Abstract
Evaluation of genetic distances between maize inbred lines can be used to maximise heterosis in hybrids. This study was conducted to determine genetic distances among 25 maize inbred lines in the Maize streak virus disease (MSVD) QTL region to develop MSVD-resistant hybrids. The inbred lines were genotyped using 19 simple sequence repeat (SSR) markers that are known to be associated with MSVD resistance in maize. All amplification products were in the range of 68–290 bp. In total, 94 SSR alleles were detected, with a mean of 4.95 alleles per locus. The average polymorphic information content value was 0.56. Gene diversity (He) values ranged from 0.00 to 0.853 with an average of 0.594, whereas heterozygosity (Ho) values attained an average of 0.039, ranging from 0.00 to 0.095. There were three major clusters and five subclusters, from which potential breeding lines could be sampled. These results confirmed the diversity found among the maize lines within the MSVD QTL region used in this study. In general, the grouping of the inbred lines by SSR markers is consistent with pedigree information. The data from SSR markers was useful in identifying lines that could be used to design new hybrids and new breeding populations for MSVD resistance.
Introduction
Maize is the chief support of most of Africa's rural economies (Oluwafemi et al. Citation2008; Stevens Citation2008), including Mozambique, in which it is the staple food and is grown in all of its agro-ecological zones (Denic et al. Citation2002). Despite this, the average yield of maize in Africa is the lowest in the world and, as a result, fails to meet the high demands in most sub-Saharan countries (Denic et al. Citation2002; Magenya et al. Citation2008). Numerous viral pathogens affect maize productivity, with Maize streak virus disease (MSVD) being considered as the most significant biological threat to food security in Africa (Bosque-Perez Citation2000; Shepherd et al. Citation2007; Martin and Shepherd Citation2009). This is a major concern and can only be addressed by solving production constraints to improve crop yields.
Virus resistance is usually associated with one or two major resistance loci, which facilitate marker-assisted selection, but resistance genes have been found to cluster in the maize genome (Redinbaugh et al. Citation2004). Genomic regions associated with resistance to MSVD have been identified in several studies using different populations in diverse environments and these studies have revealed that resistance is quantitatively inherited with a varying number of genes involved (Pernet et al. Citation1999; Welz et al. Citation1998; Mawere et al. Citation2006). Mawere et al. (Citation2006) reported that MSVD resistance is expressed by a major gene and two or three modifying genes. Pernet et al. (Citation1999) also proposed that MSVD resistance was controlled by two genetic systems, one from a major gene on the short arm of chromosome 1 and the other conditioned by minor genes on chromosomes 2, 3 and 10, that confer quantitative resistance. Minor quantitative trait loci (QTL) effects have been detected at bins 3.06, 5.03 and 8.07 (Asea et al. Citation2008). The major QTL, designated msv1, was identified on the short arm of chromosome 1 (1S – bin1.04) (Welz et al. Citation1998; Kyetere et al. Citation1999; Mawere et al. Citation2006). The stability of QTL across populations has been shown to be variable; however, this is not the case for MSVD QTL (Pernet et al. Citation1999).
A study conducted by Danson et al. (Citation2006) used three DNA markers: bnlg1811, umc1917 and umc1144, which are contained between bin 1.04 and 1.05 of maize chromosome 1 to screen 115 recombinant inbred lines for resistance to MSVD. These markers were able to differentiate resistant from susceptible lines. A study by Asea et al. (Citation2008) further examined a consensus MSVD QTL in bin 1.04 as a potential target for selection in improving host resistance. Maize streak field evaluations and subsequent selections were conducted in Zimbabwe in a population of 410 F2:3 lines derived from hybridisation between inbred line CML202 with known resistance to MSVD and the susceptible line VP31. It was concluded that the major locus conferring resistance to MSVD on chromosome 1 was significant (P < 0.05) for resistance across seasons and explained 23% of phenotypic variations in the F2:3 generation. Markers used for this current study were developed by Danson et al. (Citation2006), Asea et al. (Citation2008), the MaizeGDB database (http://www.maizegdb.org) and from a study by Lagat et al. (Citation2008) in which the QTL for resistance to MSVD in one resistant source, MAL13, crossed to one elite line, MAL9, were mapped using SSRs. Conventional maize breeders may benefit from the use of molecular markers in order to improve selection intensity and maximise genetic gain (Collard et al., Citation2005).
The adoption of hybrids in maize production has resulted in increased yields across the world (Warburton et al. Citation2002). Maize breeding relies on the available genetic diversity (Karanja et al. Citation2009). Improved hybrids of maize are developed by making use of information on the genetic relationships and diversity among elite materials (Dias et al. Citation2003; Diniz et al. Citation2005). Evaluating genetic diversity among the elite lines aids in the estimation of genetic variation and thus the degree of heterosis to be expected among segregating progeny for pure-line cultivar development (Biswas et al. Citation2008; Salem et al. Citation2008; Karanja et al. Citation2009).
Heterosis, also known as hybrid vigour, is a phenomenon in which the offspring show superiority over their parents either in yield, vigour, increased size, rate of growth or other reproductive factors (Duvick Citation1999; Virmani et al. Citation2003). The term coined by Shull (Citation1952) can be used for the expression of adaptive traits such as increased resistance to disease and drought tolerance, with the hybrid of choice exceeding the best parent in superiority. However, superiority is lost with every successive generation of self-fertilisation, thus maximum heterosis is expressed in the F1 generation (Meyer et al. Citation2004). The manifestation of heterosis depends on genetic divergence of the two parental varieties (Hallauer and Miranda Citation1988). Morphological, pedigree, physiological, biochemical and molecular data can be used to identify elite inbred lines to be crossed for a superior hybrid (Smith and Smith Citation1989). However, molecular markers can detect variation at the DNA sequence level (Diniz et al. Citation2005) and genetic distances are used to group similar germplasm as the first step in identifying potentially useful heterotic patterns (Melchinger Citation1999).
Simple sequence repeat (SSR) markers, also known as microsatellites (He et al. Citation2003; Molnar et al. Citation2003), have been extensively used to characterise germplasm collections in major cereal crops including wheat (Salem et al. Citation2008; Ijaz and Khan Citation2009) and maize (Taramino and Tingey Citation1996; Smith et al. Citation1997; Li et al. Citation2002; Danson et al. Citation2006; Aguiar et al. Citation2008; Cholastova et al. Citation2011). Different repeat numbers in SSRs can be treated as separate ‘alleles’ and the site can be treated as highly polymorphic with multiple alleles for the detection of variation in populations (Akkaya et al. Citation1992). Microsatellites are highly abundant, simple to analyse, co-dominant, economical and are easily assayed using the polymerase chain reaction (PCR) with primers specific to conserved regions flanking the repeat array (Yu et al. Citation2000). Compared with other marker types, SSRs are advantageous due to their abundance in plant genomes and large number of alleles per locus, making them highly polymorphic even among closely related cultivars due to naturally occurring mutations, and thus they can distinguish between closely related species (Brown et al. Citation1996; Weising et al. Citation2005), providing greater power of discrimination. Hence, they are useful for assigning heterotic groups for maize lines (Enoki et al. Citation2002; Li et al. Citation2002; Xu et al. Citation2004).
Simple sequence repeat technology is a reliable and effective means of assessing genetic diversity and is a powerful tool for grouping germplasm (Reif et al. Citation2003). In maize the prediction of hybrid performance has been found to be effective using SSR markers (Drinic et al. Citation2002). Hybrid yield was significantly correlated with genetic distance based on SSR markers in maize (Reif et al. Citation2003; Xu et al. Citation2004). It was also found that SSR marker-based genetic distance is highly significantly correlated with grain yield (positive) and days to anthesis and silking (negatively), therefore suggesting that high grain yield and earliness of hybrids can be predicted from SSR marker determined distances of the parental lines (Wegary et al. Citation2013).
The objectives of the study were, therefore, to determine the genetic diversity among the 25 maize inbred lines using 19 SSR markers that are known to be associated with MSVD resistance in maize. The information will be used in the selection of the most appropriate parents out of the potential MSVD resistance donors for the introgression of the MSVD resistant gene msv1 into the different Mozambican lines that are adapted to the lowland environment but are susceptible to MSVD. The information will be crucial in devising future hybrid breeding programmes that will emphasise MSVD resistance in Mozambique.
Materials and methods
Plant material
A total of 25 maize inbred lines were used in this study. All maize varieties, along with their pedigrees, MSVD and downey mildew (DM) disease ratings are listed in . Some of the maize inbred lines were from CIMMYT, Zimbabwe (CML505, CML509, P13, P14, P15, P16, P17 and P18) and PA1 from the Crop Breeding Institute (CBI) in Zimbabwe. The CIMMYT lines are potential donors for MSVD resistance (CIMMYT Citation2009). Line PA1 has high yield potential but is susceptible to MSVD and adapted to mid-altitude conditions, hence was included as a standard check. Although the P lines (P13, P14, P15, P16, P17 and P18) are MSVD resistant, they are susceptible to DM disease and not adapted to the tropical conditions of the lowlands of coastal Mozambique. Lines CML505 and CML509 have baseline resistance to DM and are adapted to the tropical lowland conditions. The LP group of lines and E designated inbred lines developed by the maize research programme in Mozambique are susceptible to MSVD (Denic Citation2005; Fato Citation2010). These lines were therefore suitable recipients of the MSVD resistance gene.
Table 1: Features of the 25 maize inbred lines used in the study. DM = downy mildew, MSV = maize streak virus
DNA extraction
The 25 maize inbred lines were grown in a tunnel at the University of KwaZulu-Natal, Pietermaritzburg, South Africa, during 2009. At four weeks after planting, leaf tissue samples (five plants per line) were harvested and total DNA was extracted from each of the genotypes using a cetyltrimethylammonium bromide (CTAB) method. The tissue was macerated in 1.5 ml centrifuge tubes with three 5 mm stainless steel beads and shaken in a TissueLyser bead beater for 2 min. Immediately, 500 µl of pre-warmed (65 °C) (CTAB) extraction buffer (100 mM Tris-HCl [pH 8.0], 700 mM sodium chloride [NaCl], 10 mM ethylene diamine tetraacetic acid [EDTA], 2% CTAB, 2% polyvinyl pyrrolidone (PVP-40), 5 mM ascorbic acid and 4 mM sodium diethyldithiocarbamic acid] and vortexed for a few seconds. The samples were incubated at 65 °C for 60 min followed by cooling on ice for 15 min. Subsequently, 200 µl chloroform/ isoamyl alcohol (24:1) was added under the fume hood and phases were separated by centrifugation for 10 min at 12 000 rpm at room temperature. Then 200 µl of the supernatant was transferred into new sterile 1.5 ml centrifuge tubes, which contained 200 µl cold isopropanol. The precipitated DNA was then collected by centrifugation for 15 min with 12 000 rpm. The supernatant was drained off immediately and then pellets washed with 100 µl of 70% cold ethanol. The pellet was dissolved in 100 µl TE buffer (10 mM Tris and 1 mM EDTA, pH 8.0) and stored at -20°C.
Simple sequence repeats primer selection
Primers targeting the MSVD QTL region were selected as the objective of this study was to develop MSVD resistance hybrids. Therefore the parental lines were screened with primers from the MSVD QTL region to determine the amount of genetic diversity present at this specific locus to select the lines most divergent at the MSVD QTL region to hopefully obtain the highest heterozygosity in the F1 generation.
Nineteen SSR gene-specific markers for msv1, which confers resistance to MSVD, were used to genotype the lines (). The primers that were identified in previous studies (Danson et al. Citation2006; Asea et al. Citation2008; Lagat et al. Citation2008) and from the MaizeGDB database (http://www.maizegbd.org) were chosen based on bin locations to detect genetic diversity at that region of the genome. Markers linked to the msv1 gene have mostly been identified on bin 1.04, thus more primers on this bin were selected.
Table 2: Primer sequences of the 19 SSR markers used in this study
PCR amplification and detection conditions
The PCR reactions were carried out in a final volume of 12 µl, containing 1× PCR buffer, 3 mM MgCl2, 2.5 mM dNTPs, 10 mM forward and reverse primers, 0.6 U Taq DNA polymerase and approximately 40 ng DNA template with the final volume made up with double-distilled sterile water. The PCR reaction was carried out in a touchdown programme with a first denaturation at 94 °C for 2 min, followed by eight cycles of (1) denaturation at 94 °C for 30 s, (2) annealing at 60 °C for 30 s and (3) extension at 72 °C for 45 s, with the annealing temperature being reduced by 1 °C per cycle. This procedure was followed by 25 cycles of (1) denaturation at 94 °C for 30 s, (2) annealing at 52 °C for 30 s and (3) extension at 72 °C for 45 s, and a final extension at 72 °C for 5 min. The target sequences were amplified by using forward primers fluorescently labelled with either VIC (green), FAM (blue), PET (red) or NED (yellow). The PCR products were denatured by heating at 95 °C for 5 min and separated by capillary gel electrophoresis using an ABI3130 automatic DNA sequencer (Applied Biosystems, Johannesburg, South Africa). The allele sizes of amplified PCR fragments were identified on the basis of size in comparison with DNA molecular weight markers. The size of the amplified fragments was determined by the software programme GeneMapper 4.1 (Applied Biosystems, Johannesburg, South Africa).
Data analysis
PowerMarker 3.25 (Liu and Muse Citation2005) was used to determine polymorphic information content (PIC), gene diversity and heterozygosity values for each SSR marker used in the study. The expected heterozygosity (He) and observed heterozygosity (Ho) were used to evaluate the genetic diversity within the set of cultivars. Expected heterozygosity, i.e. the probability that two alleles from the same locus would be different when chosen at random, was calculated for each SSR locus according to Nei (Citation1973):
where pi is the frequency of the ith allele in a locus for individual p.
Observed heterozygosity was calculated by dividing the number of heterozygous individuals by the number of individuals scored. Polymorphic information content for the SSR markers in the sample DNA was calculated as:
For co-dominant markers such as SSRs and RFLPs, data can be scored as allele frequencies and as binary traits (1 = allele presence, 0 = allele absence) (Warburton and Crossa Citation2002). The allele frequency data from PowerMarker 3.25 was used to export the data in binary format for analysis with NTSYS-PC 2.1 software (Exeter Software, Setauket, NY, USA) (Dice Citation1945). NTSYS-PC 2.1 only accepts binary data coding. The 0/1 matrix was used to calculate genetic similarity based on the Dice coefficient (Dice Citation1945). A dendrogram was constructed using the unweighted paired group method using arithmetic averages (UPGMA) method as implemented in NTSYS-PC 2.1 to infer genetic relationships and phylogeny. The MXComp function was used to determine the R value for the dendrogram (Rohlf Citation1998).
Results
Genetic diversity
A total of 94 alleles were amplified among 25 maize inbred lines and the numbers of alleles scored for SSR loci ranged from one to nine. The average number of alleles was 4.95. The maximum number of alleles (nine) was detected at the bnlg105 locus. The PIC value of the SSR markers, which is a measure of allele diversity at a locus, ranged from 0.000 to 0.838 with an average of 0.556 (). Nine SSR loci (bnlg490, bnlg105, umc1122, umc1811, umc1086, umc1551, umc2169, phi330507 and umc1221) exhibited PIC values higher than 0.6, indicating that they were potentially informative in detecting differences among the inbred lines.
Table 3: Details of polymorphisms and genetic analysis of 19 maize microsatellite markers across the 25 maize inbred lines
Di- and tri-nucleotide repeat markers were the most abundant. The He values were in the range of 0.00 (phi227562, umc1917) to 0.853 (bnlg105) with a mean gene diversity value of 0.594. The Ho values ranged from 0.00 to 0.318 with an average of 0.0387. The most polymorphic SSRs based on PIC value (≥0.75), genetic diversity (≥0.75) and alleles (≥6) were bnlg105, umc1551 and umc1086.
Analysis of maize genotype associations
The estimates of similarity coefficients among the 25 inbred lines ranged from 15% to 94%. The highest similarity index of 0.94 was obtained between P14 and P13, followed by E77 and E75 (0.89) and E47 and E46 (0.86). The lowest similarity value of 0.15 was between E75 and LP21 and also between E77 and LP21 (). The results on similarity were also substantiated by the dendrogram ().
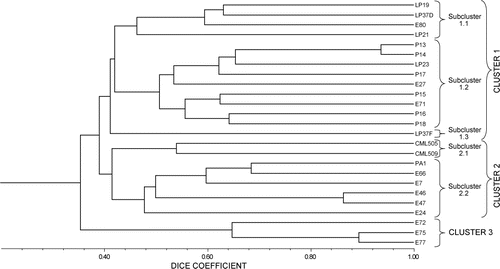
Table 4: Similarity matrix for the 25 maize inbred lines based on 19 SSR markers
The majority of bifurcations in the dendrogram occurred at genetic distances above 0.30. All the 25 maize genotypes were grouped into three major clusters: 1, 2 and 3 at a 40% similarity. Major Cluster 1 (MC1) was further divided into three subclusters at a 44% similarity coefficient. The subclusters derived from MC1 were as follows: Subcluster 1.1 consisted of Mozambican inbred lines LP19, LP37D, LP21 and E80; Subcluster 1.2 grouped CIMMYT lines P13, P14, P17, P15, P16 and P18 and some Mozambican lines LP23, E27 and E71; and Subcluster 1.3 comprised the Mozambican line LP37F. Major Cluster 2 (MC2) was divided into two subclusters at a 48% similarity with the CIMMYT lines CML505 and CML509 being grouped together in Subcluster 2.1 at a 54% similarity. The LP23 line was in a different cluster from lines CML505 and CML509, which reflects the wide genetic distances among the lines. The similarity value between LP23 and CML509 was 0.28 and 0.41 between LP23 with CML505.
Lines P13 and P14 of MC1, Subcluster 1.2 were the most related with a 94% similarity value. The lowest similarity value of 0.15 was between the lines E75 and LP21 and between E77 and LP21 followed by E27 and E24, E77 and LP37F and also between E72 and LP21 (0.21).
Most of the Mozambican E lines (E66, E7, E46, E47 and E24) were grouped into Subcluster 2.2 of MC2 with the CBI line PA1 at the 46% similarity. Major Cluster 3 (MC3) consisted of the lines E72, E75 and E77 at 64% similarity. All the CIMMYT inbred P lines and the Mozambican inbred LP lines clustered in MC1. The R value for the dendrogram was 0.85.
Discussion
Analysis of genetic diversity using simple sequence repeats
Genetic diversity within a population can be expressed as the number of alleles detected at a single locus (Hoxha et al. Citation2004). The average number of alleles obtained per primer in the present study was 4.95, which is comparable to the 5.2 alleles that were reported for 50 SSR markers in 85 tropical maize lines (Laborda et al. Citation2005). However, the average number of alleles observed is smaller than the 7.4 alleles that were reported for 79 SSR markers in 15 lines (Xu et al. Citation2004). The total number of alleles in diversity studies is usually proportional to the sample size (Xu et al. Citation2004), which can partly explain the observed differences between the studies. A study by Liu et al. (Citation2003) on 260 inbred lines across tropical, subtropical and temperate maize inbred lines characterised with 94 SSRs detected a total of 2 039 alleles with a mean of 21.7 alleles per locus. Higher genetic diversity in that study can be attributed to the more diverse and larger number of inbreds (Vigouroux et al. Citation2005). Sharopova et al. (Citation2002) developed 1 051 maize SSR primers and concluded that polymorphism increased significantly with the increase in the number of repeat units. The inclusion of more dinucleotide repeat SSRs, which tend to be more polymorphic than longer repeat motifs, was also found to increase polymorphism levels (Masi et al. Citation2003, Vigouroux et al. Citation2005).
Three of the primers used (bnlg105, umc1551 and umc1086) exhibited high polymorphism. These can be effectively used in future molecular breeding programmes for the identification of highly diverse maize lines in hybrid development focusing on MSVD resistance. The parameters used for the determination of polymorphic SSR loci were also previously used by Babu et al. (Citation2012) and Yao et al. (Citation2007) to screen inbred lines in India and China, respectively.
The majority of bifurcations in the dendrogram occurred at genetic distances above 0.30, which was also observed in a study by Weising et al. (Citation2005) on indigenous Swedish mandate cultivars. This indicates that the 25 lines tested in the present study were not closely related to one another. Xia et al. (Citation2005) used SSR markers to assess genetic diversity in CIMMYT maize lines and the study did not reveal clear clustering amongst the lines assessed. However, in the current study, the genetic similarity index using SSR markers identified three major clusters: MC1, MC2 and MC3. All the CIMMYT P lines were grouped into MC1, which is consistent with pedigree information. These lines were developed from a common genetic background for MSVD resistance. The CIMMYT maize lines, CML505 and CML509, did not cluster in MC1 with the rest of the CML lines, which is also in agreement with pedigree information. These observations are supported by the study by Yuan et al. (Citation2002) in which all of the CIMMYT lines clustered together according to pedigree. According to Warburton et al. (Citation2002), CIMMYT inbred lines are usually drawn from a mixture of populations that contain a broad genetic base. The CML505 and CML509 lines were derived from the same population with adaptation to the tropical lowland and resistance to downy mildew disease. In agreement with pedigree information, lines E46 and E47 were placed in the same cluster 2, because both lines were derived from ZM521. However, it was observed that the E group of lines, which were derived from the same population ‘ZM621’ (except E7), were allocated to different clusters with three lines in MC3, two in MC2 and three in MC1, which is not congruent with pedigree information. This can be explained by the fact that the base population ZM621 is broad based. It was developed as a synthetic hybrid population between heterotic group A and group B lines involving more than eight lines, and has gone through several cycles of recombination at CIMMYT in Harare.
The clustering of the Mozambican inbred LP lines also partly reflects the pedigree information and origin of the lines. The lines LP37D and LP37F are sister lines derived from the same base population Pop44 from CIMMYT, but they are placed in different subclusters since LP37D has dent grain texture whereas LP37F has flint grain. Lines LP23 and LP19 were developed under lowland tropical conditions in Mozambique and Nigeria, respectively, and were derived from different populations; hence they were grouped in different subclusters within MC1. The actual pedigree data for the line LP21 could not be established, so the observation that it was classified in the same subcluster as LP19 suggests that they have a similar genetic background or have similar allele frequencies. These lines may be placed in the same heterotic group in the programme in Mozambique. The importance of the origin of the lines is also reflected by the classification of PA1 in Subcluster 2.2 together with lines E46, E47 and E66, among others, which were derived from the mid-altitude populations ZM521 and ZM621 in Zimbabwe. The genetic distance matrices derived from the SSRs were highly correlated (r = 0.85), indicating that the SSRs have distinguishable power to detect polymorphism and are appropriate for genetic diversity analysis among maize inbred lines.
Implications for breeding new hybrids
Studies report that for the production of hybrids with better yield performance, it is best to use lines with larger genetic distances as parents as these increase genetic variation (Yuan et al. Citation2002; Shahnejat-Bushehri et al. Citation2005; Biswas et al. Citation2008). Small genetic distances reveal that lines are closely related and thus should not be crossed with each other if hybrid vigour is to be maximised. Among the 25 inbred lines, E75 and LP21 as well as E77 and LP21 were the most distantly related parental lines with the lowest similarity values of 0.15, indicating that the two lines are divergent and contain different allele frequencies that can be exploited to produce hybrids. The lines E24 and LP19, with a low similarity value of 0.26, would also be recommended for use in producing hybrids.
The lowland-adapted and MSVD-resistant donor lines (CML505 and CML509) had low similarity values, ranging between 27% and 54%, with the set of lines from Mozambique (P and E series), indicating the existence of large genetic distances and, therefore, are divergent at the MSVD QTL region. Hence, it would be expected that productive hybrids could be obtained by making crosses between the CMLs and the Mozambican lines because they have a complimentary gene frequency that might result in high heterosis. The fact that LP21 and LP23 were placed in a different cluster with the MSVD donor lines CML505 and CML509, suggests that productive hybrids can be produced by combining the DM resistant lines with the MSVD resistant lines, respectively. The lines CML505 and CML509 were previously characterised, and displayed MSVD resistance with ratings of 1.5 and 2, respectively (CIMMYT Citation2009), whereas Denic (Citation2005) characterised the LP inbred lines, including LP23 and LP21, and reported that they are highly resistant to DM. Most importantly, the results indicate that LP19 and LP21 are in the same subcluster whereas LP23, which originated in Nigeria, is in a different subcluster, which suggests that a three-way-cross hybrid among these lines (LP19, LP21 and LP23) should be productive as general combining ability is minimised within the cluster and maximised between the clusters. This analysis has been confirmed by the maize breeders in Mozambique from practical experience with these lines.
Implications for developing new inbred lines
In addition, classification of MSVD resistance donor lines in two different clusters MC1 (P13 through to P18) and MC2 (CML505 and CML509) indicates that they can be regarded as different heterotic groups. This has implications for managing the breeding programme in Mozambique. In order to fix inbred lines over shorter periods, it is crucial to develop breeding populations by crossing lines with similar allele frequencies. Lines CML505 and CML509 are the potential donors for MSVD resistance to the Mozambican lines in Cluster 2 (E66, E7, E46, E47 and E24). On the other hand, the MSVD potential donor lines P13, P14, P15, P16, P17 and P18 could be used to improve MSVD resistance in the other Mozambican lines in Cluster 1 (E80, E27, E71, LP19, LP37D, LP37F and LP23) because they are likely to have a similar gene frequency. Unfortunately, there are no MSVD resistance donor lines that were placed in Cluster 3; hence another set of potential donor lines for use to improve resistance of these lines (E72, E75 and E77) to MSVD should be found.
Conclusions
The following conclusions were drawn. There is genetic variation among the 25 inbred lines in the MSVD QTL region as they were assigned to three major clusters and, in general, the genotypic analysis confirmed the pedigree data. This indicates that breeding new MSVD resistant hybrids from this set of germplasm would be viable. Existence of genetic distance between the lines indicates that productive hybrids can be designed such that lines from different groups can be crossed so that they can complement each other in hybrids. The study also identified the potential MSVD resistance donors for use to improve the lines in Mozambique, which also forms the basis for devising a breeding strategy that is appropriate for the breeding programme in Mozambique.
Acknowledgements
This study was funded by a grant from the Generation Challenge Programme (GCP). The authors wish to acknowledge the International Maize and Wheat Improvement Centre (CIMMYT) and Institute of Agriculture of Mozambique (IIAM) for the provision of germplasm for this study.
References
- Aguiar CG, Schuster I, Amaral AT Jr, Scapim CA, Viera ESN. 2008. Heterotic groups in tropical maize germplasm by testcrosses and simple sequence repeat markers. Genetics and Molecular Research 4: 1233–1244.
- Akkaya MS, Bhagwat AA, Cregan PB. 1992. Length polymorphisms of simple sequence repeat DNA in soybean. Genetics 132: 1132–1139.
- Asea G, Vivek BS, Bigirwa G, Lipps PE, Pratt RC. 2008. Validation of consensus quantitative trait loci associated with resistance to multiple foliar pathogens of maize. Phytopathology 99: 540–547.
- Babu BK, Pooja P, Bhatt JC, Agrawal PK. 2012. Characterisation of Indian and exotic quality protein maize (QPM) and normal maize (Zea mays L.) inbreds using simple sequence repeat (SSR) markers. African Journal of Biotechnology 11: 9691–9700.
- Biswas MK, Mondal MAA, Hossain M, Islam R. 2008. Utilization of genetic diversity and its association with heterosis for progeny selection in potato breeding programs. Science 6: 882–887.
- Bosque-Perez NA. 2000. Eight decades of Maize streak virus research. Virus Research 71: 107–121.
- Brown SM, Hopkins MS, Mitchell SE, Senior ML, Wang TY, Duncan RR, Gonzalez-Candelas F, Kresovich S. 1996. Multiple methods for the identification of polymorphic simple sequence repeats (SSRs) in sorghum (Sorghum bicolor L. Moench). Theoretical and Applied Genetics 93: 190–198.
- Cholastova T, Soldanova M, Pokorny R. 2011. Random amplified polymorphic DNA (RAPD) and simple sequence repeat (SSR) marker efficacy for maize hybrid identification. African Journal of Biotechnology 10: 4794–4801.
- CIMMYT. 2009. CIMMYT maize inbred lines (CML). Mexico, DF: CIMMYT. Available at http://apps.cimmyt.org/english/wps/obtain_seed/cml-germplas.htm [accessed 9 August 2011].
- Collard BCY, Jahufer MZZ, Brouwer JP, Pang ECK. 2005. An introduction to markers, quantitative trait loci (QTL) mapping and marker-assisted selection for crop improvement: the basic concepts. Euphytica 142: 169–196.
- Danson J, Lagat M, Ininda J, Kimani M. 2006. Application of simple sequence repeats (SSRs) markers to study the resistance of locally adapted maize hybrids to damaging Maize streak virus disease. African Journal of Biotechnology 5: 1430–1434.
- Denic M. 2005. Maize breeding program: breeding activities and national trials. National Agriculture Research Institute Annual Progress Report. Maputo: Department of Agriculture and Farming Systems.
- Denic MP, Chauque C, Jose M, Langa D, Mariote D, Fato P, Haag WH. 2002. Maize screening for multiple stress tolerance and agronomic traits. In: Friesen DK, Palmer AFE (eds), Proceedings of the 7th Eastern and Southern Africa RegionalMaize Conference, 5–11 February 2002, Nairobi, Kenya. Nairobi: CIMMYT. pp 88–91.
- Dias LAS, Cruz JM, Barros CD, Salamao TMF. 2003. Genetic distance and its association with heterosis in cacao. Brazilian Archives of Biology and Technology 46: 339–347.
- Dice LR. 1945. Measures of the amount of ecologic association between species. Ecology 26: 297–302.
- Diniz LEC, Ruas CF, Carvalho VP, Torres FM, Ruas EA, Santos MO, Sera T, Ruas PM. 2005. Genetic diversity among forty coffee varieties assessed by RAPD markers associated with restriction digestion. Brazilian Archives of Biology and Technology 48: 511–521.
- Drinic SM, Trifunovic S, Drinic G, Konstantinov K. 2002. Genetic divergence and its correlation to heterosis in maize as revealed by SSR-based markers. Maydica 4: 1–8.
- Duvick DN. 1999. Heterosis: feeding people and protecting natural resources. In: Coors JG, Pandey S (eds), The genetics and exploitation of heterosis in crops. Madison: American Society of Agronomy, Crop Science Society of America, and Soil Science Society of America. pp 19–29.
- Enoki H, Sato H, Koinuma K. 2002. SSR analysis of genetic diversity among maize inbred lines adapted to cold regions of Japan. Theoretical and Applied Genetics 104: 1270–1278.
- Fato P 2010. Investigation of heterotic patterns and genetic analysis of Downy mildew resistance in Mozambican lowland maize (Zea mays L.) germplasm. PhD thesis, University of KwaZulu-Natal, Pietermaritzburg, South Africa.
- Hallauer AR, Miranda Filho JB. 1988. Quantitative genetics in maize breeding (2nd edn). Ames: Iowa State University Press.
- He G, Meng R, Newman M, Gao G, Pittman RN, Prakash C. 2003. Microsatellites as DNA markers in cultivated peanut (Arachis hypogaea L.). BMC Plant Biology 3: 3.
- Hoxha S, Shariflou MR, Sharp P. 2004. Evaluation of genetic diversity in Albanian maize using SRR markers. Maydica 49: 97–103.
- Ijaz S, Khan IA. 2009. Molecular characterisation of wheat germplasm using microsatellite markers. Genetics and Molecular Research 8: 809–815.
- Karanja J, Amugune NO, Ininda J, Kimatu JN, Danson JW. 2009. Microsatellite analysis of the correlation between molecular and morphological traits in assorted maize inbred lines. African Crop Science Journal 17: 133–144.
- Kyetere DT, Ming R, McMullen MD, Pratt RC, Brewbaker J, Musket T. 1999. Genetic analysis of tolerance to Maize streak virus in maize. Genome 42: 20–26.
- Laborda PR, Oliveira KM, Garcia AAF, Paterniani MEAGZ, de Souza AP. 2005. Tropical maize germplasm: what can we say about its genetic diversity in the light of molecular markers?. Theoretical and Applied Genetics 111: 1288–1299.
- Lagat M, Danson J, Kimani M, Kuria A. 2008. Quantitative loci for resistance to Maize streak virus disease in maize genotypes used in hybrid development. African Journal of Biotechnology 7: 2573–2577.
- Li Y, Du J, Wang T, Shi Y, Song Y, Jia J. 2002. Genetic diversity and relationships among Chinese maize inbred lines revealed by SSR markers. Maydica 47: 93–101.
- Liu K, Muse S. 2005. PowerMarker: an integrated analysis environment for genetic marker analysis. Genetics and Population Analysis 21: 2128–2129.
- Liu K, Goodman M, Muse S, Smith JS, Buckler E, Doebley J. 2003. Genetic structure and diversity among maize inbred lines as inferred from DNA microsatellites. Genetics 165: 2117–2128.
- Magenya OEV, Mueke J, Omwega C. 2008. Significance and transmission of Maize streak virus disease in Africa and options for management. African Journal of Biotechnology 7: 4897–4910.
- Martin DP, Shepherd DN. 2009. The epidemiology, economic impact and control of maize streak disease. Food Security 1: 305–315.
- Masi P, Spagnoletti-Zeuli PL, Donini P. 2003. Development and analysis of multiplex microsatellite markers sets in common bean (Phaseolus vulgaris L.). Molecular Breeding 11: 303–313.
- Mawere S, Vincent V, de Meyer J, Pixley KV. 2006. Resistance of four inbred maize lines to inoculation with 20 isolates of Maize streak virus from Zimbabwe. Plant Disease 90: 1485–1489.
- Melchinger AE. 1999. Genetic diversity and heterosis. In: Coors JG, Pandey S (eds), The genetics and exploitation of heterosis in crops. Madison: American Society of Agronomy, Crop Science Society of America. pp 99–118.
- Meyer RC, Otto T, Becher M, Altmann T. 2004. Heterosis of biomass production in Arabidopsis establishment during early development. Plant Physiology 134: 1813–1823.
- Molnar S, Rai JS, Charette M, Cober ER. 2003. Simple sequence repeat (SSR) markers linked to E1, E3, E4 and E7 maturity genes in soybean. Genome 46: 1024–1036.
- Nei M. 1973. Analysis of gene diversity in subdivided populations. Proceedings of the National Academy of Sciences of the USA 70: 3321–3323.
- Oluwafemi S, Thottappilly G, Alegbejo MD. 2008. Search for variability among mastreviruses showing symptoms of Maize streak virus from different regions of Nigeria by polymerase chain reactions. Journal of Plant Protection Research 48: 1–6.
- Pernet A, Hoisington D, Franco J, Isnard M, Jewell D, Jiang C, Marchand JL, Reynaud B, Claszmann JC, Gonzalez de Leon D. 1999. Genetic mapping of Maize streak virus resistance from the mascarene source. Resistance in line D211 and stability against different virus clones. Theoretical and Applied Genetics 99: 524–539.
- Redinbaugh MG, Jones MW, Gingery RE. 2004. The genetics of virus resistance in maize (Zea mays L.). Maydica 49: 183–190.
- Reif JC, Melchinger AE, Xia XC, Warburton ML, Hoisington DA, Vasal SK, Srinivasan G, Bohn M, Frisch M. 2003. Genetic distance based on simple sequence repeats and heterosis in tropical maize populations. Crop Science 43: 1275–1282.
- Rohlf FJ. 1998. NTSYSpc numerical taxonomy and multivariate analysis system version 2.0. Setauket, NY: Exeter Software.
- Salem KFM, El-Zanaty AM, Esmail RM. 2008. Assessing wheat (Triticum aestivum L.) genetic diversity using morphological characters and microsatellite markers. World Journal of Agricultural Sciences 5: 538–544.
- Shahnejat-Bushehri AA, Torabi S, Omidi M, Ghannadha MR. 2005. Comparison of genetic and morphological distance with heterosis with RAPD markers in hybrids of Barley. International Journal of Agriculture and Biology 7: 592–595.
- Sharopova N, McMullen MD, Schultz L, Schroeder S, Sanchez-Villeda H, Gardiner J, Bergstrom D, Houchins K, Melia-Hancock S, Musket T. 2002. Development and mapping of SSR markers for maize. Plant Molecular Biology 48: 463–481.
- Shepherd DN, Mangwende T, Martin DP, Bezuidenhout M, Thomson JA, Rybicki EP. 2007. Inhibition of Maize streak virus (MSV) replication by transient and transgenic expression of MSV replication-associated protein mutants. Journal of General Virology 88: 325–336.
- Shull GH 1952. Beginnings of the heterosis concept. In: Gowen JW (ed.), Heterosis. Ames: Iowa State University Press. pp 14–48.
- Smith JSC, Smith OS. 1989. Comparison of heterosis among hybrids as a measure of relatedness with that to be expected on the basis of pedigree. Maize Genetics Cooperative Newsletter 63: 86–87.
- Smith JSC, Chin ECL, Smith OS, Wall SJ, Senior ML, Mitchell SL, Kresovich S, Ziegle J. 1997. An evaluation of the utility of SSR loci as molecular markers in maize (Zea mays L.): comparisons with data from RFLPs and pedigree. Theoretical and Applied Genetics 95: 163–173.
- Stevens R. 2008. Prospects for using marker-assisted breeding to improve maize production in Africa. Journal of the Science of Food and Agriculture 88: 745–755.
- Taramino G, Tingey S. 1996. SSR for germplasm analysis and mapping in maize. Genome 39: 277–287.
- Vigouroux Y, Mitchell S, Matsuoka Y, Hamblin M, Kresovidi S, Smith SJC, Jaqueth J, Smith OS, Doebley J. 2005. An analysis of genetic diversity across the maize genome using microsatellites. Genetics 169: 1617–1630.
- Virmani SS, Sun ZX, Mou TM, Jauhar Ali A, Mao CX. 2003. Two-line hybrid rice breeding manual. Los Baños, Philippines: International Rice Research Institute.
- Warburton M, Crossa J. 2002. Data analysis in the CIMMYT Applied Biotechnology Center for fingerprinting and genetic diversity studies (2nd edn). Mexico, DF: CIMMYT. Available at http://repository.cimmyt.org/xmlui/bitstream/handle/10883/3493/79065.pdf?sequence=1 [accessed 9 November 2012].
- Warburton ML, Xianchun X, Ambriz S, Diaz L, Vasal SK, Gonzalez F. 2004. Genetic relationships among the CIMMYT maize lines developed for Asia and from other CIMMYT breeding programs. In: Srinivasan G, Zaidi PH, Prasanna BM, Gonzalez F, Lesnick K (eds), Proceedings of the Eighth Asian Regional Maize Workshop: New technologies for the new millenium, 5–8 August 2002, Bangkok, Thailand. Mexico, DF: CIMMYT. pp 3–9. Available at http://repository.cimmyt.org/xmlui/handle/10883/778 [accessed 9 March 2011].
- Wegary D, Vivek B, Labuschagne M. 2013. Association of parental genetic distance with heterosis and specific combining ability in quality protein maize. Euphytica 191: 205–216.
- Weising K, Nybom H, Wolff K, Kahl G. 2005. DNA fingerprinting in plants: principles, methods and applications (2nd edn). London: Taylor and Francis/CRC Press.
- Welz HG, Schechert A, Pernet A, Pixley KV, Geiger HH. 1998. A gene for resistance to the Maize streak virus in the African CIMMYT maize inbred line CML202. Molecular Breeding 4: 147–154.
- Xia XC, Reif JC, Melchinger AE, Frisch M, Hoisington DA, Beck D, Pixley K, Warburton ML. 2005. Genetic diversity among CIMMYT maize inbred lines investigated with SSR markers. Crop Science 45: 2573–2582.
- Xu S-X, Liu J, Lui G-S. 2004. The use of SSRs for predicting the hybrid yield and yield heterosis in 15 key inbred lines of Chinese maize. Hereditas 141: 207–215.
- Yao Q, Yang K, Pan G, Rong T. 2007. Genetic diversity of maize (Zea mays L.) landraces from Southwest China based on SSR data. Journal of Genetics and Genomics 34: 851–860.
- Yu K, Park SJ, Poysa V, Gepts P. 2000. Markers into a molecular linkage map of common bean (Phaseolus vulgaris L.). Journal of Heredity 91: 429–434.
- Yuan L, Zhang S, Warburton ML, Li X, Fu J, Li M. 2002. Assessment of genetic similarities among maize inbred lines using SSR markers. In: Srinivasan G, Zaidi PH, Prasanna BM, Gonzalez F, Lesnick K (eds), Proceedings of the Eighth Asian Regional Maize Workshop: New technologies for the new millenium, 5–8 August 2002, Bangkok, Thailand. Mexico, DF: CIMMYT. pp 50–58. Available at http://repository.cimmyt.org/xmlui/handle/10883/778 [accessed 9 March 2011].