ABSTRACT
The Colorado River is facing a crisis resulting from demands that have outstripped diminishing supplies, exacerbated by a severe multi-decadal drought and the effects of climate change. As participants directly involved in recent agreements seeking to address this challenge, we describe how a critical basin-wide lens has been developed through information transparency, including the evolution and voluntary exchange of computational models. To achieve greater sustainability, further resolve and commitment from water managers in the United States and Mexico is necessary to ensure the required enhancement of the knowledge systems that will be used to inform extremely difficult and critical decisions.
Introduction
Optimistic projections of water supplies made a century ago, the onset of a severe drought and uncertainties of climate change have forced water managers throughout the Colorado River Basin to transition from emphasizing exploitation of water resources to encouraging sustainable use through enhanced analysis and encouraging dialogue among competing interests (Karambelkar & Gerlak, Citation2020). A key element to seeking more sustainable utilization has increasingly been, and will increasingly be, the evolution of decision support systems to help develop policies in a way that is more inclusive of partners and stakeholders.
The management of the basin relies heavily on data collected, forecasts produced and technical modelling to help inform decision-makers. A critical element of building agreements has been improving the processes by which information is accessed, exchanged and trusted by competing interests. For many years, most information sources and analytical capabilities were developed and maintained within United States’ federal government agencies. As water stress increased, the US federal government recognized the need to level the information playing field among competing stakeholders within and external to its national borders. By increasing access to information and knowledge, the US has sought to dismantle potential risks associated with hegemonic and unequal power dynamics, and position itself as an ‘honest technical broker’ (Butler et al., Citation2021).
A series of successful negotiations over the last 20 years both among the seven US Basin States of the Colorado River and between the US and Mexico can at least partially – or perhaps significantly – be attributed to the use of data and information management tools. While results produced by models have been influential, another important factor has been the role that models have played to develop a shared understanding of the system, identify solutions and build a sense of trust. We leverage the analytical framework of Cash et al. (Citation2003) that describes requirements for knowledge systems, such as water management models, to be credible, salient and legitimate to effectively manage resources. Credibility refers to the scientific adequacy of the technical evidence and arguments, saliency refers to the relevance of the assessment tool for decision-makers, and legitimacy reflects the perception that the information provided is respectful of divergent values, unbiased and fair in its treatment of opposing interests.
We highlight the fact that the co-authors of this Technical Note have been directly involved over the last two decades to bring together stakeholders for these objectives, one having served until 2020 as Regional Director of the Lower Colorado Region for the US Bureau of Reclamation (hereafter Reclamation) and one having served as Mexico’s Commissioner for the International Boundary and Water Commission (IBWC). Furthermore, the lead author served as a consultant to facilitate bi-national cooperation and has supported model interpretation and analyses for various non-governmental organisations (NGOs), states, and Tribal governments through the years. Although sustainability has not yet been fully achieved on the Colorado River, our objective is to share reflections from within recent efforts on the use of models to reach this critical goal and describe the key challenges for future model use.
Physical properties and institutional framework of the Colorado River
The Colorado River Basin is in the south-western United States and north-western Mexico and nourishes a vast region of natural beauty and human-dominated landscapes (). The river emerges from the snow-capped Rocky Mountains, passes through desert canyons and flows into the Sea of Cortez. Along its 1400 mile (2300 km) journey, the river irrigates over 5.7 million acres (2.3 million ha) and supplies water to more than 40 million people in the US and Mexico. The basin also includes the sovereign lands of 30 Native American Tribes, who have used the waters long before European settlement. Although the entire flow of the river is diverted before it reaches the sea, the watercourse sustains threatened and endangered species and allows an extensive recreational economy to thrive.
Figure 1. Map of the Colorado River Basin including lands outside of the basin that use Colorado River water.
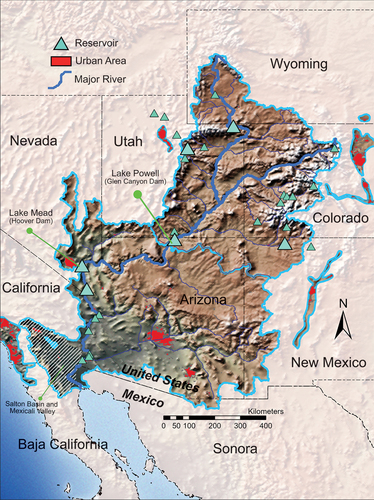
The management of the Colorado River is guided by a compendium of interstate compacts, federal laws, court decrees, secretarial guidelines and a bi-national treaty collectively known as the Law of the River (Macdonnell et al., Citation1995). The first component was the 1922 Colorado River Compact among the US Basin States, which divided the Upper Basin (primarily consisting of Colorado, New Mexico, Utah and Wyoming) from the Lower Basin (primarily consisting of Arizona, California and Nevada), and allocated an equal amount of water (7.5 maf or about 9.25 bcm) for each, as measured at the river’s crossing point between the basins (). Furthermore, the Compact stated that future obligations to Mexico would be shared between the Upper and Lower Basins, which was quantified in 1944 as 1.5 maf/year (about 1.85 bcm/year) in the bi-national treaty.
Figure 2. Timeline of major developments in the Colorado River Basin.
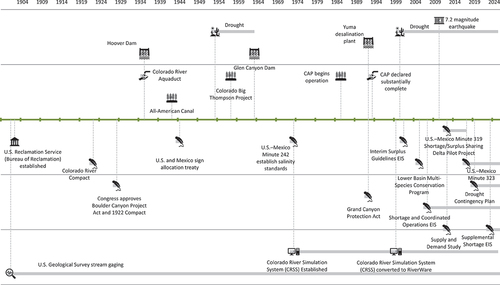
It has long been recognized that the allocation of 16.5 maf/year (about 20.4 bcm/year) exceeds the natural supply of water, which has averaged 14.6 maf/year (18.0 bcm/year) at the Lees Ferry Gauge since 1906. What has been recently understood is the Compact negotiators not only underestimated the available supplies, but also eschewed evidence to the contrary (Kuhn & Fleck, Citation2019). US Geologic Survey studies available at the time suggested average natural flows at Lees Ferry were 15 maf/year or less, while others suggested up to 18.1 maf/year would be available. With momentum for hydropower and irrigation development underway, the negotiators clearly chose to use the more optimistic estimates to help reach an agreement at that time.
Shortly after the Colorado Compact was signed, the Boulder Canyon Project Act of 1928 initiated the construction of the Hoover Dam that would form Lake Mead. This dam could capture almost two years of average flow, eliminate downstream flood risks and assure reliable supplies for Arizona, California, Nevada and, eventually, Mexico. The Glen Canyon Dam was constructed following the Colorado River Storage Project Act in 1956, forming Lake Powell just upstream of the division between the Upper and Lower Basin. The combined storage of these two large reservoirs can capture 3.6 years of average annual flow. Between these two dams sits the Grand Canyon, through which flows are highly regulated, profoundly altering the ecosystem (Bruckerhoff et al., Citation2022).
The Law of the River has endured over a century, but today water managers are facing new challenges that were neither fully addressed by their predecessors nor could have been predicted. With the completion of the Central Arizona Project (CAP) in 1993, river managers were aware the demands for water would soon exceed supplies. Exacerbating overallocation, the average annual natural flows since 2000 have fallen by 20% compared with flows measured before 2000. This current ‘millennium’ drought is substantially driven by climate change and aridification (Milly & Dunne, Citation2020; Udall & Overpeck, Citation2017).
Before and throughout the ‘millennium’ drought, several regulatory measures have reduced consumptive uses. California’s demands once exceeded their 4.4 maf allocation and had become dependent on Arizona’s unused apportionment. Following Arizona’s CAP completion, California relied on the system being declared in surplus conditions (Fulp & Harkins, Citation2001). In 2000, an environmental impact statement (EIS) known as the Interim Surplus Criteria was finalized resulting in a record of decision (ROD) which clarified the conditions by which surplus would be declared. Shortly thereafter, Reclamation launched another EIS process to specify how consumptive uses might be reduced during water-scarce conditions. The 2007 Interim Shortage Guidelines EIS ROD specified consumptive use reductions (i.e., shortage volumes) within Arizona and Nevada as Lake Mead’s elevation declined. As the drought endured, it was apparent that these reductions would be insufficient. The Basin States signed Drought Contingency Plans (DCP) in 2019 that specified further use reductions to all three Lower Basin States and established Upper Basin conservation mechanisms. Parallel to these efforts, two implementation agreements to the 1944 bi-national treaty, known as Minutes 319 and 323, were signed in 2012 and 2017, respectively, that inter alia would reduce Mexico’s uses generally in proportion to the reductions in the US. Once the DCP was agreed to in the US, a Binational Water Scarcity Contingency Plan specified in Minute 323 also came into effect.
Despite these measures, the combined storage of lakes Powell and Mead has fallen from 95% full in 2000 to merely 25% full in 2023 and Glen Canyon Dam is facing the possible loss of hydropower generation (Wheeler et al., Citation2022). To protect hydropower facilities, the US federal government asserted that it would impose larger reductions on water users if the drought persists and the states are not able to reach an agreement on their own volition. A Supplement to the Interim Shortage Guidelines (SEIS) is underway to specify these actions. Furthermore, all existing agreements that require consumptive use reductions are set to expire by 2026. Preparations for a subsequent post-2026 EIS and new bi-national negotiations are underway, both of which are expected to embrace a much wider range of hydrologic uncertainty.
The growing role of models in the Colorado River
Policies for the Colorado River are shaped by data-driven analyses. The use of computers to manage the river began in the late 1970s with the development of FORTRAN-based models by Reclamation. These models included the Colorado River Simulation System (CRSS) for long-term planning, a 24-month study model for developing Annual Operation Plans (AOP), and short-term models to schedule dam releases (Stevens, Citation1986). Simulations using the CRSS are conducted over a multi-decade planning horizon to examine implications of proposed management policies across a variety of potential future conditions. These results provide insight to better understand the impacts of proposals on consumptive and non-consumptive uses as well as other resources. The initial focus of these models was their saliency to support practical decision-making.
The FORTRAN-based CRSS model was initially run on a mainframe computer. To increase accessibility, in 1995 the CRSS was ported to Intel-based personal computers and workstations. As the physical and regulatory system increased in complexity, new infrastructure, diversions and operational policies needed to be added to the CRSS maintain its credibility. However, the model was ‘hard-wired’ with respect to the spatial configuration of the elements being simulated, as well as the logic that simulated the system operation, making it difficult to modify. As the original model developers were reaching a retirement age, a deep institutional knowledge of the CRSS was at risk of being lost. Furthermore, a wider variety of stakeholders desired to become more knowledgeable and engaged with the management of the river. It was clear that the existing design of the CRSS would soon become insufficient to meet future needs (Fulp et al., Citation1999).
To respond to this challenge, Reclamation teamed with the Tennessee Valley Authority in 1994 to sponsor a research and development programme to create a flexible modelling system that could be applied in multiple contexts. The product was a software platform known as RiverWare (Zagona et al., Citation2001), developed by the Center for Advanced Decision Support for Water and Environmental Systems (CASDWES) at the University of Colorado Boulder. With a graphical user interface (GUI), RiverWare allows models to be more transparent and customizable. Users can add, remove or modify objects that represent physical water features and write hierarchical rules that represent operational policies. Reclamation sought to replicate the FORTRAN-based CRSS in the RiverWare platform, resulting in a RiverWare-based version of the CRSS.
Enhancing the legitimacy of the CRSS was also an objective of Reclamation. Alongside the RiverWare and the CRSS model development team, stakeholders were invited to join a user involvement group to facilitate the development process that aimed to ensure usability and increase acceptance by key stakeholders (Fulp et al., Citation1999). The tasks for this group were (1) to discuss and review model requirements; (2) to suggest improvements and functionalities; (3) to develop problem scenarios to direct the design, development and testing phases; (4) to participate in testing of the models; and (5) to be focal points for information transfer to and from the respective organizations. Colorado River participants included water management agencies from the Basin States, water districts and other federal agencies. Other water users, notably the Mexican government, Tribal water users, and non-governmental groups advocating for environmental concerns, were not involved. By 1996 the FORTRAN-based CRSS had been replicated by RiverWare. Other efforts have been made to simulate the Colorado River (Sánchez et al., Citation2009), however the complexities of the Law of the River are insufficiently depicted. Other models focus on portions of the basin with greater spatial and temporal resolution (Medellín-Azuara et al., Citation2007; Parsons & Bennett, Citation2006), but the CRSS remains the most credible depiction of the basin-wide river and its management.
With demands in the basin nearly exceeding supplies, the new RiverWare-based CRSS would be used in forthcoming efforts to address the imbalance. Reaching new agreements would also require significant stakeholder understanding and acceptance of Reclamation’s analyses, thus methods to effectively communicate model outputs were needed.
During the development of the Interim Surplus and Shortage EISs during the late 1990s and 2000s, Reclamation held multiple stakeholder meetings with state and Tribal governments, irrigation districts, municipalities, NGOs, recreational interests, etc. who had interests in the implications of proposed policies. Communicating complex model outputs was facilitated by a graphical policy analysis tool (GPAT) that statistically analyses model results and generates plots to compare the impacts of the proposals.
To provide a deeper understanding of the modelling process, encourage engagement and solicit feedback, Reclamation officially launched a Colorado River Stakeholder Technical Modelling Work Group in March 2010, comprised primarily of technically oriented participants from interested stakeholder groups and their consultants. Since its inception, this group has become a community through which model updates and results are discussed in online seminars and disseminated via web-based files. Participants can download the model to examine how the results were developed and provide direct feedback to Reclamation. Differing from the user involvement group formed during the conversion of the CRSS into the RiverWare platform, this group encompasses a wider array of stakeholders, some with strong modelling skills and others who may lack modelling backgrounds but have dedicated effort to learn how the model works and interpret the outputs. Anyone is allowed to join this group, but participants must request to be added to an electronic mailing list. Since the formation of this group, engagement has substantially increased and includes participants from state and federal agencies, municipalities, irrigation districts, NGOs, academics, Tribal Nations, Mexican government agencies and consultants supporting these stakeholders. Reclamation does not yet provide formal training on the CRSS but works with stakeholders to help understand and use the model.
By Reclamation visually demonstrating model results, facilitating access, answering questions regarding assumptions and being explicit about model limitations, many stakeholders have increasingly accepted the CRSS as the most useful portrayal of the river system (Wheeler et al., Citation2018). However technical RiverWare training and purchasing a licence remain practicalities to fully understand and use the CRSS.
Modelling for the bi-national process
The CRSS has been widely used to enhance bilateral cooperation between the United States and Mexico (Rivera-Torres et al., Citation2021). The 2000 Interim Surplus Criteria and 2007 Interim Shortage Guidelines expressly considered surplus and shortage conditions within the United States, but for the Basin States to agree to the provisions proposed, there was a recognition that Mexico would be included in a sequential bi-national process. Prior to 2007, the Mexican water managers had minimal information on how the US dams were operated, nor did they have access to the models being used by Reclamation. With a growing pressure of shortages, this information asymmetry presented a challenge for both countries. For Mexico to agree to adjust their deliveries under drought conditions, they needed better information on how water was managed in the US.
Mexico understood the important role CRSS has played over the years, and the US recognized the advantage of negotiating with an empowered partner. In 2008, Mexico’s National Water Commission (CONAGUA) requested CADSWES to provide a RiverWare training in Mexico City, inviting their technical staff, participants from the Mexican section of the International Boundary and Water Commission (CILA), and researchers from universities. Reclamation thereafter provided direct access to the CRSS model and responded to enquiries regarding model details. By Mexican institutions building internal capacity and the US providing transparency, the CRSS model served as an effective boundary object that facilitated a mutual understanding of the system and promoted dialogue both across the border and between science and policymakers (Wheeler et al., Citation2018).
To further prepare for forthcoming negotiations, a conceptual cooperation framework was being established when an earthquake struck in April 2010 that damaged distribution canals, inhibiting Mexico’s ability to fully use their annual allocation. This window of opportunity led to the signing of Minute 318 of the 1944 Treaty, allowing Mexico to temporarily store unused water in Lake Mead. Negotiations began shortly thereafter to reach a more comprehensive agreement between the two countries, which continued for over one year with face-to-face meetings held on alternating sides of the border and included representatives from national and state governments, major water users, and NGOs.
Throughout the negotiations, proposed operational policies were evaluated using the CRSS. Staff from Reclamation, CONAGUA, IBWC/CILA jointly and iteratively developed alternatives and tested them using the CRSS, with regular input from state agency representatives, irrigation and municipal districts, and NGOs. The result was Minute 319 to the 1944 Treaty that presented a five-year arrangement specifying how Mexico would reduce uses during times of shortage, receive additional water during times of surplus, extend Mexico’s ability to store water within Lake Mead, allow US-funded water conservation efforts in Mexico, and establish a pilot programme to restore limited flows to the once ecologically vibrant Colorado River Delta. Provisions developed were expanded in Minute 323, which would come into effect in alongside the Drought Contingency Plan. With Minute 323 expiring by 2026, the two nations will again rely on the CRSS to reach a new agreement on how to bring the river to a more sustainable state.
Challenges for future model use
Throughout policy development since 2000, the RiverWare based CRSS was used extensively to inform negotiations and identify viable management alternatives. Throughout these efforts, numerous legitimate concerns have been raised by stakeholders regarding the assumptions, abilities and limitations of the CRSS to adequately represent the risks being faced. These challenges however are not simply model limitations but provide Reclamation an opportunity to enhance the CRSS and stakeholders with an opportunity to become engaged in the problem-solving process using a basin-wide lens. We highlight four such challenges and provide insights on how these might be addressed.
The challenge of hydrologic assumptions
The signatories of the 1922 Colorado River Compact recognized the amount of water assumed to be available profoundly shapes how much can be allocated. Recent EIS efforts faced a similar situation when considering how far consumptive uses must be reduced. Beginning in the 1980s with the original CRSS, the primary assumption guiding the selected policies was that future hydrologic conditions are likely to mimic the conditions of the past. More specifically, Reclamation assumed that each year of a reconstructed basin-wide gaged record, which goes back to around 1906, is equally likely to occur in the future. Although Reclamation began research efforts in the early 2000s to enable the use of alternative hydrologic scenarios in CRSS based on tree-ring records and climate modelling output, the gaged record continued to be used for decision-making until recently, despite the growing body of scientific evidence that climate change could profoundly impact the Colorado River (Milly & Dunne, Citation2020; Udall & Overpeck, Citation2017).
As our understanding of climate change has increased, the implications have been incrementally incorporated into the CRSS. Reclamation’s 2012 Basin Study used a wide variety of climate change projections to assess possible supply–demand imbalances and identify potential solutions. The 2019 DCPs focused on a ‘stress-test’ hydrology, which only used historical flows since 1988, implicitly acknowledging that recent hydrologic conditions are more likely to be representative of the future.
Incorporating hydrologic uncertainty will continue to be challenging, particularly in contentious negotiations that seek agreements over specific allocations or shortage volumes. In the forthcoming renegotiation of the post-2026 agreements, Reclamation is not only incorporating climate change projections into the CRSS model inputs, but also invoking a ‘deep uncertainty’ approach that seeks to avoid any presumption of how statistically likely any future projection may be. A focus will be to seek options that are robust to a deeply uncertain future (Smith et al., Citation2022). Since we no longer can assume what the future average hydrology will be, planning for multiple possible future ‘average’ conditions will be critical, including a continuation or worsening of the current drought. Sharing this new approach through the Stakeholder Technical Modelling Work Group will be critical to understanding and adapting to this unknown future.
The challenge of demand assumptions
The use of the CRSS as an authoritative modelling tool for Colorado River management has required broad acceptance of its assumptions from key stakeholders. Foremost in this stakeholder group are water agencies of the Basin States, who provide projections of future uses. The Lower Basin States already fully use their 7.5 maf (about 9.25 bcm) apportionment as defined in the Colorado River Compact, however the Upper Basin States have not yet developed their entire equivalent water right. Despite no significant increases in Upper Basin uses for over 30 years, the Upper Colorado River Commission (UCRC) provides Reclamation with projections of aspirational future water use increases to safeguard their future development (Wang et al., Citation2020). When incorporating these assumptions within the CRSS, the result is a systematic overestimation of risks to downstream water users.
Considering both aspirational and likely demand futures will be critical in an increasingly constrained system. This is particularly important to maintain the credibility of the CRSS analyses. Reclamation’s 2012 Basin Study used a number of development ‘storylines’ to demonstrate future demands and the CRSS can readily be used to evaluate alternative demand policies (Wheeler et al., Citation2022). Future operational policies may require exploring various demand management approaches, and a recognition of the political nature of assumptions embedded into the CRSS.
The challenge of spatial and temporal resolution
The CRSS originally focused on the operation of Lakes Powell and Mead to meet the demands of the Lower Basin. As uses of the model increased, expectations of what it could provide also increased. The CRSS operates on a monthly basis, therefore aspects that require sub-monthly time scales (e.g., daily or hourly flows) cannot, in general, be explicitly simulated or evaluated with the CRSS such as certain environmental or hydropower objectives that require large flows over short periods of time. Simulating such process generally requires post-processing monthly CRSS results.
Limitations on the spatial resolution of the CRSS have also been highlighted, particularly within the Upper Basin where a vast number of tributaries and water diversions exist. The CRSS aggregates multiple small inflows and diversions, therefore accuracy limitations exist when examining the impacts on specific reaches and users. Furthermore, the CRSS does not simulate water rights within states, which are better represented by local models.
Knowing how the CRSS works can help stakeholders understand what information Reclamation can and cannot realistically provide, hence clarifying its salience for developing particular policies. Furthermore, enhanced stakeholder engagement can provide recommendations on how to improve model resolution.
The challenge of growing regulatory and model complexity
The development of the RiverWare-based CRSS was motivated by the desire to simulate evolving policies alongside increased transparency of the model and assumptions. However, as the CRSS has been used to support the evolution of increasingly complex policies, the model itself has become increasingly complex for users to understand and manage. Despite significant efforts by Reclamation to make the model more accessible, many stakeholders continue to perceive the CRSS as a ‘black box’ that few agencies or individuals can significantly modify. Making changes to the model is non-trivial and requires a high degree of modelling expertise. As the physical and regulatory environment becomes more complex, Reclamation must reflect those complexities in the CRSS to maintain its credibility, however this must be balanced with the legitimacy of the model in the eyes of stakeholders by making the model structure understandable while continuing to make it accessible to well-trained stakeholders.
Conclusions
The aim of this article has been to describe how the CRSS has been increasingly important for managing the Colorado River and illustrate how it will be integral to develop policies that will enable reaching a more sustainable state. Ever since it became clear that the negotiators of the 1922 Colorado Compact overestimated the amount of water available, the basin has known that a day of reckoning would arrive when the demands on the system would exceed supplies. This reckoning came more abruptly than expected with the onset of a severe drought beginning in 2000. Furthermore, the effects of climate change and aridification are accelerating the need to reduce basin-wide demands even more to match an increasingly uncertain supply. Without a doubt, a basin-wide lens will be indispensable to devising and implementing such policies.
The widespread use of the CRSS for facilitating recent Colorado River policy development has made the strengths and limitations of the model explicit and provided insight to how it can be used in the future. Avoiding further system decline will require additional and extensive analyses of unknown futures, which will necessitate increased stakeholder engagement with the assumptions and results of those analyses. Identifying the most applicable supply and demand scenarios is critical to maintain the credibility of the model. Furthermore, enhancing stakeholder’s understanding of the spatial and temporal characteristics of the CRSS, as well as how it represents elements of the Law of the River, will increase the model’s legitimacy and augment its saliency for addressing challenges and concerns.
Despite the best efforts of the authors and many others involved in the management of the Colorado River over recent decades, the system storage has continued to decline. To achieve a more sustainable state, we believe the CRSS model will continue to be an important tool to support policy development in the Colorado River and there will be an ever-greater need for stakeholders and water managers to embrace a basin-wide perspective to evaluate possible future risks and identify appropriate responses.
Disclosure statement
Kevin Wheeler has provided consulting services for the United States Bureau of Reclamation, State of Colorado, Central Arizona Project, Environmental Defense Fund, Pacific Institute, Western Resource Advocates, and Utah State University. Terrance Fulp served from 2012 until 2020 as the Regional Director of the Lower Colorado Region for the United States Bureau of Reclamation. Roberto F. Salmon Castelo served from 2009 until 2020 as the Mexican Commissioner for the International Boundary and Water Commission (IBWC).
Additional information
Funding
References
- Bruckerhoff, L. A., Wheeler, K., Dibble, K. L., Mihalevich, B. A., Neilson, B. T., Wang, J., Yackulic, C. B., & Schmidt, J. C. (2022). Water storage decisions and consumptive use may constrain ecosystem management under severe sustained drought. JAWRA Journal of the American Water Resources Association, 58, 654–672. https://doi.org/10.1111/1752-1688.13020
- Butler, A., Fulp, T., Prairie, J., & Witherall, A. (2021). Water resources management in the Colorado River Basin. In R. Ferrier & A. Jenkins (Eds.), Handbook of catchment management 2e (pp. 441–463). Wiley-Blackwell.
- Cash, D. W., Clark, W. C., Alcock, F., Dickson, N. M., Eckley, N., Guston, D. H., Jäger, J., & Mitchell, R. B. (2003). Knowledge systems for sustainable development. Proceedings of the National Academy of Sciences, 100(8), 8086–8091. https://doi.org/10.1073/pnas.1231332100
- Fulp, T., Harkins, J. (2001). Policy analysis using RiverWare: Colorado River interim surplus guidelines. In D. Phelps & G. Shelke (Eds.), World water and environmental resources congress – Bridging the gap (pp. 1–10). American Society of Civil Engineers.
- Fulp, T., Vickers, W., Williams, B., & King, D. (1999). Replacing an institutional model: The Colorado River simulation system exampled. In Proceedings of the ASCE Waterpower ’99 Conference.
- Karambelkar, S., & Gerlak, A. K. (2020). Collaborative governance and stakeholder participation in the Colorado River Basin: An examination of patterns of inclusion and exclusion. Natural Resources Journal, 60, 1–46. https://www.jstor.org/stable/26912770
- Kuhn, E., & Fleck, J. (2019). Science be dammed: How ignoring inconvenient science drained the Colorado River. University of Arizona Press.
- Macdonnell, L. J., Getches, D. H., & Hugenberg, W. C. (1995). The law of the Colorado River: Coping with severe sustained drought 1. JAWRA Journal of the American Water Resources Association, 31(5), 825–836. https://doi.org/10.1111/j.1752-1688.1995.tb03404.x
- Medellín-Azuara, J., Lund, J. R., & Howitt, R. E. (2007). Water supply analysis for restoring the Colorado River Delta, Mexico. Journal of Water Resources Planning and Management, 133(5), 462–471. https://doi.org/10.1061/(ASCE)0733-9496(2007)133:5(462)
- Milly, P. C. D., & Dunne, K. A. (2020). Colorado River flow dwindles as warming-driven loss of reflective snow energizes evaporation. Science, 367(6483), 1252–1255. https://doi.org/10.1126/science.aay9187
- Parsons, R., & Bennett, R. (2006). Reservoir operations management using a water resources model. Operating Reservoirs in Changing Conditions, 304–311. https://doi.org/10.1061/40875(212)3
- Rivera-Torres, M., Gerlak, A. K., & Jacobs, K. L. (2021). Lesson learning in the Colorado River Basin. Water International, 46(4), 567–577. https://doi.org/10.1080/02508060.2021.1913782
- Sánchez, H., González, E., Patiño, C., & Villalobos, A. (2009). Surface water management model for the Colorado River Basin. In C. A. Brebbia & V. Popov (Eds.), WIT transactions on ecology and the environment (pp. 35–46). WIT Press.
- Smith, R., Zagona, E., Kasprzyk, J., Bonham, N., Alexander, E., Butler, A., Prairie, J., & Jerla, C. (2022). Decision science can help address the challenges of long-term planning in the Colorado River Basin. JAWRA Journal of the American Water Resources Association, 58(5), 735–745. https://doi.org/10.1111/1752-1688.12985
- Stevens, D. (1986). Computer models in lower Colorado River operations. Journal of Water Resources Planning and Management, 112(3), 395–408. https://doi.org/10.1061/(ASCE)0733-9496(1986)112:3(395)
- Udall, B., & Overpeck, J. (2017). The 21st century Colorado River hot drought and implications for the future. Water Resources Research, 53(3), 2404–2418. https://doi.org/10.1002/2016WR019638
- Wang, J., Wheeler, K., Rosenberg, D., & Schmidt, J. C., 2020. Managing the Colorado River for an uncertain future The Future of the Colorado River Project. Utah State University, https://qcnr.usu.edu/coloradoriver/files/WhitePaper4.pdf
- Wheeler, K. G., Robinson, C. J., & Bark, R. H. (2018). Modelling to bridge many boundaries: The Colorado and Murray–Darling River basins. Regional Environmental Change, 18(6), 1607–1619. https://doi.org/10.1007/s10113-018-1304-z
- Wheeler, K. G., Udall, B., Wang, J., Kuhn, E., Salehabadi, H., & Schmidt, J. C. (2022). What will it take to stabilize the Colorado River? Science, 377(6604), 373–375. https://doi.org/10.1126/science.abo4452
- Zagona, E. A., Fulp, T. J., Shane, R., Magee, T., & Goranflo, H. M. (2001). Riverware: A generalized tool for complex reservoir system modeling 1. Journal of the American Water Resources Association, 37(4), 913. https://doi.org/10.1111/j.1752-1688.2001.tb05522.x