. Results
The stratigraphical occurrences of 50 taxa have been investigated. Most of them correspond to Spiniferites or Achomosphaera species recorded in Quaternary strata. Some extinct taxa are also depicted when their morphology is close to that of recent species (e.g. Spiniferites lenzii, Spiniferites twistringiensis and Spiniferites pseudofurcatus), as well as some species possibly related to the motile Gonyaulax spinifera complex.
3.1. Spiniferites ramosus, oldest occurrence of the genus?
Duxbury (Citation1977), Davey (Citation1979, Citation1982), Williams and Bujak (Citation1985), Costa and Davey (Citation1992), Stover et al. (Citation1996) consider that, at least in NW Europe, the first appearance of Spiniferites ramosus marks the lowermost Valanginian. This is also what Hardenbol et al. (Citation1998) followed by Ogg and Ogg (Citation2008c) report for the Boreal/North Atlantic domain but, according to the lowermost Berriasian occurrences recorded by Monteil (Citation1992, Citation1993), they consider an earlier occurrence in the Tethyan domain. That point of view is still confirmed by many experienced palynostratigraphers (James Riding, personnal communication).
Such a statement deserves a closer scrutiny since several Spiniferites ramosus occurrences were recorded in Jurassic strata. Deflandre (Citation1938) mentioned a single specimen attributed to Hystrichosphaera furcata (now Spiniferites ramosus) from the Lower Oxfordian cliffs of Villers-sur-Mer (France). The description given by the author was very succinct, with no mention of trifid processes. Due to its poor preservation, the specimen was not illustrated. Such a record seems not suitable for biostratigraphy. Sarjeant (Citation1960) reported one specimen of Hystrichosphaera furcata that was illustrated (pl. 14, fig. 17) from the ‘Upper Calcareous Grit’ Formation from Yorkshire (England). The age of this formation is considered as late Oxfordian (op. cit.). Still from Yorkshire in the 'Ampthill Clay' Formation, Sarjeant (Citation1962) reported two specimens (not depicted) of Hystrichosphaera furcata from an Oxfordian pit exposure. It is of note that the collective efforts of lots of reliable palynologists over many years have never replicated these occurrences in NW Europa. Gitmez and Ertug (Citation1999) studied five Upper Jurassic sections from Turkey and mentioned few sparce specimens of Spiniferites ramosus in only one of them. The illustrated specimen (pl. 9, fig. 4-5) does not look like Spiniferites ramosus sensu holotype. In addition, this specimen appears very fresh and could be a contamination from the Neogene upper part of the outcrop. In their synthesis, Pestchevitskaya et al. (Citation2011) recorded Spiniferites ramosus from a little below the Jurassic/Cretaceous (J/K) boundary (late Upper Volgian) in outcrops from Sub-Polar Ural, and from the J/K boundary near Laptev Sea. Unfortunately no specimen was depicted. Other specimens have been reported from Jurassic strata, but in boreholes for which the possibility of caving cannot be excluded (e.g. Brideaux Citation1977, Upper Jurassic of NW Canada; Chen Citation1978 early-middle Kimmeridgian and Portlandian from Madagascar; Thusu and Vigran Citation1985 unspecified Jurassic age from Libya, considered by the authors as caved; Guy-Ohlson Citation1986, Toarcien-Aalenian of Sweden). These borehole occurrences have not been considered in this work.
These records show that there are no unequivocal reports of Spiniferites ramosus from the Jurassic supported by photographic evidence. Cretaceous occurrences (particularly Neocomian one) are numerous enough to be considered as significant.
Berriasian (lowermost Cretaceous) records of Spiniferites ramosus show a scaterred distribution since it was reported in various outcrops from Iran (Ashraf Citation1979), India (Kumar Citation1980), Gulf of Mexico (Riley and Fenton Citation1984), Libya (Thusu et al. Citation1989), SE France (Monteil Citation1992, Citation1993), NW Siberia (Lebedeva and Nikitenko Citation1999).
If we consider the bibliographical data as reliable, particularly those from outcrops, it appears that the geographical extension of Spiniferites ramosus appears restricted during the Berriasian, and almost worldwide during the Valangian.
Thus, we can consider that the first occurrence datum (FOD) of Spiniferites ramosus can be placed in the lowermost Berriasian and the Valanginian worldwide extension could corresponds to its first common occurrence (FCO). After that, the species presents consistent ISA values above ca 0.2 from the Valanginian until present days (). Two ISA optima are clearly distinct around Maastrichtian and Quaternary times with an interposed minimum centered on the Middle Miocene ().
Figure 1. ‘Index of stratigraphical abundance’ (ISA) of some stratigraphically long ranging Spiniferites and Achomosphaera species. The chronostratigraphicalframework (left column) is from ICS (Citation2016). Species are presented in order of appearance. The scale bar corresponds to ISA =0.1.
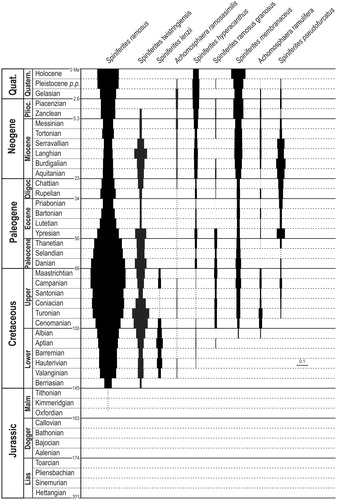
Davey (Citation1979) defined Spiniferites ramosus as a biostratigraphical marker of the ‘Spiniferites ramosus’ dinozone (Davey Citation1979) covering the mid-Early Valanginian to the top Valanginian in the Boreal realm. That marker could correspond to the FCO of the species.
Several other species of Spiniferites first appear during the Berriasian (e.g. Spiniferites alatus Duxbury Citation1977, Spiniferites “multibrevis” now Spiniferites twistringiensis (Maier Citation1959) Fensome et al. Citation1990) which makes uncertain whether Spiniferites ramosus would be the oldest representative of the genus Spiniferites. Achomosphaera who is a genus morphologically very close to Spiniferites might have been the first of the two to appear since Achomosphaera neptunii (Eisenack Citation1958) Davey and Williams (Citation1966) was recorded as early as the Tithonian (uppermost Jurassic; Harding et al. Citation2011).
3.2. Neogene and Quaternary Spiniferites plexus
The first and last occurrences of the selected species are presented in . Some species deserve further attention and are discussed below. The ISAs of the Quaternary and Pliocene species that first appear before the Cretaceous/Paleogene boundary are shown in . Those which inception is post-Cretaceous are shown in .
Figure 2. ‘Index of stratigraphical abundance’ (ISA) of some stratigraphically short ranging Spiniferites and Achomosphaera species. The chronostratigraphicalframework (left column) is from ICS (Citation2016). Species are presented in alphabeticalorder. The scale bar corresponds to ISA =0.1.
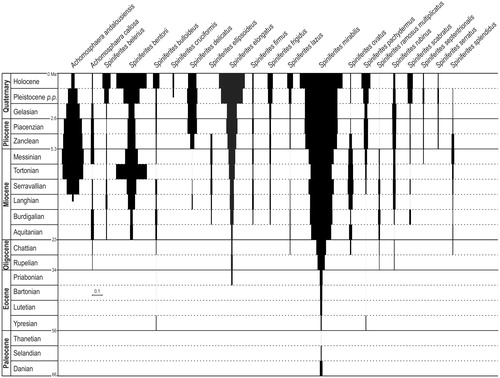
Table 2. Taxonomical references of the taxa studied with the age of their first and last appearances. FAD: First appearance datum; LAD: Last appearance datum; FCO: First common occurrence; LCO: Last common occurrence. The arrow indicates the species is still living. See section ‘References’ for the full references of the citations.
Both Achomosphaera andalousiensis Jan du Chêne (Citation1977) and Spiniferites septentrionalis Harland (Citation1977) possess processes with fenestrate/trabeculate distal tips and the distinction is not always easy (see Londeix et al. Citation2018). Some authors consider these species as synonym, and it is difficult, in the absence of clear illustrations, to know which of these two species has been recorded. It is probable that the compiled record of each of these species does not refer to a unique taxon. Nevertheless, the FAD of Achomosphaera andalousiensis seems to be upper Langhian (Powell Citation1986b; McCarthy and Mudie Citation1996, see also Dybkjær and Piasecki Citation2010) while the first appearance of Spiniferites septentrionalis is upper Tortonian-Messinian (Harland Citation1979). Spiniferate cysts with trabeculate/fenestrate distal tips are still present today. However, it is difficult to assign them to one or the other of these taxa since they present intermediate features (e.g. Morzadec-Kerfourn Citation1979; Mangin Citation2002). Nevertheless, Spiniferites septentrionalis is present in Upper Quaternary (Harland Citation1977) sediments and typical Achomosphaera andalousiensis have been recorded and illustrated by Head (Citation1997) from Piacenzian strata. Achomosphaera andalousiensis gave its name to a dinozone established by Piasecki (Citation1980) that covers almost the entire upper Miocene (e.g. Piasecki Citation1980; Manum et al. Citation1989; McCarthy et al. Citation2013).
Remarks: When Harland (Citation1977) erected Spiniferites septentrionalis, he mentioned Philip C. Reid according who (personnal communication) the new species was synonymous with Spiniferites ramuliferus as recorded by Reid (Citation1974). In that work Reid considered the specimen figured by Downie and Singh (Citation1970) as Hystrichosphaera ramosa () as synonymous of Spiniferites ramuliferus. Neither Reid nor Downie and Singh evoked the presence of perforated or trabeculate process terminations. Synonymy of Spiniferites septentrionalis with Spiniferites ramuliferus sensu Reid (Citation1974) (non Deflandre) is not followed here, however Spiniferites ramuliferus sensu Reid (Citation1974) and Hystrichosphaera ramosa sensu Downie and Singh (Citation1970) (non Ehrenberg) seem to correspond to the same taxon.
Figure 3. ‘Index of stratigraphical abundance’ (ISA) of some cyst species possibly related to the motile Gonyaulax spinifera complex. The chronostratigraphicalframework on left column is from ICS (Citation2016). Species are presented in alphabeticalorder. The scale bar corresponds to ISA =0.1.
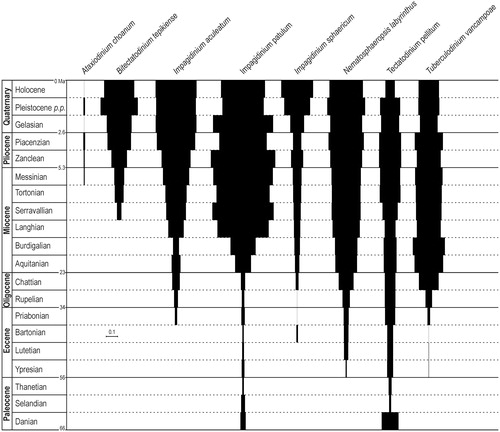
The first formal occurrence of Achomosphaera callosa Matsuoka (Citation1983) is Londeix and Lopes (Citation2014) from Aquitanian stratotype, but it is likely that this species appeared earlier in the Chattian (Powell Citation1986b as Achomosphaera cf. crassipellis) or in the upper Rupelian (Stampian, Londeix, unpub. data).
Achomosphaera ramosasimilis (Yun Citation1981) Londeix et al. Citation1999 was firstly recorded in the Santonian from Germany but the synonymy provided by the original author ranges from the Neocomian (as Hystrichosphaeridium ramuliferum, Gocht Citation1959) to the Upper Oligocene (as Achomosphaera ramulifera, Cookson and Eisenack Citation1974). Londeix (Citation1990) recorded this species in the Upper Valanginian from SE France and it was also recorded in the Gelasian from Sicily (Londeix et al. Citation1999). The youngest occurrence might be in Lower Pleistocene from Labrador Sea as Achomosphaera ramulifera (de Vernal and Mudie Citation1989).
Remarks: In the note accompanying the original description of Achomosphaera ramosasimilis Yun (Citation1981) states that the shape and arrangement of the processes and the structure of the central body are equivalent to Spiniferites ramosus ramosus. This taxon is here considered as a concept that coresponds to Spiniferites ramosus without septa between the processes.
The earliest suitable records of Spiniferites bentorii (Rossignol Citation1964) Wall and Dale (Citation1970) seem to be from Aquitanian by Edwards (Citation1986) and Powell (Citation1986b) respectively from South Carolina and NW Italy. The Paleogene records of Spiniferites bentorii (e.g. Frederiksen Citation1969; Bujak Citation1973; Islam Citation1984; Powell et al. Citation1996; Gedl Citation2005) are herein considered as not suitable since sparse, not illustrated, or with questionable identification.
The early record of Spiniferites bentorii subsp. truncata (Rossignol Citation1964) Wall and Dale (Citation1970) in the Upper Cretaceous from Western Xinjiang (China) by Yu and Zhang (Citation1980) appears very isolated compared to the other records for this taxon. This record is not taken into account here and the stratigraphical range of Spiniferites bentorii truncata is considered to be limited to its restricted and rare occurrences int the Quaternary.
The lowest occurrence of Spiniferites bulloideus Deflandre and Cookson 1955 might be Ypresian (Auffret and Graus-Cavagnetto Citation1975) but this record appears discrepant since the other oldest occurrences are significantly younger: Chattian (Londeix, unpub. data) and Aquitanian (Londeix and Lopes Citation2014).
Spiniferites cruciformis Wall and Dale in Wall et al. Citation1973 is endemic to the Black Sea, Marmara Sea, Caspian Sea, Aral Sea, and eastern Mediterranean (Zonneveld et al. Citation2013) and occurs mainly in brackish environments. Applying biostratigraphy in a such context is generaly not easy, and in the present case the oldest occurrences of this species are poorly dated: hypothetically within the range of Pliocene to Pleistocene (Eaton Citation1996) or Middle Pleistocene (Koreneva and Kartashova Citation1978). The undisputed oldest occurrences were recorded in the upper Pleistocene (Wall et al. Citation1973; Mudie et al. Citation2002, Citation2007; Londeix et al. Citation2009). Williams et al. (Citation1998) consider the stratigraphical range of Spiniferites cruciformis from 0.17 to 0.01 Ma. The species might be not extinct since it is present in some surface sediments of the ‘paratethysian’ area (Zonneveld et al. Citation2013).
The earliest clear occurrence of Spiniferites elongatus Reid Citation1974 seems to be from Tortonian from Northern Japan (Matsuoka Citation1983; Matsuoka et al. Citation1987). Closely related morphologies to Spiniferites elongatus were sporadically found in Rupelian strata in a section from Belgium (De Coninck Citation2001) and from a borehole from the Norwegian-Greenland Sea (Poulsen et al. Citation1996). All these records are earlier than the Pleistocene FAD stated by Williams et al. (Citation1998) and Ogg and Ogg (Citation2008a) and are in agreement with the Late Miocene occurrence stated by Bujak and Matsuoka Citation1986 and Stover et al. (Citation1996).
The stratigraphy of Spiniferites hyperacanthus (Deflandre and Cookson 1955) Cookson and Eisenack Citation1974 started in the Lower Cretaceous (). Its lowest occurrence lies in the Hauterivian from Germany (Kirsch and Below Citation1995). Otherwise, the Cretaceous species Spiniferites lenzii Below 1982c (see ) is a morphologically very similar species since, although slightly smaller, it also bears numerous intergonal processes. This morphological similarity casts doubt on the identifcation of the Cretaceous cysts as Spiniferites hyperacanthus, and the absence of illustration for these records does not eliminate the doubt (e.g. Masure Citation1988; Below and Kirsch Citation1994; Fiet and Masure Citation2001). In the same way, the record of Spiniferites lenzii in Cenozoic strata (Matsuoka Citation1983) is remote from the latest (Maastrichtian) continuous occurrence. It might be the result of a reworking, and it is not considered here. All of these occurrences are discussed in Section 4 (see below).
The oldest record of Spiniferites mirabilis (Rossignol Citation1964) Sarjeant Citation1970 is from the lowermost Danian of Israel (Eshet et al. Citation1992), and is confirmed by the record of a Spiniferites sp. cf. S. mirabilis in Danian from Senegal (de Klasz et al. Citation1987; Jan du Chêne Citation1988).
The Dinoflagellate cyst Zone V of Costa and Downie (Citation1979) covers the Upper Eocene and its lower boundary is marked by the first occurrence of Spiniferites mirabilis. It is now clear that the FAD of that species is older (, ).
The lowest occurrence of Spiniferites pachydermus (Rossignol Citation1964) Reid Citation1974 seems to located in the Serravallian (Matsuoka Citation1983) or the Upper Miocene (Mudie Citation1989; McMinn Citation1993; Suc et al. Citation1995) but in all the cases older than the Gelasian age stated by Ogg and Ogg (Citation2008a).
Spiniferites pseudofurcatus subsp. obliquus (Wall Citation1967) Lentin and Williams Citation1973 has almost exclusively been recorded by Wall in Quaternary sediments from the Caribbean Sea. The sole other record is by Wrenn and Kokinos (Citation1986) from an Upper Pleistocene section in Gulf of Mexico in which reworking is frequent. The stratigraphical range of Spiniferites pseudofurcatus (Klumpp Citation1953) Sarjeant, Citation1970 appears to end before the onset of the Quaternary (, ), so the specific allocation of the obliquus subspecies is questioned.
Foucher (Citation1974) recorded in the upper Turonian from France the oldest occurrence of Spiniferites pseudofurcatus (Klumpp Citation1953) Sarjeant, Citation1970. The highest ISA values of that species spans from the Ypresian to the Serravallian (). Some specimens have been recorded in Plio-Quaternary strata, but they occur rarely except in Mediterranean area where reworking is frequent (Versteegh and Zonneveld Citation1994; Corradini in Guerrera et al. Citation1985; Londeix et al. Citation1999).
The FAD of Spiniferites rubinus (Rossignol Citation1962 ex Rossignol Citation1964) Sarjeant Citation1970 appears to lie in the upper Burdigalian (offshore Portugal, McCarthy and Mudie Citation1996). The morphology of the specimen illustrated by El Beialy (Citation1990, pl.4, fig.1) from the Middle/Late Oligocene from Egypt appears too different from Rossignol’s species to be taken into account.
The lowest occurrence of Spiniferites splendidus Harland Citation1979 seems to be Chattian (Zevenboom Citation1996), even if there are few records of this taxon. Williams et al. (Citation1998) and Ogg and Ogg (Citation2008a) consider the last occurrence of Spiniferites splendidus as an index species for the mid/upper Zanclean boundary (synchronous with the top of Martini’s (Citation1971) NN13 calcareous nannofossils Zone). This datum should be considered obsolete since Mudie (Citation1986) and Stoker et al. (Citation1994) recorded Spiniferites splendidus in Gelasian strata. Furthermore, Mertens et al. (this volume) propose a synonymy with Spiniferites mirabilis.
The original description of Spiniferites multibrevis indicates the presence of intergonal processes, sometime up to three (Davey and Williams Citation1966). That feature being shared with Spiniferites hyperacanthus, the stratigraphy of this taxon is studied here. As Spiniferites twistringiensis (Maier Citation1959) Fensome et al. Citation1990 is considered to be a senior synonym of Spiniferites multibrevis (Davey and Williams Citation1966) Below 1982c (see Sarjeant Citation1983; Fensome and Williams Citation2004) the stratigraphical ranges of both species have been merged (, ). The overal range of Spiniferites twistringiensis appears to be Berriasian (as Spiniferites multibrevis: Ashraf Citation1979; Heilmann-Clausen Citation1987) to Serravallian () but it might be still present at Holocene (Grill and Quattroccio Citation1996).
3.3. Cyst species possibly related to the motile Gonyaulax spinifera complex
The FAD of Bitectatodinium tepikiense Wilson 1973 appears to be Lower Miocene within the planktonic foraminiferal Biozones N5 to N7, i.e. Burdigalian or may be upper Aquitanian (Powell Citation1988b, Citation1992; Stover et al. Citation1996) ().
Moshkovitz and Habib Citation1993 report Impagidinium aculeatum (Wall Citation1967) Lentin and Williams 1981 from Danian sediments. Although the material comes from outcrops, precluding caving, that record is far older from other ones that are Middle (Firth Citation1996, as Impagidinium sp. cf. I. aculeatum) to Upper Eocene (Brinkhuis Citation1992; Brinkhuis and Biffi Citation1993, as Impagidinium sp. cf. I. aculeatum; Gedl and Leszczynski Citation2005, as Impagidinium aculeatum).
It is recognized that Impagidinium patulum (Wall Citation1967) Stover and Evitt Citation1978 FCO is recorded since Lower Miocene (e.g. Powell Citation1986a, Citation1992; Brinkhuis et al. Citation2003; Dybkjaer Citation2004). Its first appearance is difficult to pinpoint since many occurrences were recorded from Danian strata (Moshkovitz and Habib Citation1993) to Upper Oligocene (e.g. Strauss Citation1993; Eidvin et al. Citation1998; Dybkjaer Citation2004), sometimes considered as caving (e.g. Eidvin et al. Citation1998) or with morphologies distant to the type material. Since illustration is not always provided, it is difficult to state.
Head and Nøhr-Hansen (Citation1999) consider Xenicodinium (as Tectatodinium) rugulatum to be a taxonomic junior synonym of Tectatodinium pellitum, therefore, the records taken into account herein are those of both names. The base of the dinoflagellate cyst ‘X. rugulatum Zonule’ proposed by Hansen (Citation1977) and located at the base of the Danian marks the FAD of Tectatodinium pellitum which is just below the base of the calcareous nannofossil Zone NP2 (Stover et al. Citation1996; Ogg and Ogg, Citation2008b), and approximately corresponds to the base of the foraminiferal Zone Plb, according to Berggren et al. (Citation1995). The older records of that taxon are thought doubtful (because questioned identification or not illustrated) by Head and Nøhr-Hansen (Citation1999).
The FCO of Tuberculodinium vancampoae (Rossignol Citation1962) Wall (Citation1967) is probably Priabonian as recorded by several authors (e.g. Costa et al. Citation1988; El-Bassiouni et al. Citation1988; Brinkhuis Citation1992; De Coninck Citation1999). The records by Fechner and Mohr (Citation1988) and Kar (Citation1985) respectively from Ypresian field section and Lutetian might indicate an earlier stratigraphically position for the appearance of this taxon. The record by Tea-yassia et al. (Citation1999) from lower Maastrichtian is probably due to caving and is not considered here.
4. Discussion
4.1. About Spiniferites ramosus and some others
On the whole, the stratigraphical range of a species is only meaningful when we deal with the same taxon. Since the objective of this study is to depict the stratigraphical continuity of Spiniferites species recorded in Quaternary sediments, the reference morphologies are those of Quaternary species as described in Quaternary literature (e.g. Harland Citation1983; Powell Citation1992; Rochon et al. Citation1999). Many morphological, environmental and sometimes genetic data are available for Quaternary species. The genetic stability over time of the dinoflagellate species is impossible to establish, however, it has been agreed that Spiniferites species have sufficient taxonomical/morphological stability to follow them through geological times.
This is particularly the case for Spiniferites ramosus with a stratigraphical range of nearly 145 Ma, which seems (an oddity in the biosphere evolution) a very peculiar (even strange) case in the biosphere story. Without genetic fingerprinting, the concept of the morphological species is the lone guiding thread we can follow along such time intervals. In the case of Spiniferites ramosus, it corresponds to an ovoid central body with a smooth to shagreenate surface, with gonal processes only and distal trifid, then sometimes bifid, terminations (see Mertens et al. and Londeix et al. Citation2018). The length of the process is variable and intergonal process may occasionally be present (maximum one per suture). This morphological concept corresponds to the Cretaceous type of the species, illustrated by Davey in Davey and Williams (Citation1966, fig.8) and is consistent with the Quaternary specimens (e.g. Rochon et al. Citation1999, pl.9, fig.4-6). Although stratigraphically distant, these two specimens illustrate the morphological standard of the species. The stratigraphical range depicted here concerns Spiniferites ramosus ramosus and excluded the many subspecies (> 32, Fensome and Williams Citation2004) assigned to Spiniferites ramosus.
Figure 4. ISA comparison of some species. Abscissa: geological stages from the Berriasian (lowermost Cretaceous) to the Holocene.
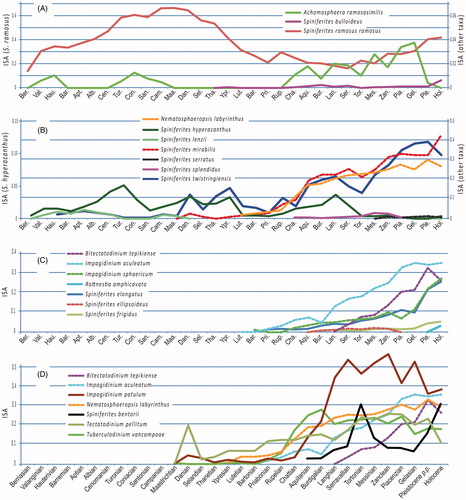
Figure 5. (A) Appearance chronology of five dinoflagellate cyst species according to their geological records as depicted by their ‘index of stratigraphical abundance’ (ISA). (B) Phylogeny of some living Gonyaulax species, inferred from partial LSU rDNA sequence data (redrawn after Ellegaard et al. Citation2003). The name of the cysts corresponding to their motile stage is enclosed in square brackets.
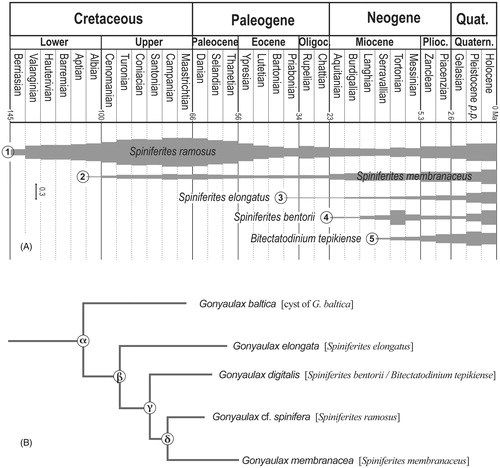
If we accept that a species is ubiquitous because it is able to adapt to many environmental conditions, it is logical that its stratigraphical perenity is important. This is indeed what is observed for Spiniferites ramosus which proves to be a common species in modern sediments and whose current geographical distribution is very important, especially in environments with high seasonality (e.g. Zonneveld et al. Citation2013). Thus the long stratigraphical range of Spiniferites ramosus might be regarded as a taxonomical stability, at least in a paleontological point of view. The ‘index of stratigraphical abundance’ for Spiniferites ramosus shows two main optima: one during the Late Cretaceous and the second one during the Quaternary (). In addition, Spiniferites ramosus ISA variations show that its FCO is in the base of Valanginian while its FOD is in the lowermost Berriasian.
The main features of Spiniferites hyperacanthus are the regular presence of several intergonal processes and low (or absent) septa. However, some modern specimens of this species show an unexpected morphological plasticity with the presence of well expressed septa (see Limoges et al. Citation2018). Such morphology is very close to that of Spiniferites lenzii and Spiniferites twistringiensis leading to wonder how to distinguish these taxa and whether they represent various morphotypes of the same species. The taxonomical identity of these taxa is not questioned here, but the discontinuous ISAs of Spiniferites hyperacanthus and Spiniferites lenzii are puzzling (). Nevertheless, the presence of low to absent septa (i.e. Spiniferites hyperacanthus) appears to be a feature more frequent during Neogene and Quaternary times than in previous periods. This is also observed with Spiniferites mirabilis ISA (), a species morphologically close to Spiniferites hyperacanthus. Spiniferites cf. Spiniferites lenzii illustrated by Oboh-Ikuenobe et al. (Citation1998, pl.9, figs. 2-3) from the upper Maastrichtian offshore Ivory closely ressembles Spiniferites hyperacanthus. Caving is unlikely since many specimens were encountered (op. cit.). On the opposite, specimen illustrated by Lister and Batten (Citation1988, pl.11, fig.6) as Spiniferites hyperacanthus appear very close to Spiniferites lenzii. Since it is difficult to to evaluate the records of non-illustrated Cretaceous specimens, no occurrence of this period has been ruled out here. On the other hand, the Plio-Pleistocene record of Spiniferites lenzii from Japan (Matsuoka Citation1983) is not taken into account.
The emergence of Spiniferites morphologies with intergonal processes appears relatively early (Berriasian-Valanginian), showing that it is probably a character deeply rooted into the genus genome.
4.2. ISA comparison for some possibly related taxa
During the Montréal and Ostend round tables dealing with Spiniferites and Achomosphaera (see Mertens et al. this volume) the synonymization of some species was discussed. The ISAs of some of them are here discussed.
Spiniferites ramosus, Achomosphaera ramosasimilis and Spiniferites bulloideus are morphologically close species. The first appearance of Spiniferites ramosus and Spiniferites bulloideus appear far apart, respectively Berriasian and no older than Ypresian ( and ). The time lapse between the first appearance of Spiniferites ramosus and Achomosphaera ramosasimilis appears closer, since the latter appears in the Valanginian. In addition, no similarity is observed between Spiniferites ramosus and Spiniferites bulloideus ISAs () but variations of Achomosphaera ramosasimilis ISA are very similar to those of Spiniferites ramosus during the Neogene and the Pliocene, leading to consider a possible taxonomical relationship between these two taxa.
With the same approach, Spiniferites mirabilis and Spiniferites hyperacanthus ISAs appear to be correlated from the Rupelian to the Pleistocene (). The similarity of their ISAs reinforces the assumption they could be two morphotypes of a same taxon. The records of Spiniferites serratus and Spiniferites splendidus are too few to make significative the comparison of their ISA to that of Spiniferites mirabilis.
It is of note that the ISA of Nematosphaeropsis labyrinthus is very close to that of Spiniferites mirabilis () This may suggest a possible link between the two taxa although the two morphologies are very different (intergonal processes and antapical veil in Spiniferites mirabilis, presence of trabeculae in Nematosphaeropsis labyrinthus).
Several types of cysts are possibly produced by Gonyaulax spinifera-type motile cells: e.g. Spiniferites elongatus, Spiniferites membranaceus, Spiniferites ramosus, Spiniferites bulloideus, Spiniferites mirabilis, Nematosphaeropsis labyrinthus, Bitectatodinium tepikiense, Tectatodinium pellitum, Ataxiodinium choane etc. (Rochon et al. Citation2009).
The ISAs variations through geological time of Spiniferites elongatus and Impagidinium sphaericum show an impressive covariance (). It gives substance to similar ecological affinities between the two species that are generally associated with sediments in polar to sub-tropical waters (Zonneveld et al. Citation2013; de Vernal et al. Citation2018). It also questions whether a biological affinity might exist between the two taxa. As respectively depicted by Helenes (Citation1986) and Ellegaard et al. (Citation2003) the paratabulations of Impagidinium sphaericum and Spiniferites elongatus show differences in the apical and precingular 6’’ paraplates, indicating their ISA covariance is rather due to ecological similarities instead of taxonomical ones. According to Harland (Citation1983), the motile cell of Impagidinium sphaericum would be a Gonyaulax sp. indet. That of Spiniferites elongatus is considered to be Gonyaulax spinifera or Gonyaulax elongata (Head Citation1996; Ellegaard et al. Citation2003). It is noteworthy that Helenes (Citation1986) already indicated paratabulation similarities between what he called Sphaericum pattern (represented by Impagidinium sphaericum) and the Spinifera pattern related to Gonyaulax spinifera.
To a lesser extent, the ISA variations of Spiniferites elongatus and Bitectatodinium tepikiense also show a fair similarity (), which probably has to be interpreted as ecological similarities. The records of Spiniferites ellipsoideus, Spiniferites frigidus and R. amphicavata are too few to make the comparison of their ISAs significant to that of Spiniferites elongatus.
Cysts of the motile species Gonyaulax digitalis (Pouchet Citation1883) Kofoid Citation1911 are thought to be referable to Spiniferites bentorii (cf. Head Citation1996) or to Bitectatodinium tepikiense (Ellegaard et al. Citation2003). The comparison of ISA of Spiniferites bentorii and Bitectatodinium tepikiense does not show any relationship between these two taxa ().
No other covariance has been detected between taxa related to Gonyaulax spinifera-type motile cells.
As inferred from partial LSU rDNA sequence data, Ellegaard et al. (Citation2003, fig.46) propose a phylogeny of some Gonyaulax motile species. According to this method, Gonyaulax baltica would have appeared first and Gonyaulax membranacea would have appeared last (). In this tree, Gonyaulax elongata, Gonyaulax digitalis (= Spiniferites bentorii or Bitectatodinium tepikiense, Head Citation1996; Ellegaard et al. Citation2003) and Gonyaulax cf. spinifera (= Spiniferites ramosus, Ellegaard et al. Citation2003) appear successively between the two former species. That topology differs from the appearance chronology of the cyst equivalent of these species since Spiniferites ramosus first occurs at ca. 145 Ma (lowermost Berriasian) and the cyst of Gonyaulax baltica only in the Holocene (less than 11 ka). In addition, the order of appearance of Bitectatodinium tepikiense, Spiniferites bentorii, Spiniferites elongatus, Spiniferites membranaceus is respectively around 13 Ma (Serravallian), 23 Ma or maybe 58 Ma (Aquitanian or Thanetian), 37 Ma (Priabonian) and 112 Ma (Albian) (, ). The stratigraphical appearances seem therefore to show a different pattern from that obtained by genetic analysis of motile cells, but this difference can simply mean that the stratigraphical range takes into account type morphologies, and not biological species.
5. Conclusion
The ‘index of stratigraphical abundance’ of the Quaternary Spiniferites species show only few similarities between them. Some couples of taxa show ISAs’ similarities, such as Spiniferites mirabilis and Spiniferites hyperacanthus, and to a lesser degree, Spiniferites ramosus and Achomosphaera ramosasimilis. ISAs’ comparisons with species outside of the Spiniferites genus sometimes show striking similarities such as for Spiniferites mirabilis and Nematosphaeropsis labyrinthus, and for Spiniferites elongatus and Impagidinium sphaericum. In a first approach, the similarities of ISA between two taxa could have been interpreted as indicative of cyst morphotypes belonging to the same species. The example of Spiniferites elongatus and Impagidinium sphaericum refutes this hypothesis since these two cysts do not have the same tabulation, which excludes the possibility that they could belong to the same species. This leads to dismiss the idea to use ISAs as a tool for taxonomical discrimination. However, it could be a suitable way to discern taxa having similar ecological preferences.
The fact remains that the ISAs allow a better visualization of abundance variations of a taxon, or a morphology, over time than the classic linear stratigraphical range. The ISA proves to be a valuable tool for locating in time the FCO and the LCO.
Moreover, it would be interesting to test the ISAs of some species (of Spiniferites and others) on a regional scale and not on a global scale, as was carried out in this paper, to refine the stratigraphical interest of taxa.
Acknowledgements
I wish to express my gratitude to James Riding (British Geological Survey) for his review enriched by his subsequent advice and constructive exchanges. Stephen Louwye (Ghent University) is thanked for his helpful and constructive reviews of the manuscrit and for his patience to improve the text, as well as Tristan Cantat-Gaudin (University of Padova) and Fabienne Marret (University of Liverpool).
References
- Ashraf AR, Erben HK. 1986. Palynologische Untersuchungen an der Kreide/Tertiär-Gernze west-mediterraner Regionen. Paläeontographica Abteilung B. 200(1):111–163.
- Ashraf AR. 1979. Die räto-jurassischen floren des Iran und Afghanistans. 6; Jurassische und unterkretazische dinoflagellaten und acritarchen aus Nordafghanistan. Palaeontographica Abteilung B. 169(4–6):122–158.
- Auffret JP, Graus-Cavagnetto C. 1975. Les formations Paléogènes sous-marines de la Manche Orientale. Données palynologiques. Bull Soc Géol France Ser 7. 17(5):641–655.
- Below R, Kirsch K-H. 1994. Die verteilung des palynophytoplanktons einer Dunkel/Hell/Dunkel sequenz des Hochsten Apt (Jacobi-Zone) von Vohrum (Niedersachsen/Deutschland) – neue methodische Ansatze bei der quantitativen Analyse Mariner Floren. Paläeontographica Abt B. 232(1):59–102.
- Berggren WA, Kent DV, Swisher CC III, Aubry M-P. 1995. A revised Cenozoic geochronology and chronostratigraphy. In: Berggren WA, Hardenbol J, editors. Geochronology, time scales and global stratigraphic correlation. Vol. 54. Tulsa: SEPM Special Publication; p. 130–212.
- Brideaux WW. 1977. Taxonomy of upper jurassic – lower cretaceous microplankton from the Richardson Mountains, District of MacKenzie, Canada. Geological Survey of Canada Bulletin 281, 89 p.
- Brinkhuis H, Biffi U. 1993. Dinoflagellate cyst stratigraphy of the Eocene/Oligocene transition in Central Italy. Mar Micropaleontol. 22(1):131–183.
- Brinkhuis H, Munsterman DK, Sengers S, Sluijs A, Warnaar J, Williams GL. 2003. Late Eocene – Quaternary dinoflagellate cysts from ODP Site 1168, off Western Tasmania. Proc Ocean Drill Prog Sci Results. 189:1–36.
- Brinkhuis H. 1992. Late Eocene to early Oligocene Dinoflagellate cysts from central and northeast Italy. PhD Rijksuniversiteit, Utrecht, 169 p.
- Bujak JP. 1973. Microplankton from the Barton Beds of the Hampshire Basin, England [Unpublished Ph.D. Thesis]. Sheffield: University of Sheffield, 455 p.
- Bujak N, Matsuoka K. 1986. Late Cenozoic dinoflagellate cyst zonation in the western and northern Pacific. Am Assoc Stratigraphic Palynologists. 17:7–25.
- Chen YY. 1978. Jurassic and Cretaceous palynostratigraphy of a Madagascar well [Unpublished PhD Thesis]. Arizona: Department of Geosciences, University of Arizona; 264 p.
- Claparède E, Lachmann J. 1857. Etudes sur les infusoires et les Rhizopodes. Mémoires de l'Institut Genévois. 6:392–412.
- Cookson IC, Eisenack A. 1974. Mikroplankton aus Australischen Mesozoischen und Tertiären Sedimenten. Paläeontographica Abt B. 148(1):44–93.
- Costa LI, Davey RJ. 1992. Dinoflagellate cysts of the Cretaceous System. In: Powell AJ, editor. A stratigraphic index of dinoflagellate cysts. London:. Chapman and Hall; p. 99–153. (British Micropaleontological Society Publication Series).
- Costa LI, Downie C. 1979. Dinoflagellates. In: Montadert L. et al., editors. Sites 405 and 406. Deep Sea Drilling Project, Initial Reports 48: Washington: U.S. Government Printing Office; p. 228–232.
- Costa LI, Manum SB, Meyer KJ. 1988. Great Britain/Norway;The Viking Graben. In: R. Vinken, compiler. The Northwest European tertiary basin. Vol. 100. Hannover: Geologisches Jahrbuch Reihe A; p. 330–332.
- Davey RJ, Williams GL. 1966. The genera Hystrichosphaera and Achomosphaera. In: Davey RJ, Downie C, Sarjeant WAS, Williams GL, editors. Studies on Mesozoic and Cainozoic Dinoflagellate Cysts. British Museum (Natural History) Geology, Bulletin, Supplement 3: London; p. 28–52.
- Davey RJ. 1979. The stratigraphic distribution of dinocysts in the Portlandian (latest Jurassic) to Barremian (Early Cretaceous) of northwest Europe. Am Assoc Stratigr Palynol Contrib Ser. 5B:49–81.
- Davey RJ. 1982. Dinocyst stratigraphy of the latest Jurassic to Early Cretaceous of the Haldager No. 1 borehole, Denmark. Geological Survey of Denmark, Series B, No. 6, 57 p.
- De Coninck J. 1999. Organic-walled phytoplankton biostratigraphy of the Eocene – Oligocene transition in the Kallo Borehole and the Rupelian stratotype area (northwestern Belgium). Bull Soc Belge Géol Paléontol d’Hydrol. 105(4):171–209.
- De Coninck J. 2001. Organic-walled microfossils in the Oligocene Grimmertingen and Neerrepen Sand Members from the Grimmertingen Type Locality. Geological Survey of Belgium, Professional Paper 2001/2, 294:58 p.
- de Klasz I, de Klasz S, Colin J-P, Jan du Chêne R, Ausseil-Badie J, Bellion Y, Peypouquet J-P. 1987. Apport de la micropaléontologie (foraminifères, ostracodes, dinoflagellés) à la connaissance stratigraphique et paléogéographique de la Formation des Madeleines (Danien du Sénégal). Cahiers de Micropaléontologie, special issue. 2(3–4):5–27.
- De Schepper S, Head MJ, Louwye S. 2009. Pliocene dinoflagellate cyst stratigraphy, palaeoecology and sequence stratigraphy of the Tunnel-Canal Dock, Belgium. Geol Mag. 146 (1):92–112.
- de Vernal A, Eynaud F, Henry M, Limoges A, Londeix L, Matthiessen J, Marret F, Pospelova V, Rochon A, Van Nieuwenhove N. 2018. Distribution and (paleo)ecological affinities of the main Spiniferites taxa in the Northern Hemisphere. Palynology. 42 Suppl:1.
- de Vernal A., Mudie P.J. 1989. Pliocene and Pleistocene palynostratigraphy at ODP Sites 646 and 647, eastern and southern Labrador Sea. Proc Ocean Drilling Prog Sci Results. 105:401–422.
- Deflandre G. 1938. Sur le microplancton des mers Jurassiques, conservé à l'état de matière organique dans les Marnes de Villers-Sur-Mer. Comptes Rendus Hebdomadaires des Séances de l’Académie des Sciences. 206:687–689.
- Diesing KM. 1866. Revision der Prothelminthen. Abtheilung: Mastigophoren. Sitzungsberichte der Kaiserlichen Akademie der Wissenschaften. Mathematisch-Naturwissenschaftliche Classe. Abteilung 1, Mineralogie, Botanik, Zoologie, Anatomie, Geologie und Paläontologie. 52:287–401.
- Dodge JD. 1989. Some revisions of the Family Gonyaulacaceae (Dinophyceae) based on a scanning electron microscope study. Bot Mar. 32:275–298.
- Downie C, Singh G. 1970. Dinoflagellate cysts from estuarine and raised beach deposits at Woodgrange, Co. Down, N. Ireland. Grana Palynol. 9:124–132.
- Duxbury S. 1977. A palynostratigraphy of thr Berriasian to Barremian of the Speeton Clay of Speeton, England. Palaeontographica Abt B. 160(1–3):17–67.
- Dybkjaer K. 2004. Dinocyst stratigraphy and palynofacies studies used for refining a sequence stratigraphic model–uppermost Oligocene to lower Miocene, Jylland, Denmark. Rev Palaeobot Palynol. 131:201–249.
- Dybkjær K, Piasecki S. 2010. Neogene dinocyst zonation for the eastern North Sea Basin, Denmark. Review of Palaeobotany and Palynology. 161:1–29.
- Eaton GL. 1996. Seriliodinium, a new Late Cenozoic dinoflagellate from the Black Sea. Rev Palaeobot Palynol. 91:151–169.
- Edwards LE. 1986. Late Cenozoic dinoflagellate cysts from South Carolina, U.S.A. In: Wrenn JH, Duffield SL, Stein JA, editors. Papers from the First Symposium on Neogene Dinoflagellate cyst biostratigraphy. College Station (TX): American Association of Stratigraphic Palynologists, Contribution Series 17: p. 47–58.
- Ehrenberg CG. 1837. Über das Massenverhältniss der jetzt lebenden Kiesel-Infusorien und über ein neues Infusorien-Conglomerat als Polierschiefer von Jastraba in Ungarn. Abhandlungen der Königlichen Akademie der Wissenschaften zu Berlin, aus dem Jahre 1836, Physikalische Klasse. 1:109–135.
- Eidvin T, Goll RM, Grogan P, Smelror M, Ulleberg K. 1998. The Pleistocene to Middle Eocene stratigraphy and geological evolution of the Western Barents Sea Continental Margin at Well Site 7316/5-1 (Bjornoya West area). Norsk Geologisk Tidsskrift. 78(1):99–123.
- Eisenack A. 1958. Mikroplankton aus dem norddeutschen Apt, nebst einigen Bemerkungen über fossile Dinoflagellaten. Neues Jahrbuch für Geologie und Paläontologie. Abhandlungen. 106:383–422.
- El-Bassiouni AE, Ayyad SN, El-Beialy SY. 1988. On the Eocene-Oligocene boundary in Alam El-Bueib. IX:Western Desert, Egypt. Rev Esp Micropaleontología. 20(1):59–70.
- El-Beialy SY. 1990. Palynology, palaeoecology, and dinocyst stratigraphy of the Oligocene through Pliocene succession in the Quantara-1 Well, Eastern Nile Delta, Egypt. J Afr Earth Sci. 11(3):291–307.
- Ellegaard M, Daugbjerg N, Rochon A, Lewis J, Harding IC. 2003. Morphologic and genetic (LSU rDNA) variation within Spiniferites/Gonyaulax (Dinophyceae), including the cyst-theca relationship of Spiniferites elongatus and phylogenetic analysis of the position of Spiniferites and Bitectatodinium within the Gonyaulacales. Phycologia. 42:151–164.
- Ellegaard M, Lewis J, Harding IC. 2002. Cyst-theca relationship, life cycle, and effects of temperature and salinity on the cyst morphology of Gonyaulax baltica sp. nov. (Dinophyceae) from the Baltica Sea area. J Phycol. 58:775–789.
- Eshet Y, Moshkovitz S, Habib D, Benjamini C, Magaritz M. 1992. Calcareous nannofossil and dinoflagellate stratigraphy across the Cretaceous/Tertiary boundary at Hor Hahar, Israel. Mar Micropaleontol. 18(3):199–228.
- Fechner GG, Mohr B. 1988. Early Eocene spores, pollen and microplancton assemblages from Fehmarn Island, North Germany. Tertiary Res. 9(1):147–168.
- Fensome RA, Williams GL. 2004. The Lentin and Williams Index of fossil dinoflagellates 2004 Edition. Am Assoc Stratigr Palynol Contrib Ser. 42:909 p.
- Fensome RA, Williams GL, Barss MS, Freeman JM, Hill JM. 1990. Acritarchs and fossil prasinophytes: an index to genera, species and infraspecific taxa. Am Assoc Stratigr Palynol Contrib Ser 25:771 p.
- Fiet N, Masure E. 2001. Les dinoflagellés albiens du bassin de Marches-Ombrie (Italie): proposition d'une biozonation pour le domaine téthysien. Cretaceous Res. 22(1):63–77.
- Firth JV. 1996. Upper Middle Eocene to Oligocene dinoflagellate biostratigraphy and assemblage variations in Hole 913B, Greenland Sea. Proc ODP Sci Res. 151:203–242.
- Foucher J-C. 1974. Microfossiles des silex du Turonien suptrieur de Ruyaulcourt (Pas-de-Calais). Ann Paléontol Invert. 60:113–164.
- Foucher J-C. 1975. Dinoflagellés et acritarches des silex crétacés du Bassin de Paris: une synthèse stratigraphique. Ann Sci Univ Reims Assoc Rég pour l’étude Recherche Sci. 13(1):8–10.
- Frederiksen NO. 1969. Stratigraphy and palynology of the Jackson Stage (Upper Eocene), and adjacent strata of Mississippi and Western Alabama [Unpublished Ph.D. Thesis]. University of Wisconsin; 355 p.
- Gedl P. 2005. Late Eocene – early Oligocene organic-walled dinoflagellate cysts from Folusz, Magura Nappe, Polish Carpathians. Acta Palaeobot. 45(1):27–83.
- Gedl P, Leszczynski S. 2005. Palynology of the Eocene-Oligocene Transition in the Marginal Zone of the Magura Nappe at Folusz (Western Carpathians, Poland). Geol Carpathica (Geologicky Zbornik) 56(2):155–167.
- Gitmez GU, Ertug K. 1999. Dinoflagellate cysts and acritarchs from the Jurassic-Cretaceous boundary, Northwest Anatolia, Turkey. Micropaleontology. 45(1):69–98.
- Gocht H. 1959. Mikroplankton aus dem nordwestdeutschen Neokom (Teil II). Paläeontologische Zeitschrift. 33(1):50–89.
- Grill SC, Quattrocchio ME. 1996. Fluctuaciones eustaticas durante el Holoceno a partir del regis de paleomicroplancton; Arroyo Napostá Grande, sur de la Provincia de Buenos Aires. Ameghiniana. 33(4):435–442.
- Guerrera F, Coccloni R, Corradini D, Bertoldi R. 1985. Caratteristiche lito-sedimentologiche e micropaleontologiche (foraminiferi, dinoflagellafi, pollini e spore) di successioni tripolacee plioceniche del bacino di Caltanissetta. Boll della Soc Geol Ital. 103:629–660.
- Guy-Ohlson D. 1986. Jurassic palynology of the Vilhelmsfalt bore no.1, Scania, Sweden: Toarcian-Aalenian. Stockholm: Swedish Museum of Natural History, Section of Palaeobotany, 127 p.
- Hansen JM. 1977. Dinoflagellate stratigraphy and echinoid Dinoflagellate biostratigraphy and depositional environdistribution in Upper Maastrichtian and Danian deposits ment of the type Maastrichtian (Late Cretaceous, ENCI from Denmark. Bulletin of the Geological Society of Den- Quarry, The Netherlands). Mar Micropaleontology. 31(26):1–26.
- Hardenbol J, Thierry J, Farley MB, Jacquin T, De Graciansky P-C, Vail PR. 1998. Mesozoic and Cenozoic sequence chronostratigraphic framework of European basins. In De Graciansky P-C, Hardenbol J, Jacquin T, Vail PR, editors. Mesozoic and Cenozoic Sequence Stratigraphy of European Basins. Vol. 60. Tulsa: SEPM Special Publication, Chart 5.
- Harding IC, Smith GA, Riding JB, Wimbledon WAP. 2011. Inter-regional correlation of Jurassic/Cretaceous boundary strata based on the Tithonian-Valanginian dinoflagellate cyst biostratigraphy of the Volga Basin, western Russia. Rev Palaeobot Palynol. 167:82–116.
- Harland R, Reid PC, Dobell P, Norris G. 1980. Recent and sub-recent dinoflagellate cysts from the Beaufort Sea, Canadian Arctic. Grana. 19(3):211–225.
- Harland R. 1977. Dinoflagellate cysts from the Bearpaw formation (upper campanian to Maastrichtian) of Montana. Palaeontology. 20(1):179–193.
- Harland R. 1978. Quaternary and Neogene dinoflagellate cysts. In: Thusu B, editor. Distribution of biostratigraphically diagnostic dinoflagellate cysts and miospores from the Northwest European continental shelf and adjacent areas. Vol. 100. College Station (TX): Continental Shelf Institute; p. 7–17.
- Harland R. 1979. Dinoflagellate biostratigraphy of Neogene and Quarternary sediments at holes 400/400A in the Bay of Biscay (Deep Sea Drilling Project Leg 48). In: Montadert L, Roberts DG, editors. Initial Reports of the DSDP 48: p. 531–545.
- Harland R. 1983. Distribution maps of recent dinoflagellate cysts in bottom sediments from the North Atlantic Ocean and adjacent seas. Palaeontology. 26(2):321–387.
- Head MJ, Nøhr-Hansen H. 1999. The extant thermophilic Tectatodinium pellitum (al. Tectatodinium rugulatum) from the Danian of Denmark. J Paleontol. 73(4):577–579.
- Head MJ, Westphal H. 1999. Palynology and Paleoenvironments of a Pliocene Carbonate Platform: The Clino Core, Bahamas. J Paleontol. 73(1):1–25.
- Head MJ. 1996. Chapter 30. Modern dinoflagellate cysts and their biological affinities. In: Jansonius J, McGregor DC, editors. Palynology: principles and applications. Dallas: American Association of Stratigraphic Palynologists Foundation; 3: p. 1197–1248.
- Head MJ. 1997. Thermophilic dinoflagellate assemblages from the Mid-Pliocene of eastern England. J Paleontol. 71(2):165–193.
- Head MJ. 2007. Last Interglacial (Eemian) hydrographic conditions in the southwestern Baltic Sea based on dinoflagellate cysts from Ristinge Klint, Denmark. Geol Mag. 144(6):987–1013.
- Heilmann-Clausen C. 1987. Lower Cretaceous dinoflagellate biostratigraphy in the Danish Central Trough. Danmarks Geologiske Undersøgelse. 17:1–89.
- Helenes J. 1986. Some variations in the paratabulation of gonyaulacoid dinoflagellates. Palynology. 10(1):73–110.
- Hultberg SU, Malmgren BA. 1995. Evolutionary patterns in the dinoflagellate cyst genus Spiniferites (Cretaceous through Quaternary): relationships to sea-level changes. Hist Biol. 10:341–357.
- ICS. 2016. International Chronostratigraphic Chart, v2016/04. International Commission on Stratigraphy. http://www.stratigraphy.org/ICSchart/ChronostratChart2016-04.jpg.
- Islam MA. 1984. A study of early Eocene palaeoenvironments in the Isle of Sheppey as determined from microplankton assemblage composition. Tertiary Res. 6:11–21.
- Jan du Chêne RE. 1988. Étude systématique des kystes de dinoflagellés de la Formation des Madeleines (Danien du Sénégal). Cahiers de micropaléontologie, Nouvelle série. 2:147–174.
- Kar RK. 1985. The fossil floras of Kachchh. IV– tertiary palynostratigraphy. Palaeobotanist. 34:1–280.
- Kirsch KH, Below R. 1995. Quantitative Untersuchung der Dinoflagellatenverteilung in den hell/dunkel-Rhythmiten des Hauterive-Barrême-Grenzbereichs im Niedersächsischen Becken (Norddeutschland) am Beispiel des Profils der Tongrube Otto Gott bei Sarstedt. Paläeontographica Ab B. 236(4):105–146.
- Klumpp B. 1953. Beitrag zur Kenntnis der Mikrofossilien des mittleren und oberen Eozän. Palaeontographica. Abteilung A. 103:377–406.
- Kofoid CA. 1911. Dinoflagellata of the San Diego region, IV. The genus Gonyaulax, with notes on its skeletal morphology and a discussion of its generic and specific characters. University of California Publications in Zoology. 8:187–286.
- Koreneva EV, Kartashova GG. 1978. Palynological study of samples from holes 379A, 380A, Leg 42B. Initial Rep DSDP. 42(2):951–992.
- Köthe A. 1990. Paleogene dinoflagellates from Northwest Germany; biostratigraphy and paleoenvironment. Geol Jahrbuch Reihe A. 118:3–111.
- Kuhlmann G. 2004. Hoge resolutie stratigrafie en paleo-milieu veranderingen in de zuidelijke Noordzee tijdens het Neogeen Een geïntegreerde studie van Laat Cenozoische marine afzettingen in het noordelijke gedeelte van het Nederlandse offshore gebied (met een samenvatting in het Nederlands). Geologica Ultraiectina, Mededelingen van de Faculteit Geowetenschappen Universiteit Utrecht. 245:1–205.
- Kumar A. 1980. Early Cretaceous dinocysts and acritarchs from the Krishna-Godavari Basin, Andhra Pradesh, India. International Palynological Conference, 5th, Abstracts, 210. Cambridge.
- Lebedeva NK, Nikitenko BL. 1999. Dinoflagellate cysts and microforaminifera of the Lower Cretaceous Yatria River section, Subarctic Ural, northwest Siberia (Russia). Biostratigraphy, palaeoenvironmental and palaeogeographic discussion. Grana. 38(2–3):134–143.
- Lentin JK, Williams GL. 1973. Fossil dinoflagellates: index to genera and species. Geological Survey of Canada. Paper 73–42; p. 176.
- Lewis J, Rochon A, Ellegaard M, Mudie P, Harding I. 2001. The cyst–theca relationship of Bitectatodinium tepikiense (Dinophyceae). Eur J Phycol. 36(2):137–146.
- Lewis J, Rochon A, Harding IC. 1999. Preliminary observations of cyst-theca relationships in Spiniferites ramosus and Spiniferites membranaceus (Dinophyceae). Grana. 38:113–124.
- Limoges A, Londeix L, Mertens KN, Rochon A, Pospelova V, de Vernal A, del Carmen Cuéllar T. 2018. Towards an identification key for Pliocene and Quaternary Spiniferites taxa bearing intergonal processes based on observations from estuarine and coastal environments. Palynology. 42 Suppl:1 (this volume).
- Lister JK, Batten DJ. 1988. Stratigraphic and palaeoenvironment distribution of Early Cretaceous dinoflagellate cysts in the Hurlands Farm Borehole, West Sussex, England. Paläeontographica Abt B. 210(1):9–89.
- Liu D, Shi Y, Di B, Sun Q, Wang Y, Dong Z, Shao H. 2012. The impact of different pollution sources on modern dinoflagellate cysts in Sishili Bay, Yellow Sea, China. Mar Micropaleontology. 84–85:1–13.
- Londeix L, Benzakour M, de Vernal A, Turon J-L, Suc J-P. 1999. Late Neogene dinoflagellate cyst assemblages from the Strait of Sicily, Central Mediterranean Sea: paleoecological and biostratigraphical implications. In: Wrenn JH, Suc J-P Suc, Leroy SAG, editors. The Pliocene: time of change. Dallas: American Association of Stratigraphic Palynologists Foundation; p. 65–91.
- Londeix L, Herreyre Y, Turon J-L, Fletcher W. 2009. Last glacial to holocene hydrology of the Marmara Sea as inferred by the dinoflagellate cysts record. Rev Palaeobot Palynol. 158:52–71.
- Londeix L, Jan du Chêne R. 1998. Stratigraphie des kystes de dinoflagellés du Burdigalien stratotypique bordelais (France). Geobios. 30(3):283–294.
- Londeix L, Lopes S. 2014. Les dinokystes de l’Aquitanien stratotypique: biostratigraphie et quantification de paramètres environnementaux. In: Londeix L (coord.), editor. Stratotype aquitanien. Paris: Muséum national d’Histoire naturelle, Biotope; 416 p. (Patrimoine géologique, 5).
- Londeix L, Zonneveld K, Masure E. 2018. Operational taxonomy of Quaternary Spiniferites sensu lato and identification. Palynology. 42 Suppl:1 (this volume).
- Londeix L. 1990. La distribution des kystes de dinoflagellés dans les sédiments hémipélagiques, (Ardèche), et pélagiques, (Arc de Castellane, S.E. de. la France), en Domaine Vocontien, du Valanginien terminal au Barrémien inferieur. Biostratigraphie et relations avec la stratigraphie séquentielle. Bordeaux 1 University [PhD thesis] 478; 606 p.
- Louwye S, Head MJ, De Schepper S. 2004. Dinoflagellate cyst stratigraphy and palaeoecology of the Pliocene in northern Belgium, southern North Sea Basin. Geol Mag. 141(3):353–378.
- Maier D. 1959. Planktonuntersuchungen in tertiären und quartären marinen Sedimenten. Ein Beitrag zur Systematik, Stratigraphie und Ökologie der Coccolithophorideen, Dinoflagellaten und Hystrichosphaerideen vom Oligozän bis zum Pleistozän. Neues Jahrbuch für Geologie und Paläontologie. Abhandlungen. 107:278–340.
- Mangin S. 2002. Distribution actuelle des kystes de dinoflagellés en Méditerranée occidentale et application aux fonctions de transfert. Diplôme d’Etudes Approfondies dissertation. Bordeaux: Bordeaux 1 University; 34 p.
- Manum SB, Boulter MC, Gunnarsdottir H, Rangnes K, Scholze A. 1989. Eocene to Miocene palynology of the Norwegian Sea (ODP Leg 104). In: Eldholm O, et al. editors. Proceedings Ocean Drilling Program, College Station (TX): Scientific Results 104: p. 611–639.
- Mao S. 1989. V. Dinoflagellata. In: Hao Y, Mao S, Ruan P, Su X, Sun S, Wang Z, Yin J, Zheng H, editors. Quaternary microbiotas and their geological significance from northern Xisha Trench of South China Sea. Wuhan, China: China University of Geosciences Press; p. 132–147.
- Marret F, de Vernal A, Pedersen TF, McDonald D. 2001. Middle Pleistocene to Holocene palynostratigraphy of Ocean Drilling Program Site 887 in the Gulf of Alaska, northeastern North Pacific. Can J Earth Sci. 38(3):373–386.
- Martini E. 1971. Standard tertiary and quaternary calcareous nannoplankton zonation. In: Farinacci A, editor. Proceeding 2nd international conference on Planktonic Microfossils; Roma, 2:p. 739–785.
- Masure E. 1988. Le genre Maghrebinia Below, 1981, nouvelle interprétation et amendement. Bulletin des Centres de Recherches Exploration-Production Elf-Aquitaine. 12(1):361–381.
- Matsuoka K. 1983. Late Cenozoic dinoflagellates and acritarchs in the Niigata district, Central Japan. Palaeontographica Abt B. 187(1–3):89–154.
- Matsuoka K, Bujak JP, Shimazaki T. 1987. Late Cenozoic dinoflagellate cyst biostratigraphy from the west coast of northern Japan. Micropaleontology. 33:214–229.
- McCarthy FMG, Katz ME, Kotthoff U, Browning JV, Miller KG, Zanatta R, Williams RH, Drljepan M, Hesselbo SP, Bjerrum CJ, et al. 2013. Sea-level control of New Jersey margin architecture: palynological evidence from Integrated Ocean Drilling Program Expedition 313. Geosphere. 9(6):1457–1487.
- McCarthy FMG, Mudie PJ. 1996. Palynology and dinocyst biostratigraphy of Upper Cenozoic sediments from ODP Leg 149 Sites 898 and 90tl, Iberian Abyssal Plain. Proc ODP Sci Res. 149:241–265.
- McMinn A. 1993. Neogene dinoflagellate cyst biostratigraphy from Sites 815 and 823, Leg 133, Northeastern Australian Margin. Proc ODP Sci Res. 133:97–105.
- Mertens KN, Van Nieuwenhove N, Gurdebeke PR, Aydin H, Bogus K, Bringué M, Dale B, De Schepper S, de Vernal A, Ellegaard M, et al. 2018. Summary of round table discussions about Spiniferites and Achomosphaera occuring in Pliocene to modern sediments. Palynology. 42 Suppl:1.
- Monteil E. 1992. Kystes de dinoflagellés index (Tithonique-Valanginien) du sud-est de la France. Proposition d’une nouvelle zonation palynologique. Revue de Paléobiologie. 11(1):299–306.
- Monteil E. 1993. Dinoflagellate cyst biozonation of the Tithonian and Berriasian of south-east France. Correlation with the sequence stratigraphy. Bulletin des Centres de Recherches Exploration-Production Elf-Aquitaine. 17:249–273.
- Morzadec-Kerfourn MT. 1979. Etude des organismes les kystes de dinoflagelles. In: Burollet PF, Clairefond P, Winnock E, editors. La mer Pelagienne. Géologie Méditerranéenne. 6(1):221–248.
- Moshkovitz S, Habib D. 1993. Calcareous nannofossil and dinoflagellate stratigraphy of the Cretaceous-Tertiary boundary, Alabama and Georgia. Micropaleontology. 39(2):167–191.
- Mudie PJ, Rochon A, Aksu AE, Gillespie H. 2002. Dinoflagellate cysts, freshwater algae and fungal spores as salinity indicators in Late Quaternary cores from Marmara and Black Seas. Mar Geol. 190(1–2):203–231.
- Mudie PJ. 1986. Palynology and dinoflagellate biostratigraphy of Deep Sea Drilling Project Leg 94, Sites 607 and 611, North Atlantic Ocean. Deep Sea Drilling Proj Initial Rep. 94:785–812.
- Mudie PJ. 1989. Palynology and dinocyst biostratigraphy of the Late Miocene to Pleistocene, Norwegian Sea; ODP Leg 104, Sites 642 to 644. Proc ODP Sci Res. 104:587–610.
- Mudie, PJ, Marret F, Aksu AE, Hiscott RN, Gillespie H. 2007. Palynological evidence for climatic change, anthropogenic activity and outflow of Black Sea Water during the Late Pleistocene and Holocene: centennial- to decadal-scale records from the Black and Marmara Seas. Quaternary Int. 167–168:73–90.
- Oboh-Ikuenobe FE, Yepes O, Greggs JM. 1998. Palynostratigraphy, palynofacies, and thermal maturation of Cretaceous-Paleocene sediments from the Côte d’Ivoire-Ghana Transform Margin. Proc Ocean Drilling Prog Sci Res. 159:277–318.
- Ogg JG, Ogg G. 2008a. Pliocene/Pleistocene. (0–6 Ma Time-Slice). Update to Geological Time Scale 2004 (Gradstein, F.M., Ogg, J.G., Smith, A.G., et al., Cambridge Univ. Press) and The Concise Geologic Time Scale (Ogg, J.G., Ogg, G., Gradstein, F.M., 2008). https://engineering.purdue.edu/Stratigraphy/charts/Timeslices/0_Plio_Pleist.pdf.
- Ogg JG, Ogg G. 2008b. Paleogene. (33–66 Ma time-slice). Update to Geological Time Scale 2004 (Gradstein, F.M., Ogg, J.G., Smith, A.G., et al., Cambridge Univ. Press) and The Concise Geologic Time Scale (Ogg, J.G., Ogg, G., Gradstein, F.M., 2008). https://engineering.purdue.edu/Stratigraphy/charts/Timeslices/2_Paleogene.pdf.
- Ogg JG, Ogg G. 2008c. Late Jurassic. (139–169 Ma time-slice). Update to Geological Time Scale 2004 (Gradstein, F.M., Ogg, J.G., Smith, A.G., et al., Cambridge Univ. Press) and The Concise Geologic Time Scale (Ogg, J.G., Ogg, G., Gradstein, F.M., 2008). https://engineering.purdue.edu/Stratigraphy/charts/Timeslices/5_JurCret.pdf.
- Palynodata Inc. & White JM. 2008. Palynodata Datafile: 2006 version, with Introduction by J.M. White. Geological Survey of Canada Open File 5793. http://paleobotany.ru/index.php?id =25.
- Pestchevitskaya E, Lebedova N, Ryabokon A. 2011. Uppermost Jurassic and lowermost Cretaceous dinocyst successions of Siberia, the Subarctic Urals and Russian Platform and their interregional correlation. Geol Carpathica. 62(3):189–202.
- Piasecki S. 1980. Dinoflagellate cyst stratigraphy of the Miocene Hodde and Gram Formations, Denmark. Geol Soc Denmark Bull. 29:53–76.
- Pouchet G. 1883. Contribution á l’étude des cilioflagellés. Journal of Anatomy and Physiology. 19:399–455.
- Poulsen NE, Manum SB, Williams GL, Ellegaards M. 1996. Tertiary dinoflagellate biostratigraphy of Sites 907, 908 and 909 in the Norwegian-Greenland Sea. In: Thiede J, Myhre AM, Firth JV, Johnson GL, Ruddiman WF, editors. Proceedings of the Ocean Drilling Program. Vol. 151. College Station (TX): Scientific Results; p. 255–287.
- Powell AJ. 1986a. A dinoflagellate cyst biozonation for the Late Oligocene to Middle Miocene succession of the Langhe Region, northwest Italy. Am Assoc Stratigraphic Palynol Contrib Ser. 17:105–127.
- Powell AJ. 1986b. The stratigraphic distribution of Late Miocene dinoflagellate cysts from the Castellanian superstage stratotype, Northwest Italy. Am Assoc Stratigraphic Palynol Contrib Ser. 17:129–149.
- Powell AJ. 1988. A preliminary investigation into the Neogene dinoflagellate cyst biostratigraphy of the British southwestern approaches. Bulletin des Centres de Recherche Exploration-Production Elf-Aquitaine. 12(1):277–311.
- Powell AJ, editor. 1992. A stratigraphic index of dinoflagellate cysts. London: British Micropalaeontological Society Publications Series; 290 p.
- Powell AJ, Brinkhuis H, Bujak JP. 1996. Upper Paleocene-Lower Eocene dinoflagellate cyst sequence biostratigrapahy of Southeast England. In: Knox RWO, et al. editors. Correlation of the early Paleogene in Northwest Europe. Vol. 101. London: Geological Society Special Publication; p. 145–183.
- Price AM, Pospelova V. 2014. Spiniferites multisphaerus, a new dinoflagellate cyst from the Late Quaternary of the Guaymas Basin, Gulf of California, Mexico. Palynology. 38:101–116.
- Radi T, de Vernal A. 2004. Dinocyst distribution in surface sediments from the northeastern Pacific margin (40–60°N) in relation to hydrographic conditions, productivity and upwelling. Rev Palaeobot Palynol. 128:169–193.
- Reid PC. 1974. Gonyaulacacean dinoflagellate cysts from the British Isles. Nova Hedwigia Beihefte. 25:579–637.
- Riley LA, Fenton JPG. 1984. Palynostratigraphy of the Berriasian to Cenomanian sequence at Deep Sea Drilling Project Site 535, Leg 77, southeastern Gulf of Mexico. Deep Sea Drilling Proj Initial Rep. 77:675–690.
- Rochon A, de Vernal A, Turon J-L, Matthiessen J, Head MJ. 1999. Distribution of Recent dinoflagellate cysts in surface sediments from the North Atlantic Ocean and adjacent areas in relation to sea-surface parameters. Am Assoc Stratigr Palynol Contrib Ser. 35:146 p.
- Rochon A, Lewis J, Ellegaard M, Harding IC. 2009. The Gonyaulax spinifera (Dinophyceae) “complex”: Perpetuating the paradox? Rev Palaeobot Palynol. 155:52–60.
- Rossignol M. 1962. Analyse pollinique de sédiments marins quaternaires en Israël II. — Sédiments pleistocénes. Pollen et Spores. 4:121–148.
- Rossignol M. 1964. Hystrichosphères du Quaternaire en Méditerranée orientale, dans les sediments pléistocènes et les boues marines actuelles. Revue de Micropaléontologie. 7(2):83–99.
- Sarjeant WAS. 1960. Microplankton from the Corallian rocks of Yorkshire. Proc Yorkshire Geol Soc. 32(4):389–408.
- Sarjeant WAS. 1962. Microplankton from the Ampthill Clay of Melton, South Yorkshire. Palaeontology. 5(3):478–497.
- Sarjeant WAS. 1970. The genus Spiniferites Mantell, 1850 (Dinophyceae). Grana. 10:74–78.
- Sarjeant WAS. 1979. Middle and Upper Jurassic dinoflagellate cysts: the world excluding Noth America. Am Assoc Stratigr Palynol Contrib Ser. 5B:133–157.
- Sarjeant WAS. 1983. A restudy of some Dinoflagellate cyst holotypes in the University of Kiel Collections. IV. The Oligocene and Miocene holotypes of Dorothea Maier (1959). Meyniana. 35:85–137.
- Stoker MS, Leslie AB, Scott WD, Briden JC, Hine NM, Harland R, Wilkinson IP, Evans D, Ardus DA. 1994. A record of Late Cenozoic stratigraphy, sedimentation and climate change from the Hebrides Slope, northeast Atlantic Ocean. J Geol Soc Lond. 151(2):235–249.
- Stover LE, Brinkhuis H, Damassa SP, De Verteuil L, Helby RJ, Monteil E, Partridge AD, Powell AJ, Riding JB, Smelror M, et al. 1996. Chapter 19. Mesozoic‐Tertiary dinoflagellates, acritarchs and prasinophytes. In: Jansonius J, McGregor DC, editors. Palynology: principles and applications. Vol. 2. Dallas: American Association of Stratigraphic Palynologists Foundation; p. 641–750.
- Stover LE, Evitt WR. 1978. Analyses of pre-Pleistocene organic-walled dinoflagellates. Stanford University Publications, Geological Sciences. 15:300 p.
- Strauss C. 1993. Taxonomie und biostratigrafie des marinen mikroplanktons mit organischer wandung im Oligo-Miozan Ostdeutschlands. Disseration Bergakademie Freiberg, 110 p.
- Suc J-P, Violanti D, Londeix L, Poumot C, Robert C, Clauzon G, Gautier F, Turon J-L, Ferrier J, Chikhi H, et al. 1995. Evolution of the Messinian Mediterranean environments; the Tripoli Formation at Capodarso (Sicily, Italy). Rev Palaeobot Palynol. 87(1):51–79.
- Sun XK, Song ZC. 1992. Quaternary dinoflagellates from arenaceous dolomite in Hainan Island. (in Chinese with English summary). Acta Micropalaeontologica Sinica. 9(1):45–52.
- Tea-Yassia J, Digbehib ZB, Yaoa KR, Glohia BV. 1999. Étude de quelques palynomorphes du crétace supérieur du bassin offshore de Côte d’Ivoire: implications biostratigraphiques et paléoenvironnementales. J Afr Earth Sci. 29(4):783–798.
- Thusu B, van der Eem JGLA, El-Mehdawi A, Bu-Argoub F. 1989. Jurassic-Early Cretaceous palynostratigraphy in northeast Libya. In Arnauti E, et al., editors. The subsurface palynostratigraphy of Northeast Libya. Libya: Special Publication of the University of Garryounis; p. 171–213.
- Thusu O, Vigran JB. 1985. Middle – Late Jurassic (Late Bathonian – Tithonian) Palynomorphs. J Micropalaeontol. 4(1):113–130.
- Versteegh GJM, Zonneveld KAF. 1994. Determination of palaeoecological preferences of dinoflagellates by applying detrended and canonical correspondence analysis to Late Pliocene dinoflagellate cyst assemblages of the South Italian Singa section. Rev Palaeobot Palynol. 84(1):181–199.
- Wall D, Dale B, Harada K. 1973. Descriptions of new fossil dinoflagellates from the Late Quaternary of the Black Sea. Micropaleontology. 19:18–31.
- Wall D, Dale B. 1970. Living hystrichosphaerid dinoflagellate spores from Bermuda and Puerto Rico. Micropaleontology. 16:47–58.
- Wall D. 1967. Fossil microplankton in deep-sea cores from the Caribbean Sea. Palaeontology. 10(1):95–123.
- Warny S. 1999. Mio-Pliocene palynology of the Gibraltar Arc: a new perspective on the Messinian Salinity Crisis [Unpublished Ph.D. dissertation]. Louvain (BE): Université Catholique de Louvain, Faculté des Sciences; 347 p.
- Williams GL, Brinkhuis H, Bujak J, Damassa S, Hochuli PA, De Verteuil L, Zevenboom D. 1998. Cenozoic Biochronostratigraphy – Dinoflagellate cysts. In: De Graciansky PC, Hardenbol J, Jacquin T, Vail PR, editors. Mesozoic and Cenozoic Sequence Chronostratigraphic Framework of European Basins. Mesozoic and Cenozoic Sequence Stratigraphy of European Basins. Vol. 60. Tulsa: SEPM Special Publication.
- Williams GL, Bujak JP. 1985. 18. Mesozoic and Cenozoic dinoflagellates. In: Bolli HM, Saunders JB, Perch‐Nielsen K, editors. Plankton stratigraphy. Cambridge: Cambridge University Press; p. 847–964. (Cambridge Earth Science Series).
- Williams GL, Stover LE, Kidson EJ. 1993. Morphology and stratigraphic ranges of selected Mesozoic-Cenozoic dinoflagellate taxa in the northern hemisphere. Geological Survey of Canada paper 92–10, Ottawa (CA); 137 p.
- Wrenn JH, Kokinos JP. 1986. Preliminary comments on Miocene through Pleistocene dinoflagellate cysts from De Soto Canyon, Gulf of Mexico. College Station (TX): American Association of Stratigraphic Palynologists, Contributions Series 17:169–225.
- Yu J, Zhang W. 1980. Upper Cretaceous dinoflagellate cysts and acritarchs of Western Xinjiang. (Sinkiang Uighur). Bull Chinese Acad Geol Sci. 2(1):93–119. (in Chinese with English summary).
- Yun H. 1981. Dinoflagellaten aus der Oberkreide (Santon) von Westfalen. Palaeontographica, Abteilung B. 177:1–89.
- Yun H, Byun H, Park YA. 2000. Paleoenvironments interpretation based on microfossil assemblages from intertidal sediments of the Haenam Bay. J Paleontol Soc Korea. 16(2):123–144.
- Zevenboom D. 1996. Late Oligocene – Early Miocene dinoflagellate cysts from the Lemm Carrosio Section, (northwest Italy): Biostratigraphy and palaeoenvironmental interpretation. G Geol Ser 3A. 58(1):81–93.
- Zhao YY, Morzadec-Kerfourn MT. 1994. Nouveaux kystes de dinoflagellés: Spiniferites pacificus nov. sp. et Pentadinium netangei nov. sp. du Pléistocène du nord-ouest Pacifique. Geobios. 27(3):261–269.
- Zhu H. 2000. On the dynamics of Permian phytoprovincial succession of the Tarim Plate. In: Song Z, editor. Palynofloras and Palynomorphs of China. Hefei: Press of University of Science and Technology of China; p. 28–40.
- Zonneveld KAF, Marret F, Versteegh GJM, Bonnet S, Bouimetarhan I, Crouch E, de Vernal A, Elshanawany R, Edwards L, Esper O, et al. 2013. Atlas of modern dinoflagellate cyst distribution based on 2405 datapoints. Rev Palaeobot Palynol. 191:1–197.