Abstract
This study documents the detailed palynology of the Bridge Creek Member, Greenhorn Limestone Formation, at the Rock Canyon anticline outcrop, west of Pueblo, Colorado, USA. The section is the formally ratified Global Boundary Stratotype Section and Point (GSSP) for the base of Turonian Stage and corresponding Lower Turonian Substage, and the only proposed GSSP for the base of the Middle Turonian Substage. The authors’ previously published palynological data from the underlying Cenomanian strata in the nearby US Geological Survey (USGS) Portland-1 core, and from cores and an outcrop of Cenomanian, Turonian and earliest Coniacian age in SW Texas, are reviewed for biostratigraphy. Dinoflagellate cyst ranges and events in both areas are calibrated against other published bio- and chemostratigraphical (carbon isotope) data and an astronomical age model. A regional dinoflagellate cyst zonation is proposed, adapted from a Central and Northern European scheme, comprising three zones and four subzones. Comparison with vintage published palynological data from other states, including Arizona, Kansas, Utah, Wyoming and Montana, indicates that the zonation is probably applicable to marine sections throughout the Cretaceous Western Interior Seaway of the USA. The new palynological data from around the Lower–Middle Turonian boundary at Pueblo support its proposal as a GSSP.
1. Introduction
1.1. Background and objectives
Dinoflagellate cysts are widely used in biostratigraphical studies of the Upper Cretaceous Series. They have been used to guide conventional hydrocarbon exploration and development drilling in many regions, including Wyoming, USA (the Frontier Formation and Greenhorn Formation; Burgess Citation1971) and Northern Europe, in both the northerly argillaceous Shetland Group facies (Costa & Davey Citation1992) and southerly calcareous Chalk Group facies (Schiøler & Wilson Citation1993). More recently, they have been proposed for use in guiding unconventional shale oil/shale gas reservoir drilling of the Eagle Ford Group in Texas, USA (Eldrett et al. Citation2015a; Dodsworth Citation2016). It has been known for some time that several dinoflagellate cysts have widespread range bases and tops in the Cenomanian and Turonian stages and that they are therefore of utility in identifying their substages (Clarke & Verdier Citation1967; Burgess Citation1971; Foucher Citation1979, Citation1980, Citation1981, Citation1983; Williams & Bujak Citation1985; Williams et al. Citation2004).
The overall objectives of the Subcommission on Cretaceous Stratigraphy (SCS) which fit within the International Union of Geological Sciences’ policy are: to establish a standard global stratigraphical subdivision and nomenclature for the Cretaceous; to produce a stratigraphical table displaying agreed subdivisions to stage and substage level, marking boundaries that are defined by a Global Boundary Stratotype Section and Point (GSSP; Remane et al. Citation1996; http://cretaceous.stratigraphy.org). Following the formal ratification of GSSPs for some of the Upper Cretaceous stage boundaries, i.e. the bases of the Cenomanian Stage (Kennedy et al. Citation2004), Turonian Stage (Kennedy et al. Citation2005), Santonian Stage (Lamolda et al. Citation2014) and Maastrichtian Stage (Odin & Lamaurelle Citation2001), it is appropriate to undertake detailed palynological calibrations of these global reference sections. Additionally, palynological studies of proposed GSSPs, such as a report on dinoflagellate cysts across a candidate base of the Coniacian Stage GSSP in Poland (Olde et al. Citation2016), strengthen the case for their selection by the SCS.
Upper Cretaceous stages are divided into lower, middle and upper substages. The base of a lower substage is defined by the same GSSP as the corresponding base of stage boundary. However, middle and upper substage boundaries have, to date, received less attention and none are yet formally ratified. One of the best documented is the proposed GSSP for the base of the Middle Turonian Substage (Bengtson et al. Citation1996; Kennedy et al. Citation2000), at the same locality as the formally ratified GSSP for the base of the Turonian Stage/Lower Turonian Substage (Kennedy et al. Citation2005), Rock Canyon anticline outcrop, west of Pueblo, Colorado, USA (abbreviated to ‘Pueblo’ in the text; ; ) and the nearby USGS core Portland-1 (Sageman et al. Citation2006; ). In this paper, we follow the SCS’s convention of using upper case for a substage that has been formally defined or proposed, e.g. Lower and Middle Turonian. In the case of the Cenomanian Stage, a threefold subdivision has been agreed by the SCS (Tröger et al. Citation1996). For the base of the Upper Cenomanian Substage, Tröger et al. (Citation1996) recommended southern France as a suitable region for a type section, though a proposed site was not selected by them. The Middle–Upper Cenomanian boundary is commonly marked at the level of replacement of Acanthoceras ammonites by the genus Calycoceras (Hancock Citation1991), which can also be correlated with carbon isotope criteria (Kennedy & Gale Citation2006). Age estimates of stage and substage boundaries as calculated by Ogg et al. (Citation2016) are applied in this paper.
Figure 1. Turonian paleogeographical reconstruction with site locations: grey shaded area, landmass; dotted line, palaeo-shelf; CLIP, Caribbean Large Igneous Province; HALIP, High-Arctic Large Igneous Province. MT, Montana. WY, Wyoming. Colorado: Global Boundary Stratotype Section and Point, GSSP, for the base of the Turonian Stage and proposed GSSP for the base of the Middle Turonian Substage (Kennedy et al. Citation2000, Citation2005), Rock Canyon anticline, west of Pueblo (38°20.36’N, 104°31’W; ); USGS Portland-1 core (38°22.36’N, 105°01.18’W). SW Texas localities (): Morocco: Tarfaya, on the eastern margin of the proto-Atlantic Ocean.
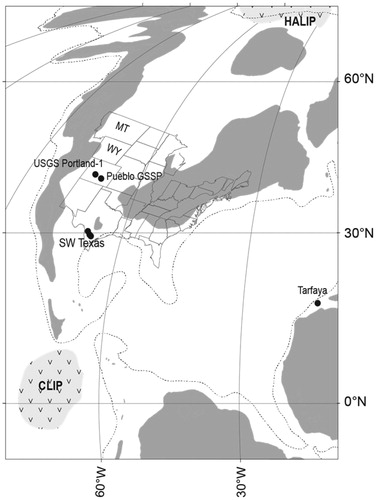
Figure 2. Sketch maps of locations. (a) The area around the Pueblo Reservoir (based on Scott Citation1964), including the Global Stratotype Standard Section on the north side of the reservoir, and the points for the base of the Turonian Stage, Bed 86, and the proposed Middle Turonian Substage, Bed 120 (Kennedy et al. Citation2000, Citation2005). Grey shading shows outcrop of Greenhorn Limestone Formation. (b) SW Texas map showing outcrop and core locations (black stars): Lozier Canyon outcrop (29°53.37’N, 101°48.22’W); Innes-1 core (29°49.51’N, 101°37.34’W); Iona-1 core (29°13.51’N, 100°44.49’W); Well ‘X’ core. Grey shading shows outcrop of Eagle Ford Group (from Eldrett et al. Citation2017).
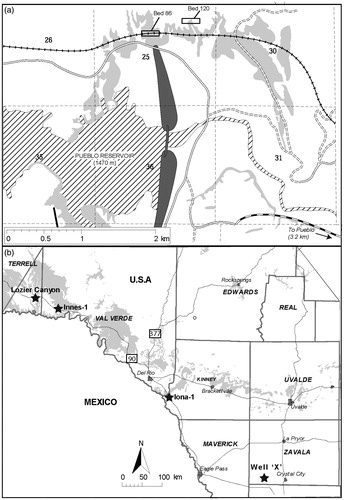
Figure 3. Lithological succession in the Bridge Creek Member of the Greenhorn Limestone Formation on the north side of the Pueblo Reservoir State Recreation area: (a) Depth below/height above base of Bridge Creek Member. (b) Chronostratigraphy. (c) Lithostratigraphy. (d) Lithology. (e) δ13Corg, data from Bowman and Bralower (Citation2005). (f) Ammonite zones after Kennedy and Cobban (Citation1991), Cobban (Citation1993) and Kennedy et al. (Citation2000). Note that the Watinoceras devonense, Pseudaspidoceras flexuosum and Vascoceras birchbyi units are often treated as subzones of a Watinoceras coloradoense Zone or Watinoceras spp. Zone in published literature. (g) Inoceramid bivalve zonation by Walaszczyk and Cobban in Kennedy et al. (Citation2000). (h) Planktonic foraminifera from Caron et al. (2005) and Keller and Pardo (Citation2004). (i) Subzones from Keller and Pardo (Citation2004). There is a difference between Keller and Pardo (Citation2004) and Caron et al. (2005) on the base of Helvetoglobotruncana helvetica. This difference is marked by the vertical line in the figure. (j) Nannofossil zones are from Bralower (Citation1988) and Bralower and Bergen (Citation1998). (k)–(l) Dinoflagellate cyst zones and subzones are from this paper.
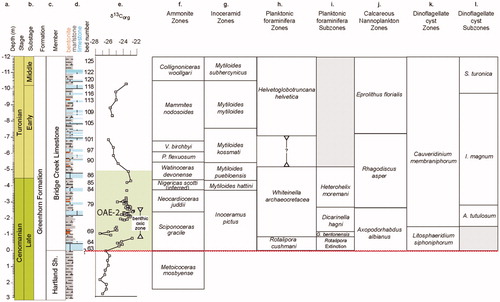
In the Cretaceous Western Interior Seaway (KWIS) of the USA, the Lower and Middle Cenomanian Substages are mainly represented by the Graneros Shale Formation in central Colorado (), and the Buda Limestone Formation and lower Eagle Ford Group in SW Texas. Previous dinoflagellate cyst investigations of the interval are from the Portland-1 core in Colorado (Eldrett et al. Citation2015a, Citation2017), and outcrops and cores of the Buda Limestone (Cornell Citation1997; Eldrett et al. Citation2015a, Citation2017) and lower Eagle Ford (Brown & Pierce Citation1962; Christopher Citation1982; Donovan et al. Citation2012; Schwab et al. 2011; Eldrett et al. Citation2014, Citation2015a, Citation2015b, Citation2017; Dodsworth Citation2016) in SW Texas. Coeval marine deposits in central Wyoming and southern Montana, the Frontier Formation, were reported by Burgess (Citation1971).
Figure 4. USGS Portland-1 core data (Eldrett et al. Citation2017): (a) depth/age; (b) chronostratigraphy; (c) lithostratigraphy; (d) lithology; (e) δ13Corg, data from Du Vivier et al. (Citation2014), Eldrett et al. (Citation2014, Citation2017), Joo and Sageman (Citation2014); (f) redox-sensitive trace metal enrichments (TMEF); (g) total organic carbon (TOC); (h) amorphous organic matter (AOM); (i) foraminiferal test linings, with micropaleontological abundance data as c.p.g. for both calcareous and agglutinated (agglut.) benthic foraminifera; (j) prasinophyte algae; (k) dinoflagellate cysts, Bosedinia cf. sp. 1 and 3 of Prauss (Citation2012b); (l) terrigenous palynomorphs; (m) T:M ratio; (n) dinoflagellate cysts P:G ratio; (o) Shannon–Wiener and Simpson–Hunter dinoflagellate cyst diversity; (p) dinoflagellate cyst zonation. *Cauveridinium membraniphorum Interval Zone.
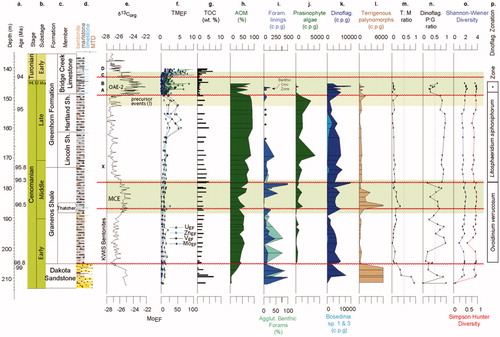
The Upper Cenomanian, Lower Turonian and Middle Turonian substages are represented by the Lincoln Shale Member, Hartland Shale Member and Bridge Creek Member of the Greenhorn Limestone Formation in central Colorado ( and ). Previous palynological investigations of the Upper Cenomanian to Lower Turonian interval are from Pueblo: (1) Courtinat (Citation1993), 21 spot samples from the lower Bridge Creek Member (S. gracile to lower W. coloradoense Ammonite Zones), focusing on possible relationships between palynofacies and lithology; (2) Li and Habib (Citation1996), 14 spot samples from the same interval, focusing on palynofacies and the ratio of chorate to proximochorate-proximate dinoflagellate cysts; (3) Dodsworth (Citation2000), 53 samples from the lower to middle Bridge Creek Member (S. gracile to lower M. nodosoides Ammonite Zones), focusing on biostratigraphy and palaeoenvironments, published in support of the then proposed (and subsequently ratified) base of the Turonian GSSP; (4) Harris and Tocher (Citation2003), 42 samples from a comparable interval (S. gracile to basal M. nodosoides Ammonite Zones), focusing on regional correlation with coeval intervals from three other central KWIS sections: Bunker Hill in Kansas, ca. 600 km to the east; Wahweap Wash in Utah, ca. 600 km to the west; and Blue Point in Arizona, ca. 730 km to the southwest. None of these previous palynological studies covers the higher part of the outcrop at Pueblo, including the Lower–Middle Turonian Substage boundary (upper M. nodosoides to C. woollgari Ammonite Zones). There are also no published palynological studies of marine sections from the Upper Turonian Substage and Coniacian Stage in Colorado. Previous palynological investigations of the Upper Cenomanian, Turonian and lowermost Coniacian interval of the upper Eagle Ford Group and overlying Austin Chalk Formation in SW Texas are by Eldrett et al. (Citation2014, 2015a, Citation2015b, Citation2017) and Dodsworth (2016).
Figure 5. Pueblo outcrop. Legend same as and , except for (k), Ccm is Cyclonephelium compactum–Cauveridinium membraniphorum c.p.g.
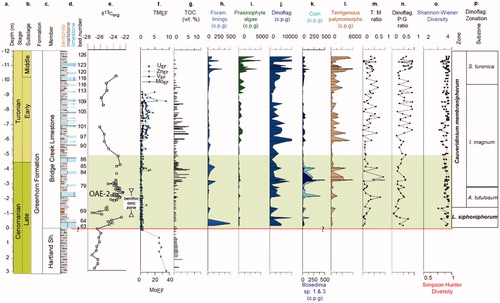
This paper documents 26 samples from the higher part of the Pueblo outcrop, Beds 110 to 125, which for the first time provide palynological data from strata that span the proposed base of the Middle Turonian Substage GSSP (Bed 120). It also provides a re-issuance of data from the underlying 53 samples previously reported by Dodsworth (Citation2000), Bed 63 (base of Bridge Creek Member) to Bed 109, with updated dinoflagellate cyst nomenclature, following the recommendations of Williams et al. (Citation2017), including taxa published since the year 2000, e.g. Senoniasphaera turonica (formerly Canningia sp. A of Marshall & Batten Citation1988 and Senoniasphaera rotundata alveolata of Pearce et al. Citation2003, Citation2009 and Prince et al. Citation2008). Due to space constraints, some taxa were grouped at generic level on the Pueblo range chart in Dodsworth (Citation2000), e.g. Heterosphaeridium, Isabelidinium/Eurydinium and Subtilisphaera, but were discussed in the corresponding text at species level. Here, they are plotted at species level in a larger supplemental range chart. More detail is also provided on the supplemental range chart for terrigenous pollen and spores and for reworked taxa.
The authors’ palynological data from the Portland-1 core, which were previously published and discussed with emphasis on palaeoenvironments (Eldrett et al. Citation2017), are reviewed here with respect to Cenomanian biostratigraphy. Our high-resolution palynological studies of the Cenomanian, Turonian and lowermost Coniacian deposits in SW Texas are also reviewed and correlation with the Pueblo and Portland-1 bioevents is discussed. The data for Portland-1 core and SW Texas cores Iona-1, Innes-1 and ‘Well X’ () are archived at the data repository PANGEA. The Digital Object Identifier DOI of the dataset is https://doi.org/10.1594/PANGAEA.877411. Eldrett et al. (Citation2015a) used uranium–lead (U–Pb) dates from individual zircons from 10 bentonite layers in Iona-1 to provide geochronological constraints for astronomical (obliquity, eccentricity and average spectral misfit) age models for individual events, including 12 dinoflagellate cyst bioevents. Here, ages for a further 23 organic-walled phytoplankton bioevents in Iona-1 have been derived using the obliquity model. The method has also been applied to Portland-1 (Eldrett et al. Citation2017). A formal dinoflagellate cyst zonation of the Cenomanian, Turonian and lowermost Coniacian in the Western Interior of the US, calibrated to these ages, is proposed here for the first time and applied to the sections documented.
1.2. Geological setting
The Late Cretaceous Epoch was characterized by a sustained warm climate resulting in high eustatic sea levels and numerous epicontinental seaways, including the KWIS. Several major global perturbations in the carbon cycle occurred, termed ‘oceanic anoxic events’ (OAE), the most prominent spanning at the Cenomanian–Turonian transition and named OAE-2 (Schlanger & Jenkins 1976; Schlanger et al. Citation1987). This interval in particular was marked by a globally recognized positive carbon isotope (δ13C) excursion, reflecting the widespread removal of δ12C-enriched organic matter in marine sediments under global anoxic conditions (Jenkyns Citation2010 and references therein). However, the deposition of organic-rich, fine-grained sediments varied both temporally and spatially, being modulated and ultimately dependent on local and regional processes (basin restriction, water stratification, bottom currents, sediment input) in addition to global phenomena (sea level change, orbital forcing; Eldrett et al. Citation2014, Citation2015b, Citation2017; Minisini et al. 2017). Less prominent maxima in the Cenomanian and Turonian δ13C curve in the KWIS, e.g. a Middle Cenomanian Event (MCE), can also be correlated inter-regionally (), particularly with England and Japan (Jarvis et al. Citation2006; Uramoto et al. Citation2013; Joo & Sageman Citation2014; Eldrett et al. Citation2015a).
Figure 6. Isotope data and correlation between studied sites and the English Chalk Reference section. Data sources: δ13C for English Chalk (Jarvis et al. Citation2006), USGS Portland-1 (Du Vivier et al. Citation2014; Eldrett et al. Citation2014, Citation2017; Joo & Sageman Citation2014), SW Texas cores (Eldrett et al. Citation2014, Citation2015a, Citation2017) and Pueblo (Bowman & Bralower Citation2005). Note variable depth scales. Wavy horizontal red lines represent hiatal surfaces. Green shading, positive δ13C carbon isotope events (CIEs); orange shading, negative CIEs. Isotopic events nomenclature follows that of Jarvis et al. (Citation2006), with the exception of precursor events (after Eldrett et al. Citation2014), which are now thought to occur within the OAE-2 CIE but may not be recorded in the Portland-1 core or the Pueblo GSSP due to a hiatal surface at the base of the Bridge Creek Member (Eldrett et al. Citation2017).
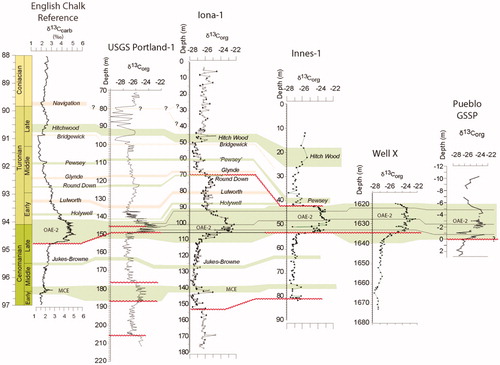
The KWIS submerged North America along a north–south axis (), at times mixing Boreal Realm waters from the north (present-day Canada) with Tethyan Realm waters from the south (Gulf of Mexico and proto-Atlantic Ocean) (Kauffman Citation1977, Citation1984a, Citation1985; Eicher & Diner Citation1985; Hay et al. Citation1993; Kauffman & Caldwell Citation1993; Slingerland et al. Citation1996; Elderbak & Leckie Citation2016; Eldrett et al. Citation2017; Lowery et al. Citation2018). In this paper, we refer to northern KWIS, including marine deposits in Canada, central KWIS including the USA to the north of Texas, and southern KWIS (Texas).
The Dakota Sandstone Formation (Albian?–earliest Cenomanian; Section 4.1) is one of the oldest Cretaceous sedimentary units in central Colorado (Gustason & Kauffman Citation1985), recording both nearshore marine and non-marine deposition during onset ‘Greenhorn’ marine transgression (Kauffman Citation1977). In Portland-1, it contains long-ranging terrigenous spores and pollen in the lower part, 211.61 to 211.53 m, and dinoflagellate cysts associated with freshwater algae from 209.59 to 205.11 m (Eldrett et al. Citation2017). At 204.39 m, it is overlain unconformably by the marine Graneros Shale Formation of Early to Middle Cenomanian age, including the Thatcher Limestone Member. Dinoflagellate cysts remain relatively abundant and diverse throughout the Graneros Shale ().
In Texas, the Buda Limestone Formation was initially deposited during the Early Cenomanian but is overlain unconformably by the organic-rich (ca. 1 to >6% total organic carbon) lower Eagle Ford Group. The Buda Limestone comprises highly diverse and low-abundance dinoflagellate cyst assemblages, dominated by gonyaulacoid taxa, ‘G-cysts’ (Cornell Citation1997; Eldrett et al. Citation2017), probably reflecting a low-nutrient, oligotrophic setting (cf. Harland Citation1988; Sluijs et al. Citation2005). By contrast, the lower Eagle Ford is generally characterized by a decline in dinoflagellate cyst species diversity, the palynological assemblages comprising high absolute abundance of green algal prasinophyte phycomata (Tyson Citation1995), peridinioid taxa, ‘P-cysts’, being the major component of the dinoflagellate cyst community (Eldrett et al. Citation2015b, Citation2017; Dodsworth Citation2016), indicative of eutrophic and stratified water column conditions (cf. Prauss Citation2007; Sluijs et al. Citation2005).
During Middle Cenomanian to Middle Turonian times, the marine Greenhorn Limestone Formation was deposited in central Colorado, comprising the interbedded restricted to open marine Lincoln Shale Member (Sageman & Johnson Citation1985), the mainly restricted marine Hartland Shale Member (Sageman Citation1985) and the more open-marine Bridge Creek Member. A δ18O carbonate curve from Pueblo published by Pratt (Citation1985, Figure 11), shows a broad up-section positive shift in values between the base of the Lincoln Shale and base of the Bridge Creek, interpreted as reflecting an increase in salinity to normal marine levels in the central KWIS during the peak Greenhorn transgression. Dinoflagellate cysts comparable to those informally described from Tarfaya, Morocco () by Prauss (Citation2012a, 2012b, Citation2012c, Citation2015), Bosedinia cf. sp. 1 and 3 of Prauss (Citation2012b), which had a mainly proto-Atlantic Tethyan Realm abundant distribution during the Late Cretaceous (Eldrett et al. Citation2017), became established in abundance during deposition of the lower Eagle Ford in SW Texas, below and above the regionally correlative ‘X-bentonite’ in Iona-1 and Innes-1, and over a shorter stratigraphical range farther north in Portland-1, above the X-bentonite (ca. 173.5 m), in the uppermost Lincoln Shale and Hartland Shale (). In both areas their abundance is associated with low-diversity agglutinated benthic foraminiferal assemblages, redox-sensitive trace metal enrichments and organic biomarkers, consistent with deposition under oxygen-deficient conditions (Sun et al. Citation2016; Eldrett et al. Citation2017; Minisini et al. 2017).
The upper parts of the lower Eagle Ford and the upper Eagle Ford Group were deposited in Texas during Middle Cenomanian to Late Turonian times. A sea level rise associated with the lower–upper Eagle Ford boundary in Texas and the Hartland Shale–Bridge Creek boundary in Colorado correlates with a decrease in organic carbon content and an increase in carbonate content, probably marking the transition from restricted-circulation, proto-Atlantic influenced conditions from the south to more Boreal open-marine-influenced conditions from the north (see below). Many of the Bridge Creek limestone and bentonite beds at Pueblo can be correlated across wide areas of the central KWIS (Hattin Citation1971, Citation1975, Citation1985; Sageman et al. Citation2006). Despite a globally contemporaneous carbon isotope (δ13C) excursion, sediments of the Bridge Creek and upper Eagle Ford were mostly oxygenated during OAE-2 in the KWIS, as indicated by limited or no enrichment in redox-sensitive elements (Meyers Citation2007; Denne et al. Citation2014; Eldrett et al. Citation2014, Citation2017; ).
The Cenomanian–Turonian transition was an interval of extreme warmth (Huber et al. Citation2002; Forster et al. Citation2007). However, the early stages of OAE-2 were characterized by a short-lived interval of cooler Boreal waters spreading southwards across Europe, termed the ‘Plenus Cold Event’ (PCE) by Gale and Christensen (Citation1996). In mid-latitude sites including Central Europe and central–southern KWIS, Boreal Realm dinoflagellate cysts, such as Cyclonephelium compactum–Cauveridinium membraniphorum () and Isabelidinium magnum, became consistent in occurrence from Late Cenomanian times onwards which has been interpreted as evidence for the PCE in the KWIS as well as in Europe (Eldrett et al. Citation2014, Citation2017; Van Helmond et al. Citation2016). In the central and southern KWIS, this interval is marked by the persistent abundance of benthic fauna (Elderbak & Leckie Citation2016 and references therein) and termed the ‘benthonic zone’ (Eicher & Worstell Citation1970) or the ‘benthic oxic zone’ (Keller & Pardo Citation2004), occurring at Pueblo in beds 68–78 of the Bridge Creek. This interval of improved water column and seafloor oxygenation has also been linked to inflow of a northerly Boreal water-mass that ventilated the seaway during a period of cooler climate conditions (Eldrett et al. Citation2014, Citation2017; Van Helmond et al. Citation2016). However, some workers still consider the PCE to be mainly restricted to Europe (Elderbak & Leckie Citation2016).
A negative δ18O shift within the lower Bridge Creek, Beds 78–85 at Pueblo, correlates with relatively organic-rich deposition during OAE-2 there () and was interpreted by Pratt (Citation1985) to reflect the spread of subsaline surface waters into the KWIS during their deposition. This interpretation was supported by Leckie (Citation1985), who reported anomalous large proportions of biserial planktonic foraminifera (Heterohelix spp.) in the beds, and Dodsworth (2000), who reported significantly larger numbers of terrigenous palynomorphs, mainly gymnosperm bisaccate pollen, than in adjacent strata (). The rapid rise in heterohelicids (surface-dweller opportunist foraminifera) has since been recorded at a correlative stratigraphical level across the KWIS, including Texas (Donovan & Staerker Citation2010; Donovan et al. Citation2012; Lowery et al. Citation2014; Eldrett et al. Citation2015a), and in Mexico (Núñez-Useche et al. Citation2016). It is attributed to the better adaptation of these organisms to sudden environmental change (temperature, salinity, nutrient level) and/or a decline in water column oxygenation (Keller & Pardo Citation2004; Caron et al. Citation2006; Reolid et al. Citation2015) and/or is associated with a brief incursion of a dysoxic proto-Atlantic water-mass into the KWIS (Eldrett et al. Citation2017). In the central KWIS, a paucity of ammonites and bivalve molluscs from this interval has been correlated with a global faunal mass extinction (Kauffman Citation1984b; Elder Citation1987, Citation1989; Harries & Kauffman Citation1990; Harries Citation1993).
In terms of ammonite provincialism, Cobban (Citation1984) reported Cenomanian–Turonian Boreal genera such as Dunveganoceras and Scaphites as having a typically northern KWIS distribution while genera such as Vascoceras, Nigericeras, Romaniceras and Thomasites, which are of value for correlation with Tethyan sequences, are mostly confined to the southernmost part of the KWIS. However, Tethyan ammonite faunas were able to migrate northwards into the central KWIS during intervals of improved water column oxygenation (Batt Citation1993) and are recorded from above the Hartland Shale–Bridge Creek boundary at Pueblo (Kauffman Citation1984a). North to south migration also occurred; Kennedy et al. (Citation1987) noted the presence of Boreal ammonite faunas as far south as SW Texas in intra-Lower Turonian strata (Pseudaspidoceras flexuosum Ammonite Zone; cf. ).
1.3. The proposed base of the Middle Turonian GSSP
The Turonian Working Group of the SCS held meetings during the Second International Symposium on Cretaceous Stage Boundaries in Brussels, 8–16 September 1995. A report of the conclusions of the Working Group was provided by Bengtson et al. (Citation1996). A GSSP at the base of Bed 86 at Pueblo () was proposed and has subsequently been formally ratified by the SCS as the base of Turonian Stage/Lower Turonian Substage (Kennedy et al. Citation2005). With respect to the base of Middle Turonian GSSP, Bengtson et al. (Citation1996) proposed, ‘The first occurrence of the ammonite Collignoniceras woollgari (Mantell 1822) in the section at Rock Canyon anticline west of Pueblo, Colorado, exposing the Bridge Creek Member of the Greenhorn Limestone. According to current state of knowledge this level lies within Bed 120 and coincides with the first occurrence of the inoceramid bivalves Mytiloides hercynicus (Petrascheck 1903) and Inoceramus cuvierii J. Sowerby, 1814, in the section.’ Following the Brussels meeting, a ballot of the 39 members of the Working Group was 33 in favour of the above proposal, four against and two abstentions. The negative voters argued that the boundary stratotype should be selected in Europe, where the historical stage stratotypes are, and that the Rock Canyon anticline section is still too poorly known, in particular with respect to microfossil stratigraphy. Kennedy et al. (Citation2000) provided further inoceramid data for the section in support of the proposed substage boundary level. The present paper provides the first palynological data, including dinoflagellate cyst stratigraphy, also in support of the proposal, addressing the criticism of insufficient microfossil data. Detailed information on precise location and access at the proposed base of the Middle Turonian GSSP can be found in Kennedy et al. (Citation2000).
2. Material and methods
Seventy-nine channeled samples (Bridge Creek, BC series) from approximately 12 m of the lower and middle Bridge Creek Member at Pueblo were prepared and analyzed for palynology. The higher 26 samples (BC54 to BC80, Beds 110 to 125) were prepared in the laboratory at the same time as the lower 53 samples (BC01 to BC53, Beds 63 to 109) but had not been analyzed for palynology at the time of publication of Dodsworth (Citation2000). Five grams, or multiples thereof, of crushed, dried material from each rock sample was dissolved in hydrochloric acid (HCl 35%) and hydrofluoric acid (HF 40%) in order to remove carbonate and silicate minerals, respectively. Preparations were sieved with a 10 μm mesh. Palynomorphs and brown and black wood fragments (vitrinite and inertinite) dominate the >10 μm kerogen in the lower part of the succession at Pueblo (S. gracile, lower N. juddii and lower W. coloradoense Ammonite Zones). Some of these preparations contained transparent, ‘cloudy’ amorphous organic matter (AOM) which was removed by a ‘nitric wash’, i.e. two minutes of oxidation with nitric acid (70% HNO3). In the upper N. juddii Ammonite Zone and upper W. coloradoense–M. nodosoides Ammonite Zones, the >10 μm kerogen fraction from the mudstone samples is dominated by dark-colour, clumped AOM. Fragments of this material outnumber palynomorphs by a ratio of several hundreds or thousands to one. Between three minutes and 36 hours of oxidation with Schulze’s solution (70% HNO3 supersaturated with potassium chlorate, KClO3), followed by one subsequent rinse with 2% potassium hydroxide (KOH) solution, were used to liberate palynomorphs from the AOM. The >10 μm kerogen fraction from the interbedded limestones at these levels is composed of palynomorphs, brown and black wood fragments and dark-colour clumped AOM. In general, oxidation of preparations from such limestones was restricted to a nitric wash. In the upper M. nodosoides–C. woollgari Ammonite Zones, the >10 μm kerogen fraction from both mudstones and the relatively argillaceous limestone samples at these levels are dominated by dark-colour, clumped AOM, and extended oxidation with Schulze’s solution, as described above, was applied.
All preparations were stained with Safranin O solution (red stain). Approximately equal portions of organic residues from each sample were strewn over four 22 × 22 mm cover slips, dried and mounted onto microscope slides using Petropoxy 154. ‘Absolute abundance’, i.e. the estimated concentration of palynomorphs in the samples (counts per gram, c.p.g.), was calculated using the volumetric method described by Dodsworth (Citation2000).
Three hundred palynomorphs were counted from one microscope slide for each sample and remaining material from up to four microscope slides was subsequently scanned for additional rare taxa. Relative abundance categories follow Dodsworth (Citation2000, Figures 6, 7, 12) and are described as rare (1, 2 or outside the count), common (3–29) or abundant (30+). Standard palaeoenvironmental parameters were calculated for the Pueblo section, including: (i) the ratio between terrestrial (T) and marine (M) palynomorphs (T:M ratio) as a proxy for terrestrial input; (ii) the ratio between P-cysts and G-cysts (P:G ratio) of the dinoflagellate cyst assemblage as a proxy of nutrient input; (iii) the diversity of the dinoflagellate cyst assemblages was calculated using both Shannon–Wiener (H) and Simpson–Hunter (D) indexes. Detailed discussion of these palaeoenvironmental parameters is provided in Eldrett et al. (Citation2017). As mentioned above, the higher 26 samples documented here for the first time received extended laboratory oxidation with Schulze’s solution, which can skew the P:G ratio due to a preferential wholesale removal of G-cysts by relatively severe oxidation (Dodsworth Citation1995, Citation2000, Citation2004a, Figure 4). It should be noted that the prominent modern P-cyst family, Protoperidiniaceae, probably do not extend back stratigraphically before the Campanian Stage of the Cretaceous (Bujak & Davies Citation1983). Protoperidiniaceae, in contrast to the much more resilient mid-Cretaceous P-cysts recorded here (and other Cenozoic P-cyst families), are readily removed from preparations by relatively mild laboratory oxidation such as a nitric wash (pers. obs.; Harland et al. Citation1980; Davies et al. Citation1982) or by natural oxidation of Quaternary sediments below the seafloor (Reichart & Brinkhuis Citation2003; Zonneveld et al. Citation2007).
Figure 7. Tabulation of Cenomanian, Turonian and lowermost Coniacian dinoflagellate cyst and prasinophyte bioevents in SW Texas (TX) and Colorado (CO), with bioevents listed in order of occurrence in Iona-1. Age estimates are derived from the astronomical (obliquity) age model presented in Eldrett et al. (Citation2015a). Pueblo area lithostratigraphy is taken from Kauffman and Pratt (Citation1985). Pueblo ammonite zones are given next to the bed numbers in which phytoplankton bioevents occur (); Sciponoceras gracile (S.g.), Neocardioceras juddii (N.j.), Mammites nodosoides (M.n.), Collignoniceras woollgari (C.w.). The position of the base of the Turonian Stage in Portland-1 core is taken from Sageman et al. (Citation2006), correlating with the GSSP outcrop base of Bed 86. * The stratigraphically highest productive sample analyzed in a section. u/c = a probable >2 million years hiatus below the base of the intra-Middle Turonian (Langtry Member, upper Eagle Ford Group) in Innes-1 and Lozier Canyon (Eldrett et al. Citation2015a, Citation2017). Early–Middle Cenomanian boundary (ca. 96.6 Ma) in CO and TX: in Portland-1, LO Ovoidinium verrucosum at 178.33 m and FO consistent/common Litosphaeridium siphoniphorum at 178.10 m occur in the basal Middle Cenomanian, within and immediately above the carbon isotope MCE (cf. ); in Iona-1 and Innes-1, FO common L. siphoniphorum occurs around the base of the MCE. Middle–Late Cenomanian boundary (ca. 95.7 Ma) in CO and TX: onset Bosedinia abundance during the Middle Cenomanian in Texas pre-dates that in Colorado (Late Cenomanian). ‘Low’ next to a bioevent indicates a stratigraphically low occurrence relative to the other sections: in Iona-1, an isolated occurrence of rare O. verrucosum at 171.51 m occurs within the Lower Cenomanian; an isolated occurrence of common Adnatosphaeridium tutulosum at 104.18 m is below the level of its LO in the other sections; at Pueblo, FO consistent and FO common Senoniasphaera turonica are within the upper part of the Lower Turonian while these events are stratigraphically higher, within the upper part of the Middle Turonian, in Iona-1 and Innes-1; at Lozier Canyon, an influx of common Senoniasphaera rotundata at 44.18 m, within the Langtry Member, is below the FO of common S. rotundata in the Austin Chalk in Iona-1 and Innes-1. ‘High’ next to the LO of Adnatosphaeridium? chonetum in Iona-1 indicates a stratigraphically higher, possibly reworked basal Turonian LO, relative to the other sections, where its LO is intra-Upper Cenomanian. See main text for further discussion.
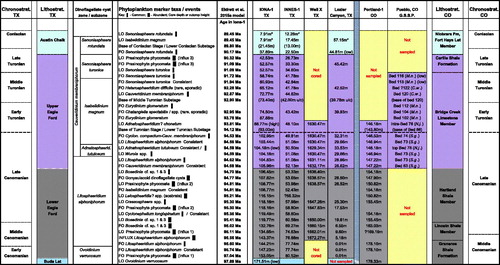
Plate 1. Dinoflagellate cysts and sporomorphs from Pueblo, Colorado. All specimens, apart from figure 11, were magnified at 400×. Pueblo beds, corresponding BC sample/slide numbers and specimen England Finder coordinates are given (see Supplement for detail of sampling). Figure 1. Adnatosphaeridium tutulosum (Cookson & Eisenack 1960) Morgan 1980, Bed 67 (BC05A), T21/1; Figure 2. Adnatosphaeridium? chonetum (Cookson & Eisenack Citation1962) Davey 1969, Bed 66 (BC04A), Q34/0; Figure 3. Litosphaeridium siphoniphorum (Cookson & Eisenack Citation1958) Davey & Williams Citation1966, Bed 66 (BC04A), M27/3; Figure 4. Pterodinium? pterotum (Cookson & Eisenack Citation1958) Pavlishina 1990, Bed 66 (BC04A), H17/1; Figure 5. Bosedinia cf. sp. 1 of Prauss, Citation2012b; see Eldrett et al. Citation2017 (Supplement) for taxonomic discussion); Bed 85 (BC26A), Q33/4; Figure 6. Laciniadinium biconiculum McIntyre 1975, Bed 65 (BC03A), U27/4; Figure 7. Heterosphaeridium difficile (Manum & Cookson 1964) Ioannides 1986, Bed 111 (BC55A), L21/4 (operculum only); Figure 8. Isabelidinium? globosa (Davey, Citation1970) Lentin & Williams 1977, Bed 121 (BC67A), W31/2; Figure 9. Questionable Alterbidinium ioannidesii Pearce Citation2010, Bed 100 (BC40A), K23/1. The specimen appears to be an autocyst, rather than having two wall layers as seen on complete specimens of A. ioannidesii (cornucavate to circumcavate) and has less well-developed tabulation than typical specimens; Figure 10. Alterbidinium ‘daveyi’ (Stover & Evitt 1978) Lentin & Williams 1985, Bed 115 (BC59A), S35/0; Figure 11. Wuroia spp., Bed 66 (BC04A), R25/2 (specimen magnified at 200×; the plate’s scale bar equates to 100 μm for this specimen); Figure 12. Questionable Raphidodinium fucatum Deflandre 1936, Bed 124 (BC74A), M28/3. A partially obscured specimen with a shorter spine length to body width ratio than is typical for R. fucatum; Figure 13. Microdinium setosum Sarjeant 1966, Bed 74 (BC11A), W21/2; Figure 14. Eurydinium glomeratum (Davey Citation1970) Stover & Evitt 1978, Bed 122 (BC70A), X22/1; Figure 15. Isabelidinium magnum (Davey Citation1970) Stover & Evitt 1978, Bed 125 (BC77A), P23/0; Figure 16. Trithyrodinium suspectum (Manum & Cookson 1964) Davey Citation1969, Bed 121 (BC66A), U31/2; Figure 17. Classopollis spp. (tetrad), gymnosperm conifer (Pinophyta) pollen, Bed 125 (BC79A), U27/3; Figure 18. Complexiopollis complicatus Goczán 1964, angiosperm (Normapolles) pollen, Bed 121 (BC69A), U31/2; Figure 19. Complexiopollis spp., Bed 78 (BC16A), P25/2; Figure 20. Complexiopollis spp., Bed 82 (BC22.5A), T39/0; Figure 21. Atlantopollis spp., angiosperm (Normapolles) pollen, Bed 95 (BC36A), K30/4; Figure 22. Trisolissporites radiatus (Chlonova 1960) Tschudy 1973, miospore, Bed 82 (BC22A), P37/2.
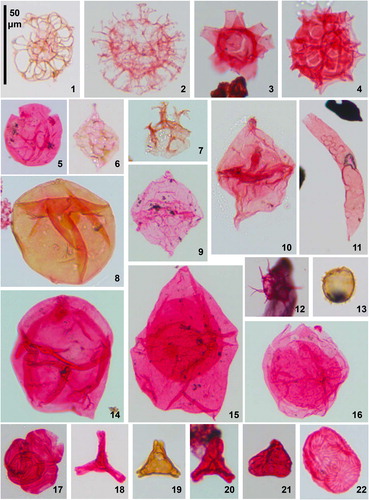
Plate 2. Dinoflagellate cysts from Pueblo and Portland-1, Colorado. Specimens were magnified at 400×; the 50 μm scale bar applies to all specimens. Pueblo beds, corresponding BC sample/slide numbers, Portland-1 depths/slides, and specimen England Finder co-ordinates are given. Figure 1. Cauveridinium membraniphorum (Cookson & Eisenack 1962) Masure in Fauconnier & Masure 2004, Bed 110 (BC54A), R30/1; Figure 2. Senoniasphaera turonica (Prössl Citation1990, ex Prössl 1992) Pearce et al. Citation2011, Bed 124 (BC76A), S33/0; Figure 3. Senoniasphaera rotundata Clarke & Verdier Citation1967, Bed 113 (BC58A), E38/3; Figure 4. Heterosphaeridium conjunctum Cookson & Eisenack 1968, Bed 112 (BC56A), L31/1; Figure 5. Canningia senonica Clarke & Verdier Citation1967, Bed 102 (BC44A), V32/0; Figure 6. Subtilisphaera pontis-mariae (Deflandre 1936) Lentin & Williams 1976, Bed 121 (BC67), S32/3; Figure 7. Senoniasphaera microreticulata Brideaux & McIntyre Citation1975, Portland-1, 204.39 m, O15/4; Figure 8. Tenua hystrix Eisenack, 1958, Bed 124 (BC76A), Q36/0; Figure 9. Florentinia? torulosa (Davey & Verdier Citation1976) Lentin & Williams 1981, Bed 102 (BC42A), V32/2; Figure 10. Ovoidinium verrucosum ostium (Davey Citation1970) Lentin & Williams 1975, Portland-1, 205.15 m, T25/1; Figure 11. Ovoidinium verrucosum verrucosum (Cookson & Hughes Citation1964) Davey Citation1970, Portland-1, 205.15 m, X31/4; Figure 12. Kiokansium williamsii Singh Citation1983, Portland-1, 208.96 m, E13/2; Figure 13. Luxadinium propatulum Brideaux & McIntyre Citation1975, Portland-1, 209.59 m, V26/3.
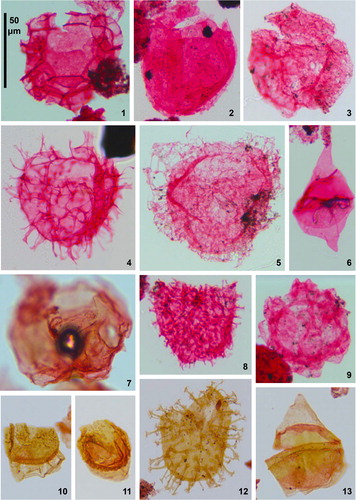
All the Pueblo microscope slides are curated in the collections of the Centre for Palynology, Department of Animal and Plant Sciences, University of Sheffield, UK. A full range chart of palynological data is available in the Supplement. Full author names and synonyms of dinoflagellate cysts can be found in Williams et al. (Citation2017).
3. Results
3.1. Palynology across the Lower–Middle Turonian boundary at Pueblo
A description and discussion of the palynology of the lower 53 samples from Pueblo (Beds 63 to 109) can be found in Dodsworth (Citation2000). In these samples, the purer micritic limestone beds tend to contain lower recovery (200–3000 c.p.g.) uncompressed palynomorphs that are dominated by dinoflagellate cyst assemblages while the mudstone inter-beds contain higher concentrations (1000–17,000 c.p.g.) of compressed/flattened palynomorphs (). The 26 newly analyzed Pueblo samples (Beds 110 to 125) also yielded predominantly marine phytoplankton assemblages; mainly dinoflagellate cysts together with subordinate prasinophyte phycomata and foraminiferal test linings. Concentration of palynomorphs is generally higher from Bed 121 (BC65) to Bed 125 (BC80; >10,000 c.p.g. in most mudstone lithologies) than from the Bed 110 (BC54) to Bed 120 (BC64; generally <10,000 c.p.g. in mudstones). Dinoflagellate cyst assemblages are diversified (Shannon–Weiner: >3.0; Simpson–Hunter: >0.8); a total of 153 taxa were recorded from the 26 samples, with between 28 and 75 taxa present in each sample. P-cyst taxa are prominent in most samples (P:G ratio >0.5): Palaeohystrichophora infusorioides is abundant throughout the section while Alterbidinium ‘daveyi’, Isabelidinium? globosum, Isabelidinium magnum, Subtilisphaera pontis-mariae and Subtilisphaera spp. are generally common; Trithyrodinium suspectum and Trithyrodinium spp. are abundant from Bed 121 (BC66) to the top of Bed 125 (BC80); Eurydinium glomeratum is common to abundant from Bed 112 (BC57) and above.
G-cysts are mostly represented by gonyaulacacean and areoligeracean taxa. The former include Spiniferites ramosus, Spiniferites twistringiensis, Florentinia spp., Pterodinium cingulatum cingulatum and sporadic influxes in some limestone beds of Hystrichodinium pulchrum, Beds 111, 118 and 120 (BC55, BC62, BC64), and Tanyosphaeridium spp., Beds 111 and 113 (BC55, BC58). Areoligeracean taxa include Tenua hystrix, abundant from Bed 116 to basal Bed 121 (BC60 to BC65), spanning the Lower–Middle Turonian boundary in Bed 120, Cauveridinium membraniphorum, which is common up to Bed 120 (BC64), and Senoniasphaera turonica, which is common from Bed 116 (BC60) to the top of the sampled section. Specimens of Heterosphaeridium conjunctum and Heterosphaeridium heteracanthum are common between Bed 90 (BC34) and Bed 116 (BC60).
Land-derived (terrigenous) pollen and spores are mainly represented by gymnosperm pollen (bisaccate pollen and Classopollis), with subordinate angiosperm Normapolles pollen (Atlantopollis and Complexiopollis) and tricolpate pollen, and pteridophyte/bryophyte spores. Gymnosperm pollen comprise up to a third of total palynological assemblages (T:M ratio >0.25).
3.2. Distribution of reworked palynomorphs at Pueblo
Reworked palynomorphs are rare at Pueblo. Pteridophyte spores reworked from Carboniferous deposits, mainly Densosporites spp. and Lycospora pusilla, are consistent from Bed 63 (BC02) to Bed 78 (BC18) in the Upper Cenomanian section, and occur sporadically in the Turonian section. Taeniate (striate) bisccate pollen that are probably reworked from Permo-Triassic deposits are also sporadically recorded.
Isolated specimens of dinoflagellate cysts that are probably reworked from Jurassic deposits were recorded from the Upper Cenomanian section; Chytroeisphaeridia chytroeides in Beds 64–65 (BC03), Scriniodinium spp. and questionable Gonyaulacysta jurassica in Bed 78 (BC13.5), and Rhynchodiniopsis cladophora in Beds 70–71 (BC09) and Bed 85 (BC26). Isolated specimens of taxa probably reworked from Albian to Lower Cenomanian deposits were recorded from the basal Middle Turonian section; Endoceratium turneri in Bed 121 (BC67 and BC69), Kiokansium unituberculatum in Bed 122 (BC70) and Nyktericysta? vitrea and Ovoidinium scabrosum/verrucosum in Bed 122 (BC71).
4. Biozonation
Many of the dinoflagellate cyst taxa recorded in the present study have stratigraphical ranges broader than Cenomanian and Turonian. The distribution of well-documented regional/inter-regional range base (First Occurrence, FO) and range top (Last Occurrence, LO) data from this interval is discussed below with reference to Portland-1, Pueblo and the SW Texas sections. Vintage dinoflagellate cyst zonation schemes are based mainly on data from southern England and northern France (Clarke & Verdier Citation1967; Foucher Citation1981) and eastern Canada (Williams Citation1977), but can be broadly applied to the central and southern KWIS. The most recent proposed Central and Northern European scheme (Olde et al. Citation2015a), which reviews in detail the earlier schemes of the Upper Cenomanian to Lower Coniacian interval, is applied here with modifications, as most marker taxa are present over the studied interval. Zone ages given in the following section are based on an astronomical (obliquity) age model applied to the named bioevents in Portland-1 (Eldrett et al. Citation2017) and Iona-1 (Eldrett et al. Citation2015a; ).
Palynological zonation of non-marine Upper Cretaceous sections in the KWIS (Nichols Citation1994), including continental settings in Wyoming (Lynds & Slattery Citation2017) and Utah (Akyuz et al. Citation2016), are based on pollen. The entire study interval considered here for dinoflagellate cyst zonation (Lower Cenomanian to lowermost Coniacian) is tentatively correlated with the Nyssapollenites albertensis Singh, 1971 (Pollen) Interval Biozone of Nichols (Citation1994). The presence of marine sections containing dinoflagellate cysts in central Wyoming and southern Montana (Burgess Citation1971) indicates that the dinoflagellate cyst zonation defined here may be applicable to the coeval Frontier Formation and Greenhorn Formation in those areas.
4.1. Albian–Cenomanian boundary interval
The Dakota Sandstone in Portland-1 contains dinoflagellate cysts from 209.59 m to 205.11 m, including taxa that are indicative of proximity to the Albian–Cenomanian boundary: rare to common Ovoidinium spp., mainly specimens transitional between O. scabrosum and O. verrucosum, and Senoniasphaera microreticulata, which has a (possibly reworked) LO in the first sample analyzed (204.39 m) above the unconformity at the base of the overlying Graneros Shale. The LO of O. scabrosum occurs near the top of the Albian Stage in Northern Europe (Costa & Davey Citation1992; Duxbury Citation2001). Brideaux and McIntyre (Citation1975), studying the Northwest Territories in Canada, and Århus (Citation1991), studying north Greenland, southeastern Spitsbergen and the Barents Sea, considered the total stratigraphical range of S. microreticulata to correlate with the base and top of the Middle Albian Substage. Williams and Bujak (Citation1985) indicated a broader range for the taxon, coinciding with entire Albian Stage. In Portland-1, the top of the Dakota Sandstone (205.10 m) is assigned a date of ∼99 Ma based on carbon isotope correlation with the astronomically calibrated Iona-1 core (Eldrett et al. Citation2017), which is younger than the estimated age of the base of Early Cenomanian Substage at its GSSP section in France, ca. 100.5 Ma (International Chronostratigraphic Chart, v. 2017/02; http://cretaceous.stratigraphy.org), suggesting that S. microreticulata may range into the lowermost Cenomanian in Portland-1.
4.2. The Ovoidinium verrucosum interval zone
Definition of zone: From the first sample stratigraphically above the LO of Senoniasphaera microreticulata (base of zone) to the stratigraphically highest sample below the FO of common Litosphaeridium siphoniphorum (top of zone).
Depth range: Portland-1, 201.27 m to 178.33 m; Iona-1, base not seen, top at 144.63 m.
Age of zone: Early to Middle Cenomanian (ca. 96.8 Ma to 96.4 Ma in Portland-1, , Eldrett et al. Citation2017; top at ca. 96.6 Ma in Iona-1, ).
Discussion: The Graneros Shale in Portland-1, from its base (205.10 m) up to the proposed unconformity at the top of the carbon isotope Middle Cenomanian Event (MCE) at ca. 178.20 m (; Eldrett et al. Citation2017), contains dinoflagellate cyst taxa, including rare Ovoidinium verrucosum, with its LO at 178.33 m, and Luxadinium propatulum, LO at 179.86 m. Burgess (Citation1971) reported O. verrucosum to be common over its stratigraphical range in central Wyoming and southern Montana. The LO of O. verrucosum has been used as a Lower Cenomanian marker event in North America and northern Europe (Cookson & Hughes Citation1964; Burgess Citation1971; Williams & Bujak Citation1985; Costa & Davey Citation1992; Scott et al. Citation2018, who dated it at 96.43 Ma in Kansas). In Portland-1, the correlation of its LO with the MCE suggests that it ranges into the basal part of the Middle Cenomanian in that section. It is very rare in the Buda Limestone of SW Texas, as are most P-cysts (Cornell Citation1997; Eldrett et al. Citation2017; Section 1.2), but is consistently present in the Lower Cenomanian part of the siliciclastic Woodbine Formation in eastern Texas (Beach Citation1985).
We propose using the stratigraphically highest sample below the FO of common Litosphaeridium siphoniphorum to define the top of the O. verrucosum Interval Zone. In Portland-1, this is coincident with the LO of O. verrucosum (178.33 m), occuring within the MCE. The FO of common L. siphoniphorum in Portland-1 (178.10 m) occurs immediately above the top of the MCE but occurs around the base of the MCE in Iona-1 and Innes-1 (cf. and ).
4.3. The Litosphaeridium siphoniphorum interval zone
Definition of zone: From the FO of common Litosphaeridium siphoniphorum (base of zone) to the LO of common Litosphaeridium siphoniphorum (top of zone).
Depth range: Portland-1, 178.10 m to 147.22 m; Iona-1, 144.21 m to 104.83 m.
Outcrop range at Pueblo: Bed 63 (BC01; base not seen) to Bed 73 (BC10).
Age of zone: Middle to Late Cenomanian (ca. 96.4 Ma to 94.54 Ma in Portland-1, , Eldrett et al. Citation2017; ca. 96.6 Ma to 94.62 Ma in Iona-1, ).
Discussion: In Central and Northern Europe, Olde et al. (Citation2015a) proposed a Cenomanian Litosphaeridium siphoniphorum Zone, with the top defined by an intra-Upper Cenomanian bioevent, LO common L. siphoniphorum. They did not define a base for the zone. For the central and southern KWIS, we propose using this zone with the additional criterion of using the regional FO of common L. siphoniphorum as its base. The FO of the taxon occurs in Upper Albian deposits in Europe (Davey & Verdier Citation1971; Foucher Citation1981) but the FO of consistent L. siphoniphorum is stratigraphically higher than this in the KWIS, occurring in the upper part of the Graneros Shale in Portland-1 (178.10 m) and just above the base of the lower Eagle Ford in SW Texas, the FO of common L. siphoniphorum occurring in the same sample or a short distance above (). This may also be the case in a proto-Atlantic site at Tarfaya, Morocco (), where FO common L. siphoniphorum is calibrated to the upper part of the Rotalipora reicheli Foraminiferal Zone (Prauss Citation2012a), in proximity to the Lower–Middle Cenomanian boundary.
The LO of consistent L. siphoniphorum is coincident with the LO of common L. siphoniphorum at Pueblo (and with the top of the zone), in Bed 73, but the two events are separated by a short distance in Portland-1 (28 cm; Eldrett et al. Citation2017), Iona-1 (139 cm; Eldrett et al. Citation2015a) and Lozier Canyon (70 cm; Dodsworth Citation2016), possibly reflecting more expanded sections and/or higher-density sampling. In southern England, LO consistent and LO common L. siphoniphorum are coincident in Plenus Marls Bed 6 at Lulworth and Eastbourne (Dodsworth Citation2000; Pearce et al. Citation2009). Isolated specimens have sporadically been recorded in the Turonian at Pueblo and elsewhere; the Isle of Wight, southern England (Clarke & Verdier Citation1967), the Touraine area of France (Foucher Citation1982) and the Münster Basin of Germany (Marshall & Batten Citation1988). Foucher (Citation1983) recorded an isolated specimen from Coniacian deposits. It is uncertain whether these isolated Turonian and younger occurrences are as a rare species or as reworking. Previous reports of LO consistent L. siphoniphorum in Lower Turonian deposits (Williams & Bujak Citation1985; Costa & Davey Citation1992) derive from former assignment of the Plenus Marls in southern England to this substage (Jefferies 1962, Citation1963); it has subsequently been confidently reassigned to the Upper Cenomanian Substage (Jarvis et al. Citation1988; Gale et al. Citation1993). An Upper Cenomanian LO of consistent L. siphoniphorum has been reported worldwide, including other locations in southern England (Davey Citation1969; Hart et al. Citation1987), the Witch Ground Graben, central North Sea (Harker et al. Citation1987), France (Foucher Citation1979, Citation1980; Courtinat et al. Citation1991), northern Spain (Mao & Lamolda Citation1999), northern Germany (Marshall & Batten Citation1988), eastern US (Aurisano Citation1989), Ocean Drilling Project holes including Demerara Rise (Leg 207, Site 1260) and Kerguelen Plateau (Leg 183, Site 1138; Eldrett et al. Citation2017), Australia (Morgan Citation1980; McMinn Citation1988) and New Zealand (Hasegawa et al. Citation2013; Schiøler & Crampton Citation2014).
Supplementary bioevents: Burgess (Citation1971) used the FO of Alterbdinium ‘daveyi’ (i.e. the comparable form Deflandrea sp. cf. D. acuminata) as an intra-Cenomanian correlative event in central Wyoming and southern Montana. Singh (Citation1983) suggested a Middle Cenomanian FO for A. ‘daveyi’ and LO for Kiokansium williamsii in Alberta, Canada. Williams (Citation2006) cited a date of 96.2 Ma for the LO of K. williamsii in the Scotian Basin, Canada. In Portland-1, both events occur at 169.19 m, just above the base of the Upper Cenomanian as picked by Eldrett et al. (Citation2017), ca. 95.6 Ma, following the suggested level for the Middle–Upper Cenomanian boundary (Kennedy & Gale Citation2006; Ogg et al. Citation2016; Section 1.1).
Within the L. siphoniphorum Interval Zone, there are other correlative bioevents across the central and southern KWIS, including: a lower influx of prasinophyte phycomata, an influx of Bosedinia cf. sp.1 and sp.3 of Prauss (Citation2012b), an upper influx of prasinophyte phycomata, FO Isabelidinium magnum and FO Cauveridinium membraniphorum (). While the first three events appear to be organic rich and facies controlled, probably related to incursion of anoxic–dysoxic proto-Atlantic water-masses from a southerly direction, and are diachronous, i.e. occurring later farther north in Colorado than in SW Texas (Section 1.2), the latter two events are useful supplemental correlative events within the Upper Cenomanian. The FOs of consistent I. magnum (154.18 m in Portland-1; Bed 63 [BC01] at Pueblo) and C. membraniphorum (147.22 m in Portland-1; Bed 63 [BC02] at Pueblo), taxa more commonly encountered in northerly Boreal water-masses (Eldrett et al. Citation2014, Citation2015a, Citation2017; Van Helmond et al. Citation2016), are regionally correlative. The FO of I. magnum also occurs in the Upper Cenomanian outside the KWIS, eastern Canada, Labrador–Baffin Seaway (Pedersen & Nøhr-Hansen Citation2014; Nøhr-Hansen et al. Citation2016) and Northern Europe (Costa & Davey Citation1992). The FO of C. membraniphorum is recorded from deposits as old as Middle Albian (Foucher Citation1981), but only consistently from the Middle Cenomanian in high-latitude sites (Van Helmond et al. Citation2016); its consistent FO at higher stratigraphic levels still, i.e. intra-Upper Cenomanian, in many mid-latitude sites such as Pueblo, other sites within the central KWIS (Harris & Tocher Citation2003), and Texas (Eldrett et al. Citation2014, Citation2015a, Citation2017), may reflect a southerly incursion of a Boreal water-mass to the KWIS and Central Europe, the Plenus Cold Event (Section 1.2).
The FO of Dinogymnium spp. occurs at 147.90 m in Portland-1 and Bed 66 (BC04) at Pueblo. Burgess (Citation1971) demonstrated the correlation of its FO (i.e. FO Dinogymnium heterocostatum) in proximity to the Frontier Formation–Greenhorn Formation boundary, as defined in central Wyoming and southern Montana.
At Pueblo, the LO of Pterodinium? pterotum occurs in Bed 68 (BC06). Davey and Williams (Citation1966) and Clarke and Verdier (Citation1967) recorded it from the Middle and Upper Cenomanian in southern England, its LO coinciding with that of L. siphoniphorum within the Plenus Marls on the Isle of Wight. The two events also correlate at Melton Ross in eastern England (unpubl. data, cf. Dodsworth Citation2000, Figure 12).
4.4. The Cauveridinium membraniphorum interval zone
Definition of zone: From the first sample stratigraphically above the LO of common Litosphaeridium siphoniphorum (base of zone) to the higher of either the LO of Cauveridinium membraniphorum or the LO of Isabelidinium magnum (top of subzone).
Depth range: Portland-1, 146.94 m to 145.24 m (shallowest core sample analyzed); Iona-1, 104.18 m to 7.91 m.
Outcrop range at Pueblo: Bed 74–75 (BC11) to Bed 125 (BC80; highest sample analyzed).
Age of zone: Late Cenomanian to Early Coniacian (ca. 94.62 Ma to 89.45 Ma in Iona-1, ).
Discussion: In Central and Northern Europe, Olde et al. (Citation2015a) proposed a Cauveridinium membraniphorum Zone, an interval zone defined between LO common L. siphoniphorum (intra-Upper Cenomanian) and LO C. membraniphorum (Lower Coniacian). It is composed of a lower unnamed interval, a Senoniasphaera turonica Subzone (base marked by the FO of common S. turonica) and a Raetiaedinium truncigerum Subzone (base marked by the FO of consistent R. truncigerum). As the latter taxon is not recorded from Turonian strata in central and southern KWIS sections investigated to date (), we propose modifications to the C. membraniphorum Zone in this region, splitting it into Adnatosphaeridium tutulosum, Isabelidinium magnum, Senoniasphaera turonica and Senoniasphaera rotundata Subzones. As the maker taxon C. membraniphorum is rarely recorded above the Turonian Stage in Northern Europe (Costa & Davey Citation1992) and has not to date been recorded in the KWIS from above the Turonian in Kansas (Scott et al. Citation2018) or Texas (this paper), we also propose using the alternative LO of Isabelidinium magnum to define the top of the zone if I. magnum is recorded at a stratigraphically higher level (see Section 4.4.4).
4.4.1. The Adnatosphaeridium tutulosum interval subzone
Definition of subzone: From the first sample stratigraphically above the LO of common Litosphaeridium siphoniphorum (base of subzone) to the LO of consistent Adnatosphaeridium tutulosum (top of subzone).
Depth range: Portland-1, 146.94 m to 146.18 m; Iona-1, 104.18 m (single sample).
Outcrop range at Pueblo: Bed 74–75 (BC11) to top of Bed 78 (BC18).
Age of subzone: Late Cenomanian (ca. 94.59 Ma in Iona-1, ).
Discussion: The LO of Adnatosphaeridium tutulosum occurs in Bed 78 equivalent at Bunker Hill in Kansas (Harris & Tocher Citation2003) and in a comparable stratigraphical position at Lozier Canyon outcrop and Innes-1 and ‘Well X’ cores in SW Texas (). At locations in Europe, LO A. tutulosum also occurs within the uppermost Cenomanian, above LO consistent/common L. siphoniphorum in southern England (Plenus Marls, Bed 8 and Bed 6, respectively; Dodsworth Citation2000; Pearce et al. Citation2009), eastern England (Dodsworth Citation1996; Citation2000, Figure 12), northern Germany (Marshall & Batten Citation1988), southern France (cf. Courtinat et al. Citation1991; Jarvis et al. Citation2011) and at the same level as LO L. siphoniphorum in northern France (Foucher Citation1983) and Crimea (Dodsworth Citation2004a).
Supplementary correlative events include: LO consistent Wuroia spp. occurs at, or just above, the base of the subzone. The taxon occurs between Beds 63 and 73 (BC01 to BC10) at Pueblo and from 147.90 m to 146.94 m in Portland-1. It is also present in the SW Texas sections () but has not been reported outside the KWIS.
LO Adnatosphaeridium? chonetum is consistently recorded from Upper Cenomanian strata (Bed 63 to intra-Bed 78; BC01 to BC17) at Pueblo and from 147.90 m to 146.18 m in Portland-1. Harris and Tocher (Citation2003) recorded a similar distribution at Bunker Hill in Kansas, Wahweap Wash in Utah and Blue Point in Arizona. In the SW Texas sections () it also has an uppermost Cenomanian LO, apart from in Iona-1, where its (possibly reworked) LO occurs in basal Turonian strata. The event has not been reported outside the KWIS though the occurrence of A? chonetum in Cenomanian deposits was noted by Davey (Citation1969) in Northern Europe and by Cookson and Eisenack (Citation1962) and Backhouse (Citation2006) in Australia.
A single specimen of Microdinium setosum was recorded in Beds 74–75 (BC11) at Pueblo. In Northern Europe, this taxon occurs consistently in the Cenomanian (Clarke & Verdier Citation1967) but can occur sporadically as high as Middle Turonian in some areas (Costa & Davey Citation1992).
The FO of abundant Cyclonephelium compactum and common Cauveridinium membraniphorum occur in Beds 74–75 (BC11) at Pueblo, with an acme of C. membraniphorum in Bed 84 (BC25). There is also an isolated influx of common C. membraniphorum in the uppermost Cenomanian at Lozier Canyon (32.31 m) and Innes-1 (47.10 m) but at a higher stratigraphical level (78.28 m; Lower Turonian) in Iona-1. In Iona-1, both taxa and forms transitional between them are common in the uppermost Cenomanian to Lower Turonian (72.75 m to 102.95 m; Eldrett et al. Citation2015a). In Europe, they have a common uppermost Cenomanian to Lower Turonian occurrence in Germany (Marshall & Batten Citation1988; Prauss Citation2006), Crimea (Dodsworth Citation2004a), Poland (Dodsworth Citation2004b) and France (Foucher Citation1983; Courtinat et al. Citation1991). Cauveridinium membraniphorum is rare or absent in coeval Tethyan sections farther south, in Spain (Lamolda & Mao Citation1999; Peyrot Citation2011; Peyrot et al. Citation2011, Citation2012) and Morocco (Prauss Citation2012a, Citation2012b).
The LO of Carpodinium obliquicostatum occurs in Bed 78 (BC14) at Pueblo. Although rare and sporadic in occurrence, its range top is reported to approximately coincide with that of A. tutulosum in Northern Europe (Marshall & Batten Citation1988; Dodsworth Citation1996, Citation2000).
4.4.2. The Isabelidinium magnum interval subzone
Defintion of subzone: From the first sample stratigraphically above the LO of consistent Adnatosphaeridium tutulosum (base of subzone) to the first sample stratigraphically below the FO of common Senoniasphaera turonica (top of subzone).
Depth range: Iona-1, 103.44 m to 59.88 m.
Outcrop range at Pueblo: Bed 79 (BC19) to Bed 115 (BC59).
Age of subzone: Late Cenomanian to Early Turonian (Colorado) or Middle Turonian (Texas; ca. 94.59 Ma to 91.72 Ma in Iona-1, ).
Discussion: Isabelidinium magnum and Isabelidinium? globosum are common from Bed 104 (BC46) to the top of the sampled section at Pueblo and there is an influx of prominent I. magnum from Bed 106 (BC49) to Bed 108 (BC52). The geographical extent of this influx is not known, as it was not reported in the other central KWIS sections analyzed by Harris and Tocher (Citation2003) and is not present in the SW Texas sections. By contrast, in the northern KWIS, e.g. Saskatchewan and Alberta (Davey Citation1970; Bloch et al. Citation1999), both taxa are abundant throughout the Upper Cenomanian to Middle Turonian interval. Along with Eurydinium glomeratum (see below), their general southward decline in numbers between Canada, Colorado and Texas probably reflects a cooler more northerly water-mass preference. In Northern Europe, there is a comparable decline in numbers of I. magnum between the northerly argillaceous Shetland Group and southerly calcareous Chalk Group (Costa & Davey Citation1992) while I.? globosum and E. glomeratum are rare or absent in European sections.
Supplementary correlative events include: The LO of Pterodinium cingulatum reticulatum occurs in Bed 79 (BC19) at Pueblo. It is common between Beds 64–65 (BC03) and Beds 76–77 (BC12). Clarke and Verdier (Citation1967) also noted a range top around the Cenomanian–Turonian boundary level on the Isle of Wight.
The FOs of Heterosphaeridium difficile, Florentinia buspina and Florentinia? torulosa are Lower Turonian biostratigraphical marker events in Northern Europe (Davey & Verdier Citation1976; Foucher Citation1980, Citation1981, Citation1983; Tocher & Jarvis 1987; Tocher in Jarvis et al. Citation1988; Costa & Davey Citation1992; FitzPatrick Citation1995; Pearce et al. Citation2003, Citation2009). At Pueblo, all three taxa are rare and sporadic in the I. magnum Interval Subzone. Heterosphaeridium difficile is first recorded in an isolated sample (BC36) from Bed 95 (Lower Turonian) but is more consistent in occurrence in the Middle Turonian, from Bed 122 (BC72). It is a common component of the younger (Coniacian–Santonian) Niobrara Formation in Colorado (unpubl. data). The taxon is first recorded from Middle Turonian deposits in Iona-1 (65.12 m) and Innes-1 (41.78 m). The FO of F. buspina at Pueblo has been recorded in Bed 101 by Harris and Tocher (Citation2003) and slightly higher, Bed 105 (BC48), in this study. Harris and Tocher (Citation2003) also recorded its FO in Bed 99 equivalent at Blue Point, Arizona, and Bed 102 equivalent at Bunker Hill, Kansas. It has not been recorded in the Eagle Ford sections in SW Texas. The FO of F.? torulosa was used as an intra-Turonian biozonal event by Fitzpatrick (Citation1995). It is sporadically recorded from Bed 102 (BC42) and above at Pueblo, at 24.24 m in Innes-1, and from 24.87 m to 10.75 m in Iona-1.
Heterosphaeridium spp., including H. conjunctum and H. heteracanthum, is common to abundant from Bed 90 (BC34) to Bed 116 (BC60) at Pueblo. The geographical extent of this influx is not known, as it was not reported in the other central KWIS sections analyzed by Harris and Tocher (Citation2003) and is not present in the SW Texas sections.
Rare and sporadic specimens of questionable Alterbidinium ioannidesii occur in Bed 100 (BC40) and above at Pueblo. Alterbidinium ioannidesii is a common component of the younger (Coniacian–Santonian) Niobrara Formation in Colorado (unpubl. data). It has not been recorded from the Eagle Ford Group or lower part of the Austin Chalk Formation in the SW Texas sections. Pearce (Citation2010) documented it from the Lower Campanian in Norfolk, eastern England.
The FO of Canningia senonica () occurs in Bed 102 (BC43) at Pueblo. Clarke and Verdier (Citation1967) first published the taxon from Santonian deposits on the Isle of Wight. They mainly differentiated it from the longer-ranging (Upper Cenomanian to Middle Turonian at Pueblo) type species Canningia reticulata by a higher reticulum. Santos et al. (Citation2018) considered it to be a junior synonym of C. reticulata, based on the co-occurrence of both taxa in Turonian deposits in NE Brazil, and suggested the morphological differences might be linked to palaeoenvironments. However, we retain C. senonica because it has a different (shorter) stratigraphical range from C. reticulata at Pueblo and in southern England (cf. Clarke & Verider 1967; Pearce et al. Citation2003, Citation2009).
At Pueblo, the FO of Eurydinium glomeratum occurs in Bed 102 (BC44; intra-Lower Turonian), with the FO of abundant E. glomeratum in Bed 112 (BC57). It has a comparable FO at Bunker Hill in Kansas (Bed 98 equivalent; Harris & Tocher Citation2003). It is common from a stratigraphically lower level, i.e. from the Upper Cenomanian, through to the Middle Turonian in Saskatchewan and Alberta, western Canada (Davey Citation1970; Bloch et al. Citation1999). In the southern KWIS, it has a Lower to Middle Turonian range in the relatively expanded, stratigraphically complete section in Iona-1 (98.88 m to 70.45 m; Eldrett et al. Citation2015a). It was not recorded at Lozier Canyon or Innes-1, where it may be missing in strata eroded in the Lower–Middle Turonian unconformity at the base of the Langtry Member. This correlates with a regional Lower–Middle Turonian hiatus identified throughout much of the KWIS (Ewing Citation2013) with an estimated duration of >2 million years at Lozier Canyon and Innes-1. The unconformity is much less intense (estimated duration of <0.2 million years) in the more distal Iona-1 core (duration estimates from Eldrett et al. Citation2015a, Citation2017).
The FO of Chatangiella spp. (rare, sporadic) was recorded from Bed 104 (BC47) at Pueblo in this study. Harris and Tocher (Citation2003) also reported an FO of Chatangiella spectabilis from a slightly lower stratigraphic level at Pueblo (Bed 101), Blue Point in Arizona (Bed 98 equivalent) and Bunker Hill in Kansas (Bed 95 equivalent). In SW Texas, it is also first recorded from the Lower Turonian in Iona-1 (74.50 m) and in proximity to the base Middle Turonian sedimentological pick in Innes-1 (43.42 m).
The FO of Membranilarnacia polycladiata occurs within the Lower Turonian at Pueblo (Bed 111, BC55) and Iona-1 (79.51 m), and within the Middle Turonian in Innes-1 (34.60 m). However, it was originally published from Albian deposits in Australia (Cookson & Eisenack Citation1958; Eisenack Citation1963) and has been recorded throughout the Cenomanian and Turonian in NW Germany (Prössl Citation1990).
4.4.3. The Senoniasphaera turonica interval subzone
Definition of subzone: From the FO of common Senoniasphaera turonica (base of subzone) to the first sample stratigraphically below the FO of common Senoniapshaera rotundata (top of subzone).
Depth range: Iona-1, 58.74 m to 40.35 m.
Outcrop range at Pueblo: Bed 116 (BC60) to Bed 125 (BC80; the highest sample analyzed).
Age of subzone: Early Turonian (Colorado) or Middle Turonian (Texas) to Late Turonian (ca. 91.72 Ma to 90.17 Ma in Iona-1, ).
Discussion: Pearce et al. (Citation2003, Citation2011) differentiated Senoniasphaera turonica from Senoniasphaera rotundata by the former’s possession of a relatively thickened ectophragm and greater ornament development on the ventral surface. Specimens close to the holotype of S. rotundata (Clarke & Verdier Citation1967, Plate 14, Figure 2) have relatively continuous reticulum ectophragm development across the dorsal surface. Both species exhibit a wide range of morphological variation (Prince et al. Citation1999; Pearce et al. Citation2011), particularly in regard to extent of the ectophragm reticulum coverage. Both species are present in the central and southern KWIS. Many KWIS specimens have been subjected to ectophragm bleaching caused by the laboratory oxidation applied to most samples, which in some cases hinders consistent and confident differentiation between the two species.
In Europe, S. turonica is occasionally recorded from Middle and Upper Cenomanian deposits (Pearce et al. Citation2011) but it shows a widespread FO consistent and FO common occurrence in intra-Lower or basal Middle Turonian deposits (Foucher Citation1980; Tocher & Jarvis 1987; Jarvis et al. Citation1988; Lamolda & Mao Citation1999; Pearce et al. Citation2003, Citation2009). Within the Turonian at Banterwick Barn, Berkshire, southern England, two main occurrences of S. turonica were identified by Pearce et al. (Citation2003, Citation2011): An intra-Lower to Middle Turonian interval, separated from an intra-Middle to Upper Turonian interval by a section in which the taxon is not recorded. The intra-Lower to Middle Turonian occurrence, between the ‘Lulworth’ and ‘Round Down’ marl beds, which can be correlated with Portland-1 and Iona-1 on carbon isotope criteria (), shows a comparable distribution to that at Pueblo where FO consistent (Bed 113, BC58) and FO common S. turonica (Bed 116, BC60) occur above the OAE-2 isotope event, within the upper part of the Lower Turonian. In Texas, FO consistent and FO common S. turonica occur at a higher stratigraphical level, at the ‘Pewsey’ carbon isotope event, as calibrated in Iona-1 (Eldrett et al. Citation2015a) and Innes-1 cores (cf. and ), possibly correlating with the base of the intra-Middle to Upper Turonian occurrence at Banterwick Barn.
We follow the definition of Olde et al. (Citation2015a) for the base of the S. turonica Interval Subzone as the FO of common S. turonica but acknowledge that it is picked at different stratigraphical levels in Colorado and Texas, as discussed above. For central and southern KWIS, we differ from Olde et al. (Citation2015a) in placing the top of the subzone at the FO of common S. rotundata.
Supplementary correlative events include: The LO of common Cauveridinium membraniphorum occurs in Bed 120 (BC64) at Pueblo and 72.77 m in Iona-1. It provides a useful regional proxy for the Lower–Middle Turonian boundary.
The FO of abundant Trithyrodinium suspectum/spp. occurs in Bed 121 (BC66) at Pueblo, an event of potential correlative value within the central KWIS. However, in SW Texas, the genus Trithyrodinium is common to abundant at several levels throughout the Middle Cenomanian to Upper Turonian section (Eldrett et al. Citation2015a; Dodsworth Citation2016).
At Pueblo, a single specimen of questionable Raphidodinium fucatum was recorded in Bed 124 (BC74), a dinoflagellate cyst taxon with a Middle Turonian range base in Northern Europe (Costa & Davey Citation1992).
4.4.4. The Senoniasphaera rotundata interval subzone
Definition of subzone: From the FO of common Senoniapshpaera rotundata (base of subzone) to the higher of either the LO of Cauveridinium membraniphorum or the LO of Isabelidinium magnum (top of subzone).
Depth range: Iona-1, 37.89 m to 7.91 m (the shallowest rich recovery core sample analyzed).
Age of subzone: Late Turonian to Early Coniacian (ca. 90.17 Ma to 89.45 Ma in Iona-1, ).
Discussion: Although rare specimens of Senoniasphaera rotundata are recorded in the Bridge Creek Member at Pueblo (from Bed 113, BC58), and the upper Eagle Ford Group in Iona-1 and Innes-1, they are relatively consistent and common in the Austin Chalk in the latter localities (). In Colorado, they are common in the (Coniacian–Santonian) Niobrara Formation (unpubl. data). At Lozier Canyon, a single sample with common S. rotundata occurs at a stratigraphically lower level than in Iona-1 and Innes-1, within the Langtry Member of the upper Eagle Ford Group (Dodsworth Citation2016) but still within the Upper Turonian. The KWIS observations are in agreement with the those of Pearce et al. (Citation2003, Citation2011), who noted that specimens close to the holotype of S. rotundata are more typical of Upper Turonian and Coniacian deposits at Banterwick Barn in England, although the genus is mainly represented by S. turonica in Lower and Middle Turonian deposits there. Pearce et al. (Citation2003) reported an overlap within Upper Turonian deposits of the common occurrences of S. rotundata and S. turonica. Notwithstanding the issues of consistent identification and potentially diachronous basal common occurrence within the Upper Turonian in the KWIS, we propose an S. rotundata Subzone, defined by the FO of its common occurrence.
The LO of Cauveridinium membraniphorum occurs in the Lower Coniacian in Europe (see Olde et al. Citation2015a for a review), though it is relatively rare and sporadic above the top of the Turonian Stage, which has given rise to its LO being alternatively reported as top Turonian by some European workers (Costa & Davey Citation1992). Cauveridinium membraniphorum is present in some Austin Chalk core samples analyzed from Iona-1 (LO at 10.75 m) and Innes-1 (LO at 25.98 m). The alternative bioevent for the top of the zone/subzone is LO Isabelidinium magnum, which also has a Lower Coniacian LO in Northern Europe (Costa & Davey Citation1992) and is recorded in shallower rich recovery core samples in Iona-1 (7.91 m) and Innes-1 (17.45 m) and the highest outcrop sample at Lozier Canyon (57.15 m). This supports an age not younger than Early Coniacian for the sampled lower part of the Austin Chalk, in agreement with macrofossil evidence for an Austin Chalk age not younger than Coniacian (Cobban et al. Citation2008) and the astronomical age model for the Austin Chalk from Iona-1 that places the base Coniacian at ca. 21 m depth; with the FO of the calcareous nannofossil Marthasterites furcatus Bukry, 1969 at 18.67 m (Eldrett et al. Citation2015a). Note that the subsequent assignment of the ‘Navigation’ carbon isotope event to the negative δ13C excursion overlying the Hitchwood/Late Turonian-Coniacian events in Iona-1 (ca. 44 m; Eldrett et al. Citation2017) is not consistent with this base Coniacian age assignment and therefore may more accurately represent a negative isotopic shift within the Late Turonian Hitchwood event ().
5. Discussion
5.1. The Lower–Middle Turonian substage boundary at Pueblo
Although a regional unconformity is developed around the Lower–Middle Turonian boundary interval over much of the southern and central KWIS (see Section 4.4.2), Kennedy et al. (Citation2000) noted that there is no macrofossil or sedimentary evidence in the pelagic, climatically driven, rhythmically bedded sequence at Pueblo for stratigraphic gaps or for condensation in proximity to the proposed substage boundary level, Bed 120. This is also the case with palynology, as there are no sudden changes in assemblage composition around Bed 120. However, there is an increase in areoligeracean dinoflagellate cyst taxa, mainly abundant Tenua hystrix, from Bed 116 to basal Bed 121 (Section 3.1), spanning the proposed boundary. Areoligeraceae may have a relatively nearshore affinity (Brinkhuis & Zachariasse Citation1988; Harker et al. Citation1990, p. 202–204; Li & Habib Citation1996; Olde et al. Citation2015b) and their influx could reflect an interval of relatively low sea level. This interpretation is supported by a minor influx of reworked dinoflagellate cysts probably derived from Albian to Lower Cenomanian deposits in Beds 121 and 122 (Section 3.2). Higher palynomorph concentration in Beds 121 to 125 (Section 3.1), including >1000 gymnosperm pollen c.p.g. in many samples (; Supplement), could also be considered consistent with greater dilution of biogenic carbonate by an increased supply of terrigenous mud during deposition of the relatively argillaceous Middle Turonian part of the Bridge Creek Member. A broad increase in prasinophyte relative and absolute abundance from Bed 98 to Bed 125 (; Supplement) may reflect sea-level highstand and subsequent fall after maximum Greenhorn transgression (cf. Prauss Citation1993, Citation2000).
5.2. Comparison with the Western Canada sedimentary basin
The upper part of the Belle Fourche Formation and the Second White Specks Formation in Saskatchewan and Alberta, western Canada, contain palynological assemblages that are comparable to those of intra-Early to Middle Turonian age in this study (Section 4.4.2), including abundant Isabelidinium magnum, Isabelidinium? globosum and Eurydinium glomeratum, and Heterosphaeridium difficile, which is rare in the upper Belle Fourche but can become abundant in the Second White Specks (Bloch et al. Citation1999). These deposits were tentatively dated as Late Cenomanian to early Middle Turonian in age by Bloch et al. (Citation1999) but our calibration to formal substages suggests that their age range may be younger, i.e. intra-Early to Middle Turonian. Alternatively, common occurrences of these Boreal taxa at Pueblo may reflect much later migration into the central KWIS than their earliest common occurrences in western Canada, as suggested in Section 4.4.2.
6. Conclusions
A dinoflagellate cyst zonation is proposed for the Western Interior of the USA, comprising three zones and four subzones. In the Iona-1 (SW Texas) and Portland-1 (Colorado) cores, the zonation is calibrated by radiometric dates from bentonites and astronomical age modeling of the inter-bedded sedimentary strata. Available published biostratigraphical data from molluscs, foraminifera and calcareous nannofossils, and inter-regional carbon isotope stratigraphy, further constrain the zonation at the Pueblo GSSP outcrop (Colorado) and three other sections in SW Texas.
Although the zonation provides a working scheme that is potentially applicable to marine sections throughout the Western Interior, regional differences in ranges of several taxa are recognized. Some diachronous dinoflagellate cyst events probably followed incursion of proto-Atlantic Tethyan Realm water-masses from south to north; onset Bosedinia abundance during the Middle Cenomanian in Texas pre-dates that in Colorado (Late Cenomanian). Conversely, some events probably followed Boreal water-masses southwards; onset Isabelidinium/Eurydinium abundance in Canada (Late Cenomanian?) may pre-date that in Colorado (Early Turonian).
The base of the Senoniasphaera turonica Interval Subzone cannot be treated as an isochronous level across the central and southern KWIS. It occurs in the upper part of the Lower Turonian at the Pueblo GSSP but is stratigraphically higher, in the upper part of the Middle Turonian, in the SW Texas cores Iona-1 and Innes-1. It is possible that S. turonica migration into the region occurred in two main pulses (as seen in Banterwick Barn, England), only the later event reaching Texas.
Pueblo_Chart_-_Supplement-edited_20180508.pdf
Download PDF (7.9 MB)Acknowledgements
The late Erle G. Kauffman (University of Colorado at Boulder) kindly supplied the rock samples from Pueblo. We thank our Shell colleagues for internal review and Shell Exploration and Production Inc. for permission to publish. James B. Riding (editor), Martin A. Pearce (reviewer) and an anonymous reviewer made helpful suggestions for improving the manuscript.\
Disclosure statement
No potential conflict of interest was reported by the authors.
Additional information
Notes on contributors
Paul Dodsworth
PAUL DODSWORTH has worked as full-time biostratigraphical consultant to the oil and gas industry since 1996. He founded StrataSolve Ltd in 2006. His expertise mainly focuses on the Upper Jurassic, Cretaceous and Cenozoic palynology and stratigraphy of Europe, North and South America, the Middle East and Africa. He has analyzed over 90 offshore wells at site, mainly Eocene–Paleocene sections in the Central North Sea and West of Shetland Isles (UK Continental Shelf).
James S. Eldrett
JAMES S. ELDRETT joined Shell Exploration and Production in 2006 as a biostratigrapher. Between 2011 and 2015, James worked in the Integrated Geoscience Research Team based in Houston, US and subsequently with the Regional Geology team in Rijswijk, the Netherlands. His research covers all aspects of global geology, specialising in stratigraphy and geochemistry. The underlying research materials for this article can be accessed at.
References
- Akyuz I, Warny S, Famubode O, Bhattacharya JP. 2016. Palynology of the Upper Cretaceous (Turonian) Ferron Sandstone Member, Utah, USA: identification of marine flooding surfaces and Milankovitch cycles in subtropical, ever-wet, paralic to non-marine palaeoenvironments. Palynology. 40:122–136.
- Århus N. 1991. Dinoflagellate cyst stratigraphy of some Aptian and Albian sections from North Greenland, southeastern Spitsbergen and the Barents Sea. Cretac Res. 12:209–225.
- Aurisano RW. 1989. Upper Cretaceous dinoflagellate biostratigraphy of the subsurface Atlantic coastal plain of New Jersey and Delaware. Palynology. 13:143–179.
- Backhouse J. 2006. Albian (Lower Cretaceous) dinoflagellate cyst biostratigraphy of the Lower Gearle Siltstone, southern Carnarvon Basin, Western Australia. Palynology. 30:43–68.
- Batt R. 1993. Ammonite morphotypes as indicators of oxygenation in a Cretaceous epicontinental sea. Lethaia. 26:49–63.
- Beach TL. 1985. Woodbine Palynofacies – their relationship to Tuscaloosa of Gulf Coast. Abstract. The AAPG/Datapages Combined Publications Database. AAPG Bull. 69:237.
- Bengtson P, Cobban WA, Dodsworth P, Gale AS, Kenndey WJ, Lamolda MA, Matsumoto T, Reyment RA, Seibertz E, Tröger K-A. 1996. The Turonian stage and substage boundaries. In: Rawson PF et al. (Eds.). Proceedings of the Second International Symposium on Cretaceous Stage Boundaries; Sep 8–16; 1995; Brussels. Bruxelles: l'Institut Royal des Sciences Naturelles; p. 69–79. (Bulletin de l'Institut Royal des Sciences Naturelles de Belgique – Sciences de la Terre; No. 66).
- Bloch JD, Schroder-Adams CJ, Leckie DA, Craig J, McIntyre DJ. 1999. Sedimentology, micropaleontology, geochemistry, and hydrocarbon potential of shale from the Cretaceous Lower Colorado Group in western Canada. (Geological Survey of Canada, Bulletin; vol. 531). Canada, ISBN No. 0-660-17729-3.
- Bowman AR, Bralower TJ. 2005. Paleoceanographic significance of high-resolution carbon isotope records across the Cenomanian-Turonian boundary in the Western Interior and New Jersey coastal plain, USA. Mar Geol. 217:305–321.
- Bralower TJ. 1988. Calcareous nannofossil biostratigraphy and assemblages at the Cenomanian–Turonian boundary interval: implications for the origin and timing of oceanic anoxia. Paleoceanography. 3:275–316.
- Bralower TJ, Bergen JA. 1998. Cenomanian–Santonian calcareous nannofossil biostratigraphy of a transect of cores drilled across the western interior seaway. In: Dean WE & Arthur MA, editors. Stratigraphy and paleoenvironments of the Cretaceous western interior seaway, USA. Tulsa (OK): SEPM; p. 59–77. (SEPM, Concepts in Sedimentology and Paleontology; vol. 6).
- Brideaux WW, McIntyre DJ. 1975. Miospores and microplankton from Aptian–Albian rocks along Horton River, Ottawa, Canada: District of Mackenzie. p. 1–85. (Geological Survey of Canada, Bulletin; vol. 252). pl. 1–14.
- Brinkhuis H, Zachariasse WJ. 1988. Dinoflagellate cysts, sea level changes and planktonic foraminifers across the Cretaceous–Tertiary boundary at El Haria, N.W. Tunisia. Mar Micropaleontol. 13:153–191.
- Brown CW, Pierce RL. 1962. Palynologic correlations in Cretaceous Eagle Ford Group, northeast Texas. Am Assoc Pet Geol Bull. 46:2133–2147.
- Bujak JP, Davies EH. 1983. Modern and fossil Peridiniineae. Dallas Tex: The American Association of Stratigraphic Palynologists Foundation. (AASP Contribution Series; vol. 13). ISSN 0160-8843.
- Burgess JD. 1971. Palynological Interpretation of frontier environments in central Wyoming. Geoscience Man. 3:69–82.
- Caron M, Dall’Agnolo S, Accarie H, Barrera E, Kauffman EG, Amédro F, Robaszynski F. 2006. High-resolution stratigraphy of the Cenomanian-Turonian boundary interval at Pueblo (USA) and Wadi Bahloul (Tunisia): stable isotope and bio-events correlation. Geobios. 39:171–200.
- Christopher RA. 1982. The Occurrence of the Complexiopollis–Atlantopollis Zone (Palynomorphs) in the Eagle Ford Group (Upper Cretaceous) of Texas. J Paleontol. 56:525–541.
- Clarke RFA, Verdier J-P. 1967. An investigation of microplankton assemblages from the Chalk of Isle of Wight, England. Verhandelingen der Koninklijke Nederlandsche Akademie van Wetenschappen, Afdeling Natuurkunde, Eerste Reeks 24:1–96.
- Cobban WA. 1984. Mid-Cretaceous ammonite zones, Western Interior, United States. Bull. Geol. Soc. Denmark. 33:71–89.
- Cobban WA. 1993. Diversity and distribution of Late Cretaceous ammonites, Western Interior, U.S. In: Kauffman EG, Caldwell WGE, editors. Evolution of the Western Interior Basin. p. 435–452. (Geological Association of Canada Special Paper; vol. 39). St Johns, NL, Canada.
- Cobban WA, Hook SC, McKinney KC. 2008. Upper Cretaceous molluscan record along a transect from Virden, New Mexico, to Del Rio, Texas. New Mexico Geology. 30:75–92.
- Cookson IC, Eisenack A. 1958. Microplankton from Australian and New Guinea Upper Mesozoic sediments. Proc R Soc Vic. 70. p. 19–78; pl.1–12.
- Cookson IC, Eisenack A. 1962. Additional micrplankton from Australian Cretaceous sediments. Micropalaeontology. 8:485–507.
- Cookson IC, Hughes NF. 1964. Microplankton from the Cambridge Greensand (Mid-Cretaceous). Palaeontology. 7:37–59.
- Cornell WC. 1997. Dinoflagellate cysts from the Buda Limestone (Cenomanian), Cerro de Cristo Rey, Dona Ana County, New Mexico. Rev Palaeobot Palynol. 98:153–157.
- Costa LI, Davey RJ. 1992. Dinoflagellate cysts of the Cretaceous System. In, Powell, AJ, editor. A Stratigraphic Index of Dinoflagellate Cysts. London: Chapman & Hall.
- Courtinat B, Crumière J-P, Méon H, Schaaf A. 1991. Les associations de kystes de dinoflagellés du Cénomanien-Turonien de Vergons (Bassin Vocontien France). Geobios. 24:649–666.
- Courtinat B. 1993. The significance of palynofacies fluctuations in the Greenhorn Formation (Cenomanian-Turonian) of the Western Interior basin, USA. Mar Micropaleontol. 21:249–257.
- Davies EH, Bujak JP, Williams GL. 1982. The application of dinoflagellates to paleoenvironmental problems. Proceedings of the Third North American Paleontological Convention. Vol. 1. Canada: Geological Survey of Canada. 123–131.
- Davey RJ. 1969. Non-calcareous microplankton from the Cenomanian of England, northern France and North America, Part I. Bulletin of the British Museum (Natural History). Geology. 17:3107–3180.
- Davey RJ. 1970. Non-calcareous microplankton from the Cenomanian of England, northern France and North America, Part II. Bull Br Mus Nat Hist Geol. 18:333–397.
- Davey RJ, Verdier J-P. 1971. An investigation of microplankton assemblages from the Albian of the Paris Basin. Verhandlingen der Koninklijke Nederlanse Akademie van Wetenschappen. Afdeling Natuurkunde, Eerste Reeks. 26:pl. 1–7.
- Davey RJ, Verdier JP. 1976. A review of certain non-tabulate Cretaceous hystrichosphaerid dinocysts. Rev Palaeobot Palynol. 22:307–335.
- Davey RJ, Williams GL. 1966. IV. The genera Hystrichosphaera and Achomosphaera. In: Davey RJ, Downie C, Sarjeant WAS, Williams GL, editors. Studies on Mesozoic and Cainozoic dinoflagellate cysts. London: British Museum; 28–52.
- Denne RA, Hinote RE, Breyer JA, Kosanke TH, Lees JA, Engelhardt-Moore N, Spaw JM, Tur N. 2014. The Cenomanian–Turonian Eagle Ford Group of South Texas: insights on timing and paleoceanographic conditions from geochemistry and micropaleontologic analyses. Palaeogeogr Palaeoclimatol Palaeoecol. 413:2–28.
- Du Vivier ADC, Selby D, Sageman BB, Jarvis I, Gröcke DR, Voigt S. 2014. Marine 187Os/188 Os isotope stratigraphy reveals the interaction of volcanism and ocean circulation during Oceanic Anoxic Event 2. Earth Planet Sci Lett. 389:23–33.
- Dodsworth P. 1995. A note of caution concerning the application of quantitative palynological data from oxidized preparations. J Micropalaeontol. 14:6.
- Dodsworth P. 1996. Stratigraphy, microfossils and depositional environments of the lowermost part of the Welton Chalk Formation (late Cenomanian to early Turonian, Cretaceous) in eastern England. Proc Yorks Geol Soc. 51:45–64.
- Dodsworth P. 2000. Trans-Atlantic dinoflagellate cyst stratigraphy across the Cenomanian-Turonian (Cretaceous) Stage boundary. J Micropalaeontol. 19:69–84.
- Dodsworth P. 2004(a). The palynology of the Cenomanian-Turonian (Cretaceous) boundary succession at Aksudere in Crimea, Ukraine. Palynology. 28:129–141.
- Dodsworth P. 2004(b). The distribution of dinoflagellate cysts across a Late Cenomanian carbon isotope (δ13C) anomaly in the Pulawy borehole, central Poland. J Micropalaeontol. 23:77–80.
- Dodsworth P. 2016. Palynostratigraphy and palaeoenvironments of the Eagle Ford Group (Upper Cretaceous) at the Lozier Canyon outcrop reference section, west Texas, USA. Palynology. 40:357–378.
- Donovan AD, Staerker TS. 2010. Sequence stratigraphy of the Eagle Ford (Boquillas) Formation in the subsurface of South Texas and outcrops of West Texas. Gulf Coast Assoc Geol Soc Trans. 60:861–899.
- Donovan AD, Staerker TS, Pramudito A, Weiguo L, Corbett M, Lowery CM, Romero AM, Gardner RD. 2012. The Eagle Ford outcrops of West Texas: a laboratory for understanding heterogeneities within unconventional mudstone reservoirs. GCAGS J. 1:162–185.
- Duxbury S. 2001. A palynological zonation scheme for the Lower Cretaceous – United Kingdom Sector, Central North Sea. N Jb Geol Paläont Abh. 219:95–137.
- Eicher DL, Diner R. 1985. Foraminifera as indicators of water mass in the Cretaceous Greenhorn Sea, Western Interior. In: Pratt LM, Kauffman EG, Zelt FB, editors. Fine-Grained Deposits of Cyclic Sedimentary Processes. p. 60–71. (Society of Economic Paleontologists and Mineralogists Guidebook; 4). Tulsa, OK.
- Eicher DL, Worstell P. 1970. Cenomanian and Turonian foraminifera from the Great Plains, United States. Micropaleontology. 16:269–324.
- Eisenack A. 1963. Zur Membranilarnax-Frage. Neues Jahrb Geol Paläontol Monatsh. 2:98–103.
- Elder WP. 1987. The paleoecology of the Cenomanian–Turonian (Cretaceous) stage boundary extinction at Black Mesa, Arizona. Palaios. 2:24.
- Elder WP. 1989. Molluscan extinction patterns across the Cenomanian–Turonian stage boundary in the Western Interior of the United States. Paleobiology. 15:299–320.
- Elderbak K, Leckie RM. 2016. Paleocirculation and foraminiferal assemblages of the Cenomanian-Turonian Bridge Creek Limestone bedding couplets: productivity vs. dilution during OAE2. Cretac Res. 60:52–77.
- Eldrett JS, Minisini D, Bergman SC. 2014. Decoupling of the carbon cycle during Oceanic Anoxic Event 2. Geology. 42:567–570.
- Eldrett JS, Ma C, Bergman SC, Lutz B, Gregory JG, Dodsworth P, Phipps M, Hardas P, Minisini D, Ozkan A, et al. 2015(a). An astronomically calibrated stratigraphy of the Cenomanian, Turonian and earliest Coniacian from the Cretaceous Western Interior Seaway, USA: implications for global chronostratigraphy. Cretac Res. 56:316–344.
- Eldrett JS, Ma C, Bergman SC, Ozkan A, Minisini D, Lutz B, Jackett SJ, Macaulay C, Kelly AE. 2015(b). Origin of limestone–marlstone cycles: astronomic forcing of organic-rich sedimentary rocks from the Cenomanian to early Coniacian of the Cretaceous Western Interior Seaway, USA. Earth Planet Sci Lett. 423:98–113.
- Eldrett JS, Dodsworth P, Bergman SC, Wright M, Minisini D. 2017. Water-mass evolution in the Cretaceous Western Interior Seaway of North America and equatorial Atlantic. Clim Past. 13:855–878.
- Ewing T. 2013. Eagle Ford-Colorado Connection: Cenomanian to Coniacian in Southwestern North America. AAPG Search Discovery Article ID: 30288
- Fitzpatrick MEJ. 1995. Dinoflagellate cyst biostratigraphy of the Turonian (Upper Cretaceous) of southern England. Cretac Res. 16:757–791.
- Forster A, Schouten S, Moriya K, Wilson PA, Sinninghe Damsté JS. 2007. Tropical warming and intermittent cooling during the Cenomanian/Turonian oceanic anoxic event 2: sea surface temperature records from the equatorial Atlantic. Paleoceanography. 22. doi: 10.1029/2006PA001349.
- Foucher J-C. 1979. Distribution stratigraphique des kystes de dinoflagellés et des acritarches dans le Crétacé Supérieur du Bassin de Paris et de l’Europe septentrionale. Palaeontographica Abt B. 169:78–105.
- Foucher J-C. 1980. Dinoflagellés et acritarches dans le Crétacé du Bolonnais. Pp. 233, 288–297, 310–311 in Robaszynski et al., Synthèse biostratigraphique de l’Aptien au Santonien du Boulonnais à partir de sept groups paléontologiques: Foraminifères, nannoplancton, dinoflagellés et macrofaunes – Zonations micropaléontologiques integrées dans le cadre du Crétacé boreal nord-européen. Revue de Micropaléontologie 22(4):195–321.
- Foucher J-C. 1981. Kystes de dinoflagellés dans le Crétacé Moyen Européen: Proposition d’une Echelle Biostratigraphique pour le Domaine Nord-occidental. Cretac Res. 2:331–338.
- Foucher J-C, 1982. Dinoflagelles et Acritarches du Turonien stratotypique (affleurments du Saumurois, sondage de Civray-de-Touraine). Pp. 147–150, 171–173, 176 in Robaszynski F, Alcaydé G, Amedro F, Badillet G, Damotte R, Foucher J-C, Jardiné S, Legoux O, Manvit H, Monciardini C, Sornay J. Turonien de la Region-type: Saumurois et Touraine. Stratigraphie, biozonations, sédimentologie. Bull Centres Rech Explor – Prod. Elf-Aquitaine 6.
- Foucher J-C. 1983. Distribution des kystes de dinoflagellés dans le Crétacé Moyen et Supérieur du Bassin de Paris. Cahiers de Micropaleontologie 4:23–41.
- Gale AS, Jenkyns HC, Kennedy WJ, Corfield RM. 1993. Chemostratigraphy versus biostratigraphy: data from around the Cenomanian-Turonian boundary. J Geol Soc Lond. 150:29–32.
- Gale AS, Christensen WK. 1996. Occurrence of the belemnite Actinocamax plenus in the Cenomanian of SE France and its significance. Bulletin of the Geological Society of Denmark. 43:68–77.
- Gustason ER, Kauffman EG. 1985. The Dakota Group and the Kiowa-Skull Creek Cyclothem in the Canyon City – Pueblo area. In: Pratt LM, Kauffman EG, Zelt FB, editors. Fine-grained Deposits of Cyclic Sedimentary Processes. p. 72–89. (Society of Economic Paleontologists and Mineralogists Guidebook; 4). Tulsa, OK.
- Hancock JM. 1991. Ammonite scales for the Cretaceous System. Cretac Res. 12:259–291.
- Harker SD, Gustav SH, Riley LA. 1987. Triassic to Cenomanian stratigraphy of the Witch Ground Graben. In, Brooks SJ, Glennie K, editors. Petroleum Geology of North-West Europe. London: Graham & Trotman; p. 809–818.
- Harker SD, W.A.S S, Caldwell WGE. 1990. Late Cretaceous (Campanian) organic-walled microplankton from the Interior Plains of Canada, Wyoming and Texas: biostratigraphy, palaeontology and environmental interpretation. Palaeontographica Abt B. 219:1–243.
- Harland R. 1988. Dinoflagellates, their cysts and Quaternary stratigraphy. New Phytol. 108:111–120.
- Harland R, Reid PC, Dobell P, Norris G. 1980. Recent and sub-Recent dinoflagellate cysts from the Beaufort Sea, Canadian Arctic. Grana. 19:211–225.
- Harries PJ. 1993. Dynamics of survival following the Cenomanian–Turonian (Upper Cretaceous) mass extinction event. Cretac Res. 14:563–583.
- Harries PJ, Kauffman EG. 1990. Patterns of survival and recovery following the Cenomanian–Turonian (Late Cretaceous) mass extinction in the Western Interior Basin, United States. In Kauffman EG, Walliser OH, editors. Extinction Events in Earth History. Berlin: Springer; p. 277–298. (Lectute Notes in Earth History; 30).
- Harris AJ, Tocher BA. 2003. Palaeoenvironmental analysis of Late Cretaceous dinoflagellate cyst assemblages using high-resolution sample correlation from the Western Interior Basin, USA. Mar Micropaleontol. 48:127–148.
- Hart MB, W, P.P.E Clements RG, Burnett JA, Tocher BA, Batten DJ, Lister JK, MacLennan AM. 1987. The Isle of Wight. Cretaceous. In Lord AR, Bown PR, editors. Mesozoic and Cenozoic Stratigraphical Micropalaeontology of the Dorset Coast and Isle of Wight. Southern England: British Micropalaeontological Society; p. 88–149. (British Micropalaeontological Society Guide Book; 1).
- Hasegawa T, Crampton JS, Schiøler P, Field B, Fukushi C, Kakizaki Y. 2013. Carbon isotope stratigraphy and depositional oxia through Cenomanian/Turonian boundary sequences (Upper Cretaceous) in New Zealand. Cretac Res. 40:61–80.
- Hattin DE. 1971. Widespread, synchronously deposited, burrow mottled limestone beds in Greenhorn Limestone (Upper Cretaceous) of Kansas and Central Colorado. Am Assoc Pet Geol Bull. 55:412–431.
- Hattin DE. 1975. Stratigraphy and depositional environment of Greenhorn Limestone (Upper Cretaceous) of Kansas. (Kansas Geological Bull; 209). 128 p.
- Hattin DE. 1985. Distribution and significance of widespread, time-parallel pelagic limestone beds in Greenhorn Limestone (Upper Cretaceous) of the central Great Plains and southern Rocky Mountains. In: Pratt, L.M., Kauffman, E.G., Zelt, F.B. (Eds.) Fine-grained Deposits of Cyclic Sedimentary Processes. p. 28–37. (Society of Economic Paleontologists and Mineralogists Guidebook; 4). Tulsa, OK.
- Hay WW, Eicher DL, Diner R. 1993. Physical oceanography and water masses of the Cretaceous Western Interior Seaway. In: Caldwell WEG, Kauffman EG, editors. Evolution of the Western Interior Basin. p. 297–318. (Geological Association of Canada Special Paper; 39). St Johns, NL, Canada.
- Huber BT, Norris RD, MacLeod KG. 2002. Deep-sea paleotemperature record of extreme warmth during the Cretaceous. Geology. 30:123–126.
- Jarvis I, Carson GA, Cooper K, Hart MB, Horne D, Leary PN, Rosenfeld A, Tocher BA. 1988. Chalk microfossil assemblages and the Cenomanian–Turonian (late Cretaceous) oceanic anoxic event, new data from Dover, England. Cretac Res. 9:3–103.
- Jarvis I, Gale AS, Jenkyns HC, Pearce MA. 2006. Secular variation in Late Cretcaeous carbon isotopes: a new δ13C carbonate reference curve for the Cenomanian-Campanian (99.6–70.6 Ma). Geol Mag. 143:561–608.
- Jarvis I, Lignum JS, Gröcke DR, Jenkyns HC, Pearce MA. 2011. Black shale deposition, atmospheric CO2 drawdown, and cooling during the Cenomanian–Turonian Oceanic Anoxic Event. Paleoceanography. PA3201, 26. https://doi.org/10.1029/2010PA002081
- Jefferies RPS. 1963. The palaeoecology of the Actinocamax plenus Subzone (Lowest Turonian) in the Anglo-Paris Basin. Palaeontology. 4: 609–647.
- Jefferies RPS. 1963. The stratigraphy of the Actinocamax plenus Subzone (Turonian) in the Anglo-Paris Basin. Proceedings of the Geologists’ Association. 74:1–30.
- Jenkyns HC. 2010. Geochemistry of oceanic anoxic events. Geochem Geophys Geosyst. 11. doi: 10.1029/2009GC002788.
- Joo YJ, Sageman BB. 2014. Cenomanian to Campanian carbon isotope chemostratigraphy from the Western Interior Basin, U.S.A. J Sediment Res. 84:529–542.
- Kauffman EG. 1977. Geological and biological overview: Western Interior Cretaceous Basin. In Kauffman EG, editor. Cretaceous facies, faunas and paleoenvironments across the Western Interior Basin. Denver, Colorado: The Rocky Mountain Association of Geologists. The Mountain Geologist; p. 75–100.
- Kauffman EG. 1984(a). Paleobiogeography and evolutionary response dynamic in the Cretaceous Western Interior Seaway of North America. In Westermann, G.E.G. (Ed.) Jurassic–Cretaceous biochronology and paleogeography of North America. p. 273–306. (Geological Association of Canada Special Paper; 27).
- Kauffman EG. 1984(b). The fabric of marine extinctions. In Berggren WA, Van Couvering J, editors. Catastrophes and Earth History: The New Uniformitarianism. Princeton, NJ: Princeton University Press; p. 151–246.
- Kauffman EG. 1985. Cretaceous evolution of the Western Interior Basin of the United States. In Pratt LM, Kauffman EG, Zelt FB, editors. Fine-grained Deposits and Biofacies of the Cretaceous Western Interior Seaway: Evidence of Cyclic Sedimentary Processes. p. IV–XIII. (Society of Economic Paleontologists and Mineralogists, Guidebook 4). Tulsa, OK.
- Kauffman EG, Caldwell WGE. 1993. The Western Interior Basin in space and time. In: Kauffman EG, Caldwell WGE, editors. Evolution of the Western Interior Basin. p. 1–30. (Geological Association of Canada Special Paper 39). St Johns, NL, Canada.
- Kauffman EG, Pratt LM. 1985. A field guide to the stratigraphy, geochemistry, and depositional environments of the Kiowa-Skull Creek, Greenhorn, and Niobrara marine cycles in the Pueblo–Cañon city area, Colorado. In: Pratt LM, Kauffman EG, Zelt FB, editors. Fine-grained deposits of cyclic sedimentary processes. FRS/1–FRS/26. (Society of Economic Paleontologists and Mineralogists Guidebook 4).
- Keller G, Pardo A. 2004. Age and paleoenvironment of the Cenomanian-Turonian global stratotype section and point at Pueblo, Colorado. Mar Micropaleontol. 51:95–128.
- Kennedy WJ, Cobban WA. 1991. Stratigraphy and inter-regional correlation of the Cenomanian–Turonian transition in the Western Interior of the United States near Pueblo, Colorado: a potential boundary stratotype for the base of the Turonian stage. Newsl Stratigr. 24:1–33.
- Kennedy WJ, Gale AS. 2006. The Cenomanian Stage. Proc Geol Assoc. 117:187–205.
- Kennedy WJ, Gale AS, Bown PR, Caron M, Davey RJ, Gröcke D, Wray DS. 2004. The Global Boundary Stratotype Section and Point (GSSP) for the base of the Cenomanian Stage, Mont Risou, Hautes-Alpes, France. Episodes. 21:591–532.
- Kennedy WJ, Walaszczyk I, Cobban WA. 2000. Pueblo, Colorado, USA, Candidate Global Boundary Stratotype Section and Point for the base of the Turonian Stage of the Cretaceous and for the Middle Turonian substage, with a revision of the Inoceramidae (Bivalvia). Acta Geol Pol. 50:295–334.
- Kennedy WJ, Walaszczyk I, Cobban WA, Dodsworth P, Elder WP, Gale AS, Scott GR, Hancock JM, Voigt S, Kirkland JI. 2005. The Global Boundary Stratotype Section and Point for the base of the Turonian Stage of the Cretaceous: Pueblo, Colorado, U.S.A. Newsl Stratigr. 24:1–104.
- Kennedy WJ, Wright CW, Hancock JM. 1987. Basal Turonian ammonites from West Texas. Palaeontology. 30:27–74.
- Lamolda MA, Mao S. 1999. The Cenomanian-Turonian boundary event and dinocyst record at Ganuza (northern Spain). Palaeogeogr Palaeoclimatol Palaeoecol. 150:65–82.
- Lamolda MA, C.R.C P, Peryt D, Pons JM. 2014. The Global Boundary Stratotype and Section Point (GSSP) for the base of the Santonian Stage, ‘Cantera de Margas’, Olazagutia, Northern Spain. Episodes. 37:2–13.
- Leckie RM. 1985. Foraminifera of the Cenomanian-Turonian boundary interval, Greenhorn Formation, Rock Canyon anticline, Pueblo, Colorado. In: Pratt LM, Kauffman EG, Zelt FB, editors. Fine-grained deposits of cyclic sedimentary processes. p. 139–149. (Society of Economic Paleontologists and Mineralogists Guidebook 4). Tulsa, OK.
- Li H, Habib D. 1996. Dinoflagellate stratigraphy and its response to sea level change in Cenomanian-Turonian sections of the Western Interior of the United States. Palaios. 11:15–30.
- Lowery CM, Corbett MJ, Leckie RM, Watkins D, Romero AM, Pramudito A. 2014. Foraminifera and nannofossil paleoecology and paleoceanography of the Cenomanian-Turonian Eagle Ford Shale of southern Texas. Palaeogeogr Palaeoclimatol Palaeocol. 413:49–65.
- Lowery CM, Leckie RM, Bryant R, Elderbak K, Parker A, Polyak DE, Schmidt M, Snoeyenbos-West O, Sterzinar E. 2018. The Late Cretaceous Western Interior Seaway as a model for oxygenation change in epicontinental restricted basins. Earth Sci Rev. 177:545–564.
- Lynds RM, Slattery JS. 2017. Correlation of the Upper Cretaceous strata of Wyoming. Laramie, Wyoming: Wyoming State Geological Survey Open File Report 2017–3.
- Mao S, Lamolda MA. 1999. Quistes de dinoflagelados del Cenomaniense superior y Turoniense inferior de Ganuza, Navarra, II. – Biostatigafía. Revista Española de Paleontolgía, no. extr. Homenaje Al Prof. J. Truylos. 195–203.
- Marshall KL, Batten DJ. 1988. Dinoflagellate cyst associations in Cenomanian-Turonian ‘Black Shale’ sequences of northern Europe. Rev Palaeobot Palynol. 54:85–103.
- McMinn A. 1988. Outline of a Late Cretaceous dinoflagellate zonation of northwest Australia. Alcheringa. 12:137–156.
- Meyers SR. 2007. Production and preservation of organic matter: The significance of iron. Paleoceanography. 22, PA4211, https://doi.org/10.1029/2006PA001332.
- Minisini D, Eldrett JS, Bergman SC, Forkner R. 2018. Chronostratigraphic framework and depositional environments in the organic-rich, mudstone-dominated Eagle Ford Group, Texas, USA. Sedimentology. 65: 1520–1557.
- Morgan R. 1980. Palynostratigraphy of the Australian Early and Middle Cretaceous. Geological Survey of New South Wales. Palaeontol Mem. 18:1–153.
- Nichols DJ. 1994. A revised palynostratigraphic zonation of the non-marine Upper Cretaceous, Rocky Mountain region, United States. In Caputo MV, Peterson JA, Franczyk KJ, editors. Mesozoic systems of the Rocky Mountain region. Denver (CO): Rocky Mountain Section, SEPM; p. 503–521.
- Nøhr-Hansen H, Williams GL, Fensome RA. 2016. Biostratigraphic correlation of the western and eastern margins of the Labrador–Baffin Seaway and implications for the regional geology. Geol Surv Den Greenl Bull. 37:74.
- Núñez-Useche F, Canet C, Barragán R, Alfonso P. 2016. Bioevents and redox conditions around the Cenomanian–Turonian anoxic event in Central Mexico. Palaeogeography, Palaeoclimatology, Palaeoecology. 449:205–226.
- Odin GS, Lamaurelle MA. 2001. The global Campanian-Maastrichtian stage boundary. Episodes. 24:229–238.
- Ogg JG, Ogg GM, Gradstein FM. 2016. A Concise Geologic Time Scale 2016. Elsevier B.V. ISBN: 978-0-444-63771-0.
- Olde K, Jarvis I, Pearce M, Uličný D, Tocher B, Trabucho-Alexandre J, Gröcke D. 2015. A revised northern European Turonian (Upper Cretaceous) dinoflagellate biostratigraphy: integrating palynology and carbon isotope events. Rev Palaeobot Palynol. 213:1–15.
- Olde K, Jarvis I, Uličný D, Pearce MA, Trabucho-Alexandre J, Čech S, Gröcke DR, Laurin J, Švábenická L, Tocher BA. 2015. Geochemical and palynological sea-level proxies in hemipelagic sediments: a critical assessment from the Upper Cretaceous of the Czech Republic. Palaeogeogr Palaeoclimatol Palaeocol. 435:222–243.
- Olde K, Jarvis I, Pearce M, Walaszczyk I, Tocher B. 2016. Organic-walled dinoflagellate cyst records from a prospective Turonian – Coniacian (Upper Cretaceous) GSSP, Słupia Nadbrzezna, Poland. Cretac Res. 65:17–24.
- Pearce MA. 2010. New organic-walled dinoflagellate cysts from the Cenomanian to Maastrichtian of the Trunch borehole, UK. J Micropalaeontol. 29:51–72.
- Pearce MA, Jarvis I, Swan ARH, Murphy AM, Tocher BA, Edmunds WM. 2003. Integrating palynological and geochemical data in a new approach to palaeoecological studies: Upper Cretaceous of the Banterwick Barn Chalk borehole, Berkshire, UK. Mar Micropaleontol. 47:271–306.
- Pearce MA, Jarvis I, Tocher BA. 2009. The Cenomanian-Turonian boundary event, OAE2 and palaeoenvironmental change in epicontinental seas: new insights from the dinocyst and geochemical records. Palaeogeogr Palaeoclimatol Palaeocol. 280:207–234.
- Pearce MA, Lignum JS, Jarvis I. 2011. Senoniasphaera turonica (Prössl, 1990 ex Prössl, 1992) comb. nov., senior synonym of Senoniasphaera rotundata alveolata Pearce et al., 2003: an important dinocyst marker for the Lower Turonian chalk of NW Europe. J Micropalaeontol. 30:91–93.
- Pedersen GK, Nøhr-Hansen H. 2014. Sedimentary successions and palynoevent stratigraphy from the non-marine Lower Cretaceous to the marine Upper Cretaceous of the Nuussuaq Basin, West Greenland. Bull Can Petrol Geol. 62:261–244.
- Peyrot D. 2011. Late Cretaceous (Late Cenomanian-Early Turonian) dinoflagellate cysts from the Castilian Platform, northern Spain. Palynology. 35:267–300.
- Peyrot D, Barroso-Barcenilla F, Barrón E, Comas-Rengifo MJ. 2011. Palaeoenvironmental analysis of Cenomanian-Turonian dinocyst assemblages from the Castilian Platform (Northern-Central Spain). Cretac Res. 32:504–526.
- Peyrot D, Barroso-Barcenilla F, Feist-Burkhardt S. 2012. Palaeoenvironmental controls on late Cenomanian–early Turonian dinoflagellate cyst assemblages from Condemios (Central Spain). Rev Palaeobot Palynol. 180:25–40.
- Pratt LM. 1985. Isotopic studies of organic matter and carbonate rocks of the Greenhorn marine cycle. In: Pratt LM, Kauffman EG, Zelt FB, editors. Fine-grained Deposits of Cyclic Sedimentary Processes. p. 38–48. (Society of Economic Paleontologists and Mineralogists Guidebook 4). Tulsa, OK.
- Prauss ML. 1993. Sequence-palynology – Evidence from Mesozoic sections and conceptual framework. N Jb Geol Paläont (Abh). 190:143–163.
- Prauss ML. 2000. The oceanographic and climatic interpretation of marine palynomorph phytoplankton distribution from Mesozoic, Cenozoic and Recent sections. Göttinger Arbeiter Geol Paläontol. 75:1–235.
- Prauss ML. 2006. The Cenomanian/Turonian Boundary Event (CTBE) at Wunstorf, north-west Germany, as reflected by marine palynology. Cretac Res. 27:872–886.
- Prauss ML. 2007. Availability of reduced nitrogen chemospecies in photic-zone waters as the ultimate cause of fossil prasinophyte prosperity. Palaios. 22:489–499.
- Prauss ML. 2012.(a). The Cenomanian/Turonian Boundary event (CTBE) at Tarfaya, Morocco: palaeoecological aspects as reflected by marine palynology. Cretac Res. 34:233–256.
- Prauss ML. 2012.(b). The Cenomanian/Turonian Boundary Event (CTBE) at Tarfaya, Morocco, northwest Africa: eccentricity controlled water column stratification as major factor for total organic carbon (TOC) accumulation: evidence from marine palynology. Cretac Res. 37:246–260.
- Prauss ML. 2012.(c). Potential freshwater dinocysts from marine upper Cenomanian to upper Coniacian strata of Tarfaya, northwest Africa: three new species of Bosedinia. Cretac Res. 37:285–290.
- Prauss ML. 2015. Marine palynology of the Oceanic Anoxic Event 3 (OAE3, Coniacian-Santonian) at Tarfaya, Morocco, NW Africa – transition from preservation to production controlled accumulation of marine organic carbon. Cretac Res. 53:19–37.
- Prince IM, Jarvis I, Pearce MA, Tocher BA. 2008. Dinoflagellate cyst biostratigraphy of the Coniacian-Santonian (Upper Cretaceous): new data from the English Chalk. Rev Palaeobot Palynol. 150:59–96.
- Prince IM, Jarvis I, Tocher BA. 1999. High resolution dinoflagellate cyst biostratigraphy of the Santonian–basal Campanian (Upper Cretaceous): new data from Whitecliff, Isle of Wight, England. Rev Palaeobot Palynol. 105:143–169.
- Prössl KF. 1990. Dinoflagellaten der Kreide-Unter-Hauterive bis Ober-Turon - im niedersächsischen Becken. Stratigraphie und Fazies in der Kernbohrung Konrad 101 sowie einiger anderer Bohrungen in Nordwestdeutschland. Palaeontographica. Abteilung B. 218:93–191.
- Reichart GJ, Brinkhuis H. 2003. Late Quaternary Protoperidinium cysts as indicators of paleoproductivity in the northern Arabian Sea. Mar Micropaleontol. 49:303–370.
- Remane J, Bassett MG, Cowie JW, Gohrbandt KH, Lane HR, Michelsen O, Naiwen W. 1996. Revised guidelines for the establishment of global chronostratigraphic standards by the International Commission on Stratigraphy (ICS). Episodes. 19:77–81.
- Reolid M, Sánchez-Quiñónez CA, Alegret L, Molina E. 2015. Palaeoenvironmental turnover across the Cenomanian–Turonian transition in Oued Bahloul, Tunisia: foraminifera and geochemical proxies. Palaeogeogr Palaeoclimatol Palaeoecol. 417:491–510.
- Sageman BB. 1985. High-resolution stratigraphy and paleobiology of the Hartland Shale Member: analysis of an oxygen-deficient epicontinental sea. In: Pratt LM, Kauffman EG, Zelt FB, editors. Fine-grained Deposits of Cyclic Sedimentary Processes. p. 110–121. (Society of Economic Paleontologists and Mineralogists Guidebook 4). Tulsa, OK.
- Sageman BB, Johnson CC. 1985. Stratigraphy and Paleobiology of the Lincoln Limestone Member, Greenhorn Limestone, Rock Canyon Anticline, Colorado. In: Pratt LM, Kauffman EG, Zelt FB, editors. Fine-grained Deposits of Cyclic Sedimentary Processes. p. 100–109. (Society of Economic Paleontologists and Mineralogists Guidebook 4). Tulsa, OK.
- Sageman BB, Meyers SR, Arthur MA. 2006. Orbital timescale for the Cenomanian-Turonian boundary stratotype and OAE II, central Colorado, USA. Geology. 34:125–128.
- Santos A, Helenes J, de Araujo Carvalho M. 2018. Late Cretaceous (early Turonian) dinoflagellate cysts from the Sergipe Basin, northeastern Brazil. Palynology. doi: 10.1080/01916122.2017.1402099.
- Schiøler P, Crampton JS. 2014. Dinoflagellate biostratigraphy of the Arowhanan Stage (upper Cenomanian-lower Turonian) in the East Coast Basin, New Zealand. Cretac Res. 48:205–224.
- Schiøler P, Wilson GJ. 1993. Maastrichtian dinoflagellate zonation in the Dan Field, Danish North Sea. Rev Palaeobot Palynol. 78:321–351.
- Schlanger SO, Arthur MA, Jenkyns HC, Scholle PA. 1987. The Cenomanian-Turonian oceanic anoxic event, 1: Stratigraphy and distribution of organic carbon–rich beds and the marine excursion. Geological Society Special Publication. 26:371–399.
- Schlanger SO, Jenkyns HC. 1976. Cretaceous oceanic anoxic events: causes and consequences. Geologie En Mijnbouw. 55:179–184.
- Schwab KA, Bayliss GS, Smith MA, Yoder NB. 2011. Placoderm Desmid-like Microalgae in outcrop samples collected from the lower Eagle Ford Shale of Terrell and Val Verde Counties, Texas. AAPG Search and Discovery. Article ID: 50523
- Scott GR. 1964. Geology of the Northwest and Northeast Pueblo Quadrangles, Colorado. United States Geological Survey Miscellaneous Geological Investigations Map. Washington (DC).
- Scott RW, Oboh-Ikuenobe FE, Benson Jr DG, Holbrook JM, Alnahwi A. 2018. Cenomanian-Turonian flooding cycles: U.S. Gulf Coast and Western Interior. Cretac Res. doi: 10.1016/j.cretres.2018.03.027.
- Singh C. 1983. Cenomanian microfloras of the Peace River area, northwestern Alberta. Alberta Research Council. Bulletin. 44:317.
- Slingerland R, Kump LR, Arthur MA, Fawcett PJ, Sageman BB, Barron EJ. 1996. Estuarine circulation in the Turonian Western Interior seaway of North America. Geological Society of America Bulletin. 108:0941–0952.
- Sluijs A, Pröss J, Brinkhuis H. 2005. From greenhouse to icehouse; organic-walled dinoflagellate cysts as paleoenvironmental indicators in the Paleogene. Earth Sci Rev. 68:281–315.
- Sun X, Zhang T, Sun Y, Milliken KL, Sun D. 2016. Geochemical evidence of organic matter source input and depositional environments in the lower and upper Eagle Ford Formation, south Texas. Org Geochem. 98:66–81.
- Tocher BA, Jarvis I, 1987. Dinoflagellate cysts and stratigraphy of the Turonian (Upper Cretaceous) Chalk, near Beer, south-east Devon, England. In: Hart MB, editor. Micropalaeontology of Carbonate Environments. Chichester: Ellis Horwood; p. 138–175. (Special Publication British Micropalaeontological Society).
- Tröger K-A, Kennedy WJ, Burnett JA, Gale AS, Robaszynski F. 1996. The Cenomanian stage. In: Rawson, PF et al. (Eds.) Proceedings of the Second International Symposium on Cretaceous Stage Boundaries; Sep 8–16; 1995; Brussels. p. 57–68. (Bulletin de l'Institut Royal des Sciences Naturelles de Belgique – Sciences de la Terre, No. 66).
- Tyson RV. 1995. Sedimentary organic matter: organic facies and palynofacies. London: Chapman and Hall.
- Uramoto G-I, Tahara R, Sekiya T, Hirano H. 2013. Carbon isotope stratigraphy of terrestrial organic matter for the Turonian (Upper Cretaceous) in northern Japan: implications for ocean-atmosphere δ13C trends during the mid-Cretaceous climatic optimum. Geosphere. 9:355–366.
- Van Helmond NAGM, Sluijs A, Papadomanolaki A, Plint G, Gröcke DR, Pearce MA, Eldrett JS, Trabucho-Alexandre J, Walaszczyk I, van de Schootbrugge B, et al. 2016. Equatorward phytoplankton migration during a cold spell within the Late Cretaceous super-greenhouse. Biogeosciences. 13:2859–2872.
- Williams GL. 1977. Dinocysts: their palaeontology, biostratigraphy and palaeoecology. In: Ramsey ATS editor. Oceanic Micropalaeontology. London: Academic Press; p. 1231–1325.
- Williams GL. 2006. Palynological Analysis of Elf Hermine E-94, Scotian Basin. Dartmouth, NS: Geological Survey of Canada. (Open File. 4976:22).
- Williams GL, Brinkhuis H, Pearce MA, Fensome RA, Weegink JW. 2004. Southern Ocean and global dinoflagellate cyst events compared: index events for the Late Cretaceous–Neogene. In: Exon NF, Kennett JP, Malone MJ, editors. Proceedings of ODP, Sci. Results 189. p. 1–98.
- Williams GL, Bujak JP. 1985. Mesozoic and Cenozoic dinoflagellates. In: Bolli HM, Saunders JB, Perch-Nielsen K, editors. Plankton Stratigraphy. Cambridge Earth Sciences. Cambridge: Cambridge University Press; p. 847–964.
- Williams GL, Fensome RA, McRae RA. 2017. The Lentin and Williams index of fossil dinoflagellates 2017 edition. AASP Contribution Series No.48. American Association of Stratigraphic Palynologists Foundation. ISSN 0160-8843.
- Zonneveld KAF, Bockelmann FD, Holzwarth U. 2007. Selective preservation of organic-walled dinoflagellate cysts as a tool to quantify past net primary production and bottom water oxygen concentrations. Mar Geol. 237:109–126.