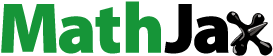
1. Introduction
Hexametaphosphate (HMP) is an inorganic condensed phosphate, which has been used in a wide variety of industries for nearly a century, originally patented to deflocculate clay and soften hard water.[Citation1,Citation2] Today, HMP is still used in the minerals processing industry as a dispersant to improve separation.[Citation3–5] It is also used in the food industry, as additive E452i, to improve the stability of whey protein drinks,[Citation6,Citation7] prevent efflorescence in fermented sausages,[Citation8] and as an emulsifying salt in processed cheese.[Citation9] HMP is used in some toothpastes, to prevent caries and reduce the amount of fluorine required.[Citation10,Citation11] HMP is particularly useful in these applications due to its ability to bind to surfaces to provide steric and electrostatic repulsion, and to form strong soluble complexes with multivalent cations. Indeed, studies have identified HMP as the most potent of the condensed phosphates for cation binding, however, the exact reason for this is somewhat unclear because of confusion about the structure of HMP.[Citation12,Citation13] While important for all of these industries, understanding the structure of HMP is of particular importance for its emerging biomedical applications. These include biomaterial formulations such as cements,[Citation14] materials for controlled antibiotic release[Citation15,Citation16] and nanoparticulate drug delivery vehicles,[Citation17] but also using HMP as the active therapeutic to combat pathological calcifications, such as heterotopic ossification[Citation18] and kidney stones.[Citation19] It is particularly important to know the precise structure of HMP for clinical applications in order to reliably and reproducibly predict efficacy and ADME (absorption, distribution, metabolism and excretion) properties, to overcome regulatory hurdles.
There are currently two popular ideas for the structure of HMP. Some studies state that HMP is a 12 membered ring (),[Citation8,Citation14,Citation17,Citation20] while others claim HMP is a linear polyphosphate ().[Citation3,Citation9,Citation21] It has also been suggested that both linear and cyclic products are available, but are both referred to as HMP commercially.[Citation11] This confusion has prevented some attempts to compare between studies, because it is not clear whether the HMP used in each is the same compound.[Citation10] Commercial suppliers of HMP often depict different molecules for the product; some show a ring structure with six phosphate units (),[Citation22] others may simply give the formula (NaPO3)n, which could suggest a ring or a very long-chain polyphosphate, however this gives no indication of length.[Citation23,Citation24] Further, commercial synonyms given for HMP include polyphosphate, suggesting the structure in , and Graham’s salt, which is a theoretical linear polyphosphate of infinite length.[Citation25] This confusion around the structure of HMP, both in the literature and from commercial suppliers, necessitates the need to study and better understand the structure of this useful chemical.
Figure 1. Suggested structures of HMP. A) A cyclic structure consisting of 6 phosphate units. B) A linear structure with a mix of chain lengths, n.
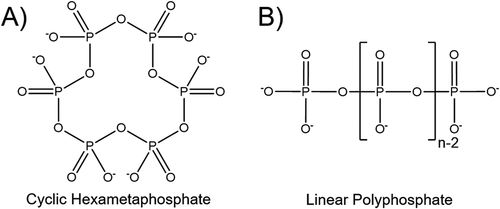
In this study, titration and 31P-NMR spectroscopy were used to study the structure of some commercial condensed phosphates. Two brands of HMP, Sigma and Fisher, as well as a Graham’s salt, were studied and compared to tripolyphosphate (TPP), a 3 membered linear polyphosphate, and trimetaphosphate (TMP), a cyclic consisting of 3 phosphate units. The average chain length, chain length distribution and cyclic content of the condensed phosphates were calculated, in order to give a clear picture of the chemical composition of these commercially important products.
2. Experimental
2.1. Materials
Deionized water (Milli-Q, Millipore) was used as solvent in the titration and chromatography experiments, and deuterium oxide (99.9 atom % D, Sigma) for 31P-NMR spectroscopy. Two brands of sodium hexametaphosphate (general purpose grade, Fisher, lot 1885988, and crystalline, 96%, Sigma, lot MKBR2816V), Graham’s salt (sodium polyphosphate, Emplura, lot 1.06529.1000), sodium tripolyphosphate (technical grade, Sigma. lot 12421JIV), and sodium trimetaphosphate (≥95%, Sigma, lot MKBQ7691V) were studied and compared.
2.2. Titration
0.5 g of the condensed phosphate was dissolved in 450 mL of deionized water, the pH was reduced to 2.5 with 1 M HCl (2–5 mL), and then the total volume made up to 500 mL. A 200 mL portion was immediately back-titrated, by adding 0.1 M NaOH (aq), in 100 µL increments, and the pH was recorded at each addition, using a pH probe (Metler Toledo). This was done within 1 hour, to minimize any hydrolysis. The remaining acidified polyphosphate solution was boiled under reflux for at least 6 hours, then left to cool for 12 hours, to fully hydrolyze the condensed phosphate into orthophosphate. A 200 mL portion of this solution was then back-titrated as above.
To explore the chain length distribution of HMP, 4 g of HMP (Fisher) was dissolved in 40 mL of deionized water, then 5 mL of acetone was added to induce precipitation. The mixture was stirred for 5 minutes to allow equilibration between the phases, then centrifuged at 4700 rpm for 10 minutes. The supernatant fluid was removed, and the precipitate phase was stored at −20°C, for a maximum of 7 days, until use, where it was acidified, diluted to 500 mL with deionized water, and titrated as above. Further acetone was then added to the supernatant phase to again cause precipitation, and this procedure was repeated with gradually increasing volumes of acetone ().
2.3. 31P-nuclear magnetic resonance spectroscopy
The phosphate species of interest was dissolved in deuterium oxide at approximately 10 mg per 650 μL. The pH was not modified, for simplicity and to allow the products to be studied as supplied. This does not alter the shape nor relative intensity of the peaks, and thus does not affect peak identification or numerical analysis, however both concentration and pH may have a small effect on the absolute value of the chemical shifts. All 31P-NMR spectra were recorded on a Bruker Avance-NEO-400 spectrometer in D2O at 298 K (400 MHz proton frequency and 162 MHz 31P frequency). Each spectrum was acquired using an acquisition time of 1.04 s, a relaxation delay of 2 s and 200 scans. H3PO4 (85%) was used as an external reference for spectrum calibration and the chemical shifts are reported on the δ scale in parts-per-million (ppm). 31P-NMR experiments were carried out without 1H decoupling. Analysis of the obtained spectra was carried out using MestReNova software. Peak assignment was carried out with reference to the literature.[Citation26,Citation27]
3. Results
3.1. Titration
A portion of an acidified solution of each condensed phosphate was titrated with NaOH, in order to find the equivalence points and pKa values. An equal volume, boiled under reflux to hydrolyze the condensed phosphate completely to orthophosphate, was then titrated in the same way. This procedure produced two curves; one for the condensed phosphate (), and one for the corresponding orthophosphate (). Each curve has two equivalence points (, arrows), between which every mole of OH− added removes a weakly dissociated proton from a phosphate molecule. Because both the polyphosphate and orthophosphate solutions, having the same volume and concentration, contain the same total number of phosphate units, the constant of proportionality between phosphate molecules and volume of base added is the same for both. Further, as the polyphosphate molecule has two weakly dissociated protons (), and orthophosphate has one (), the amount of base required to transition between the two equivalence points can be used to calculate the average chain length, n, of the polyphosphate:
Figure 2. Representative titration curves for A) a polyphosphate and B) orthophosphate, following hydrolysis, showing the degree of proton dissociation at each equivalence point, and the volumes of base required to calculate the average chain length, fo and fp. Structures of C) a linear polyphosphate, D) orthophosphate and E) a cyclic phosphate, showing the strongly (red) and weakly (blue) dissociated protons.
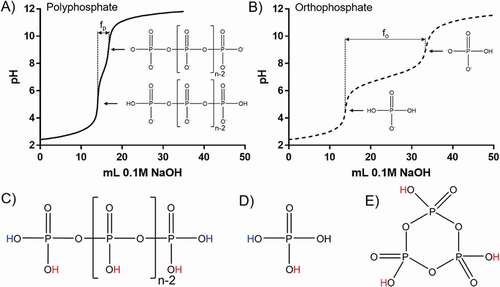
Figure 3. Condensed phosphate titration. A) Representative titration curves for TMP, TPP, HMP (Sigma and Fisher), and Graham’s salt (solid lines), and their associated orthophosphate curves following hydrolysis (h, dashed lines). B) pKa and average chain length data obtained from the condensed phosphate titration. C) Fractionation series of HMP (Fisher), with the size and average chain length of each fraction. D) Chain length distribution of HMP (Fisher), where each point is the average chain length of that fraction plotted against cumulative phosphate. B), C) and D) show mean ± SD (n = 3).
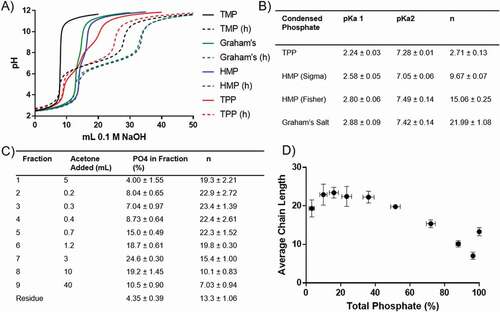
Where fo and fp are the volumes of NaOH required to move between the two equivalence points for the orthophosphate and the polyphosphate, respectively. In the case of a cyclic condensed phosphate there will be a single equivalence point, as cyclics have no end groups and therefore possess only strongly dissociated protons ().
In all cases, the first equivalence points were the same for both the condensed phosphate (, solid lines) and the corresponding orthophosphate (, dashed lines). TMP displayed a single equivalence point, suggesting all of the phosphate groups possess a single, equivalent proton. A chain length therefore cannot be quantified, and suggests either an infinitely long chain or, as expected, a cyclic (). All of the other condensed phosphates studied displayed two equivalence points, suggesting the possession of both strongly and weakly associated protons (), and allowing an average chain length to be calculated (). TPP was the shortest condensed phosphate studied (n = 2.71), followed by the two HMP products (n = 9.67 and 15.06 for the Sigma and Fisher products, respectively), and Graham’s salt displayed the greatest average chain length (n = 21.99).
To study the distribution of chain lengths in HMP (Fisher), the solution was graduated with acetone to yield a series of less complex fractions, which were then titrated as before (). This showed that over 60% of the total phosphate was present in chains of between 19 and 24 PO4 tetrahedra in length, while only 4% was present in chains with fewer than 7 units. The residual fraction appeared to have a greater average chain length than those immediately preceding it (). This is likely due to the presence of rings, which are concentrated into this fraction. The cyclic content can thus be estimated by assuming that the residual fraction has the same average chain length as the previous fraction, but that the fo value is inflated by the presence of rings. This ‘excess’ fo value, fo,ex can be calculated by:
Where fo,res is the measured fo for the residual fraction, n9 is the average chain length of the previous fraction, in this case fraction 9, and fp,res is the measured fp of the residual fraction. Using this method, 1.65 ± 0.16% of the total phosphate was found to be in the form of ring structures.
4. 31P-NMR spectroscopy
31P-NMR spectroscopy was used as a complementary technique to examine the structure of the condensed phosphates. TMP displayed a single, sharp peak, suggesting that all of the phosphates were in the same environment () as would be expected for a cyclic (). TPP displayed four clear peaks. The 1:1 doublet at −6.0 ppm is associated with the end phosphate groups (e), and occurs because both end groups are equivalent and both couple with the middle phosphate. The peak at −20.8 ppm is associated with the middle phosphate groups (m), and forms a 1:2:1 triplet because the central phosphate couples with both, equivalent, end phosphates. Further, peaks at 2.6 ppm and −6.9 ppm can be attributed to orthophosphate (o) and pyrophosphate (p), respectively (). For the two HMP products and Graham’s salt, the spectra were more complex (). Peaks associated with orthophosphate and pyrophosphate were visible in the NMR spectra of the two HMPs and a peak for TMP (t) was present in all three. Additionally, there were broad peaks for both the end groups and middle groups of the longer linear chains in these spectra. By integrating the relative area under these peaks, the percentage of phosphate present as orthophosphate, pyrophosphate and TMP could be calculated. Further, the ratio of end to middle groups could be established, and the average chain length calculated (). This calculation was carried out both including pyrophosphate as end groups, as in the titration, and excluding them. This showed that the fractions of both orthophosphate and pyrophosphate appear to decrease with average chain length. The average chain lengths, calculated including the pyrophosphate end groups, were very similar to those calculated from the titration data (NMR vs titration: 2.7 vs 2.7 for TPP, 10.7 vs 9.7 for Sigma HMP, 15.7 vs 15.1 for Fisher HMP and 21.9 vs 22.0 for Graham’s salt). However, excluding the pyrophosphate gives a higher average chain length, particularly in the Sigma HMP product.
Figure 4. 31P-NMR spectra (162 MHz, D2O) for A) TMP, B) TPP, C) HMP (Sigma), D) HMP (Fisher), and E) Graham’s salt. Peak identifications: o: orthophosphate, p: pyrophosphate, t: trimetaphosphate, e: end groups, m: middle groups. F) Summary of the orthophosphate, pyrophosphate and TMP content of each condensed phosphate, and the average chain length calculated from the 31P-NMR data, both including and excluding pyrophosphate from the calculation, where it is clearly identifiable.
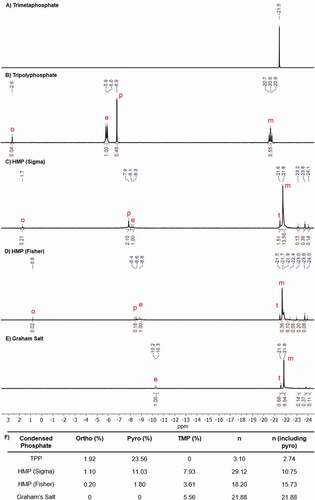
5. Discussion
HMP is a useful compound in a wide range of industries, however confusion about its structure prevents useful comparisons between studies, limits increased understanding of mechanisms of action, and inhibits translation to clinical use. Both titration and 31P-NMR spectroscopy were found to be clear and simple ways of determining the structure of condensed phosphates. 31P-NMR spectroscopy is advantageous because it is faster, and it is possible to detect individual phosphate species, while titration requires no specialist equipment. These techniques were validated using TPP and TMP. The titration curve of TPP showed two clear equivalence points, and an average chain length of 2.7 was calculated, close to its theoretical value of 3. 31P-NMR spectroscopy revealed that this was due to the presence of some orthophosphate and pyrophosphate, skewing the average. Calculating the average from the 31P-NMR spectra, and excluding the pyrophosphate as end groups, gave the average chain length of TPP as 3.1. When titrated, TMP displayed a single equivalence point, titrating like a strong monoprotic acid. This is because, as a ring structure, TMP has no end groups, and hence no weakly dissociated protons. Similarly, the 31P-NMR spectra of TMP displayed only a single sharp peak, as all of the phosphates experience the same chemical environment. This validation allows both titration and 31P-NMR spectroscopy to be recommended for the determination of the structure of an unknown inorganic condensed phosphate, for example a different HMP product to those investigated here.
It is clear from both titration and 31P-NMR spectroscopy that the two HMP products and Graham’s salt studied here contain a majority of linear species. This is most obvious when comparing them to the cyclic TMP. The titration curves for HMP display two clear equivalence points, suggesting a linear polyphosphate with both strongly and weakly dissociated protons, while the cyclic TMP, with only strongly dissociated protons, has one. The 31P-NMR spectra for HMP contain several peaks, including those indicative of middle and end groups in a polyphosphate, while the spectrum for TMP shows a single peak, corresponding to the single environment experienced by all phosphates in the ring structure. It is also clear that, with the exception of TMP, all of the polyphosphates contain a range of chain lengths. The fractionation of HMP showed that more than half of the phosphates were in chains greater than 20 units in length, however the average is skewed by the presence of smaller chains. This is also evidenced by the presence of orthophosphate and pyrophosphate in the 31P-NMR spectra of the two HMPs. As expected, the proportion of both of these species decreased as the average chain length of the polyphosphate increased.[Citation25]
However, despite being majority linear, both HMPs and Graham’s salt do appear to contain some cyclic species. TMP can be identified in the 31P-NMR spectra of all three. Additionally, the three small peaks between −23 and −24 ppm may correspond to larger rings, potentially including the 12-membered cyclic HMP molecule[Citation26] though this cannot be said definitively. In any case, as expected, TMP was the most abundant cyclic in these products.[Citation28] The cyclic content for the Fisher HMP was quantified from both the titration and 31P-NMR spectroscopy results, giving values of 1.65 and 3.61% respectively. The value from the 31P-NMR spectroscopy is likely more accurate because the approximation for the fractioned HMP titration assumes that the linear polyphosphates in the residual fraction would have the same average chain length as the previous fraction (n9 in EquationEquation 2(2)
(2) ), while it is probable that the linear phosphates remaining in the residual fraction will in fact be shorter.
An important consequence of the structure of HMP is its effectiveness in practical application. The commercial and research uses of HMP stem from two properties; its ability to chelate polyvalent cations, such as calcium and magnesium, and its affinity to bind to, and cause steric and electrostatic repulsion between, surfaces, for example those of mineral particles. Linear polyphosphates are more effective at both of these functions than cyclics,[Citation29–31] and longer chains appear to be more effective than short ones.[Citation12,Citation32] It is therefore desirable, given its applications, that HMP is predominantly linear. Further, while long chain lengths are effective, they also slow the rate of dissolution, and so a compromise is required for practical application. A patent for a method of HMP manufacture states the desired chain length to achieve this compromise as 14[Citation33]; this is similar to the chain lengths found here for the commercial HMP products.
This study has also shown that the product data provided by chemical manufacturers can be inadequate or even misleading, most obviously exemplified here by depicting HMP as a cyclic product. However, it raises the larger issue that chemical suppliers need to provide clearer, more thorough information on their products. In this case, given the confusion around the names HMP and Graham’s salt, it is suggested that use of these archaic terms ceases, and that all such products are simply named ‘sodium polyphosphate,’ with at least the average chain length specified. However, until then, it is strongly recommended that practitioners characterize products themselves, using techniques such as titration and 31P-NMR.
While understanding the structure of HMP is an important first step for its translation from research into clinical applications, the results found herein do pose some challenges for this process. Firstly, as a mixture of chain lengths and rings, HMP may possess a broad ADME profile; long chains may be absorbed and distributed more slowly than shorter ones, and rings may break down more slowly than linear chains.[Citation34,Citation35] Further, being a mixture may reduce the efficacy, or make it more difficult to predict. Orthophosphate, pyrophosphate, TPP and TMP are all poor chelators compared to longer chains,[Citation12,Citation13] and this may limit total effectiveness as, for example, these species make up more than 20% of the Sigma HMP product. Finally, the broad differences in composition between the various products exemplify how difficult it is to precisely define ‘HMP’ as a standard chemical, which is an important factor to overcome regulatory hurdles. In order to combat these issues, it may be necessary to produce a new linear polyphosphate product, with a tightly controlled chain length which contains very limited amounts of short chain and ring structures. This may then allow easier translation of the useful properties of HMP into the clinic.
6. Conclusions
HMP and Graham’s salt appear to contain a majority of linear polyphosphates with a range of chain lengths, and only a small proportion of rings, the majority of which are TMP. While the 12 membered ring may exist in very small amounts, the commonly reported cyclic HMP structure is not representative of the product. If the structure of a condensed inorganic phosphate product is in doubt, both titration and 31P-NMR spectroscopy are simple and effective techniques that can be used to ascertain the structure and average chain length. These findings may inform rational use of polyphosphates in research and industry, as well as improve understanding of their functions and mechanisms of action. However, the variability between different HMP products and the mixture of structures within each product may prevent the useful properties of HMP from being translated into clinical use, and a more monodisperse polyphosphate product may be required.
Additional information
Funding
References
- Feldenheimer, W. Treatment of Clay. US1438588A, 1922.
- Hall, R. E. Water Softening and Washing. 1956515, 1934.
- Choi, I. K.; Wen, W. W.; Smith, R. W. The Effect of a Long Chain Phosphate on the Adsorption of Collectors on Kaolinite. Miner. Eng. 1993, 6(11), 1191–1197. DOI: https://doi.org/10.1016/0892-6875(93)90096-6.
- Rao, R. R.; Kannan, T. S. Dispersion and Slip Casting of Hydroxyapatite. J. Am. Ceram. Soc. 2001, 84(8), 1710–1716. DOI: https://doi.org/10.1111/j.1151-2916.2001.tb00903.x.
- Li, Y.; Xia, W.; Pan, L.; Tian, F.; Peng, Y.; Xie, G.; Li, Y. Flotation of Low-rank Coal Using Sodium Oleate and Sodium Hexametaphosphate. J. Clean. Prod. 2020, 261, 121216. DOI: https://doi.org/10.1016/j.jclepro.2020.121216.
- Rasouli, M.; Abbasi, S.; Azarikia, F.; Ettelaie, R. On the Heat Stability of Whey Protein: Effect of Sodium Hexametaphosphate. Int. J. Dairy Technol. 2020, 73(1), 46–56. DOI: https://doi.org/10.1111/1471-0307.12626.
- Pandalaneni, K.; Amamcharla, J. K.; Marella, C.; Metzger, L. E. Influence of Milk Protein Concentrates with Modified Calcium Content on Enteral Dairy Beverage Formulations: Physicochemical Properties. J. Dairy Sci. 2018, 101(11), 9714–9724. DOI: https://doi.org/10.3168/jds.2018-14781.
- Walz, F. H.; Gibis, M.; Fritz, M.; Herrmann, K.; Hinrichs, J.; Weiss, J. Optimum Hexametaphosphate Concentration to Inhibit Efflorescence Formation in Dry Fermented Sausages. Meat Sci. 2018, 139, 35–43. DOI: https://doi.org/10.1016/j.meatsci.2018.01.008.
- Shirashoji, N.; Jaeggi, J. J.; Lucey, J. A. Effect of Sodium Hexametaphosphate Concentration and Cooking Time on the Physicochemical Properties of Pasteurized Process Cheese. J. Dairy Sci. 2010, 93(7), 2827–2837. DOI: https://doi.org/10.3168/jds.2009-2960.
- Van Dijk, J. W. E.; Borggreven, J. M. P. M.; Driessens, F. C. M. The Effect of Some Phosphates and a Phosphonate on the Electrochemical Properties of Bovine Enamel. Arch. Oral Biol. 1980, 25(8–9), 591–595. DOI: https://doi.org/10.1016/0003-9969(80)90072-2.
- Da Camara, D. M.; Miyasaki, M. L.; Danelon, M.; Sassaki, K. T.; Delbem, A. C. B. Effect of Low-fluoride Toothpastes Combined with Hexametaphosphate on in Vitro Enamel Demineralization. J. Dent. 2014, 42(3), 256–262. DOI: https://doi.org/10.1016/j.jdent.2013.12.002.
- Gosselin, R. E.; Coghlan, E. R. The Stability of Complexes Between Calcium and Orthophosphate, Polymeric Phosphate, and Phytate. Arch. Biochem. Biophys. 1953, 45(2), 301–311. DOI:https://doi.org/10.1016/S0003-9861(53)80007-X.
- Eisenstein, N.; Williams, R.; Cox, S.; Stapley, S.; Grover, L. Enzymatically Regulated Demineralisation of Pathological Bone using Sodium Hexametaphosphate. J. Mater. Chem. B. 2016, 4(21), 3815–3822. DOI:https://doi.org/10.1039/C6TB00461J.
- Hesaraki, S.; Zamanian, A.; Moztarzadeh, F. Effect of Adding Sodium Hexametaphosphate Liquefier on Basic Properties of Calcium Phosphate Cements. J. Biomed. Mater. Res. Part A. 2009, 88(2), 314–321. DOI:https://doi.org/10.1002/jbm.a.31836.
- Duckworth, P. F.; Maddocks, S. E.; Rahatekar, S. S.; Barbour, M. E. Alginate films augmented with chlorhexidine hexametaphosphate particles provide sustained antimicrobial properties for application in wound care. J. Mater. Sci. Mater. Med. 2020, 31(3), 1–9. DOI:https://doi.org/10.1007/s10856-020-06370-0.
- Barbour, M. E.; Maddocks, S. E.; Grady, H. J.; Roper, J. A.; Bass, M. D.; Collins, A. M.; Dommett, R. M.; Saunders, M. Chlorhexidine Hexametaphosphate as a Wound Care Material Coating: Antimicrobial Efficacy, Toxicity and Effect on Healing. Nanomedicine. 2016, 11(16), 2049–2057. DOI: https://doi.org/10.2217/nnm-2016-0084.
- Sang, Z.; Qian, J.; Han, J.; Deng, X.; Shen, J.; Li, G.; Xie, Y. Comparison of Three Water-soluble Polyphosphate Tripolyphosphate, Phytic Acid, and Sodium Hexametaphosphate as Crosslinking Agents in Chitosan Nanoparticle Formulation. Carbohydr. Polym. 2020, 230, 115577. DOI: https://doi.org/10.1016/j.carbpol.2019.115577.
- Robinson, T. E.; Eisenstein, N. M.; Cox, S. C.; Moakes, R. J. A.; Thompson, A. M.; Ahmed, Z.; Hughes, E. A. B.; Hill, L. J.; Stapley, S. A.; Grover, L. M. Local Injection of a Hexametaphosphate Formulation Reduces Heterotopic Ossification in Vivo. Mater. Today Bio. 2020, 7, 100059. DOI: https://doi.org/10.1016/j.mtbio.2020.100059.
- Robinson, T. E.; Hughes, E. A. B.; Wiseman, O. J.; Stapley, S. A.; Cox, S. C.; Grover, L. M. Hexametaphosphate as a Potential Therapy for the Dissolution and Prevention of Kidney Stones. J. Mater. Chem. B. 2020, 8(24), 5215–5224. DOI: https://doi.org/10.1039/d0tb00343c.
- Zhang, T.; Vandeperre, L. J.; Cheeseman, C. R. Formation of Magnesium Silicate Hydrate (M-S-H) Cement Pastes Using Sodium Hexametaphosphate. Cem. Concr. Res. 2014, 65, 8–14. DOI: https://doi.org/10.1016/j.cemconres.2014.07.001.
- Han, Y.; Liu, W.; Zhou, J.; Chen, J. Interactions between Kaolinite Al OH Surface and Sodium Hexametaphosphate. Appl. Surf. Sci. 2016, 387, 759–765. DOI: https://doi.org/10.1016/j.apsusc.2016.07.002.
- Fisher Scientific, Sodium Hexametaphosphate, Extra Pure, SLR, Powder, Mainly (Napo3)6, Fisher Chemical 500g, Plastic Powder Jar Sodium Hexametaphosphate, Extra Pure, SLR, Powder, Mainly (Napo3)6, Fisher Chemical. Fisher Scientific. https://www.fishersci.co.uk/shop/products/sodium-hexametaphosphate-extra-pure-slr-powder-mainly-napo3-6-2/10204640. (Accessed May 7, 2020).
- Sigma Aldrich, Sodium Hexametaphosphate 65-70% P2O5 Basis. https://www.sigmaaldrich.com/catalog/product/aldrich/71600?lang=en®ion=GB. (Accessed Apr 24, 2020).
- Sigma Aldrich, Sodium Hexametaphosphate Crystalline, +200 Mesh, 96%. https://www.sigmaaldrich.com/catalog/product/aldrich/305553?lang=en®ion=GB. (Accessed Apr 24, 2020).
- Van Wazer, J. R. V. Structure and Properties of the Condensed Phosphates. II. A Theory of the Molecular Structure of Sodium Phosphate Glasses 1. J. Am. Chem. Soc. 1950, 72(2), 644–647. DOI: https://doi.org/10.1021/ja01158a002.
- Greenfield, S.; Clift, M. Analytical Chemistry of the Condensed Phosphates, First ed.; Oxford, Pergamon Press, 1975.
- Christ, J. J.; Willbold, S.; Blank, L. M. Methods for the Analysis of Polyphosphate in the Life Sciences. Anal. Chem. 2020, 92(6), 4167–4176. DOI: https://doi.org/10.1021/acs.analchem.9b05144.
- Callis, C. F.; Van Wazer, J. R.; Arvan, P. G. The Inorganic Phosphates as Polyelectrolytes. Chem. Rev. 1954, 54(5), 777–796. DOI: https://doi.org/10.1021/cr60171a002.
- Cini, N.; Ball, V. Polyphosphates as Inorganic Polyelectrolytes Interacting with Oppositely Charged Ions, Polymers and Deposited on Surfaces: Fundamentals and Applications. Adv. Colloid Interface Sci. 2014, 209, 84–97. DOI: https://doi.org/10.1016/j.cis.2014.01.011.
- Rashchi, F.; Finch, J. A. Polyphosphates: A Review Their Chemistry and Application with Particular Reference to Mineral Processing. Miner. Eng. 2000, 13(10), 1019–1035. DOI: https://doi.org/10.1016/S0892-6875(00)00087-X.
- Miyajima, T.; Onaka, T.; Ohashi, S. Gel Chromatographic Study on the Interactions of Long-Chain Polyphosphate Anions with Magnesium Ions. Phosphorous Chem. 1981, 78, 377–380.
- Van Wazer, J. R.; Campanella, D. A. Structure and Properties of the Condensed Phosphates. IV. Complex Ion Formation in Polyphosphate Solutions. J. Am. Chem. Soc. 1950, 72(2), 655–663. DOI: https://doi.org/10.1021/ja01158a004.
- Buckholtz, H. E. Production of Hexametaphosphates from Other Phosphates. US4997638A, 1990.
- Crowther, J.; Westman, A. E. R. The Hydrolysis of the Condensed Phosphates: Iii. Sodium Tetrametaphosphate and Sodium Tetraphosphate. Can. J. Chem. 1956, 34(7), 969–981. DOI: https://doi.org/10.1139/v56-129.
- Watanabe, M.; Sato, S.; Saito, H. The Mechanism of the Hydrolysis of Condensed Phosphates. II. The Mechanism of the Degradation of Long-chain Polyphosphates. Bull. Chem. Soc. Jpn. 1975, 48(3), 896–898. DOI: https://doi.org/10.1246/bcsj.48.896.