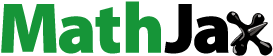
Abstract
This article provides a second manifestation of a new tradition by which the editors of Comments on Inorganic Chemistry wish to lead by example, whereby we start publishing original research content that, nonetheless, preserves the Journal’s identity as a niche for “critical discussion of the current literature” of inorganic chemistry. (For the first manifestation, see: Otten, B. M.; Melancon, K. M.; Omary, M. A. “All That Glitters is Not Gold: A Computational Study of Covalent vs Metallophilic Bonding in Bimetallic Complexes of d10 Metal Centers—A Tribute to Al Cotton on the 10th Anniversary of His Passing,” Comments Inorg. Chem. 2018, 38, 1–35.) Thus, herein we show that a trinuclear copper (I) complex {[3,5-(CF3)2Pz]Cu}3 (henceforth referred to as the “Cu trimer”) can act as a “metal-organic coating” for corrosion protection of aluminum, whereas its silver analogue, {[3,5-(CF3)2Pz]Ag}3 (i.e., the “Ag trimer”), could not. The manuscript was initially submitted to journals that usually publish on “organic coatings” but was rejected on the premise that a metal complex cannot be considered “organic” unless it is incorporated into a polymer. This issue is commented upon herein in the broader context of whether to consider metal complexes “organic,” “inorganic,” “organometallic,” or “metal-organic” materials with manifestations of the use of each classification in the literature. We have found that, upon coating the Cu trimer onto an aluminum (AA 3003) surface, potentiodynamic polarization results in 3.5% NaCl show an increase in corrosion potential (Ecorr) by ~ 0.6 V concomitant with a three-order-of-magnitude decrease in corrosion current density (icorr) from 0.025 µA/cm2 for uncoated aluminum to ~ 9.6 × 10–5 µA/cm2 for the Cu trimer-coated surface. With a double coating, the Cu trimer formed a completely insulating surface with no current flow, even at very high potential magnitude and range. Open circuit potential was used to study the stability of the Cu trimer films on the Al surface in the electrolyte solution. Scanning electron microscopy and Fourier-transform infrared spectroscopy techniques were used to characterize the structure of both the Cu trimer powder and Cu trimer film on the aluminum surface before and after the corrosion tests. The hydrophobicity of the Cu trimer coating was determined by using water drop contact angle measurements, which demonstrated an increase from 65° to 137° for the uncoated and coated aluminum, respectively. The thermal stability of the Cu trimer was analyzed using thermogravimetric analysis, giving rise to weight loss resistance up to ~190 °C. The results clearly demonstrate that the Cu trimer layers exhibit superior stability and potential for corrosion protection of aluminum surfaces in corrosive environments. The Ag trimer analogue, meanwhile, failed the “tape test” that the Cu trimer passed to assess the mechanical stability of such “metal-organic” coatings. Density functional theory (DFT) simulations provide insights on this difference upon modeling the interaction of each cyclotrimer molecule (and other analogous ones) with an Al atom on the one hand and contrasting the resulting binding energies with the corresponding dissociation energies of the metallophically-bound crystalline solid form of each trimer. Thus, it was found that Ag trimer models are bound to the Al atom at least as strongly as Cu trimer models are; yet, that bonding is not sufficiently high so as to overcome the argentophilic attraction, whereas it can overcome the cuprophilic attraction. Other explanations are also given to account for trimer interactions with aluminum oxide as well as partial oxidation of only the Cu trimer, which strengthens the interaction with the Al atom.
Graphical Abstract
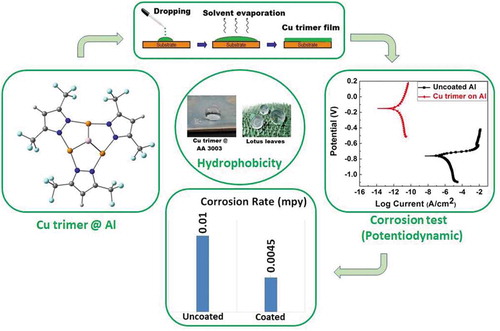
1. INTRODUCTION
The high hydrophobic properties for fluorinated polymers and metal-organic frameworks are useful for oil spill containment, hydrocarbon storage, carbon capture, and oxygen delivery,[Citation1–Citation3] among other applications.[Citation4,Citation5] Other potential benefits include using such materials as coatings to protect various metal substrates used in construction, such as aluminum and steel. The highly fluorinated copper(I)-pyrazolate trimer {[3,5-(CF3)2Pz]Cu}3 (aka “Cu trimer”),[Citation6a] which is shown in , is a well-known cyclic trinuclear complex in inorganic chemistry and photophysics owing to its demonstration of a plethora of remarkable bonding and optoelectronic properties.[Citation6b–Citation6e] This copper trimer, owing to its 18 F atoms on its six trifluoromethyl substituents per molecule, is herein investigated for its high hydrophobic character to possibly impart corrosion protection on Al substrates due to the concomitantly low surface energy expected for fluorinated functional groups. Thus, coatings of this copper trimer can protect substrates from exposure to water or oxygen, which are key components in the corrosion process.
Corrosion is responsible for some of the most expensive failures that take place in metals and other construction materials, resulting in high costs of about $276 billion in the US alone each year.[Citation7] For example, new materials and coatings are needed in fossil-fuel power plants, pipelines, metal processing equipment, transportation vehicles, chemical processing equipment, petroleum production and refining equipment, etc. In all of these industries, corrosion plays a crucial role in durability and cost. Generally, corrosion takes place when metal surfaces are exposed to salts, water, acids, and/or bases.[Citation8] In order to avoid a destructive corrosion attack, metal surfaces must be protected by using materials that have high corrosion resistance. One of the most effective techniques is coating metal surfaces with hydrophobic materials that prevent these surfaces from being exposed to corrosive environments.[Citation9] Significant improvements in corrosion protection beyond the current state of the art of such hydrophobic coatings, however, remain a dire need in order to mitigate the aforementioned high cost and associated adverse environmental impacts in global societies.
Research to develop new technologies and materials for preventing corrosion is an ongoing process. Corrosion protection coatings can be divided into two main types: barrier coating and galvanizing coating. Barrier coatings form layers that separate the underlying metal from oxygen and electrolyte attack. Galvanic coatings, on the other hand, entail materials such as zinc acting as sacrificial agents to prevent corrosion of the substrate material.[Citation10–Citation12]
Aluminum is a commonly used metallic construction material, possessing favorable properties, yet exhibiting low corrosion resistance.[Citation13–Citation16] To increase the corrosion resistance of aluminum, hydrophobic materials have been tried as barrier-type coatings. Zheng et al.[Citation17] developed a superhydrophobic coating on aluminum with a contact angle of 155.2°. Their coating showed a two-order-of-magnitude reduction in the corrosion current density (icorr) after electrochemical measurement. Silane coatings, which are commonly used as hydrophobic coatings for corrosion protection on aluminum surface, also showed reduction in the corrosion current density by less than one order of magnitude.[Citation18,Citation19]
In this work, we have for the first time identified and demonstrated the utility of the non-organometallic trinuclear copper(I) complex, {[3,5-(CF3)2Pz]Cu}3 (i.e., no metal-carbon bonds as all Cu(I) atoms are bonded to N atoms in the 1- and 2- positions of the bridging pyrazolate ring), as a corrosion protection agent on Al surfaces. The fluorinated Cu trimer and its analogues are well-documented for rich photophysical properties arising from inter- and intramolecular Cu…Cu interactions.[Citation6a–Citation6e] Such systems are mostly studied for optoelectronic applications. Since the copper trimer is expected to have good hydrophobic properties, we have demonstrated the use of the complex as a corrosion-protection coating film on an aluminum substrate. A very simple drop casting technique[Citation20] is used and the hydrophobicity, thermal stability, and corrosion protection properties are measured.
The work herein also addresses material-type categorization of metal complexes of the Cu trimer type as “organic,” “inorganic,” “organometallic,” or “metal-organic.” In addition to this issue (addressed in the point/counterpoint commentary in Sec. 4.2), the computational parts in Sec. 3.9 represent the other major portion to transform a manuscript of this type to fit the scope of Comments on Inorganic Chemistry instead of a materials science journal. Thus, text to this effect in the Abstract, Sec. 3.9, and Sec. 4.2 would manifest the new tradition whereby original research is introduced that, nonetheless, preserves the Journal’s identity as a niche for “critical discussion of the current literature” of inorganic chemistry in a full article. Alternatively, prospective authors who wish to publish a short communication may be advised to follow the commentary in either Sec. 4.2 in this article or the analogous section in its precedent sister article.[Citation21] For the short communication model, prospective authors may simply use the published literature to establish the context and incorporate any suitable illustrations from it, instead of the original research akin to that narrated in the experimental and computational data that follow.
2. EXPERIMENTAL PROCEDURES AND COMPUTATIONAL PROTOCOLS
2.1. Pretreatment of Aluminum Sample
Aluminum alloy (3003), which has the chemical composition of 0.2% Cu, 0.7% Fe, 1.5% Mn, 0.6% Si, 0.1% Zn (wt%), with the balance Al, was cut into 1 cm2 squares, and polished with a sequence of grit papers of different grades (400, 600, and 1200), then washed with distilled water to remove particulates. To activate the surface, aluminum was immersed in a solution of 5% NaOH for five minutes, followed by cleaning with distilled water and then acetone.
2.2. Coating with the Copper Trimer
The Cu trimer powder, which our research team synthesized following a published procedure,[Citation6a] was dissolved in acetone (0.1 g Cu trimer in 1 mL acetone), and the resulting solution was drop-casted onto the aluminum alloy 3003 surface. Spontaneous evaporation of the solvent resulted in a homogenous film of the Cu trimer on the aluminum surface with a controlled thickness of 20 ± 9 µm. The double coating was made by applying the same procedure twice to the same substrate with a thickness of 34 ± 7 µm.
2.3. Characterization
The Cu trimer powder and film were studied by Fourier transform infrared (FTIR) spectroscopy using a Thermo Scientific Nicolet 6700 FTIR spectrometer. The samples were run from 4000 to 500 cm–1. FTIR was used also to characterize the structure of the film after the corrosion test. Scanning electron microscopy (SEM) was done using an FEI Nova NanoSEM 230 instrument with an ETD detector to investigate the morphology of the coatings. The thermal stability of the Cu trimer powder and film, before and after corrosion testing, were investigated by thermogravimetric analysis (TGA) with a TGA Q50 V6.7 system. A contact angle measurement was used to measure the hydrophobicity of the Cu trimer using an Infinity 2 Powerscope with GIMP software system after casting a water drop to the surface of the coated substrate; control measurements were performed for uncoated AA 3003. The thickness of the film on the aluminum surface was analyzed using a Veeco Dektak 150 profilometer system.
2.4. Corrosion Testing
All corrosion tests and electrochemical experiments were done using an EG&G Parstat 4000 potentiostat instrument connected to a three-electrode cell. The working electrode was a sheet of aluminum alloy 3003 (bare Al and coated Al). The counter electrode was platinum mesh and the reference electrode was saturated calomel electrode (SCE), to which all voltage values quoted in the following are referenced. Before each test, the working electrode was immersed in the test solution for 1200 s to attain a steady-state open circuit potential (OCP). The potentiodynamic polarization runs were carried out at room temperature in a 3.5% NaCl aqueous solution with a range of ± 250 mV versus OCP and a scan rate of 0.1666 mV/s. Polarization resistance (Rp) was measured by using the linear polarization resistance (LPR) method at a scan rate of 0.1667 mV/s and ±20 mV from OCP.
2.5. Computational Protocols
The binding of aluminum atom to the trimers was studied using the Gaussian09 suite of programs[Citation22] employing Truhlar’s functional (M06)[Citation23] in conjunction with the CEP-31G(d) basis set.[Citation24–Citation26] All structures were optimized to true minima with no imaginary frequencies. The aluminum atom was handled as a neutral doublet binding to a neutral singlet of each trimer investigated, including unsubstituted [M(µ-Pz)]3 with M = Cu, Ag, or Au, and the substituted Cu trimer herein, [Cu(µ-Pz(CF3)2)]3, its Ag trimer analogue [Ag(µ-Pz(CF3)2)]3, and the non-fluorinated version of the latter, [Ag(µ-Pz(Me)2)]3. The molecular electrostatic potential (MEP) surfaces for the trimers alone were generated using an isovalue of 0.02 and a range of –2 × 10–2 to 2 × 102 atomic units.
3. RESULTS AND DISCUSSION
3.1. Spectroscopy Characterization (FTIR)
shows the FTIR spectra for the Cu trimer powder and Cu trimer as a film coated on an aluminum surface before and after corrosion testing.
Figure 2 FTIR spectra of the Cu trimer powder (a) and Cu trimer films before (b) and after (c) corrosion test.
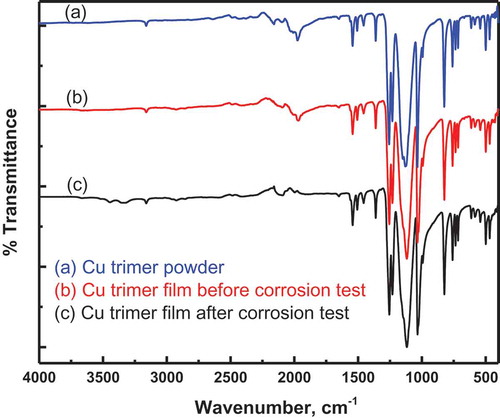
The FTIR spectra of the powder and film before and after corrosion testing show very similar absorption profiles, even in the fingerprint region. Based on these FTIR data, we conclude that there is no significant change in the structure of the Cu trimer powder after dissolving in acetone and then depositing on the substrate. Even after the potentiodynamic polarization test in the corrosive 3.5% NaCl environment for two hours, there was still no significant change in the molecular or packing structures of the Cu trimer coating, as one surmises from the IR data. Thus, the major peaks at 1032 cm–1 (C-H bend), 1126 cm–1 (C-F stretch), and 1257 cm–1 (C-N stretch) remain unaltered, and so do other functional group or fingerprint peaks in general. This is a very encouraging finding as, on many occasions, the corrosion protection agents are known to be sacrificial or undergo changes in their chemistry and topology after being subjected to such aggressive corrosion testing experiments.[Citation10–Citation12]
3.2. Coating Morphology
shows multi-faceted results that shed light onto the morphology of the coating, including scanning electron microscopy images of uncoated aluminum alloy 3003 (A) vs that coated by the Cu trimer (B); energy-dispersive X-ray spectroscopy (EDX) for the coated substrate (C); and optical images of the luminescent Cu trimer film on the coated substrate before (D) and after (E) the corrosion test, upon illumination with a hand-held UV lamp. SEM images have been collected to assess the morphology and homogeneity of the Cu trimer film on Al substrate. When the substrate is coated by the Cu trimer ()), cracks appear in the coating. This type of cracking is typical for coatings made from wet processing techniques, where shrinkage of the film takes place during the drying process.[Citation27,Citation28] To prove that this crack does not reach the aluminum surface, energy-dispersive X-ray spectroscopy (EDX) was used by focusing inside the crack and measuring the peaks for Al and Cu. EDX shows ~ 98% peak of Cu from Cu trimer layer, while Al is only ~ 2%, indicating that the crack does not reach the aluminum surface. These results illustrate rather clearly that cracks on the surface of the film are only superficial and do not have impeding effects on the corrosion protection property of the Cu trimer. More definitively, we managed to acquire additional SEM images that show a side view of the coating, which reveals both the aluminum substrate and copper trimer coating simultaneously (). These specimens unmistakeably show that the surface cracks do not extend to the substrate, manifesting a good quality of the Cu trimer as a continuous, contiguous coating. Yet further evidence is shown by the optical images of the luminescence of the Cu trimer film that, remarkably, was sustained to be exhibited uniformly, even after performing the potentiodynamic polarization test in the corrosive 3.5% NaCl air-saturated aqueous environment. The latter result of is also significant toward encapsulation applications of electronic devices, such as inorganic or organic light-emitting diodes (LEDs and OLEDs, respectively) and solar cells, which are also prone to corrosion or at least significant compromise in their efficacy upon penetration of O2 and/or H2O into their active materials.
Figure 3 Top view of SEM images of uncoated aluminum alloy 3003 (a) vs that coated by the Cu trimer (b); EDX for the same coated substrate (c) used in B; side view of SEM images of two other aluminum alloy 3003s coated by the Cu trimer (d and e), showing both the substrate and coating simultaneously; and optical images of the luminescent Cu trimer film on the coated substrate before (f) and after (g) the corrosion test.
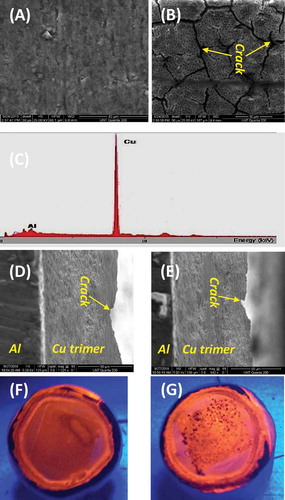
3.3. Thermogravimetric Analysis (TGA)
shows thermal stability graphs for the Cu trimer powder and film, the latter before and after the corrosion test.
As shown in these TGA graphs, the Cu trimer (powder and films) is thermally stable up to a temperature of ~190 °C, and the decompositon temperature is in the range of 190–230 °C. Thermal stability for Cu trimer comes, in part, from the strong bond between carbon and fluorine (C-F), a strong and stable bond. Such high-temperature-resistant materials are good for corrosion protection in harsh environments.
3.4. Contact Angle
provides a comparison of water drop contact angle photographs on uncoated and coated aluminum surfaces. In general, the fluorinated molecules are known to exhibit strong hydrophobic behavior. As shown in , the water droplet contact angle on the Cu trimer film is 137°, which indicates superhydrophobic behavior for this material. Increasing in the contact angle from 65° for bare aluminum to 137° for Cu trimer coating confirms that the deposition of the Cu trimer layer on the aluminum substrate enhances the hydrophobic behavior of the metal surface, which reduces the contact area between the liquid and the solid surface.
3.5. Corrosion Protection Properties of the Cu Trimer Coating
) and show the potentiodynamic polarization runs for both the uncoated and coated aluminum alloy 3003 substrates. For ), the measured Ecorr is –0.77 V for uncoated aluminum, which matches the Ecorr for this type of alloy as measured by several other researchers.[Citation29] However, for the Cu trimer film, the Ecorr has shifted anodically to –0.15 V, which is interesting since this value is more like an Ecorr for a copper coating. The corrosion current density significantly decreased for the coated aluminum compared to uncoated aluminum; i.e., from 2.5 × 10–2 for the latter to 9.6 × 10–5 µA/cm2 for the former. These results indicate the corrosion resistance for Cu trimer as a single coating on the aluminum surface. An Ag trimer film shows no significant anodic shift with higher corrosion current density compared to uncoated aluminum, indicating poor corrosion protection, which could be due to adhesion issues; see Sec. 3.8 and Sec. 3.9.
Figure 6 (a) Potentiodynamic polarization for uncoated (bare aluminum) and aluminum coated by a single coating of the Cu trimer and Ag trimer films; (b) potentiodynamic polarization for uncoated (bare aluminum) and aluminum coated by a double coating of the Cu trimer film.
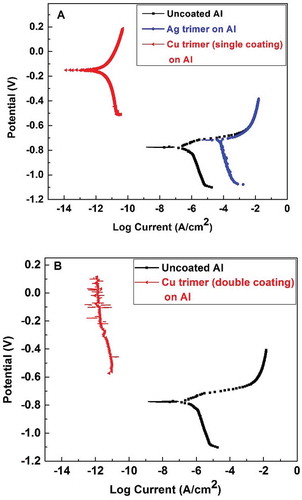
) shows the same polarization curve for the aluminum sample with Ecorr still at –0.77 V, whereas the double-coating of the Cu trimer film completely prevented current flow, even when the applied potential increased to +0.1 V. This indicates an insulation property for the Cu trimer when used as a double-coating on aluminum surface.
3.6. Corrosion Rate Calculation
For a more quantitative comparison and to measure corrosion rate, potentiodynamic polarization measurements were used to obtain βa and βc (anodic and cathodic slopes). These parameters are used with the polarization resistance (Rp), measured from LPR, to calculate the corrosion current density (icorr) from the Stern-Geary equation (Eq. 1).[Citation30]
The following equation (Eq. 2)[Citation31] can be used to calculate corrosion rate after icorr has been determined, where E.W. = equivalent weight of the corroding species, g, d = density of the corroding species, g/cm2 and mpy = milli inches per year:
The protection efficiency (PE), which represents the efficiency of the coating to protect the metal surface from the current flow, can be obtained from the measured current density values by using the following equation (Eq. 3),[Citation32] where icorr and icorr(C) = corrosion current density for uncoated aluminum and aluminum coated by Cu trimer, respectively:
Higher PE% values correspond to a lower corrosion current density (icorr) flow between the electrolyte solution and metal surface. Recently, Bagherzadeh et al.[Citation33] reported on the electrochemical corrosion behavior of magnetite/PANI nanocomposite coatings and obtained a maximum PE of 96.8% in their work.
lists the corrosion parameters and shows a positive shift in corrosion potential, –777 to –151 mV and increasing polarization resistance, 1.80 × 103 to 1.34 × 106 Ω/cm2, after the aluminum surface was coated by the Cu trimer. It also shows a decrease in corrosion current density, 2.50 × 10–2 to 9.64 × 10–5 µA/cm2, and decrease in corrosion rate, 1.00 × 10–2 to 4.50 × 10–3 mpy, for bare aluminum and the aluminum surface coated by a single coating of Cu trimer film, respectively. As a reduction in icorr for aluminum coated by the Cu trimer, this coating shows protection efficiency of PE = 99.6%. These results demonstrate strong corrosion protection behavior of the Cu trimer coating on the aluminum surface. All electrochemical tests were done for three samples of Cu trimer film on AA 3003; test data for these samples are represented in as an average ± standard deviation.
Table 1 Potentiodynamic polarization plot data for uncoated aluminum and aluminum coated by Cu trimer
3.7. Open Circuit Potential (OCP)
Potential vs time measurements for bare aluminum and aluminum coated by the Cu trimer film are shown in . The measured potential for the coated sample is higher than the potential for the uncoated sample during the time of immersion study. The OCP of aluminum coated by the Cu trimer film remained stable over the extended period of 30 days and the Ecorr value for the coating, –155 mV, matched the OCP value from day 30, indicating the stability of the Cu trimer film in an aggressive medium of 3.5% NaCl.
3.8. Adhesion Properties
shows the results of a preliminary tape test (non-standard) for measuring the adhesion of films of both the Cu trimer and its analogous Ag trimer on the aluminum surface herein. The Cu trimer film did not peel significantly with the tape, giving rise to a less than 5% removal of the film area. The rate of adhesion is categorized as “4B” according to ASTM Standard D3359, which indicates that the Cu trimer layer sticks strongly on the aluminum surface. In contrast, visual inspection alone is sufficient to demonstrate the inferior mechanical stability of the Ag trimer film, which peeled significantly with this preliminary tape test ().
3.9. DFT Simulations
We have carried out some density functional theory (DFT) simulations in an attempt to provide insights into the difference between the Cu trimer vs Ag trimer in terms of interaction with an Al atom. Results are shown in and 10 and . The approach is based on that employed by Chilukuri and Cundari, described elsewhere,[Citation34] which offers mere qualitative insights into bonding trends among material variations, given that the substrate surface is approximated by a single metal atom; more rigorous approaches are in order for subsequent investigations. With that qualification in mind, we are able to justify the adhesion of the Cu but not the Ag trimer films (), based on a combination of the theoretical insights in and on the one hand and literature precedents from our previous work on such coinage metal trimers[Citation35–Citation37] on the other. shows that both Cu and Ag trimers herein could form a half-sandwich adduct with an aluminum atom with an average Al-M distance of 2.72 and 2.85 Å, respectively; the corresponding distance to the centroid of each trimer is more similar at ~2.1 Å at the same M06 level of theory (). Naïvely, one could be misled by the 13 pm longer Al-Ag than Al-Cu distance to justify the better adhesion of the Cu film. However, our previous work[Citation34]—reinforcing and expanding that of Schmidbaur and co-workers[Citation38]—on covalent radii of monovalent coinage metals gives rise to ca. 20 pm differential between the two metals (rCu(I) = 1.11 or 1.13 Å vis-à-vis rAg(I) = 1.34 or 1.33 Å based on ref.[Citation34] or ref.[Citation36], respectively). Indeed, electrostatic interactions are more conducive to stronger bonding of Al to the Ag trimer, given its much larger quadrupole moment tensor, Qzz, than that of the Cu trimer (), and this result is consistent with previous findings of π-acidity trends[Citation37] reproduced in for the unsubstituted coinage metal pyrazolate cyclotrimers. Thus, the relative binding energy results are more trustworthy than the trimer-Al distances to derive conclusions from en route to justifying the experimental difference in adhesion between the Cu and Ag trimers on aluminum surfaces. shows that Ag trimer models are bound to the Al atom at least as strongly as Cu trimer models are, however! The slightly higher binding energy of < 1 kcal/mol for the Al bonding to the fluorinated Cu trimer vs Ag trimer is too small to draw a conclusion in support of the stronger adhesion of the Cu trimer (). Our best justification of the experimental adhesion findings lies in comparing the Al-trimer binding energies in with the magnitude of metallophilic attraction between the cyclotrimer molecules, which needs to be overcome for a trimer molecule to interact with the Al atom or an aluminum-based substrate. Yet, that bonding is not sufficiently high so as to overcome the argentophilic attraction, whereas it can overcome the cuprophilic attraction. The former is much stronger, as evidenced experimentally by the crystallographic intertrimer distance of 3.20 Å for Ag…Ag vs 3.81 and 3.99 Å for Cu…Cu.[Citation34] M06/CEP-31G(d) calculations attained 31.4 kcal/mol for the binding energy (dominated by argentophilic attraction) in the {[Ag(μ-Pz(CF3)2)]3}2 dimer-of-trimer model.[Citation35] This energy is approximately twice the binding energy of the Al atom to the [Ag(μ-Pz(CF3)2)]3 trimer molecule. Thus, the Al bonding is too weak to overcome the argentophilic attraction, but strong enough to overcome cuprophilic attraction in the loosely held chains of [Cu(μ-Pz(CF3)2)]3 molecules with ~3.9 Å average intertrimer separations (at which DFT predicts only ~2 kcal/mol dissociation energies[Citation36]) that could be overcome by the much stronger bonding with Al ().
Table 2 Binding energy of the Al atom and distance between the Al atom and centroid of the M3 unit in a half-sandwich adduct of the Al atom with various unsubstituted and substituted cyclotrimers, according to M06/CEP-31G(d) simulations
Figure 9 Top: Geometries of the Al atom binding to the Cu (left) and Ag (right) trimers. Bottom: MEP surfaces of the trimers alone. Results are according to M06/CEP-31G(d) simulations. The Qzz quadrupole tensor values are labeled for the trimers alone, representing the highest magnitude (z axis is normal to the cylotrimer plane).
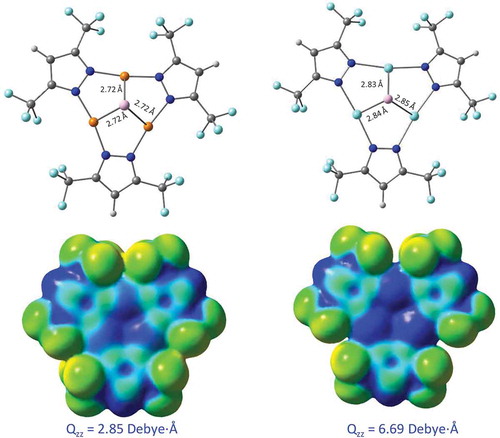
Figure 10 Left panel: Molecular structures (top), electrostatic potential showing positive and negative regions in space (ESP/middle), and molecular electrostatic potential (MEP/bottom) of unsubstituted coinage metal pyrazolate cyclotrimers. Right panel: Positive charge attraction (PCA) curves for the three trimers. Results are according to M06/CEP-31G(d) simulations using Gaussian 09 for ESP/MEP and using GAMESS for PCA. The Qzz quadrupole tensor values for the three trimers are –17, –15, and –20 Debye.Å with M = Cu, Ag, and Au, respectively, when unsubstituted, whereas the magnitude becomes positive upon CF3 substitution (see ).
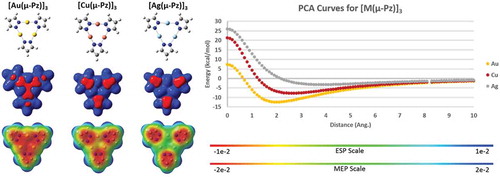
Other explanations of the stronger adhesion of the Cu trimer than that of the Ag trimer include the following arguments: (1) The interactions with aluminum oxide (instead of aluminum atom) are more relevant because the Al substrate oxidizes immediately after polishing; in fact, when we polish the AA 3003 substrate, one can visually see the mirror finish changing rather starkly as the oxide layer develops on the surface almost immediately, forming a very thin Al2O3 layer. As such, the interactions with Al2O3 instead of Al should be stronger for the Cu trimer than the Ag trimer, as Cu(I) is more oxophilic than Ag(I) by virtue of simple hard and soft acids and bases (HSAB) concepts proposed by Pearson in 1973.[Citation39] (2) Visual inspection suggests partial oxidation of only the Cu trimer—to form a blue color instead of the original powder’s white color—but not the Ag trimer, whose thin film remains white (). This should then strengthen the interaction with the Al atom only for the Cu trimer, again using the HSAB concepts.[Citation39] Interestingly, the bright orange luminescence of the copper trimer’s thin film was maintained uniformly, despite the oxidation of the Cu(I) to Cu(II) metal center, which leads us to deem the oxidation “partial.” (3) The interactions of a single Al atom with the cylotrimer molecules are exaggerated by DFT, given that the real substrate surface of AA 3003 or other aluminum-based substrates have Al atoms bonded with one another as lattice motifs within the extended structure. The electron density available to bond with the Cu or Ag trimer would then be suppressed in these “real” surfaces versus that of the Al atom. It will be more accurate to quantify the cyclotrimer interaction energy upon constructing a larger model of the substrate—that being metallic aluminum or aluminum oxide. Nonetheless, the modeling both herein and in ref.[Citation34] is meant to be qualitative to establish trends upon structural or compositional variations, whereas a more quantitative assessment should take into account all three factors (1)-(3) in this section.
4. CONCLUSIONS AND COMMENTARY
4.1. Specific Conclusions about Results in this Work
A copper(I)-based cyclic trinuclear complex, known for its rich photophysical properties, is investigated for the first time to demonstrate its corrosion protection properties on an aluminum alloy (3003) surface. The copper trimer complex was hypothesized to exhibit corrosion protection based on its fluorinated structure, which is expected to lend a hydrophobic character, verified herein. Based on these results, we have demonstrated that this Cu trimer complex does exhibit corrosion protection on an Al substrate. The FTIR spectra indicate that the Cu trimer structure does not change molecular or packing structures after deposition on aluminum surface. TGA results, on the other hand, show high thermal stability to ~200 °C. SEM images coupled with EDX and luminescence imaging demonstrate the contiguity of the Cu trimer film, which covers and protects all of the substrate surface, despite the presence of cracks on the surface. Potentiodynamic polarization runs indicate that the corrosion rate decreased and polarization resistance increased after the Cu trimer deposition on the surface, indicating that the Cu trimer film acts as a barrier layer against corrosion. The Cu trimer film is stable even after 30 days of exposure in a 3.5% NaCl aqueous solution.
4.2. A Point/Counterpoint Commentary on Metal Complexes Being “Organic” vs “Inorganic” or “Metal-Organic” Materials
The narrative described in the abstract regarding the rejection of an earlier version of the manuscript in materials science journals that usually publish on “organic coatings” warrants this commentary about what kind of material does a metal complex constitute? Is it an “organic,” “inorganic,” “organometallic,” or “metal-organic” material?
The argument for the metal complex being an “organic material” can be made on the premise that the copper complex represents an organic coating with a small metal content, as represented by the chemical formula C15H3Cu3F18N6, where carbon and other main group elements represent the bulk of the structure. This is much like the action of Ir(III)-phenylpyridine complexes (e.g., tris(phenylpyridine)iridium(III) = Ir(ppy)3) and metal phthalocyanines (e.g., CuPc) as prototypical active “organic semiconductors” in the so-called organic electronic devices, such as organic light-emitting diodes[Citation40] and organic photovoltaics,[Citation41] respectively. That usage has been the tradition in the applied physics and materials science literature; nobody argues that these semiconductors or devices should be re-named to omit the word “organic” because of the presence of the metal content in the organometallic or metal-containing organic molecule. Indeed, being a first-row transition metal, copper has a lower content in the aforementioned [Cu(μ-Pz(CF3)2)]3 copper trimer formula (wt % = 23.8%) than iridium in Ir(ppy)3 (wt % = 29.3%). The analogous “inorganic” optoelectronic materials and devices typically refer to carbon-free materials such as III-V semiconductors (i.e., binary ionic solids of trivalent group 13 metal cations and trivalent group 15 metal anions, to use the current IUPAC terminology) such as GaN and GaAs,[Citation42] binary metal oxides or chalcogenides such as MoO2 and MoS2,[Citation43] lead halide materials used in perovskite solar cells,[Citation43] or doped Si and Ge materials used in conventional inorganic solar cells,[Citation44] to name a few classes.
One may argue that the Cu trimer (among other metal complexes) does contain carbon, so that it could be considered organic, but would that render it an organic coating? Members of the organic coatings community would usually look for the presence of some kind of organic binder system—a thermoplastic or thermosetting polymer of some sort—in order to meet the definition of an “organic coating,” whereas they do not see such a binder in the work herein. This argument may be reinforced by the fact that prototypical organic electronic devices that contain metal complexes, such as phosphorescent OLEDs, usually incorporate the active phosphor such as Ir(ppy)3 in a matrix at ca. 6–12% by volume within a purely organic (metal-free) host molecule.[Citation40] A rebuttal for this argument, however, may lie in the fact that neat thin films of both fluorescent and phosphorescent metal complexes—embodied by tris(8-hydroxy-quinolinato)aluminum(III)[Citation45] and bis[3,5-bis(2-pyridyl)-1,2,4-triazolato]platinum(II) = [Pt(ptp)2],[Citation46] respectively—have been used in simple bilayer OLEDs, where they performed a dual function, as both the emitting and electron-transporting layer simultaneously, without having to be doped in a polymer or another metal-free organic molecule. The neat layers of the Cu trimer (and its Ag congener) in this article and other related articles [e.g., Citation47], Alq3 in fluorescent OLEDs,[Citation45] or Pt(ptp)2 and other complexes such as FIrpic = bis[(4,6-dif-uorophenyl)pyridinato-N,C2](picolinate)iridium(III) or FIr6 = bis(2,4-difluorophenylpyridinato)[tetrakis(1-pyrazolyl)borato]iridium(III) = FIrpic used in doing-free phosphorescent OLEDs,[Citation46] are all accepted as “organic coatings” or “organic thin films.”
Although the previous arguments, in our opinion, make a valid case to have the results in this work publishable in materials science journals on organic coatings toward corrosion resistance of metal substrates or optoelectronic devices, we hesitate to succumb to the classification of metal complexes as “organic materials.” After all, investigations of structure and properties pertaining to metal complexes fall in the realm of “inorganic chemistry” vis-à-vis “organic chemistry.” On the other hand, the distinction from the aforementioned various classes of conventional inorganic semiconductors is warranted for metal complexes—if nothing else, to avoid confusing the materials science, engineering, and applied physics communities between the two types of materials. Thus, in lieu of designating metal complexes of types like the Cu(I)/Ag(I)/Au(I) trimers herein or other hitherto mentioned Al(III), Pt(II), and Ir(III) complexes as “organic” or “inorganic” materials, we would prefer to opt for the “metal-organic” materials designation. This designation is akin to, and inspired by, the terminology used for metal-organic frameworks (MOFs) that have received increasingly significant attention in the contemporary literature since the turn of the century, as illustrated by several reviews and commentaries in this very journal.[Citation48–Citation51] Finally, the “organometallic” materials categorization is unsuitable because the trinuclear complexes of this type have no metal-carbon bonds, as all coordinate-covalent bonds involved are dative M-N bonds (see , , and ).
ACKNOWLEDGMENTS
The authors acknowledge the Materials Research Facility (MRF) at the University of North Texas (UNT) for access to the SEM experimental facilities used for this study, as well as the Advanced Materials and Manufacturing Processes Institute (AMMPI) at UNT and the Shenzhen Peacock Plan (No. 1208040050847074) for supporting the aspects related to investigating electronic device encapsulation.
DISCLOSURE STATEMENT
No potential conflict of interest was reported by the authors.
Additional information
Funding
REFERENCES
- Yang, C.; Kaipa, U.; Zhang Mather, Q.; Wang, X.; Nesterov, V.; Venero, A. F.; Omary, M. A. Fluorous Metal-Organic Frameworks with Superior Adsorption and Hydrophobic Properties toward Oil Spill Cleanup and Hydrocarbon Storage. J. Am. Chem. Soc. 2011, 133, 18094–18097. DOI: 10.1021/ja208408n.
- Yaghi, O. M.; United States Department of Energy. Technical Report (“Hydrogen Storage in Metal-Organic Frameworks”); 2012, https://www.osti.gov/biblio/1039149 (accessed Oct 20, 2018).
- Andirova, D.; Cogswell, C.; Lei, Y.; Choi, S. Effect of the Structural Constituents of Metal Organic Frameworks on Carbon Dioxide Capture. Microporous Mesoporous Mater. 2016, 219, 276–305. DOI: 10.1016/j.micromeso.
- Chen, T.; Popov, I.; Zenasni, O.; Daugulis, O.; Miljanić, O. Superhydrophobic Perfluorinated Metal–Organic Frameworks. Chem. Commun. 2013, 49, 6846. DOI: 10.1039/c3cc41564c.
- Cui, Y.; Li, B.; He, H.; Zhou, W.; Chen, B.; Qian, G. Metal–Organic Frameworks as Platforms for Functional Materials. Acct. Chem. Res. 2016, 49, 483–493. DOI: 10.1021/acs.accounts.5b00530.
- (a) Dias, H. V. R.; Polach, S.; Wang, Z. Coinage Metal Complexes of 3,5-Bis(Trifluoromethyl)Pyrazolate Ligand. J. Fluorine Chem. 2000, 103, 163–169. (b) Galassi, R.; Ghimire, M. M.; Otten, B. M.; Ricci, S.; McDougald, R. N., Jr.; Almotawa, R. M.; Alhmoud, D.; Ivy, J. F.; Rawashdeh, A. M.; Nesterov, V. N.; Reinheimer, E. W.; Daniels, L. M.; Burini, A.; Omary, M. A. Proc. Natl. Acad. Sci. U.S.A. 2017, 114, E5042–E5051. DOI:10.1073/pnas.1700890114. (c) Omary, M. A.; Rawashdeh-Omary, M. A.; Gonser, M. W. A.; Elbjeirami, O.; Grimes, T.; Cundari, T. R.; Diyabalanage, H, V. K.; Palehepitiya Gamage, C. S.; Dias, H. V. R. Inorg. Chem. 2005, 44, 8200–8210. (d) Dias, H. V. R.; Diyabalanage, H. V. K.; Eldabaja, M. G.; Elbjeirami, O.; Rawashdeh-Omary, M. A.; Omary, M. A. J. Am. Chem. Soc. 2005, 127, 7489–7501. (e) Dias, H. V. R.; Diyabalanage, H. V. K.; Rawashdeh-Omary, M. A.; Franzman, M. A.; Omary, M. A. J. Am. Chem. Soc. 2003, 125, 12072–12073. DOI: 10.1016/s0022-1139(99)00313-9.
- Thurber, C.; Electrodeposited Metal Matrix Composites for Enhanced Corrosion Protection and Mechanical Properties. Ph.D. Dissertation, Toulouse Graduate School, University of North Texas, 2016.
- Yemashova, N.; Murygina, V.; Zhukov, D.; Zakharyantz, A.; Gladchenko, M.; Appanna, V.; Kalyuzhnyi, S. Biodeterioration of Crude Oil and Oil Derived Products: A Review. Rev. Environ. Sci. Biotechnol. 2007, 6, 315–337. DOI: 10.1007/s11157-006-9118-8.
- Shabani-Nooshabadi, M.; Ghoreishi, S.; Behpour, M. Electropolymerized Polyaniline Coatings on Aluminum Alloy 3004 and Their Corrosion Protection Performance. Electrochim. Acta. 2009, 54, 6989–6995. DOI: 10.1016/j.electacta.2009.07.017.
- Tada, E.; Miura, Y. Hydrogen Absorption Behavior into Zn and Zn–Al Coated Steels during Corrosion in Aqueous Solutions. ISIJ Int. 2016, 56, 444–451. DOI: 10.2355/isijinternational.isijint-2015-405.
- Jafarzadeh, S.; Claesson, P.; Sundell, P.; Tyrode, E.; Pan, J. Active Corrosion Protection by Conductive Composites of Polyaniline in a UV-cured Polyester Acrylate Coating. Prog. Org. Coat. 2016, 90, 154–162. DOI: 10.1016/j.porgcoat.2015.10.008.
- Kowalski, D.; Ueda, M.; Ohtsuka, T. Corrosion Protection of Steel by Bi-Layered Polypyrrole Doped with Molybdophosphate and Naphthalenedisulfonate Anions. Corros. Sci. 2007, 49, 1635–1644. DOI: 10.1016/j.corsci.2006.08.018.
- Sherif, E.; Ammar, H.; Khalil, K. Effects of Copper and Titanium on the Corrosion Behavior of Newly Fabricated Nanocrystalline Aluminum in Natural Seawater. Appl. Surf. Sci. 2014, 301, 142–148. DOI: 10.1016/j.apsusc.2014.02.019.
- Musa, A.; Mohamad, A.; Al-Amiery, A.; Tien, L. Galvanic Corrosion of Aluminum Alloy (Al2024) and Copper in 1.0M Hydrochloric Acid Solution. Korean J. Chem. Eng. 2012, 29, 818–822. DOI: 10.1007/s11814-011-0233-z.
- Soliman, M.; El-Danaf, E.; Almajid, A. Effect of Equal-Channel Angular Pressing Process on Properties of 1050 Al Alloy. Mater. Manuf. Processes. 2012, 27, 746–750. DOI: 10.1080/10426914.2012.663150.
- Lashgari, M.; Malek, A. M. Fundamental Studies of Aluminum Corrosion in Acidic and Basic Environments: Theoretical Predictions and Experimental Observations. Electrochim. Acta. 2010, 55, 5253–5257. DOI: 10.1016/j.electacta.2010.04.054.
- Zheng, S.; Li, C.; Fu, Q.; Hu, W.; Xiang, T.; Wang, Q.; Du, M.; Liu, X.; Chen, Z. Development of Stable Superhydrophobic Coatings on Aluminum Surface for Corrosion-Resistant, Self-Cleaning, and Anti-Icing Applications. Mater. Desi. 2016, 93, 261–270. DOI: 10.1016/j.matdes.2015.12.155.
- Calabrese, L.; Bonaccorsi, L.; Caprì, A.; Proverbio, E. Electrochemical Behavior of Hydrophobic Silane–Zeolite Coatings for Corrosion Protection of Aluminum Substrate. J. Coat. Technol. Res. 2014, 11, 883–898. DOI: 10.1007/s11998-014-9597-4.
- Wang, Y.; Li, Y.; Wang, F. Polymeric Organo-Silane Coatings for Aluminum Alloy Corrosion Protection by Self-Assembled Metho. E-J. Chem. 2012, 9, 435–442. ISSN: 0973-4945; CODEN ECJHAO. DOI: 10.1155/2012/548143.
- Liu, Y.; Zhao, X.; Cai, B.; Pei, T.; Tong, Y.; Tang, Q.; Liu, Y. Controllable Fabrication of Oriented Micro/Nanowire Arrays of Dibenzo-Tetrathiafulvalene by a Multiple Drop-Casting Method. Nanoscale. 2014, 6, 1323–1328. DOI: 10.1039/c3nr05680e.
- Otten, B. M.; Melançon, K. M.; Omary, M. A. All That Glitters Is Not Gold: A Computational Study of Covalent vs Metallophilic Bonding in Bimetallic Complexes of d10 Metal Centers—A Tribute to Al Cotton on the Tenth Anniversary of His Passing. Comments Inorg. Chem. 2018, 38, 1–35. DOI: 10.1080/02603594.2018.1467315.
- Frisch, M. J.; Trucks, G. W.; Schlegel, H. B.; Scuseria, G. E.; Robb, M. A.; Cheeseman, J. R.; Scalmani, G.; Barone, V.; Mennucci, B.; Petersson, G. A.; et al. Gaussian, Inc.: Wallingford CT; 2009. http://gaussian.com/g09citation/
- Zhao, Y.; Truhlar, D. The M06 Suite of Density Functionals for Main Group Thermochemistry, Thermochemical Kinetics, Noncovalent Interactions, Excited States, and Transition Elements: Two New Functionals and Systematic Testing of Four M06 Functionals and 12 Other Functionals. Theor. Chem. Acc. 2008, 119, 525. DOI: 10.1007/s00214-007-0401-8.
- Stevens, W.; Basch, H.; Krauss, M. Compact Effective Potentials and Efficient Shared-Exponent Basis Sets for the First- and Second-Row Atoms. J. Chem. Phys. 1984, 81, 6026–6033. DOI: 10.1063/1.447604.
- Stevens, W.; Krauss, M.; Basch, H.; Jasien, P. Relativistic Compact Effective Potentials and Efficient, Shared-Exponent Basis Sets for the Third-, Fourth-, and Fifth-Row Atoms. Can. J. Chem. 1992, 70, 612–630. DOI: 10.1139/v92-085.
- Cundari, T.; Stevens, W. Effective Core Potential Methods for the Lanthanides. J. Chem. Phys. 1993, 98, 5555–5565. DOI: 10.1063/1.464902.
- Wang, A.; Golden, T. Electrodeposition of Oriented Cerium Oxide Films. Int. J. Electrochem. Sci. 2013, 2013, 1–10.
- Yuan, Q.; Golden, T. Electrochemical Study of Hydroxyapatite Coatings on Stainless Steel Substrates. Thin Solid Films. 2009, 518, 55–60. DOI: 10.1016/j.tsf.2009.06.029.
- Sukiman, N. L.; Zhou, X.; Birbilis, N.; Hughes, A. E.; Mol, J. M. C.; Garcia, S. J.; Zhou, X.; Thompson, G. E. Durability and Corrosion of Aluminium and Its Alloys: Overview, Property Space, Techniques and Developments. In Aluminium Alloys - New Trends in Fabrication and Applications; Ahmad, Z., Ed.; Chapter 2. IntechOpen: London (U.K.); 2012, 54. DOI: 10.5772/53752.
- Gerengi, H.; Goksu, H.; Slepski, P. The Inhibition Effect of Mad Honey on Corrosion of 2007-type Aluminum Alloy in 3.5% NaCl Solution. Adv. Mater. Res. 2013, 17, 255–264.
- Yue, J.; Cao, Y. Corrosion Prevention by Applied Coatings on Aluminum Alloys in Corrosive Environments. Int. J. Electrochem. Sci. 2015, 10, 5222–5237.
- Behpour, M.; Ghoreishi, S.; Soltani, N.; Salavati-Niasari, M.; Hamadanian, M.; Gandomi, A. Electrochemical and Theoretical Investigation on the Corrosion Inhibition of Mild Steel by Thiosalicylaldehyde Derivatives in Hydrochloric Acid Solution. Corros. Sci. 2008, 50, 2172–2181. DOI: 10.1016/j.corsci.2008.06.020.
- Bagherzadeh, M.; Haddadi, H.; Iranpour, M. Electrochemical Evaluation and Surface Study of magnetite/PANI Nanocomposite for Carbon Steel Protection in 3.5% NaCl. Prog. Org. Coat. 2016, 101, 149–160. DOI: 10.1016/j.porgcoat.2016.08.011.
- Chilukuri, B.; Cundari, T. R. Modeling the Deposition of Metal Atoms on a p-Type Organometallic Conductor: Implications for Stability and Electron Transfer. J. Phys. Chem. C 2011, 115, 5997–6003. DOI: 10.1021/jp111337r.
- Galassi, R.; Ricci, S.; Burini, A.; Macchioni, A.; Rocchigiani, L.; Marmottini, F.; Tekarli, S. M.; Nesterov, V. N.; Omary, M. A. Solventless Supramolecular Chemistry via Vapor Diffusion of Volatile Small Molecules upon a New Trinuclear Silver(I)-Nitrated Pyrazolate Macrometallocyclic Solid: An Experimental/Theoretical Investigation of the Dipole/Quadrupole Chemisorption Phenomena. Inorg. Chem. 2013, 52, 14124–14137. DOI: 10.1021/ic401948p.
- Omary, M. A.; Rawashdeh-Omary, M. A.; Gonser, M. W. A.; Elbjeirami, O.; Grimes, T.; Cundari, T. R.; Diyabalanage, H., . V. K.; Palehepitiya Gamage, C. S.; Dias, H. V. R. Metal Effect on the Supramolecular Structure, Photophysics, and Acid-Base Character of Trinuclear Pyrazolato Coinage Metal Complexes. Inorg. Chem. 2005, 44, 8200–8210. DOI: 10.1021/ic0508730.
- Tekarli, S. M.; Cundari, T. R.; Omary, M. A. Rational Design of Macrometallocyclic Trinuclear Complexes with Superior Pi-Acidity and Pi-Basicity. J. Am. Chem. Soc. 2008, 130, 1669–1675. DOI: 10.1021/ja076527u.
- Bayler, A.; Schier, A.; Bowmaker, G. A.; Schmidbaur, H. Gold Is Smaller than Silver. Crystal Structures of [Bis(Trimesitylphosphine)Gold(I)] and [Bis(Trimesitylphosphine)Silver(I)] Tetrafluoroborate. J. Am. Chem. Soc. 1996, 118, 7006. DOI: 10.1021/ja961363v.
- Pearson, R. Ed. Hard and Soft Acids and Bases; Dowden, Hutchison & Ross: Stroudsburg (PA), 1973.
- Huckaba, A. J.; Nazeeruddin, M. K. Strategies for Tuning Emission Energy in Phosphorescent Ir(III) Complexes. Comments Inorg. Chem. 2017, 37, 117–145. DOI: 10.1080/02603594.2016.1207064.
- Bortchagovsky, E. G.; Kazantseva, Z. I.; Koshets, I. A.; Nešpůrek, S.; Jastrabik, L. Optical Properties of Double-Layer Structure Phthalocyanine–Tetracyanoquinodimethane. Thin Solid Films 2004, 460, 269. DOI: 10.1016/j.tsf.2004.01.102.
- Zhao, Y.; Zhang, Y.; Yang, Z.; Yan, Y.; Sun, K. Synthesis of MoS2 and MoO2 for Their Applications in H2 Generation and Lithium Ion Batteries: A Review. Sci. Technol. Adv. Mater. 2013, 14, 043501. DOI: 10.1088/1468-6996/14/4/043501.
- Jiang, Q.; Xu, T. Organic-Inorganic Hybrid Perovskite Materials for “Nova Star” Solar Cells: State of Technology and Outstanding Challenges. Comments Inorg. Chem. 2016, 36, 200–214. DOI: 10.1080/02603594.2015.1116985.
- Shockley, W.; Queisser, H. J. J. Detailed Balance Limit of Efficiency of pn Junction Solar Cells. Appl. Phys. 1961, 32, 510. DOI: 10.1063/1.1736034.
- Tang, C. W.; VanSlyke, S. A. Organic Electroluminescent Diodes. Appl. Phys. Lett. 1987, 51, 913. DOI: 10.1063/1.98799.
- Wang, Q.; Oswald, I. W. H.; Perez, M. R.; Jia, H.; Shahub, A. A.; Qiao, Q.; Gnade, B. E.; Omary, M. A. Doping-Free Organic Light-Emitting Diodes with Very High Power Efficiency, Simple Device Structure, and Superior Spectral Performance. Adv. Funct. Mater. 2014, 24, 4746–4752. DOI: 10.1002/adfm.v24.30.
- Fang, Q.-R.; Makal, T. A.; Young, M. D.; Zhou, H.-C. Recent Advances in the Study of Mesoporous Metal-Organic Frameworks. Comments Inorg. Chem. 2010, 30, 165–195. DOI: 10.1080/02603594.2010.520254.
- Nijem, N.; Chabal, Y. J. Adsorbate Interactions in Metal Organic Frameworks Studied by Vibrational Spectroscopy. Comments Inorg. Chem. 2014, 34, 78–102. DOI: 10.1080/02603594.2014.940418.
- Gao, W.-Y.; Ma, S. Beyond Custom Design of Organic Ligands: An Integrative Strategy for Metal-Organic Frameworks Design. Comments Inorg. Chem. 2014, 34, 125–141. DOI: 10.1080/02603594.2014.956926.
- Banerjee, D.; Liu, J.; Thallapally, P. K. Separation of C2 Hydrocarbons by Porous Materials: Metal Organic Frameworks as Platform. Comments Inorg. Chem. 2015, 35, 18–38. DOI: 10.1080/02603594.2014.976704.
- Senthil Raja, D.; Liu, W.-L.; Huang, H.-Y.; Lin, C.-H. Immobilization of Protein on Nanoporous Metal-Organic Framework Materials. Comments Inorg. Chem. 2015, 35, 331–349. DOI: 10.1080/02603594.2015.1059827.