Abstract
Seedlings growing in acid soils suffer both phosphorus (P) deficiency and aluminium (Al) toxicity stresses. An experiment was conducted to study the effects of Al and P interaction on Al-tolerant (ET8) and Al-sensitive (ES8) wheat genotypes in an acid soil. This study aimed to determine the interactive effect of Al and P in soil and within plant tissue. Three Al levels (0, 50 and 150 mg aluminium chloride [AlCl3] kg−1 soil) and five P levels (0, 20, 40, 80 and 160 mg P kg−1) were used in this experiment. Bulk soil pH decreased with addition of AlCl3 to the soil. Bulk soil pH increased at 160 mg P kg−1 supply at three Al levels. Phosphorus supply reduced extractable Al in the soil in all Al treatments. Seedlings suffered more from Al toxicity in the absence of P supply. Increasing P supply resulted in increased seedling biomass under high Al toxicity. This high P ameliorated Al toxicity in the soil and may enhance ES8 growth under Al toxicity. The Al × P interaction affected the root and shoot P concentration in both genotypes and P supply had no effect on P translocation to the shoot. These results suggested that differential growth behaviour between ES8 and ET8 plays an important role in tolerance to Al toxicity irrespective of high P supply. It is concluded that the higher aluminium cation (Al3+) binding capacity in the root apoplast of ET8 seedlings contributes to its improved tolerance to Al toxicity compared to ES8 seedlings.
Introduction
Aluminium (Al) toxicity and phosphorus (P) deficiency occur together (de Miranda and Rowello Citation1989) and are the two major limiting factors for crop production in acid soils (Kochian et al. Citation2004, Fukuda et al. Citation2007, Du et al. Citation2009). The chemical reactions of Al and P are able to lower the activity of aluminium cations (Al3+) in the soil solution (Sanzonowicz et al. Citation1998, Silva et al. Citation2001, Nakagawa et al. Citation2003). Most studies consider Al toxicity and P deficiency as independent factors to evaluate the effect of P deficiency or Al toxicity on plant growth (Foy Citation1988, Yan et al. Citation1995). The limited studies on Al and P interaction in plants were conducted in solution culture (Tan and Keltjens Citation1990, Nian et al. Citation2003, Dong et al. Citation2004) with only a small number of studies being conducted in a soil system (Liao et al. Citation2006). Understanding the mechanisms underlying Al and P interactions in plants grown in a soil system is necessary to facilitate the development of acid-tolerant crops.
Genotypic differences in Al tolerance have been identified in many crops including wheat (Delhaize et al. Citation1993a, de Sousa Citation1998, Kariuki et al. Citation2007, Iqbal Citation2012a). Previous studies have suggested that the genotypic variation in Al tolerance between Al-sensitive (ES8) and Al-tolerant (ET8) wheat genotypes is associated with the exudation of malate under Al stress condition (Delhaize et al. Citation1993a, Delhaize et al. Citation1993b, Delhaize and Ryan Citation1995). However, no information is available about Al and P interactions on ET8 and ES8 seedlings grown in an acid soil.
A previous study showed that P enhances Al tolerance in Al-sensitive (ES8) wheat seedlings (Iqbal et al. Citation2010). However, it is unknown whether P enhances Al tolerance in Al-tolerant (ET8) wheat seedlings or the extent to which this might occur. The genotypic variation in Al and P interaction in an acid soil is necessary to understand how P is involved in Al-tolerance mechanisms. The aim of the present study was to investigate genotypic differences between ET8 and ES8 wheat seedlings in response to Al toxicity in soil, and how these differences might be affected by the addition of soluble P to the soil. It was hypothesised that ES8 would respond better than ET8 to P application in acid Al-toxic soils.
Materials and methods
Soil and plants
The subsoil of a Podsol (Isbell Citation2002) was collected from Frankston, Victoria (38°06′ S, 145°16′ E). The wheat (Triticum aestivum L.) ET8 and ES8 genotypes were used as test plants. Soil properties are described in .
Table 1. Properties of soils used in this study
Plant genotypic characteristics
The ES8 and ET8 wheat genotypes are near-isogenic (over 95%) lines differing in Al tolerance at the ALMT1 gene (Ahn and Matsumoto Citation2006). Both ET8 and ES8 lines were derived from a cross between the Al-tolerant cultivar Carazinho and the Al-sensitive cultivar Egret, andthe resulting progeny backcrossed eight times to Egret or derivatives of Egret (Fisher and Scott Citation1987).
Experimental design and procedure
The experiment consisted of three levels of Al (0, 50 and 150 mg aluminium chloride [AlCl3] kg−1 soil), five levels of P (0, 20, 40, 80 and 160 mg P kg−1) and two wheat genotypes (both ET8 and ES8) with three replications. The experiment was set up with a completely randomised design (Mendoza and Borie Citation1998). Both AlCl3 and dipotassium orthophosphate (KH2PO4) were used as the Al and P sources, respectively (Iqbal et al. Citation2010, Iqbal Citation2012b). The soil was preincubated with different levels of added Al at 30 °C for 7 d. Water content was maintained at 70% field capacity (9%, w/w). Dipotassium orthophosphate was added to pre-incubated soils and mixed well before sowing. Eight uniform pre-germinated seeds were sown in each plastic cup containing 200 g treated soil. The cups were covered with filter paper 2 days after sowing (DAS). Basal nutrients were not applied to minimise possible interactions between nutrients, P and Al within the soil. Water was sprayed twice per day (9:00 and 18:00) on the soil surface during plant growth. Seedlings were grown in a growth cabinet with temperature maintained at 20 °C day/18 °C night, a 10 h dark/14 h light photoperiod and average light intensity of 210 µM photons m−2 s−1.
Plant harvest and collection of rhizosphere soil
Plants were harvested 8 DAS. Whole plants were removed from each cup by gently agitating the cup to provide minimum disturbance to the roots and shoots. Intact plants were then lifted gently from the soil and shaken lightly to remove bulk soil from the roots. The remaining soil adhering to the roots of wheat seedlings was removed by gentle dunking in deionised water (DI), and was treated as rhizosphere soil. Collected rhizosphere soil was air-dried and stored in a controlled-temperature (25 °C) room until analysis. The shoot and root were separated and the shoot was dried at 70 °C for 3 d and retained for analysis. The separated roots were washed 3–4 times with deionised water (Mendoza and Borie Citation1998). Then, the whole root system was scanned with a root-system scanner using the WinRHIZO image analysis system (WIN MAC, Regent Instruments, Quebec, Canada; http://www.regentinstruments.com/) (Arsenault et al. Citation1995). The scanner was set at 13 × 19 cm for standardisation of the scanner area. After measurement of root length, roots were washed in deionised water and dried at 70 °C in an oven for 3 d and retained for analysis.
Collection and analysis of apoplast aluminium
After collection of rhizosphere soil, the separated roots were washed in DI and placed in 50 ml vials that contained 25 ml of 50 mM BaCl/2 solution to desorb Al from the root apoplast. All vials were shaken every 15 min during collection of apoplast Al and chilled to 4 °C for 45 min. After collection of apoplast Al, roots were washed with DI water and dried in oven at 70 °C for a minimum 3 d before analysis. The Al desorbed from the root apoplast was determined colourimetrically by the pyrocatechol violet (PCV) method at 585 nm (Kerven et al. Citation1989). A 50 mM BaCl2 solution was used for preparation of the Al standards during analysis of apoplast Al.
Soil analyses
Bulk and rhizosphere soil pH was determined with a 1:5 (v/v) extraction in 0.01 M CaCl2 after overnight shaking (17 h) (Weligama et al. Citation2010). Extractable Al in bulk soil was determined using the PCV method in 0.01 M CaCl2 extracts (Kerven et al. Citation1989).
Plant tissue analyses
All root and shoot samples were cut into small pieces and digested in a mixture of concentrated nitric and perchloric acids (4:1, v/v) by heating stepwise using a Tector DS 400 digestion system (Varian Australia Pty, Melbourne, Australia) until 230 °C was reached and then held for 20 min at this temperature. The Al concentration in the digest was determined colourimetrically using the PCV method (Kerven et al. Citation1989). The concentration of P in the extract was determined by the molybdovanado-phosphate method (Bassett et al. Citation1978).
Statistical analysis
Results were analysed with a two-way or three-way analysis of variance (ANOVA) using GenStat for Windows 5th edition (Lawes Agricultural Trust, Rothamstead, UK).
Results
Effect of aluminium and phosphorus addition on extractable aluminium and soil pH
The extractable Al in bulk soil declined significantly (P< 0.05) as P supply increased in all Al treatments. In contrast, the extractable Al in bulk soil increased significantly (P < 0.05) as AlCl3 concentration increased in the absence of P supply. The decrease in extractable Al concentration with P supply was more marked at 150 mg kg−1 AlCl3 than at 0 or 50 mg kg−1 AlCl3 (a, ).
Figure 1. Effect of Al and P addition on (a) extractable Al in bulk soil, (b) bulk soil pH and (c) rhizosphere pH. Data are the mean of Al-sensitive (ES8) and Al-tolerant (ET8) wheat genotypes. The vertical bar represents the LSD (P = 0.05) for the Al × P interaction
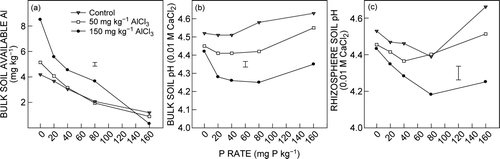
Table 2. Significance levels for the main and interactive effect of Al, P and genotypes on extractable Al in bulk soil, bulk soil pH and rhizosphere soil pH. Values are the means of six replicates for bulk soil pH and extractable Al in bulk soil, and three replicates for rhizosphere pH. Incubation was performed for 7 d at 30 °C for Al treatment and P was added directly to soils before sowing. NA = no data available
At 0 AlCl3, bulk soil pH tended to increase as P supply increased. However, with nil P supply the bulk soil pH declined significantly (P < 0.05) as AlCl3 concentration increased. Bulk soil pH for the 50 and 150 mg AlCl3 kg−1 treatments declined as P supply increased from 0 to 20 mg P kg−1, and remained stable up to 80 mg P kg−1and increased as P supply increased to 160 mg P kg−1 (b, ).
Rhizosphere soil pH did not differ significantly between the genotypes (P > 0.05). Rhizosphere soil pH declined as AlCl3 concentration increased in all of the P treatments. Rhizosphere pH decreased as P supply increased from 0 to 80 mg P kg−1 for the 0 and 150 mg AlCl3 kg−1 treatments and to 40 mg P kg−1 for the 50 mg AlCl3 kg−1 treatment, and increased as P supply further increased (c, ).
Plant growth responses
With nil AlCl3, the mean root length of ES8 seedlings increased when P supply increased from 0 to 20 mg kg−1, and then declined linearly with further increase in P supply. With 50 mg AlCl3 kg−1, the mean root length of ES8 seedlings peaked at 40 mg P kg−1 and then declined sharply with further addition of P. With 150 mg AlCl3 kg−1, the mean root length of ES8 seedlings was low and remained similar up to 40 mg P kg−1, and peaked at 80 mg P kg−1 (a, ).
Figure 2. Effect of Al and P addition on mean root length of (a) ES8 and (b) ET8, plant height of (c) ES8 and (d) ET8, and shoot dry weight (e) under G × P interaction and (f) under Al × P interaction. Data are average values of ES8 and ET8 after 8 d of growth. The vertical bar represents the LSD (P = 0.05) for the Al × P × G interaction for (a) to (d)
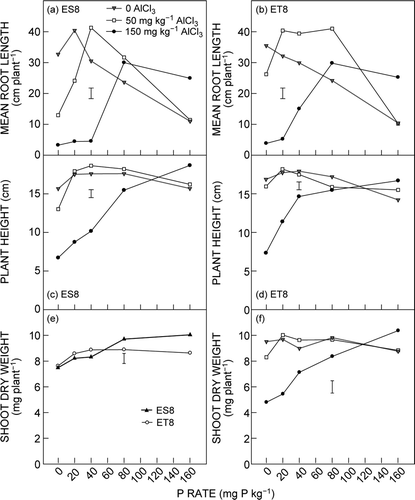
Table 3. Level of significance for the main and interactive effect of Al, P and genotypes on root length, root dry weight, plant height and shoot dry weight after 8 days of growth. Values are the means of three replicates for all measurements
At 0 and 50 mg AlCl3 kg−1, plant height of ES8 seedlings increased significantly (P < 0.05) when P supply increased from 0 to 20 mg kg−1 and remained constant up to 80 mg P kg−1, then slightly declined at 160 mg P kg−1. At 150 mg AlCl3 kg−1, plant height increased linearly and reached the maximum at 160 mg P kg−1. The plant height of ET8 in response to P supply showed a similar pattern to that of ES8. ET8 plants generally were taller than ES8 plants when P supply was low (c and d, ).
On average for the two genotypes, shoot dry weight increased with increasing P supply and reached the maximum at 40 mg P kg−1 for ES8 and at 80 mg P kg−1 for ET8. ES8 produced similar shoot biomass as ET8 at P supply ≤40 mg kg−1 but greater shoot biomass than ET8 with higher P supply (e).
When averaged over the two genotypes, shoot dry weight was not affected by P supply at 0 AlCl3. At 50 mg AlCl3 kg−1, shoot biomass slightly increased when P supply increased from 0 to 20 mg kg−1 and did not increase further at higher P supply. At 150 mg AlCl3 kg−1, shoot dry weight increased linearly with increasing P supply. There was a clear interaction between Al and P supply on shoot growth. Compared with 0 and 50 mg AlCl3 kg−1, the addition of 150 mg AlCl3 kg−1 markedly decreased shoot biomass at low supply of P but slightly increased shoot biomass at 160 mg P kg−1 (f).
Phosphorus concentrations in plants
There were no statistically significant differences in shoot P concentration between the two genotypes, nor were there any genotype interactions with P or Al additions. With nil AlCl3, P concentration in the shoot of ES8 seedlings peaked at 20 mg P kg−1 and decreased as P supply increased from 20 to 40 mg P kg−1 and remained similar up to 160 mg P kg−1. With 50 mg AlCl3 kg−1, P concentration in shoots increased gradually as P supply increased up to 160 mg P kg−1. At 150 mg AlCl3 kg−1, P concentration in the shoot did not increase when P supply increased from 0 to 40 mg P kg−1 but linearly increased as P supply further increased. The treatment effect on P concentration in the shoot of ET8 followed the same pattern (a and b, ).
Figure 3. Effect of Al and P addition on concentration of P in the shoot of (a) ES8 and (b) ET8, in the roots of (c) ES8 and (d) ET8, and total P uptake in (e) ES8 and (f) ET8. The vertical bar represents the LSD (P = 0.05) for the Al × P × G interaction
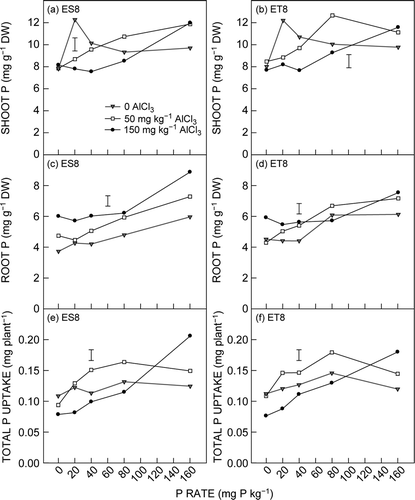
Table 4. Level of significance for the main and interactive effect of Al, P and genotypes on shoot P concentration, root P concentration and total P uptake. Values are the means of three replicates for all measurements
The concentration of P in the root generally increased with increasing P supply with all Al treatments, and the increase was greater in ES8 than ET8. In general,increasing Al addition increased P concentration but the effect of Al was less for ET8 than ES8 (c and d).
With nil AlCl3, total P uptake tended to increase with increasing P supply and peaked at 80 mg P kg−1 for ET8. At 50 mg AlCl3 kg−1, total P uptake increased with increasing P supply and also peaked at 80 mg P kg−1. However, with 150 mg AlCl3 kg−1, total P uptake linearly increased with increasing P supply up to 160 mg kg−1; the increase was greater in ES8 than ET8. On average, total P uptake was greater in ET8 than ES8 at 0 and 50 mg AlCl3 kg−1, respectively (e and f).
Aluminium concentrations in plants
The concentration of Al in the root apoplast exponentially decreased as P supply increased. Increasing Al addition increased the concentration of apoplast Al at 0 P supply and the apoplast Al in ET8 was double that in ES8 (a and b, ).
Figure 4. Effect of Al and P on root apoplast Al of (a) ES8 and (b) ET8. The vertical bars represent the LSD (P = 0.05) for the Al × P × G interaction
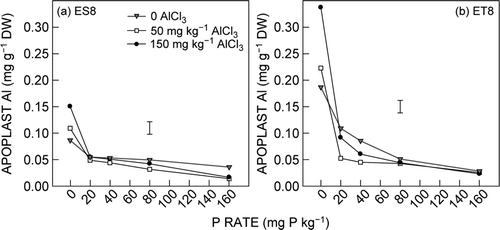
Table 5. Level of significance for the main and interactive effect of Al, P and genotypes on apoplast Al, root Al concentration, shoot Al concentration, root Al uptake, shoot Al uptake and total Al uptake. Values are the means of three replicates for all measurements
The concentration of Al in roots decreased with increasing P supply and increased with increasing Al addition. On average, ES8 had higher Al concentrations in roots than ET8 ().
The concentration of Al in shoots did not differ at 0 and 50 mg AlCl3 kg−1 and slightly decreased with increasing P supply. The addition of 150 mg AlCl3 kg−1 markedly increased Al concentrations in shoots, which contributed to the highly significant Al × P interaction (P < 0.001). The Al concentration with 150 mg AlCl3 kg−1 decreased exponentially with increasing P supply (, ).
Root Al uptake declined with increasing P supply and increased when AlCl3 addition increased from 0 to 50 mg kg−1. Root Al uptake did not differ between the50 and 150 mg AlCl3 kg−1 treatments. On average, root Al uptake by ET8 seedlings was higher than that by ES8 seedlings ( and ).
Table 6. Main effects for Al concentration in roots and total Al uptake in roots and shoots of the wheat seedlings
Shoot Al uptake declined with increasing P supply and increased with increased Al addition. There was no difference in shoot Al uptake between ET8 and ES8 seedlings ( and ).
Discussion
Aluminium and phosphorus dynamics in soil
The maximum amount of P that was added to the Podsol soil in this experiment increased to 160 mg P kg−1 from the 80 mg P kg−1 used in a previous study (Iqbal et al. Citation2010). This doubling of the maximum P amount continued to reduce the extractable Al in the bulk soil (a). The reduction in Al appeared to be more marked with the 150 mg AlCl3 kg−1 treatment. Nevertheless, the continuing reduction in extractable Al with the additional soluble P in the soil further confirms that soluble P acts as an effective detoxifying agent for plant-available Al in soil. The bulk soil pH decreased in line with the increasing levels of added Al, and decreased further at each Al level when soluble P was added (b). This is consistent with the results of the previous study (Iqbal et al. Citation2010). In addition, the pH in the rhizosphere for the different P and Al treatmentcombinations was generally similar to the pH levels in the bulk soil (a and c). This result suggests that the detoxification of Al by added soluble P and the associated reduction in soil pH was not unduly affected by, or driven by, pH changes in the rhizosphere. This evidence suggests that the Al detoxification, resulting from the precipitation of plant-available Al with the soluble P, was a general chemical reaction that occurred throughout the soil mass.
Genotypic variations in seedling growth responses
The highly significant G × Al × P interaction for shoot growth (P ≤ 0.001) in this study indicates that the shoot growth responses of ES8 and ET8 seedlings were dependent on the level of both added Al and added P. The different relative shoot responses between the ES8 and ET8 seedlings were evident in the 150 mg AlCl3 kg−1 treatments. With low rates of added P (0 and 20 mg P kg−1), there were no differences in ES8 and ET8 seedling growth for any measurement (c and d), with seedlings from both genotypes suppressed by the high Al levels. With 40 mg P kg−1, ET8 seedlings showed significantly higher growth (P < 0.05) than ES8 seedlings for all growth measurements, including root length, shoot height and shoot biomass measures. With 80 mg P kg−1, there were no significant differences between ES8 and ET8 seedlings for any growth measurement. Finally, with 160 mg P kg−1, the ES8 seedlings produced greater shoot biomass than ET8 seedlings and similar root lengths (a–d). These comparisons can be continued for the 50 mg AlCl3 kg−1 treatment, in which the ES8 seedlings produced less root growth than the ET8 seedlings with 0 and 20 mg P kg−1 With higher P application rates the ES8 seedlings were either equal or superior to the ET8 seedlings in their growth responses (e). These changes in the relative growth of seedlings of the two isogenic wheat genotypes can be attributed to the changing levels of available Al in the soil, the differences in growth potential of the wheat genotypes with low levels of soil Al, and different levels of added KH2PO4 solution.
One possible reason why ES8 seedlings can produce more shoot biomass than the ET8 seedlings at low Al toxicity levels is that the ET8 seedlings continue to secret malate from their root apices at low Al levels. Delhaize et al. (Citation1993b) reported that ET8 seedlings secreted malate continuously with as little as 0.3 µM Al in a study in which six-day-old ES8 and ET8 seedlings were grown in solutions containing different concentrations of Al. The authors reported that the amount of malate secreted over 24 h by ET8 seedlings was 0.1 µmol malate seedling−1 greater than that by ES8 seedlings at an Al concentration equivalent to a CaCl2-extractable Al concentration of 1.35 ppm. The continuous loss of malate from ET8, with an associated loss of energy and carbon, may be sufficient to reduce shoot growth caused by carbon and energy losses. In this experiment, extractable Al was 0.4 mg kg−1 at combined 160 mg P kg−1 and 150 mg AlCl3 kg−1 treatment. This lower amount of soil extractable Al may still provide the stimulus for malate secretion. It is interesting to note in another experiment reported by Weinger et al. (Citation1992), the Al-tolerant wheat cultivar Carazinho released 566 µg malate g−1 from the root without exposure to Al when grown in sand culture. Carazinho and ET8 are both Al tolerant and rely on malate secretion from their root tips to acquire Al tolerance. Thus it is possible that the growth advantage of ES8 over ET8 with low levels of Al (owing to the presence of high rates of P) could be because of the additional malate secretion by ET8.
Genotypic variation in apoplast aluminium
Significantly higher apoplast Al concentrations were present in the seedling roots of ET8 compared with ES8 in the most toxic Al treatments, i.e. with nil P for all Al treatments, and the 20 mg P kg−1 and 150 mg AlCl3 kg−1 treatments (a and b). These results explain the highly significant (P < 0.001) G × Al × P interaction (). Given the improved Al tolerance of ET8 over ES8 in the 50 mg AlCl3 kg−1 with 0 P or 20 mg P kg−1 treatment combinations, with respect to root length it is tempting to suggest that the increased apoplast Al concentration in ET8 for these treatments is related to the improved Al tolerance of the ET8 seedlings. This proposition is supported by the results of Zhang and Taylor (Citation1989). These authors conducted a solution culture study and desorbed loosely bound Al from excised roots of Al-tolerant (Atlas-66) and Al-sensitive (Neepawa) wheat cultivars by application of 0.5 mM citric acid at 0 °C. After exposure to 180 mM for 180 min, 10% more Al was desorbed from excised roots of Atlas-66 than from those of Neepawa. The authors suggested that the possible involvement of an active exclusion mechanism, achieved by means of an active efflux of Al into the apoplast in Atlas-66, may be the reason for greater accumulation of Al in its root apoplast than that of Neepawa. They also speculated that differences in desorbable Al concentrations between Al-tolerant and -sensitive cultivars may help to identify possible exclusion mechanisms. These differences may occur because of loosely or tightly bound Al capacity in the apoplast that surrounds the plasma membrane. This interpretation is consistent with the suggestion by Wagatusma and Ezoe (Citation1985) that an Al-tolerant plant is able to exclude Al fromcontact with the plasma membrane by promoting polymerization and accumulation of hydroxyl Al in the apoplast, thus contributing to detoxification of Al. This increased accumulation of Al in the apoplast in an Al-tolerant plant may help to identify the Al-tolerance mechanism.
The findings demonstrated that ET8 seedlings were superior in binding Al3+ in the root apoplast compared with ES8 seedlings specific to high Al treatment. This binding of Al in the apoplast assists in the exclusion of Al3+into roots tissue and the close exposure of Al3+ toxicity in roots to the plasma membrane of root apical cells. As a result, ET8 seedlings contained a lower root Al concentration than ES8 seedlings irrespective of Al supply in the 0 P and 150 mg AlCl3 kg−1 treatment (c and d). Thus, it seems that the higher Al3+ binding capacity in the root apoplast of ET8 seedlings contributes to its improved tolerance to Al toxicity compared with that of ES8.
Genotypic variation in aluminium and phosphorus concentrations and uptake in wheat seedlings
The differential tolerance of moderate Al toxicity between ET8 and ES8 was not related to the concentration or uptake of Al by the seedling shoots. First, there were no significant differences between genotypes in shoot Al concentration for all treatments, particularly the treatment 40 mg P kg−1 plus 150 mg AlCl3 kg−1, in which the moderate Al toxicity resulted in differences in shoot and root growth between ET8 and ES8. A second reason was that there were marked differences in shoot Al concentration (for both species) between treatments in which ET8 seedlings displayed greater tolerance to Al toxicity than ES8 seedlings. These were the 0 P, 20 mg P kg−1 plus 50 mg AlCl3 kg−1, and 40 mg P kg−1 plus 150 mg AlCl3 kg−1 treatments. In these sets of treatments, growth of ES8 was suppressed by the Al toxicity to a greater degree than ET8, but the shoot Al concentrations in these treatments were about half that of the 0 P treatment (). Shoot Al uptake also showed little direct relationship with the differential Al tolerance of the two wheat genotypes. An example of this is the marked difference in Al uptake by ES8 and ET8 shoots in the 0 P and 20 mg P kg−1 plus 150 mg AlCl3 kg−1 treatment combinations (), but root and shoot growth of both genotypes were equally suppressed by the severe Al toxicity in these treatments.
Figure 5. Effect of Al and P on shoot Al concentration. Data are the average value of ES8 and ET8. The vertical bar represents the LSD (P = 0.05) for Al × P interaction
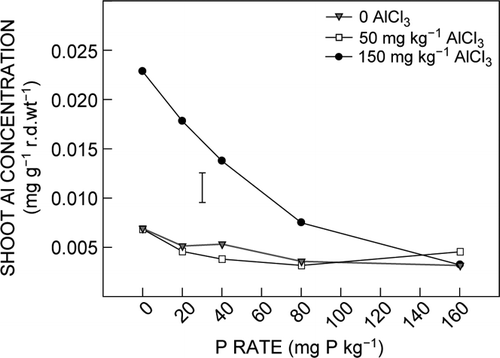
Similarly, the differential tolerance between the wheat genotypes was not related to Al concentration in the roots. Very few interactions occurred between the genotype and either P or Al addition (). The significant main effect for genotype () and the absence of interactions indicates that ES8 seedlings contained higher root Al concentrations than ET8 seedlings across all P and Al treatments. Certainly, there was no indication of markedly elevated Al concentration in the roots of ES8 for those P and Al treatment combinations producing moderate Al toxicity that enabled ET8 seedlings to produce larger shoots and roots than ES8 seedlings.
The P data do not provide any additional insights into the differential tolerance of Al toxicity between ES8 and ET8 because there were minimal differences between ES8 and ET8 seedlings with respect to their P concentration and P uptake in this study. In addition, there were no major differences in the response of the genotypes when P supply was increased to alleviate Al toxicity. Both P concentration and uptake measurements were affected by P and Al treatments resulting in highly significant P × Al interactions ().
Conclusions
The current study demonstrated that doubling the amount of added P further reduces the concentration of extractable Al in soil. These findings indicated that added soluble P is an agent for alleviating Al toxicity in soil. However, added P was more efficient for increasing shoot development but less efficient for increasing root growth. The G × P interaction showed that ES8 seedlings were more responsive than ET8 seedlings when high P was applied to the soil. ET8 seedlings might expend more energy to produce continuous malate secretion even when lower levels of Al exist in the soil solution. Thus, ES8 seedlings are able to use carbon to increase their shoot development with higher levels of P supply. ET8 seedlings also accumulated higher quantities of Al in the apoplast than ES8 seedlings owing to their increased ability to bind desorbable Al with BaCl2 in the apoplast. The findings showed that the Al tolerance of both ET8 and ES8 seedlings increased with increasing P supply. Further investigations are required to determine how P is involved in Al-tolerance mechanisms.
Acknowledgements
The author thanks the Australian government and La Trobe University for providing an International Postgraduate Research Scholarship and La Trobe University Postgraduate Research Scholarship for this study. Dr Gary Clark, Department of Agricultural Sciences, La Trobe University, Australia, is thanked for checking the manuscript before final submission.
References
- Ahn , SJ and Matsumoto , H. 2006 . The role of the plasma membrane in the response of plant roots to aluminum toxicity . Plant Signalling and Behaviour , 1 : 37 – 45 .
- Arsenault , JL , Poulcur , S , Messier , C and Guay , R. 1995 . WinRHlZO™ A root-measuring system with a unique overlap correction method . Horticulture Science , 30 : 906
- Bassett , JRC , Denney , GH , Jeffery , MK and Mendham , GHJ. 1978 . Vogel's textbook of quantitative inorganic analysis including instrumental analysis , 4th edn , London : Longman .
- Delhaize , E , Craig , S , Beaton , CD , Bennet , RJ , Jagadish , VC and Randall , PJ. 1993a . Aluminum tolerance in wheat (Triticum aestivum L) I. Uptake and distribution of aluminum in root apices . Plant Physiology , 103 : 685 – 693 .
- Delhaize , E , Ryan , PR and Randall , PJ. 1993b . Aluminum tolerance in wheat (Triticum aestivum L.) II. Aluminum-stimulated excretion of malic acid from root species . Plant Physiology , 103 : 695 – 702 .
- Delhaize , E and Ryan , PR. 1995 . Aluminum toxicity and tolerance in plants . Plant Physiology , 107 : 315 – 321 .
- de Miranda , LN and Rowello , DL. 1989 . Aluminium–phosphate interactions in wheat . New Phytologist , 113 : 7 – 12 .
- de Sousa , CNA . 1998 . Classification of Brazilian wheat cultivars for aluminium toxicity in acid soils . Plant Breeding , 117 : 217 – 221 .
- Dong , D , Peng , X and Yan , X. 2004 . Organic acid exudation induced by phosphorus efficiency and/or aluminium toxicity in two contrasting soybean genotypes . Physiologia Plantarum , 122 : 190 – 199 .
- Du , YM , Tian , J , Liao , H , Bai , CJ , Yan , XL and Liu , GD. 2009 . Aluminium tolerance and high phosphorus efficiency helps Stylosanthes better adapt to low-P acid soils . Annals of Botany , 103 : 1239 – 1247 .
- Fisher , JA and Scott , BJ. 1987 . “ Response to selection for aluminium tolerance ” . In Priorities in soil/plant relations: research for plant production , Edited by: Searle , PGE and Davey , BG . 135 – 137 . Sydney : University of Sydney .
- Foy , CD. 1988 . Plant adaptation to acid, aluminum-toxic soils . Communications in Soil Science and Plant Analysis , 19 : 959 – 987 .
- Fukuda , T , Saito , A , Wasaki , J , Shinano , T and Osaki , M. 2007 . Metabolic alterations proposed by proteome in rice roots grown under low P and high Al concentration under low pH . Plant Science , 172 : 1157 – 1165 .
- Iqbal , MT. 2012a . Acid tolerance mechanisms in soil grown plant . Malaysian Journal of Soil Science , 16 : 1 – 19 .
- Iqbal , MT. 2012b . Effect of Al compound on soil pH and bioavailability of Al in two acid soils . Turkish Journal of Agriculture and Forestry , 36 : 720 – 728 .
- Iqbal T , Sale P , Tang C. 2010 . Phosphorus ameliorates aluminium toxicity of Al-sensitive wheat seedlings . In: Gilkes RJ , Prakongkep N (eds), Proceedings of the 19th World Congress of Soil Science: Soil Solutions for a Changing World , 1–6 August 2010 , International Union of Soil Sciences , Brisbane , , Australia . pp 92 – 95 (published on DVD) .
- Isbell , RF. 2002 . The Australian soil classification , Collingwood : CSIRO .
- Kariuki , SK , Zhang , H , Schroder , JI , Edwards , J , Payton , M , Carver , BF , Raun , WR and Krenzer , EG. 2007 . Hard red winter wheat cultivar responses to a pH and aluminum concentration gradient . Agronomy Journal , 99 : 88 – 98 .
- Kerven , GL , Edwards , DG , Asher , CJ , Hallman , PS and Kokot , S. 1989 . Aluminium determination in soil solution. II. Short-term colorimetric procedures for the measurement of inorganic monomeric aluminium in the presence of organic acid ligands . Australian Journal of Soil Research , 27 : 91 – 102 .
- Kochian , LV , Hoekenga , OA and Pineros , MA. 2004 . How do crop plants tolerate acid soils? Mechanisms of aluminum tolerance and phosphorous efficiency . Annual Review of Plant Biology , 55 : 459 – 493 .
- Liao , H , Wan , H , Shaff , J , Wang , X , Yan , X and Kochian , LV. 2006 . Phosphorous and aluminum interactions in soybean in relation to aluminium tolerance. Exudation of specific organic acids from different regions of the intact root system . Plant Physiology , 141 : 674 – 684 .
- Mendoza , J and Borie , F. 1998 . Effect of Glomus etunicatum inoculation on aluminum, phosphorus, calcium and magnesium uptake of two barley genotypes with different aluminum tolerance . Communications in Soil Science and Plant Analysis , 29 : 681 – 695 .
- Nakagawa , T , Mori , S and Yoshimura , E. 2003 . Amelioration of aluminum toxicity by pretreatment with phosphate in aluminumtolerant rice cultivar . Journal of Plant Nutrition , 26 : 619 – 628 .
- Nian , H , Ahn , SJ , Yang , ZM and Matsumoto , H. 2003 . Effect of phosphorus deficiency on aluminium-induced citrate exudation in soybean (Glycine max) . Plant Physiology , 117 : 229 – 236 .
- Sanzonowicz , C , Smyth , TJ and Israel , DW. 1998 . Calcium alleviation of hydrogen and aluminum inhibition of soybean root extension from limed soil into acid subsurface solutions . Journal of Plant Nutrition , 21 : 785 – 804 .
- Silva , IR , Smyth , TJ , Israel , DW and Rufty , TW. 2001 . Altered aluminum inhibition of soybean root elongation in the presence of magnesium . Plant and Soil , 230 : 223 – 230 .
- Tan , K and Keltjens , WG. 1990 . Interaction between aluminium and phosphorus in sorghum plants I. Studies with the aluminium sensitive sorghum genotype TAM428 . Plant and Soil , 124 : 15 – 23 .
- Wagatsuma , T and Ezoe , Y. 1985 . Effect of pH on ionic species of aluminium in medium and on aluminium toxicity under solution culture . Soil Science and Plant Nutrition , 31 : 547 – 561 .
- Weligama , C , Tang , C , Sale , PWG , Conyers , MK and Liu , D. 2010 . Application of nitrogen in form increases rhizosphere alkalisation in the subsurface soil layers in an acid soil . Plant and Soil , 333 : 403 – 416 .
- Weniger , CC , Groneman , AF and Veen , JAV. 1992 . Associative N2 fixation and root exudation of organic acids from wheat cultivars of different aluminium tolerance . Plant and Soil , 139 : 167 – 174 .
- Yan , X , Lynch , JP and Beebe , SE. 1995 . Genetic variation for phosphorus efficiency of common bean in contrasting soil types: I. Vegetative response . Crop Science , 35 : 1086 – 1093 .
- Zhang , G and Taylor , GT. 1989 . Kinetics of aluminum uptake by excised roots of aluminum-tolerant and aluminum-sensitive cultivars of Triticum aestivum L . Plant Physiology , 91 : 1094 – 1099 .