ABSTRACT
Climate change will have significant impacts on inland aquaculture. This article assesses the robustness of a set of potential adaptation strategies for Northern Thailand using a rule-based assessment model to synthesize information from secondary sources, fish farmers, officials and experts. The net benefits of different strategy types vary substantially with water demand and fish demand, as well as future climate. No-regret and low-regret strategies are worthwhile under a broad range of conditions, but may not be sufficient to maintain profitability as the negative impacts of climate change unfold. The main implication is that adaptation pathways must be flexible.
Introduction
Half of the aquatic animal food consumed by humans today is now from aquaculture (Bostock et al., Citation2010). The expectation is that this fraction will grow, as will the significance of aquaculture in global food security (Troell et al., Citation2014), international seafood markets, and trade (Kobayashi et al., Citation2015). Having sufficient water of adequate quality is critical to the sustainability of aquaculture (Boyd, Li, & Brummett, Citation2012). The actual expansion of aquaculture will therefore depend on the level of growth in water demand from other users, and the ability of aquaculture to limit its own adverse impacts on water quality (Wongsupap, Weesakul, Clemente, & Das Gupta, Citation2009) and maintain or increase water productivity (Cai, Huang, Tan, & Yang, Citation2011). Furthermore, depending on location and season, aquaculture systems may be vulnerable to the impacts of climate change, which reduce water availability and quality, or increase the risks of extreme weather events that result in floods. A recent global analysis ranked freshwater and brackish aquaculture in several countries in Asia as being the most vulnerable to the impacts of climate change (Handisyde, Telfer, & Ross, Citation2017). Earlier studies focusing on Pacific Island countries, in contrast, suggest there may be growth benefits from warmer temperatures and increased rainfall for Nile tilapia production with appropriate investments in adaptation (Bell et al., Citation2013; Pickering et al., Citation2011). De Silva and Soto (Citation2009) noted that if we also take into account changes in water availability, disease risks and extreme events, then the effects of climate change on freshwater aquaculture become even more difficult to predict. Concern with these and related challenges is leading to new collaborative efforts to design more comprehensive global projections of the effects of climate change on fisheries and aquaculture (Paukert et al., Citation2017).
Adaptation to climate change in aquaculture, as in other agriculture and livestock sectors, will benefit from improvements in how climate information is shared, understood and used (Dilling & Lemos, Citation2011; Travis, Citation2014). In the specific case of aquaculture, researchers have highlighted the importance of implementing regulations and the use of standards in governing production as ways to help improve how climate-related risks are managed (Yamprayoon & Sukhumparnich, Citation2010). Likewise, there is interest in the use of financial instruments like insurance to share risks (Beach & Viator, Citation2008). In the longer term, researchers have suggested strategies like genetic improvement programmes for thermal tolerance (De Silva & Soto, Citation2009), and increased attention to watershed and aquatic ecosystems in which aquaculture is embedded, as these provide critical adaptation services (Lavorel et al., Citation2015), which may be disrupted by unsustainable aquaculture practices (Ahmed et al., Citation2013; Bush et al., Citation2010), water demands and climate change.
One key challenge for water management and policy is the uncertainty around how climate and other critical factors, like water demand and availability, will change and interact in the future (Hanjra, Blackwell, Carr, Zhang, & Jackson, Citation2012) to influence water availability for aquaculture. A possible approach to climate risk management and adaptation in such a situation is to pursue strategies that are more robust. The key feature of a robust strategy is that although it may not be as efficient under ideal conditions as the standard approach, its performance will be adequate even under sub-optimal conditions, whereas the standard system would fail with serious consequences (Anderies, Janssen, & Ostrom, Citation2004). Climate-robust aquaculture strategies are defined in this article as those that are likely to perform satisfactorily under a range of weather perturbations, seasonal changes, current climate variability, and plausible climates in the next few decades. Decision analysis and support tools which can help assess robustness have been developed (Lempert, Citation2013; Weaver et al., Citation2013), but have not yet been applied to aquaculture in the context of climate change.
The purpose of this study is to assess the robustness of alternative adaptation strategies for addressing the impacts of extreme weather events and climate change on the water resources available to aquaculture in Northern Thailand whilst also taking into account uncertain, but potentially significant concurrent changes in water demand and fish demand.
Aquaculture in Northern Thailand
Northern Thailand is an appropriate region for making broader generalizations about inland aquaculture and climate change because it includes areas which are seasonally cool and dry as well as areas that are warm and wet (). Also, significant parts of the main valleys and plains are irrigated, but other vast areas remain rainfed. Studies of future climate and water resource conditions for Northern Thailand yield a wide range of projections, with large uncertainties in precipitation (Lacombe, Hoanh, & Smakhtin, Citation2012), and thus river flows (Kotsuke, Tanaka, & Watanabe, Citation2014). Water demand from various sectors in Thailand has grown incrementally over the past couple of years (Divakar, Babel, Perret, & Gupta, Citation2011), implying that the water available for aquaculture may contract in future. Increasing demand for farmed fish for both domestic consumption and export (Department of Fisheries, Citation2013) could further increase competition for water resources and suitable rearing sites, but it could also lead to higher farm-gate prices. The region includes the upper reaches of the major rivers in Thailand (), and thus water management in the region is geopolitically significant for irrigation, flood, and drought policies. Taken together, these issues raise the challenge of identifying strategic policies which could enhance the robustness of aquaculture as a successful agro-industrial sector (Belton & Little, Citation2011), and contribute to livelihood security (Friend & Funge-Smith, Citation2002; Setboonsarng & Edwards, Citation1998).
Figure 1. (a) Map of assessment region in Northern Thailand showing provinces in which different culture systems and hatcheries were investigated, and the four major rivers which merge to become the Chao Phraya River. (b) Gridded mean temperature in Dec-Feb (DJF). (c) Annual rainfall.
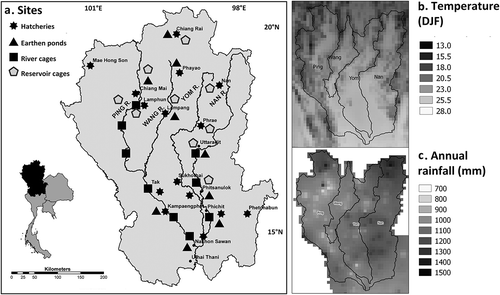
Climate-related risks
Fish farmers in Northern Thailand grow Nile tilapia and other fish in earthen ponds, and hybrid red tilapia in floating cages in rivers and reservoirs (Chaibu, Ungsethaphand, & Maneesri, Citation2004; Lebel, Lebel, & Lebel, Citation2016a; Lebel et al., Citation2013; Pimolrat, Whangchai, Chitmanat, Promya, & Lebel, Citation2013). Most farmers obtain fish fry from government or private hatcheries, where they are commonly reared in cement tanks (Uppanunchai, Apirumanekul, & Lebel, Citation2015). Climate and extreme events such as high floods, seasonal droughts, heat waves and cold spells all have significant impacts on production and profits ().
Table 1. Important risks to the profitability of fish farms and hatcheries in Northern Thailand from selected water-related impacts of extreme weather and climate change. Climate often interacts with human activities in the watershed and water management to impact water flows and quality.
For earthen-pond culture, floods, droughts, and periods with dense cloud cover are important risks (Pimolrat et al., Citation2013). Seasonal water shortages limit exchange, making it difficult to maintain pond depths and water quality (Sriyasak, Chitmanat, Whangchai, Promya, & Lebel, Citation2015). High phytoplankton concentrations in ponds can lead to low dissolved oxygen (DO) levels early in the morning due to night-time respiration by phytoplankton. The risks are highest near dawn in high-nutrient-input culture systems, following a day of prolonged and thick cloud cover, which reduces sunlight, and thus normal rates of photosynthesis by phytoplankton. Thermal stratification followed by rapid turnover with sharp changes in temperature can also cause mass-mortality events by exposing fish to low DO concentrations (Sriyasak, Chitmanat, Whangchai, & Lebel, Citation2013). Off-flavour problems attributable to geosmin from cyanobacteria in high-nutrient-input ponds also appear to vary seasonally, with the highest risks in the transition months (Feb.–April) into hotter and wetter conditions (Pimolrat, Whangchai, Chitmanat, Itayama, & Lebel, Citation2015). Fish with this musty odour fetch lower prices.
In river-cage culture, floods (including spates or high flows) are key risks because they can exhaust and injure fish and damage the cages (). Droughts (including low water flows) in the Upper Ping River at the end of the dry season in dry years constrain in-stream cage aquaculture to locations behind weirs with sufficient depth and volumes (Lebel, Whangchai, Chitmanat, & Lebel, Citation2015b; Lebel et al., Citation2013). In highly regulated rivers, water storage strategies to support dry-season irrigation or support flood protection policies downstream greatly modify water flows, and may even reverse the seasonality of low-flow risks (Lebel et al., Citation2015b). Extreme high temperatures appear to stress fish and increase risks of diseases, especially when they coincide with periods of low water levels near the beginning of the wet season (Chitmanat, Lebel, Whangchai, Promya, & Lebel, Citation2016). Heavy-rainfall events bring in high levels of sediment or high concentrations of accumulated pollutants, which can also result in mass-mortality events.
In reservoirs, important climate-related risks include those related to heat waves, cold spells, droughts, and prolonged dense cloud cover (Lebel et al., Citation2016a). Another significant seasonal risk is the sharp drop in temperature at the transition period from the wet to the cool season, when previously stratified water columns mix, bringing low-DO water from the bottom to the surface, where fish are grown in cages. If prompt action such as supplementary aeration is not taken, this can lead to mass-mortality events.
Cold spells in the more northern sites reduce fish feeding and growth in all culture systems, and hinder breeding in hatcheries (Uppanunchai et al., Citation2015). Floods in the wet season are a significant risk to hatcheries in low-lying areas, while water shortage at the end of the dry season is a significant constraint for those without good storage or all year-round supply. Heat waves and cold spells are also important risks to fish fry production.
Each of these climate-related risks today could be exacerbated by the impact of climate change on the frequency or severity of extreme-weather events (). A higher frequency and severity of tropical storms, for example, could increase the risk of floods. Water management and other factors may also interact to amplify these impacts, for example, infrastructure failures like a weir collapse were observed in 2005–06 in the upper Ping River (Lebel et al., Citation2013).
Management of climate-related risks
Climate risk management practices at multiple spatial and temporal scales are important for dealing with extreme weather as well as for climate change adaptation (). In the short term, farmers make many key decisions and actions as they react to imminent threats or recent impacts (Lebel et al., Citation2016a). In the medium term, key decisions relate to tactical calculations based on seasonally varying risks from climate and market factors (Lebel, Sriyasak, Kallayanamitra, Duangsuwan, & Lebel, Citation2016b). And in the long term, decisions are more strategic and relate to perceptions of changing water resource conditions and climate, new technologies and consumer demand (Uppanunchai, Chitmanat, & Lebel, Citation2016).
Table 2. Examples of possible adaptation responses to risks of climate change impacts on water resources important to the profitability of the aquaculture sector. Classified according to patterns in adaptation costs and risk-reduction benefits over time (response type) and scale of response.
At the farm and household level, farmers use a combination of adjustments to rearing practices and cropping calendars, as well as financial and social measures, to mitigate those risks to production and profits which they perceive as manageable (Lebel, Whangchai, Chitmanat, & Lebel, Citation2015a). For instance, in the short term, supplementary aeration is a useful tool in all culture systems when DO is low (Sriyasak et al., Citation2015). In pond culture, farmers may draw on groundwater or small-scale on-site water storage to deal with water shortages. In cage culture in rivers, farmers may move their cages to protected locations in response to imminent high flows, or harvest fish early. In the medium term, delaying stocking or reducing stocking density are common practices that help reduce risks from challenging water and climate conditions (Lebel et al., Citation2015a). Likewise, stocking larger fingerlings, which reduces the length of culture period in risky locations or seasons, is another way to reduce exposure to multiple types of risk.
Previous studies have shown that individual risks are often addressed through multiple practices and strategies; conversely, a particular management practice can have a bearing on several different risks (Lebel et al., Citation2015a). This includes some non-aquaculture-specific measures such as diversifying income sources to include off-farm activities (), which may be a source of resilience as well as funds to invest in other risk-reduction measures (Sin-Ampol, Citation2015). Self-assessment by fish farmers suggests that many farm-level reactions and tactics useful today are likely to be useful under a range of possible future climate patterns – the key issue being whether the costs of such measures are worthwhile in a particular site (AQUADAPT, Citation2014). How climate-related risks are communicated, perceived and understood is another set of issues that influence risk management practices (Lebel & Lebel, Citation2016; Lebel, Whangchai, Chitmanat, Promya, & Lebel, Citation2015c). Stocking decisions made by fish farmers in a role-playing simulation game, for example, suggests that learning about risks from experience is not easy, especially if risks are increasing or changing in severity (Lebel et al., Citation2016b).
In all culture systems, farmers recognize that key water quality and flow-related risks also need to be managed at the river-reach, irrigation system, or watershed level. Important short-term responses include sharing information and mutual assistance during emergencies (). In the medium term, the management of water-related infrastructure (dams, weirs, water-gates) is very important. For river-based cage aquaculture, the construction and operation of small weirs for local irrigation are important to manage flow depths during critical periods in the dry season, especially in the upper Ping River (Lebel et al., Citation2015a). In other river reaches, the management of water releases from large hydropower dams has a major influence on risks of low flows and depths as well as potentially destructive high flows close to the dam site (Lebel et al., Citation2015b). For earthen-pond culture, local water management and its governance at the community level also have major consequences for the capacity of fish farmers to manage water shortages and low-water-quality risks (Kengkaj, Citation2015). In reservoirs, fish farmers emphasize the importance of maintaining good relations with other stakeholders, including officials responsible for reservoir water management (Lebel et al., Citation2016a).
At the sectoral and national levels, agencies need to provide timely warning information and relief resources to local authorities. In the mid-term, the network of spatially distributed government hatcheries in Northern Thailand allows some scope for shifting production base in response to seasonal constraints on hatchery operations (Uppanunchai et al., Citation2015). Important long-term strategies are for government to support improved climate information systems, and to invest in culture of alternative species and genetic improvement. These strategies can also benefit from international cooperation, but progress is slow and has so far been disconnected from the aquaculture sector (Uppanunchai et al., Citation2016).
Methods
Data sources
This article draws on published research (summarized above), local community assessment meetings, expert advisory group meetings, and a multi-stakeholder dialogue event based on activities carried out in 2012–16. A series of participatory local assessment meetings and related activities were carried out with groups of pond and cage farmers in two locations: Chiang Mai and Phayao. In these facilitated meetings, fish farmers discussed and jointly evaluated the performance of specific practices (AQUADAPT, Citation2014). These evaluations included estimates of costs relative to expected benefits, and observations on how conditions and situations of individual farms can influence these calculations. The views of scientific experts, policy and private-sector practitioners were also solicited through in-depth interviews and six stakeholder advisory group meetings. A few long-term strategies, like ecosystem management, zoning, species selection, development of standards, and export markets, were proposed by officials (often as part of existing policies) or experts; farmers in meetings expressed views on some of these options, but for others had no position as details of the strategies were not familiar. Two strategies were proposed by the authors: ecosystem restoration and water productivity research. The information collected using these various methods guided the identification of short-, mid-, and long-term climate risk management options, and the identification of adaptation strategies for further analysis. The main findings of the assessment were presented and discussed at a multi-stakeholder event at the end of 2015, and distributed in report form in the Thai language for improved accessibility (Lebel & Chitmanat, Citation2015). Feedback on the proposed strategies informed the final version of the rule-based assessment model used in this article.
Analytical framework
The information gathered for the assessment was organized following the XLRM analytical framework used in robust decision support studies (Mehta, Depsky, Forni, Purkey, & Betancourt, Citation2013). X refers to external factors or drivers, some of which may be uncertain. In this study, these were the three scenario dimensions, of climate change, fish demand growth, and water demand growth (see below). L refers to policy or management levers, which in this study were the adaptation strategies listed in . R refers to relationships between various variables, captured here as lists of assumptions and implemented as a simple rule-based model (Tables S1–S4). M refers to robustness metrics, conceptualized here as an indicator of the number of different conditions under which risk-reduction benefits exceeded adaptation costs and aquaculture was still profitable (see below).
Climate change scenarios
Four qualitative climate change scenarios (Wetter, Drier, More Seasonal, Less Seasonal) were constructed to capture key uncertainties in future climate in Northern Thailand (Table S1) based on an earlier study on climate change impacts on hatcheries (Uppanunchai et al., Citation2015). Under the Wetter scenario, rainfall increases in both wet and dry seasons; in the Drier scenario it decreases in both seasons. In the More Seasonal scenario, rainfall increases in the wet season and decreases in the dry season; in the Less Seasonal scenario, the pattern is reversed. The risks of floods, prolonged cloud cover, intense rainfall, heat waves and droughts follow on from these assumptions. A fifth, ‘no change’ scenario (current climate variability) was also included in some analysis to assess relative changes. In joint assessment meetings with fish farmers, these scenarios were explained and understood as being similar to historical patterns of climate variability, where in some decades it rained a lot in the wet season, causing many floods, whereas in other decades, drier conditions and droughts were more common, but also potentially more extreme.
To help define plausible boundaries for individual climate scenarios and the differences among scenarios, we analyzed historical and projected climate data from the Integrated Study on Hydro-Meteorological Prediction and Adaptation to Climate Change in Thailand (Kotsuke et al., Citation2014; Watanabe et al., Citation2014). Modelled climate projections downscaled for 2040–59 under the RCP4.5 and RCP8.5 emission scenarios were compared to the 1981–2010 baseline. The wettest and driest climates from nine different models were used to set indicative average levels of change for total rainfall of +15% for the Wetter scenario and −5% for the Drier scenario. The model with the lowest and highest combined rainfall in May and October (the transition months between seasons in Northern Thailand) were used to set indicative average levels of change for those two months: −20% for More Seasonal and +10% for Less Seasonal. In the More Seasonal scenario, the rain deficit is imagined as being transferred to the wet months (June–Sept.); in the Less Seasonal scenario it is shifted to the dry months (Nov.–April). Temperature increases and the risks of heat waves were assumed to be smaller in the Less Seasonal scenario (+0.5 °C) than in the other three scenarios (+1.5 °C). Periods with heat waves and extreme high water temperatures were assumed to be more likely in the hottest period of the year, typically around May.
Water demand and fish demand scenarios
Scenarios were developed for 35 years into the future (to 2050) for water and fish demand to complement the five climate scenarios, yielding a total of 20 (2 × 2 × 5) scenarios. High-water-demand-growth scenarios assumed that demands for water from other sectors (such as irrigated rice, industry, tourism and urban areas) increase substantially (+50%) relative to current demands levels within Northern Thailand. A no- or low-water-demand-growth scenario (+5%) appears much less likely, but may arise in situations where the rice export market collapses or a slowdown in overall economic activity occurs.
The high-fish-demand-growth scenario assumed that demand for aquaculture products doubles (+100%) relative to current levels (ca. 40,000 tonnes/year), and that efforts to meet this demand are pursued vigorously in Northern Thailand. It was also assumed that high fish demand results in higher farm-gate prices and increased production. The low-fish-demand-growth scenario (+10%) is less likely, but possible if for instance, strong health or environmental concerns were to come up and incite a consumer backlash against aquaculture products. Similarly, a large influx of cheaper imports of farmed fish, such as frozen pangasius from Vietnam, or greater product knowledge could significantly shift consumers’ purchase intentions (Nguyen, Dang, Do, & Mai, Citation2015).
Assessing robustness of adaptation strategies
A simple, rule-based model was developed to help codify and synthesize information and beliefs on the efficacy and costs of adaptation options under different conditions, and to assess the relative robustness of different strategies. The model relates performance to the value of risk reduction benefits, the costs of those measures, and the levels of climate-related risks – all measured in units of baht/kg, where the denominator is interpreted as the expected production given the inputs. The advantage of this formulation is that it captures the risks and interests from the perspective of an average fish farmer. The disadvantage is that it does not address issues or constraints related to aggregate decisions, such as total production volumes. The model was developed and run as a syntax file in SPSS to allow easy exploration of multiple scenarios and the effects of combining multiple assumptions. We now describe the key features of the rule-based assessment model. Further details on parameter estimates are included in the online supplementary material (Tables S1–S4, https://doi.org/10.1080/02508060.2017.1416446). Performance of each strategy was captured by three outcome metrics: profitability, net profit difference, and robustness.
Net profitability with adaptation
The first measure of performance, net profitability with adaptation (), was conceived as the difference between sale price (
), input costs (
), fixed costs (
), adaptation costs (
) and excess risk (
) for a particular strategy (s):
Sale price is assumed to increase with fish demand, and vary slightly among culture systems given differences in typical species reared (Table S2). Costs of inputs and fixed costs are assumed to be constant and at 2015 prices. The excess risk () from climate change after taking into account adaptation ()) was defined as the difference between the sum of the initial risk (
) and the baseline risk (
), and the risk reduction factor associated with adopting a particular adaptation strategy (
).
Figure 2. Schematic showing relationships between risk components (a) and a classification of five strategy types (b–f) based on patterns of risk-reduction (RR) benefit and costs (Cs) over time.
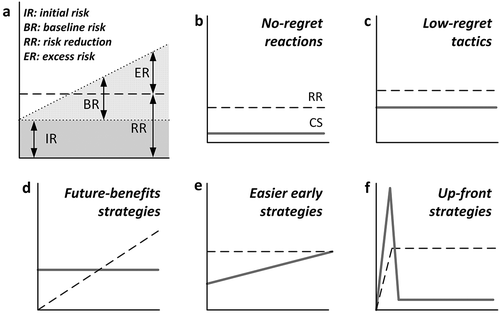
The baseline risks for floods, droughts, and heat waves followed the logic of each climate change scenario (). For example, under a Wetter scenario, flood risk increases while drought risks declines (Table S1), with further adjustment for other factors as follows:
Note: If .
Two other sets of assumptions were made to describe baseline risks. First, under a high-water-demand () scenario, drought and heat-wave risks increased while there was no change in flood risk. Second, risks from floods and droughts were increased in rivers but not for other systems (
), while for heat waves risks were higher in non-river systems. If the estimated
was negative (easier conditions than the present) then
was constrained to not go below
, as this would mean a negative total risk ()).
The levels of risk reduction () benefit for each specific adaptation strategy (s) was a rule-based function in the form of:
Several specific rules were derived, as the following examples illustrate. When climate changes (), strategies that improve information (Strategies 14, 22, 27 in Table S4) or the institutional framework for management of water (Strategies 24, 30) become more beneficial. When water demand (
) is high and there is drought risk, then strategies which store water help reduce risks (Strategies 15, 31). When fish demand (
) is higher, market strategies work better (strategies 29, 32). A few strategies were more important for small farms than for large farms (
), such as forming groups (Strategy 4) or diversifying income sources (Strategy 19), particularly if fish demand is also low. Others were better for large farms (Strategies 29, 30, 32). Some strategies were culture-system (
) specific. For instance, strengthening cages was not relevant to hatcheries or ponds, and so was excluded in estimating means for the performance indicators. The full list of final rules is given in Table S4.
Returning to Eq. (1), important assumptions were made about how adaptation costs () and risk-reduction benefits (
) varied over time. To this end, five response types were identified (–)). For instance, up-front strategies such as building water infrastructure cost a lot initially, but provide substantial benefits later, whereas no-regret and low-regret responses cost a little each year and provide smaller benefits. Easier early strategies require progressively higher investments to maintain benefits as risks increase over time. Future-benefits strategies require ongoing investments; the flow of benefits is low initially and then increases with time. ‘Reactions’ and ‘tactics’ have much shorter investment horizons than ‘strategies’, and have correspondingly lower costs, but also lower benefits, especially if risks increase greatly.
values were largely fixed at a constant value for each strategy type, with one exception, infrastructure, with a higher cost (Table S4). In this study, the start year (t = 0) was taken as 2015, and model estimates were made for various variables for 2020, 2030, 2040 and 2050. A second classification of strategies used in some analyses was to group them as infrastructural, institutional, financial, informational, or technical.
Net profit difference
The second measure of performance used was net profit difference () or the difference between net profit with adaptation (
) and without (
).
where the net profit in the absence of adaptation is given by:
Robustness index
The third measure of performance was a robustness index. A strategy was considered worthwhile if adopting it increased profits compared to not doing so ( > 0) and aquaculture was profitable (
> 0). We add the profitability criterion because a strategy might appear worthwhile in the sense that it reduces risks efficiently, but if it does not make a net profit (
), then it is better not to do aquaculture at all. One strategy was considered more robust (for a particular culture system) than another if it was worthwhile under more combinations of conditions, and conversely, more vulnerable if it was not. Thus, the robustness index (
) was defined as:
where is the total number of distinct conditions considered in a particular analysis.
Results
In this section, we use a rule-based assessment model to explore the potential performance of individual responses and response types () under a wide range of climate, water-demand, and fish-demand conditions.
Baseline risk
shows how baseline risks (without considering adaptation actions) for floods, droughts and heat waves were captured in the assessment model. The More Seasonal climate scenario represents the most challenging set of conditions, with all risks high in 2050. The Less Seasonal scenario is the most benign, with risks of both floods and droughts less than current conditions (‘no change’). In a Drier or More Seasonal climate, drought is by far the largest risk, especially when water demand is also high. Even with no change in climate, drought risks in a high-water-demand scenario increase by a projected 10 baht/kg, which can be benchmarked against the 8 baht/kg price premium farmers received in 2015 for rearing ‘antibiotic residue-free’ fish for major retailers. Flood risks are highest under the Wetter scenario. In contrast to water demand, fish demand does not have much influence on baseline risks. Heat wave risk varies less among scenarios than flood or drought risk.
Changing benefits and costs
As response types differ in how risk-reduction benefits and costs change over time (), so does their performance, once baseline risks or costs are taken into account. Under the More Seasonal scenario, all three climate risks increase substantially; thus it becomes increasingly difficult to maintain profitability out to 2050, regardless of response type ()). As expected, projected profitability is high in the two relatively benign climate scenarios (no change and Less Seasonal), and under such conditions no-regret and low-regret responses perform best. The profitability of future-benefit strategies does not decline as strongly with time as it does for other strategy types under challenging conditions (Wetter, Drier, and More Seasonal), because benefits, like risks, increase over time. Setting aside the impacts of climate change, profitability is also squeezed more with time during high water demand and low fish demand (Figure S2). In this situation, prices are low, and water for aquaculture is limited. Patterns of profitability of the strategy types over time were not strongly influenced by farm size or culture system.
The added value of adaptation
Performance was also assessed by calculating the difference in profits with and without the adaptation action. Thus, the consistent and positive profit difference for no-regret and low-regret strategies suggests that they are worthwhile under all climate scenarios ()), with the caveat that profitability declines substantially by 2050 under more challenging scenarios, and therefore aquaculture itself may no longer be worth pursuing ()). Easier-earlier strategies declined to low or negative values for profit difference in all scenarios, as risks increase with time, whereas future-benefits strategies increased to high positive values over time in challenging scenarios ()). In a Less Seasonal scenario, up-front strategies such as infrastructure are the least worthwhile approach to adaptation, as they cost a lot and there is only a small risk to adapt to.
An examination of the mean profit difference over time for individual strategies in each culture system helps understand the broader patterns by type, but also some of the internal heterogeneity in performance (). First, the up-front strategy type included two individual construction-based strategies (32, treatment; 33, dykes) that were on average either irrelevant or not worthwhile in any culture system. Second, a few individual strategies were profitable only in the one or two systems for which that practice was relevant (15, water storage for pond culture; 12, strengthen cages for river cage culture). Third, no-regret and low-regret responses yielded positive profit differences in all culture systems for which they were relevant. Fourth, future-benefits strategies varied substantially from each other and across culture systems, implying that overall, averages for this group should be treated carefully.
Figure 5. Estimated mean profitability difference with and without individual adaptation strategies in four culture systems, 2015–50. Strategy abbreviations and numbering are as in . Completely blank entries indicate a strategy is not relevant and so not applied to that culture system.
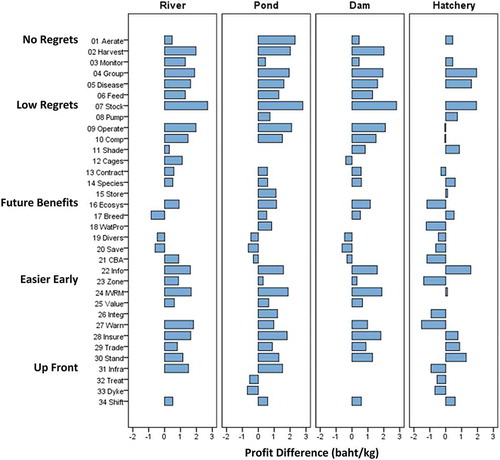
Classifying strategies by content domain () highlights the low expected value of financial strategies for all culture systems, and that overall, the strategies assessed help hatcheries less than other culture systems (in part because they are less at risk). It also shows that infrastructure solutions are of no use to fish farms already in dams, while institutional, informational and technical strategies are of similar value on average in terms of profit difference.
Robustness of strategies under uncertain conditions
On average, the mean robustness of different strategy types over time was influenced more by assumptions about future fish demand and climate change than by water demand (Figure S3). When fish demand was high, strategies were more robust, reflecting the higher prices farmers received. On average, no-regret strategies were the most robust, and future-benefits the least. Individual strategies with high robustness included several no-regret strategies: 2, harvest early; 4, form groups; 7, stocking (). Several easier and earlier strategies were also robust (28, insurance; 24, IWRM; 25, adding value). The robustness of individual adaptation responses () largely parallel patterns of profit difference ().
Discussion
The findings of this assessment underline that successful adaptation in aquaculture will need to consider climate risk management practices at multiple spatial and temporal scales. Short-term reactions before, during and after critical events (droughts, floods, heat waves) are and will remain important to reducing risks. This includes tactics like adjustments to rearing practices, providing aeration, withholding feed and moving fish, as well as sharing information about extreme flow or weather conditions and helping each other cope with extreme events. Likewise, mid-term tactics related to timing, siting, stocking and harvesting decisions each year are important under both current and future climate variability. These short- and medium-term practices are an important component of the existing adaptive capacity of fish farms and communities, and have been recognized as such in previous research studies and assessments (Bell et al., Citation2013; De Silva & Soto, Citation2009).
However, it is long-term strategies that distinguish current climate risk management from what is needed for adapting to a changing climate in the future. Attention to temporal patterns in the distribution of potential risk-reduction benefits and adaptation costs in this analysis revealed that long-term strategies are important in at least three distinct ways. First, an initiative may have a long lead time, that is, it takes time before any benefits will be apparent (future benefit). Second, an action might be taken up relatively quickly, but will have consequences for many decades into the future (up-front), when climate may no longer be the same. And third, an action might need to be repeated or sustained indefinitely, but with monitoring and flexibility to adjust to changing risks (easier early). Current policy thinking on climate change adaptation strategies has often neglected these distinctions, even though they have very different implications for patterns of investment in adaptation (Felgenhauer & Webster, Citation2013; Hallegatte, Citation2009).
Many of the expected adverse impacts of climate change on inland aquaculture are mediated by how water resources are managed, which in turn is influenced by water demand. Fish farmers recognize that key water quality and flow-related risks also need to be managed at the watershed or community level, and that this is difficult without the cooperation of other stakeholders. In a drier climate, risks of water shortages and droughts will be greatly amplified if water demand is also high, putting extreme pressure on water allocation for aquaculture. One existing constraint in the Thai context is that aquaculture’s stake in water resources development and management has not been well articulated, and thus is rarely acknowledged. Even for government hatchery centres, water allocation is vague and not guaranteed (Uppanunchai et al., Citation2015). This remains an important barrier to adaptation strategies, requiring better integration and policy coordination across fisheries, agriculture, and water management policy domains (Uppanunchai et al., Citation2016).
Management focused on climate and water that takes into account the long term and the watershed level, while necessary, is unlikely to be a sufficient response. Under current climate variability, fish farmers do not manage individual climate-related risks in isolation from other risks, and hence in the future, the need for similar practices seems inevitable. Financial sources of risks such as debt repayment, high interest rates on loans, market prices for fish, costs of inputs, and maintaining adequate savings also need to be carefully managed (Ahsan & Roth, Citation2010; Lebel et al., Citation2015a). The analysis of robustness of strategy types suggests that fish demand and prices may have a larger impact than water demand and climate change, at least in 2040–59. The inclusion of low and high fish demand growth scenarios in this analysis helped capture only some of the gross features of a complicated set of financial and market-related factors and risks (Navy, Minh, & Pomeroy, Citation2017; Rodríguez-Rodríguez & Bande Ramudo, Citation2017). Climate change could, for example, drive increases in feed costs – a key input – if it reduces ocean fish stocks that are used to make fish meal (Deutsch et al., Citation2007).
The large uncertainties about future climate, water demand, and farmed fish demand favour strategies that are robust and worth pursuing under multiple conditions, and which are valid for multiple climate-related risks. For some response options, this can be interpreted in two ways. First, responses may make farms more robust to future conditions from the perspective of fish farmers. Second, responses may make institutions or policies themselves more robust, that is, likely to continue to perform adequately under a range of future conditions. One could question, for example, whether a privately run crop insurance scheme would survive in a future world with higher flood and drought risks (Aerts & Botzen, Citation2011), even if such a scheme would be beneficial to fish farmers by making their operations more robust.
The model developed in this assessment helped us make explicit assumptions and expectations. It also helped us explore the consequences of combining assumptions. The model is simplistic, however, and the estimates it produces are crude and hard to validate. We see a couple of promising ways to take this semi-qualitative approach to robust decision analysis forward in evaluating sets of adaptation options. First, to improve realism there is a need to consider combinations of strategies ranging from those which might act largely independent to those for which there are synergies or trade-offs. Some strategies are likely to reinforce each other if pursued in parallel, like improving early-warning systems and climate information systems. Other strategies may interact more negatively, like investments in infrastructure and ecosystem restoration. Second, to improve reproducibility and validity, a more systematic set of validation exercises with independent experts is needed. Although it is unlikely that there will ever be enough accurate information on costs and risk-reduction benefits, rank-based measures might turn out to be good enough for these types of exploratory analyses. Third, the model focused on the average farmer’s profit per kilogram of fish, a highly individual perspective on returns on investment under risks that does not take into account feedback from high aggregate demand on water resources arising from higher total production volumes and so on. As resources become constrained, this is not realistic. Fourth, the assessment model was based entirely on linear changes in averages, and thus did not take into account inter-annual or inter-decadal variability in climate and associated risks. Doing so would result in more periods where investments in a strategy would have been inadequate or overkill. Climate variability would also make it much harder to learn and adjust strategies over time. But the current version of the model does not allow abandoning or switching strategies, or any form of learning.
The methods and findings of this study have international significance beyond the geographic area of Northern Thailand. First, the three classifications of strategies proposed in this study (temporal-spatial scales, cost-benefits over time, content domain) are generalizable to diverse possible culture systems and locations. Analysis at this level, we propose, is helpful for policy framework development and screening response options. Second, the highly seasonal climate and water resources conditions for aquaculture in Northern Thailand are comparable to other monsoonal locations in the Asia-Pacific where inland aquaculture is practised. The high level of commercialization of the sector in Thailand provides an early insight into how the industry may develop in other countries as demand for farmed fish grows. Third, the semi-quantitative modelling approach adopted here to help synthesize findings and expert views for the assessment may be suitable for other locations where detailed impact and cost information is not available, but where there are experiences to draw upon.
The findings are also significant for current debates on the role of climate risk management in adaptation outside the aquaculture sector. On the one hand, this study shows the practical value of better understanding of specific climate-related risks for their management. Fish farmers who understand how drought risks change with seasons are able to make timely adjustments to stocking calendars and practices, in anticipation of future water resource conditions. The participatory elements of the assessment also underlined the strong appreciation of the importance of climate variability in decision making. What remains is to find better ways to develop and share information about past climate and the next season. On the other hand, the study also suggests that more broadly framed resilience-based approaches to climate risk management are also worthwhile. These include, for example, long-term strategies to restore and manage aquatic ecosystems and watersheds so that they continue to support aquaculture activities and buffer them from extreme weather events, and diversification of livelihood activities beyond farming fish so that household income is not overly affected by climate-related disaster losses.
Conclusions
Extreme weather events and climate change already have significant impacts on the production and profitability of aquaculture in Northern Thailand. Future climate, water demand and fish demand are three important factors for longer-term viability of this sector. Because they are difficult to project with certainty, their affects were explored using scenarios. Using a novel, rule-based assessment model derived from published research and stakeholder consultations, this study showed how various individual as well as classes of adaptation strategies are likely to be relatively more or less robust. As might be expected, these evaluations vary with aquaculture system and on how far into the future you look. A key policy implication is that adaptation pathways in the aquaculture sector must be diverse enough to cater to the different risk profiles of culture systems, and flexible enough that over time, strategies can be abandoned, switched or combined, or innovations introduced, as conditions and knowledge continue to change.
Supplemental data.pdf
Download PDF (930.7 KB)Disclosure statement
No potential conflict of interest was reported by the authors.
Supplemental data
Supplemental data for this article can be accessed here.
Additional information
Funding
References
- Aerts, J. C. J. H., & Botzen, W. J. W. (2011). Climate change impacts on pricing long-term flood insurance: A comprehensive study for the Netherlands. Global Environmental Change, 21(3), 1045–1060.
- Ahmed, N., Bunting, S. W., Rahman, S., & Garforth, C. J. (2013). Community-based climate change adaptation strategies for integrated prawn-fish-rice farming in Bangladesh to promote social-ecological resilience. Reviews in Aquaculture, 5, 1–16.
- Ahsan, D., & Roth, E. (2010). Farmers’ perceived risks and risk management strategies in an emerging mussel aquaculture industry in Denmark. Marine Resource Economics, 25(3), 309–323.
- Anderies, J. M., Janssen, M. A., & Ostrom, E. (2004). A framework to analyze the robustness of social-ecological systems from an institutional perspective. Ecology and Society, 9(1), 18.
- AQUADAPT. (2014). Report of participatory assessment workshops on climate risk management with fish farmers in northern Thailand [in Thai]. Retrieved from Chiang Maihttps://aquadaptorg.files.wordpress.com/2017/10/fish-farmers-group-meeting-report-th.pdf.
- Beach, R. H., & Viator, C. L. (2008). The economics of aquaculture insurance: An overview of the U.S. pilot insurance program for cultivated clams. Aquaculture Economics and Management, 12(1), 25–38.
- Bell, J. D., Ganachaud, A., Gehrke, P. C., Griffiths, S. P., Hobday, A. J., Hoegh-Guldberg, O., … Waycott, M. (2013). Mixed responses of tropical Pacific fisheries and aquaculture to climate change. Nature Climate Change, 3(6), 591–599.
- Belton, B., & Little, D. C. (2011). Immanent and interventionist inland Asian aquaculture development and its outcomes. Development Policy Review, 29(4), 459–484.
- Bostock, J., McAndrew, B., Richards, R., Jauncey, K., Telfer, T., Lorenzen, K., … Corner, R. (2010). Aquaculture: Global status and trends. Philosophical Transactions of the Royal Society B: Biological Sciences, 365, 2897–2912.
- Boyd, C. E., Li, L., & Brummett, R. (2012). Relationship of freshwater aquaculture production to renewable freshwater resources. Journal of Applied Aquaculture, 24(2), 99–106.
- Bush, S. R., Van Zwieten, P. A. M., Visser, L., Van Dijk, H., Bosma, R., De Boer, W. F., & Verdegem, M. (2010). Scenarios for resilient shrimp aquaculture in tropical coastal areas. Ecology and Society, 15(2), 26.
- Cai, Y. P., Huang, G. H., Tan, Q., & Yang, Z. F. (2011). An integrated approach for climate-change impact analysis and adaptation planning under multi-level uncertainties. Part I: Methodology. Renewable and Sustainable Energy Reviews, 15(6), 2779–2790.
- Chaibu, P., Ungsethaphand, T., & Maneesri, S. (2004). The costs and returns of Tilapia and Tubtim (Red Tilapia) cage culture in Chiang Mai Province, Thailand. [in Thai]. Journal of Fisheries, 57(3), 244–250.
- Chitmanat, C., Lebel, P., Whangchai, N., Promya, J., & Lebel, L. (2016). Tilapia diseases and management in river-based cage aquaculture in northern Thailand. Journal of Applied Aquaculture, 28(1), 9–16.
- De Silva, S., & Soto, D. (2009). Climate change and aquaculture: Potential impacts, adaptation and mitigation. In K. Cochrane, C. De Young, G. Soto, & T. Bahri (Eds.), Climate change implications for fisheries and aquaculture: Overview of current scientific knowledge. FAO Fisheries and aquaculture technical paper 530 (pp. 151–212). Rome: FAO.
- Department of Fisheries. (2013). The strategic plan for working capital for aquatic animal production 2014-2016 [in Thai]. Bangkok: Ministry of Agriculture and Cooperatives.
- Deutsch, L., Gräslund, S., Folke, C., Troell, M., Huitric, M., Kautsky, N., & Lebel, L. (2007). Feeding aquaculture growth through globalization: Exploitation of marine ecosystems for fishmeal. Global Environmental Change, 17, 238–249.
- Dilling, L., & Lemos, M. (2011). Creating usable science: Opportunities and constraints for climate knowledge use and their implications for science policy. Global Environmental Change, 2, 680–689.
- Divakar, L., Babel, M. S., Perret, S. R., & Gupta, A. D. (2011). Optimal allocation of bulk water supplies to competing use sectors based on economic criterion - An application to the Chao Phraya River Basin, Thailand. Journal of Hydrology, 401(1–2), 22–35.
- Felgenhauer, T., & Webster, M. (2013). Multiple adaptation types with mitigation: A framework for policy analysis. Global Environmental Change, 23(6), 1556–1565.
- Friend, R., & Funge-Smith, S. (2002). Focusing small-scale aquaculture and aquatic resource management on poverty alleviation. Bangkok: FAO Regional Office for Asia and the Pacific.
- Hallegatte, S. (2009). Strategies to adapt to an uncertain climate change. Global Environmental Change, 19, 240–247.
- Handisyde, N., Telfer, T. C., & Ross, L. G. (2017). Vulnerability of aquaculture-related livelihoods to changing climate at the global scale. Fish and Fisheries, 18(3), 466–488.
- Hanjra, M. A., Blackwell, J., Carr, G., Zhang, F., & Jackson, T. M. (2012). Wastewater irrigation and environmental health: Implications for water governance and public policy. International Journal of Hygiene and Environmental Health, 215(3), 255–269.
- Kengkaj, W. (2015). Sources of social capital in the networks of fish-pond farmers and their implications for adaptive capacity. (AQUADAPT Working Paper 19). Chiang Mai: Unit for Social and Environmental Research, Chiang Mai University.
- Kobayashi, M., Msangi, S., Batka, M., Vannuccini, S., Dey, M. M., & Anderson, J. L. (2015). Fish to 2030: The role and opportunity for aquaculture. Aquaculture Economics & Management, 19(3), 282–300.
- Kotsuke, S., Tanaka, K., & Watanabe, S. (2014). Projected hydrological changes and their consistency under future climate in the Chao Phraya River Basin using multi-model and multi-scenario of CMIP5 dataset. Hydrological Research Letters, 8, 27–32.
- Lacombe, G., Hoanh, C., & Smakhtin, V. (2012). Multi-year variability or unidirectional trends? Mapping long-term precipitation and temperature changes in continental Southeast Asia using PRECIS regional climate model. Climatic Change, 113, 285–299.
- Lavorel, S., Colloff, M. J., McIntyre, S., Doherty, M. D., Murphy, H. T., Metcalfe, D. J., … Williams, K. J. (2015). Ecological mechanisms underpinning climate adaptation services. Global Change Biology, 21(1), 12–31.
- Lebel, L., & Chitmanat, C. (2015). Climate change and aquaculture in Northern Thailand: An assessment of risks and adaptation options. AQUADAPT Project. Retrieved from https://aquadaptorg.files.wordpress.com/2017/10/aquadapt-assessment-report.pdf.
- Lebel, L., & Lebel, P. (2016). Emotions, attitudes, and appraisal in the management of climate-related risks by fish farmers in Northern Thailand. Journal of Risk Research, 1–19. doi:10.1080/13669877.2016.1264450
- Lebel, L., Lebel, P., & Lebel, B. (2016a). Impacts, perception and management of climate-related risks to cage aquaculture in the reservoirs of northern Thailand. Environmental Management, 58(6), 931–945.
- Lebel, P., Sriyasak, P., Kallayanamitra, C., Duangsuwan, C., & Lebel, L. (2016b). Learning about climate-related risks: Decisions of Northern Thailand fish farmers in a role-playing simulation game. Regional Environmental Change, 16(5), 1481–1494.
- Lebel, P., Whangchai, N., Chitmanat, C., & Lebel, L. (2015a). Climate risk management in river-based Tilapia cage culture in northern Thailand. International Journal of Climate Change Strategies and Management, 7(4), 476–498.
- Lebel, P., Whangchai, N., Chitmanat, C., & Lebel, L. (2015b). Risk of impacts from extreme weather and climate in river-based Tilapia cage culture in Northern Thailand. International Journal of Global Warming, 8(4), 534–554.
- Lebel, P., Whangchai, N., Chitmanat, C., Promya, J., Chaibu, P., Sriyasak, P., & Lebel, L. (2013). River-based cage aquaculture of Tilapia in northern Thailand: Sustainability of rearing and business practices. Natural Resources, 4(5), 410–421.
- Lebel, P., Whangchai, N., Chitmanat, C., Promya, J., & Lebel, L. (2015c). Perceptions of climate-related risks and awareness of climate change of fish cage farmers in northern Thailand. Risk Management, 17, 1–22.
- Lempert, R. (2013). Scenarios that illuminate vulnerabilities and robust responses. Climatic Change, 117(4), 627–646.
- Mehta, V., Depsky, N., Forni, L., Purkey, D., & Betancourt, E. (2013). Robust decision support for integrated water resources planning in the Yuba river basin, California. Final report. Boston: Stockholm Environment Institute.
- Navy, H., Minh, T. H., & Pomeroy, R. (2017). Impacts of climate change on snakehead fish value chains in the Lower Mekong Basin of Cambodia and Vietnam. Aquaculture Economics and Management, 21(2), 261–282.
- Nguyen, P. V., Dang, N. H. X., Do, Q. L. N., & Mai, K. T. (2015). The impacts of consumers’ familiarity on their behavioral intentions towards frozen pangasius products: A study in Ho Chi Minh City, Vietnam. Review of European Studies, 7(7), 97–108.
- Paukert, C. P., Lynch, A. J., Beard Jr., T. D., Chen, Y., Cooke, S. J., Cooperman, M. S., … Winfield, I. J. (2017). Designing a global assessment of climate change on inland fishes and fisheries: Knowns and needs. Reviews in Fish Biology and Fisheries, 27(2), 393–409.
- Pickering, T., Ponia, B., Hair, C., Southgate, P., Poloczanska, E., Patrona, L., … De Silva, S. (2011). Vulnerability of aquaculture in the tropical Pacific to climate change. In J. Bell, J. Johnson, & A. J. Hobday (Eds.), Vulnerability of tropical pacific fisheries and aquaculture to climate change (pp. 647–731). Noumea, New Caledonia: Secretariat of the Pacific Community.
- Pimolrat, P., Whangchai, N., Chitmanat, C., Itayama, T., & Lebel, L. (2015). off-flavor characterization in high nutrient load tilapia ponds in northern Thailand. Turkish Journal of Fisheries and Aquatic Sciences, 15, 275–283.
- Pimolrat, P., Whangchai, N., Chitmanat, C., Promya, J., & Lebel, L. (2013). Survey of climate-related risks to Tilapia pond farms in northern Thailand. International Journal of Geosciences, 4, 54–59.
- Rodríguez-Rodríguez, G., & Bande Ramudo, R. (2017). Market driven management of climate change impacts in the Spanish mussel sector. Marine Policy, 83, 230–235.
- Setboonsarng, S., & Edwards, P. (1998). An assessment of alternative strategies for the integration of pond aquaculture into the small-scale farming system of north-east Thailand. Agriculture Economics and Management, 2(3), 151–162.
- Sin-Ampol, P. (2015). Mobility as a response for fish cage farming households in Northern Thailand to multiple risks in the dry season (AQUADAPT Working Paper 34). Chiang Mai: Unit for Social and Environmental Research, Chiang Mai University.
- Sriyasak, P., Chitmanat, C., Whangchai, N., & Lebel, L. (2013). Effects of temperature upon water turnover in fish ponds in northern Thailand. International Journal of Geosciences, 4, 18–23.
- Sriyasak, P., Chitmanat, C., Whangchai, N., Promya, J., & Lebel, L. (2015). Effect of water de-stratification on dissolved oxygen and ammonia in tilapia ponds in Northern Thailand. International Aquatic Research, 7(4), 287–299.
- Travis, W. R. (2014). What is climate risk management? Climate Risk Management, 1, 1–4.
- Troell, M., Naylor, R. L., Metian, M., Beveridge, M., Tyedmers, P. H., Folke, C., … De Zeeuw, A. (2014). Does aquaculture add resilience to the global food system? Proceedings of the National Academy of Sciences, 111, 13257–13263.
- Uppanunchai, A., Apirumanekul, C., & Lebel, L. (2015). Planning for production of freshwater fish fry in a variable climate in northern Thailand. Environmental Management, 56(4), 859–873.
- Uppanunchai, A., Chitmanat, C., & Lebel, L. (2016). Mainstreaming climate change adaptation into inland aquaculture policies in Thailand. Climate Policy, 1–13. doi:10.1080/14693062.2016.1242055
- Watanabe, S., Hirabayashi, Y., Kotsuki, S., Hanasaki, N., Tanaka, K., Mateo, C., … Oki, T. (2014). Application of performance metrics for climate models to project future river discharge in Chao Phraya River Basin. Hydrological Research Letters, 8, 33–38.
- Weaver, C., Lempert, R., Brown, C., Hall, J., Revell, D., & Sarewitz, D. (2013). Improving the contribution of climate model information to decision making: The value and demands of robust decision frameworks. WIREs Climate Change, 4, 39–60.
- Wongsupap, C., Weesakul, S., Clemente, R., & Das Gupta, A. (2009). River basin water quality assessment and management: Case study of Tha Chin River Basin, Thailand. Water International, 34(3), 345–361.
- Yamprayoon, J., & Sukhumparnich, K. (2010). Thai aquaculture: Achieving quality and safety through management and sustainability. Journal of the World Aquaculture Society, 41(2), 274–280.