Abstract
Validation of large amounts of digital hydrological data and eventual exchange of data between various organisations can benefit from the development of a structured hydrological feature coding system. In this article we describe the development of such a system and present its implementation in the CCM2 structured hydrological feature data set, covering Europe to the Urals and including Turkey. We discuss the coding of river basins, catchments, lakes and rivers. The proposed coding system is largely inspired by the work of Otto Pfafstetter with additions for coding oceans, seas, islands and lakes. Furthermore the coding system can be transferred to features that geometrically intersect the coded hydrological features and we propose some rules on how to establish this transfer.
Citation de Jager, A. L. & Vogt, J. V. (2010) Development and demonstration of a structured hydrological feature coding system for Europe. Hydrol. Sci. J. 55(5), 661–675.
Résumé
La validation de grandes quantités de données hydrologiques numériques et l'éventuel échange de données entre diverses organisations peut bénéficier du développement d'un systéme de codification des entités hydrologiques structurées. Dans cet article, nous décrivons le développement d'un tel systéme ainsi que son implémentation avec un jeu de données issues du projet CCM2, qui couvrent l'Europe jusqu'à l'Oural en incluant la Turquie. Nous discutons de la codification des bassins hydrologiques, des lacs et des riviéres. Le systéme de codification proposé est largement inspiré par le travail d'Otto Pfafstetter auquel nous avons ajouté une codification pour les océans, les mers, les îles et les lacs. De plus, le système de codification peut être utilisé pour des objets (entités) qui recoupent de manière géométrique les entités hydrologiques codifiées et nous proposons quelques régles afin de gérer la codification de ces nouveaux objets.
INTRODUCTION
In compliance with legal obligations under the European Water Framework Directive (WFD, 2000/60/EC), 29 European countries provided digital data sets containing drainage basins, rivers and lakes to the European Commission during 2004. This extensive collection of European hydrological data sets demonstrated that, in most cases, the countries did not provide a consistent coding system. The uploaded data further demonstrate that there are few initiatives to define hydro-geographic objects in a consistent manner; for example with regard to geometric descriptions, topographic naming and delimitation (e.g. where does a river start and end?). The need to collaborate across national borders and to have a common hydrological feature coding system is generally acknowledged, but requires a method which is feasible to implement, taking into account the large investments already made in developing the various data sets.
Under the Common Implementation Strategy (CIS) for the WFD, a working group of Member State experts developed a GIS Guidance Document (EC, Citation2003), containing detailed descriptions of the content and format of the different hydrographic data layers to be provided as well as a recommendation for a hydrological feature coding system. The recommended coding system was based on the work of Otto Pfafstetter (Pfafstetter, Citation1989; Verdin & Verdin, Citation1999). In the 1950s he had already proposed a systematic and hierarchical coding of river branches within a river basin. Footnote 1 Pfafstetter codes are based on a combination of the longest flow path in a hydrological system and the area drained by its tributaries. It can be replicated from the level of an entire river basin down to the level of the smallest river reach. As a consequence, this system allows a user to understand the relative topological position of any coded hydrographic feature within the entire system, solely based on its hydrographic feature code. In addition, complex topological analyses (e.g. what is upstream or downstream of a given feature) can be implemented through a relatively simple analysis of the codes, without the need for graphical display.
While Pfafstetter codes provide for a unique coding of river stretches within one river basin (Arge et al., Citation2006), in this paper we propose an extension of this system allowing for a systematic and structured coding of river basins and their catchments, thus providing a unique identifier for any drained hydrological object across the entire European continent. Such systems at national level were described by Brilly & Vidmar (Citation1995), giving clear examples of how to encode catchments. In the USA, an almost continental system was developed based on a simple “northeast–southwest” numeric ordering (FGDC, Citation2003). In total 19 regions were defined for the territory of the continental USA. Each region consists of a large river basin combined with a selection of small coastal ones and falling within the territory of the USA. In contrast to North America, Europe is characterized by a large number of inner seas that divide peninsulas and the river basins lying on them. Furthermore, the European treaties regarding water quality monitoring are signed by Member States and non-Member States of the European Union in changing alliances. Therefore, we need to define the coding system based on hydrological and topographical characteristics only.
For Europe we thus choose to define a system based on the delineations of the seas and oceans. Since many treaties and monitoring plans concern one inner sea only, a coding system based on inner seas allows a quick selection and ordering of data. In order to develop this coding system we first describe a simple hierarchical system to classify the world's oceans and seas. Subsequently we propose a coding system for the river basins draining into these seas (and oceans) from the continental landmasses and from islands. Since Europe is surrounded by many islands we also propose a codification system for these landmasses disconnected from the continent.
After having uniquely coded every river basin, we then use the Pfafstetter coding to uniquely and hierarchically identify river stretches, catchments and other hydrological features, such as lakes and marshes positioned within the catchments. Each catchment receives the code of the river stretch by which it is drained.
The proposed coding system allows for the selection and grouping of data related to water courses (e.g. quantity and/or quality) as well as their catchments (e.g. climate, topography, land cover, soil types) based on the code alone. It is, for example, possible to easily identify all upper catchments draining to any given point along the river network or to identify all river stretches downstream of a given point. The former allows, for example, identification and analysis of possible sources of pollution or analysis of the source areas of excessive or reduced rainfall, while the latter allows identification of all downstream river stretches affected by a pollution event or by a flood. The established link to the seas, oceans, and lakes further allows identifying and grouping of catchments influencing these entities. As such Pfafstetter coding and the extension we proposed for commencement coding of the river basins can be used in a number of applications in which the topography and natural drainage of the landscape is important. Such grouping and display of data is needed by authorities having to enforce environmental legislation that are currently often limited to grouping of data using administrative boundaries only (e.g. Scharf & de Jager, Citation2006; Nõges & de Jager, Citation2009). The proposed coding system has been implemented on the CCM River and Catchment Database for Europe, Footnote 2 version 2 (here referred to as CCM2).
Digital hydrographical data sets exist at various scales. While national mapping agencies provide data sets for their territory, at European and global scales, data sets like Teleatlas, the Digital Chart of the World (DCW) or the Vector Smart Map (VMap), contain hydrographic layers with global coverage. These data sets, however, are either available under restrictive license agreements only or of limited detail. In addition, they often lack a topological network and the important link between the river network and the related drainage areas (catchments). In order to overcome these limitations, and especially in order to provide a topologically consistent network of fully connected rivers and their catchments, we developed CCM2. This database provides a systematic, fully integrated and hierarchical system of rivers and related catchments for the entire European continent. It has been derived from digital elevation data and ancillary information using advanced algorithms based on mathematical morphology and including a set of hydrologically relevant attributes for rivers and catchments (Soille et al., Citation2003; Colombo et al., Citation2007; Vogt et al., Citation2007a,Citationb). Such a topological network is a basic requirement for the automatic generation of the Pfafstetter and commencement codes as described in this paper. Note that the Pfafstetter code generation also requires the size of the area drained by each river segment. CCM2 was derived from a digital elevation model at 100-m spatial resolution, resulting in a river network adequate for a mapping scale of at least 1:500 000. Detailed explanations of the methodology for deriving CCM2 and of the resulting database and its quality characteristics are provided in Vogt et al. (Citation2007a). Since CCM2 has been derived by a modelling exercise, it has limitations with respect to geometric accuracy and detail. However, the data have undergone several iterations of quality checks and currently present the most detailed and complete hydrographical data set covering the whole European continent.
HYDROGRAPHIC SYSTEMS (OCEANS, SEAS AND ENDORHEIC SYSTEMS)
Verdin & Verdin (Citation1999) proposed a codification on the Earth's river basins in which the codes are based on a set of topological rules similar to what we propose here. However they code the basins in a hierarchical system that starts with the continent on which the basin lies.
Europe, however, is a subcontinent marked by the presence of a large number of almost landlocked seas. In order to analyse the hydrological relations in such seas we think it is useful to start the coding with delineating the seas rather than the continent. The Mediterranean Basin, for example, can be regarded as a comprehensive ecological system into which the Ebro, the Danube and the Nile rivers drain and account for water quality. A hydrological feature coding system that reveals into which sea a river basin is draining and how this sea is connected to the open ocean provides a basis for enhanced and smart analysis of water related data. Therefore we propose to develop a limited hierarchical model in order to make these connections explicit in the hydrological feature coding system.
The geographical limits of seas and oceans are defined by the International Hydrographic Organi- zation (Citation1953). Initiatives to agree internationally on the names and delineations of seas date back to 1919. Amongst other reasons, they were defined in order to provide consistent names for use in logbooks. Consistent information sharing was an important driver to reach this international agreement, in which the limits of seas were defined using the location of light houses on various capes along the coast lines.
In the following we propose a coding for oceans, endorheic systems and seas for the European case.
Oceans
We propose to define a unique identifier of one letter to mark the worlds open oceans and the Mediterranean Sea. The latter is included in the class of oceans due to its size and important role for the European continent and northern Africa.
We propose to distinguish seven oceans: Arctic Ocean, Northern Atlantic Ocean, Southern Atlantic Ocean, the Indian Ocean, Northern Pacific Ocean, Southern Pacific Ocean and the Mediterranean Sea. In our coding system each ocean receives one letter as proposed in .
Table 1 Ocean codes
Note that in our implementation only Europe is considered. The ocean letter should be appended with a continental indication in case an intercontinental coding is proposed. For example, the letters “W” and “E” could allow distinguishing the American Atlantic coast (West) from the European Atlantic coast (East).
Endorheic systems
The surface of the Earth does not in all locations provide for surface water flow into an ocean. Earthquakes, glaciers, a perforated crust or lack of excess precipitation can shape landscapes in which surface water flow does not reach one of the seven oceans by the surface. Hydrographic systems that are not connected to an ocean are named endorheic systems.
In Europe, the largest endorheic basin is the Caspian Sea, a basin that drains a large part of Europe, amongst others through the Volga basin.
Endorheic systems that contain surface flow can be considered as equal to an ocean and therefore we propose a letter code to identify these systems. lists relevant endorheic systems in Europe, including Turkey and the Caucasus.
Table 2 Codes of endorheic systems
Seas
Most of the defined hydrographic systems can be subdivided into subsystems like seas, bays or straits. For grouping hydrographic features under European policies we propose a subdivision into 13 seas. This proposal could serve as a prototype for a global coding system, which can be made in a second step after experience is gained with the drawbacks and advantages of the proposal defined here.
The seas, which are sub-entities of the defined oceans, are coded with one number. The higher the number, the longer the distance to the open ocean. This numbering scheme follows the Pfafstetter logic where a code of 9 marks the source of the longest flow path and a code of 1 the outlet of a river system into a sea. In order to code the seas relevant to the European subcontinent we propose to distinguish 13 seas, as in .
Table 3 Numeric codes of seas around Europe and the oceans they belong to
In we provide a map of the defined seas as well as the land areas draining to these seas. For the limits of the seas on the European continent we used the locations referred to in the definitions of the International Hydrographic Organization (Citation1953). Note that the islands of Ireland, Great Britain and Sicily drain into more than one sea. All other islands drain fully towards one sea. South of the Caucasus and in Central Anatolia we find various endorheic systems. The boundaries of the drained land masses are derived from CCM2.
RIVER BASIN CODING
River basin coding following the Pfafstetter logic
In the proposed system, land drains to specified seas (or endorheic systems). As a consequence, river basins along the coast are ordered in a way to allow for a systematic coding of all river basins flowing into a specific sea.
The proposed ordering procedure is inspired by the Pfafstetter coding system. It implies that the four largest river basins (based on area drained) are coded with an even number and the river basins in-between (inter-basins) odd numbers. Within the groups of odd-numbered inter-basins the procedure is repeated iteratively up to five digits.
The resulting river basin numbering scheme is called the “commencement code.” The ocean code, sea code, commencement code and a Pfafstetter code at the river and catchment level together provide a unique code for hydrological features (river basins, catchments and river stretches) down to the spatial resolution of the mapped hydrographic system. We will later suggest how to add other hydrographic features such as lakes to this system.
Ordering river basins along the coast
In order to systematically code the river basins we defined the direction of the coastline as clockwise along a selected sea.
To generate this ordering automatically we selected all the lines in the CCM2 catchments along the coastline (i.e. having only one catchment and a so-called outside polygon as neighbours). Then, we start from a user-defined coastline point representing the beginning of the coast in a selected sea and follow the coastline up to a user defined enclosing point. All the points encountered along this line receive a sequence number from the start point up to the end point. Hence, provided that a connected coastline with from- and to-nodes is available, only a start and an end point of a sea are needed as input to this algorithm. Using a connected data set in which every river basin enters a sea one can achieve this ordering automatically. displays the result of such an automated ordering for the Black Sea.
River basin commencement coding
Once the coastline sorting is completed we can proceed with the commencement coding. The commencement coding is done by iteratively selecting the four largest river basins (draining the largest land areas) along the coastline of a given sea. These four basins are given numbers 2, 4, 6 and 8 clockwise along the coastline. This coding follows a Pfafstetter logic and results in the large river basins closest to the outlet of the sea being coded with 2 and 8, while the large river basins lying in-between receive codes of 4 and 6.
Subsequently the river basins in-between receive numbers 1, 3, 5, 7 and 9. Hence, and in the case of an enclosed sea, 1 and 9 are close to the connection of the sea with the ocean. In summary, one can easily sort the data along the coastline and understand from the code whether a system is large, medium or small and deduce its relative position along the coastline.
The result of the commencement coding for the Black Sea is presented in . The map reveals the notion of relative size of a river basin through the length of the code. The Black Sea has one large river basin to the south side, identified by code 8 (Kizil). Thus river basins east of this basin start with code 7 and west of it with code 9. These smaller basins are subdivided using a second number. Following this methodology and using the CCM2 data set for Europe, river basins can be identified uniquely using up to five digits.
Island coding
For the purpose of the sea definition we considered the islands of Ireland, Great Britain and Sicily as part of the continental coast (see above). This is necessary since these islands provide dividing points for seas along their coastline. Apart from these three islands, however, there are many other islands that do not define a sea border. For a unique hydrological feature coding we need to develop an identifier for these islands. Before proposing a coding method for islands, some characteristics of the complexity of this landscape feature are presented.
In the CCM2 system, which is based on a digital elevation model with a cell size of 100-m grid cell size, we find over 1200 islands with a surface area larger than 2.5 km2. In CCM2 islands are defined as pieces of land not connected to the continental landmass. This includes pieces of land connected only at one (corner) point in the grid system. In such cases a new record will be created, if grid cell data are converted to polygon type data. The result further depends on the quality of the land–sea boundary as defined in the grid system. For CCM2, a highly resolved water mask, derived to a large part from Landsat 7 data at 25-m spatial resolution, has been used for this purpose (de Jager et al., Citation2007).
This definition avoids the problem of an operator's decision as to whether a piece of land is an island or a peninsula. Based on this methodology, comparable statistics for the number and size of islands in a given sea, as well as the length of their coastline, can be generated easily. provides such statistics for large islands, defined as landmasses larger than 25 km2, and other islands, defined as landmasses of between 2.5 and 25 km2, around the European continent. In this overview an island is attributed to a certain sea depending on the shortest distance to the continental coast, including Great Britain, Ireland and Sicily.
Table 4 Island statistics per European sea region
highlights that islands can be classified according to their closest continental coast and the distance to that coast. The latter gives an indication on the isolation of the island with respect to the continent. We therefore propose an island coding in which the distance to the nearest coastline and the position of the closest point along the coastline are considered. This method allows listing of islands along a directed coastline. In we provide an example for the relatively simple case of the 13 large islands in the western Mediterranean Basin.
Table 5 Large islands in the Western Mediterranean Basin, ordered by their position along the coastline combined with their distance to this coastline
Note that in the example of , for Ibiza and Formentera, the closest distance to a coastline results in the same point along the coastline (i.e. 980 km from the starting point at Cape Trafalgar, measured clockwise towards the western tip of Sicily). In this case, Formentera gets sorted after Ibiza because it lies farther away from the continent than Ibiza. For example, the island of Asinara, lying north of Sardinia, lies closer to the northern Italian coast, than Sardinia itself. Therefore Asinara gets sorted first. The islands Sant'Antioco and San Pietro on the South of Sardinia lie closer to southern Italy and therefore are sorted after Sardinia.
provides another example of island sorting along the southern coast of the North Sea. The advantage of this sorting procedure is that one can quickly understand the relative position of an island both with respect to the coast as well with respect to the other islands.
Island coastline ordering
Once islands contain a sorting identifier, the river basins on the island receive a commencement code, following the technique described earlier. As for the continental coastline we need to define a coastline direction on the island. Since islands lie in one defined sea only, we specified a rule on where to start the coastline of an island. Arbitrarily we choose the most northwestern point on the island; once this point is (automatically) defined we follow the clockwise direction to define the coastline of the island. Thus the four largest river basins on the island will be coded 2, 4, 6 and 8, and the smaller basins (inter-basins) in-between with 1, 3, 5, 7 and 9. Using this methodology also on islands the user can understand the relative position of each basin along the coastline. In an example of such island coding is provided for the island of Sardinia. In Sardinian example, the coastline is ordered from Porto Torres in the Gulf of Asinara in the northwest. The numbers in the river basins represent the commencement code.
River coding
Once all the river basins have received a commencement code, river coding starts, following the Pfafstetter methodology. Pfafstetter codes are structured hydrological feature codes and represent a smart way to characterise the structure of a river network. Pfafstetter codes can, for example, be used to determine if discharge in a sub-catchment potentially impacts on a downstream channel. In principle, this can be achieved without the need for specific GIS analysis. As a consequence, the Pfafstetter system has been widely used (Britton, Citation2002).
Following the Pfafstetter logic, river basins and drainage networks are coded according to a numbering scheme based upon the topology of the drainage network and the size of the surface area drained. The numbering scheme is self-replicating, making it possible to provide identification numbers to the level of the smallest river reaches and catchments. For a given location it is possible to automatically identify all upstream catchments, all upstream river reaches, or all downstream river reaches solely from the code without the need to look at a graphical representation of the river network itself. This allows for fast queries on a database.
For assigning Pfafstetter codes, first the main stem of the river network of a given river basin has to be identified. This can, for example, be done automatically, based on the flow length. Once the main stem is identified, the area drained by this river (the river basin) is subdivided into coded sub-basins and inter-basins. The four largest tributaries, according to the criterion of area drained, form sub-basins. These are assigned the numbers 2, 4, 6, and 8, in the order in which they are encountered as one goes upstream along the main stem ().
Fig. 6 Principle of Pfafstetter coding of main tributaries (from Vogt et al., Citation2007a).
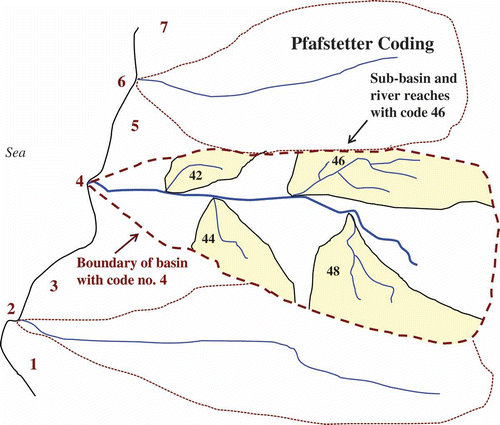
Next, the inter-basins are numbered 1, 3, 5, 7, and 9, again working upstream from the mouth of the main stem. Inter-basin 1 is the area drained by the main stem between the mouth of sub-basin 2 and the mouth of the main stem. Inter-basin 3 is the area drained by the main stem between the mouths of sub-basins 2 and 4. Inter-basin 9 always consists of the headwaters area of the main stem (see ). If a closed basin is encountered, it is assigned the number 0 (zero).
Fig. 7 Principle of Pfafstetter coding of inter-basins (from Vogt et al., Citation2007a).
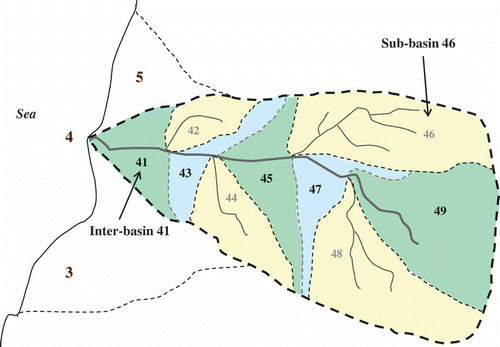
It should be noted that the river reaches along the main stem are identified by the inter-basin codes. Ultimately, the inter-basin codes define the full river network. They also identify the areas that drain in a diffused manner into that network. This system is then iteratively repeated for the sub-basins and inter-basins down to the level of the smallest river reaches (). Please note that in cases where the branching provides less than nine individual river stretches, the longest flow path (in a given sub-system) can have a final odd digit smaller than “9.” This is due to the fact that we code upstream from the river mouth. An example is the branch with code “467” in . In these cases the highest final digit identifies the longest flow path in the given sub-system. Due to its characteristics the Pfafstetter system allows the addition of more detail as more information becomes available, without necessarily disrupting the higher level codes. While implementing the Pfafstetter coding in CCM2, the code from every river reach was assigned also to the primary catchment drained by it, thus allowing for a codification not only of the river network but also of the entire area drained. The resulting coding for the Thames River basin is shown in for all river reaches and in for the sub-basins resulting from a truncation to the second Pfafstetter digit.
Fig. 8 Principle of Pfafstetter coding of sub-basins (from Vogt et al., Citation2007a).
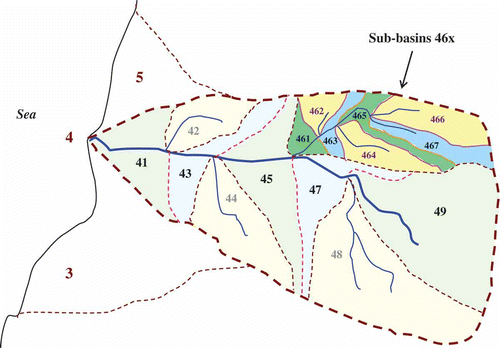
Fig. 9 Full Pfafstetter codes for the river reaches of the Thames River basin (UK) (from Vogt et al., Citation2007a).
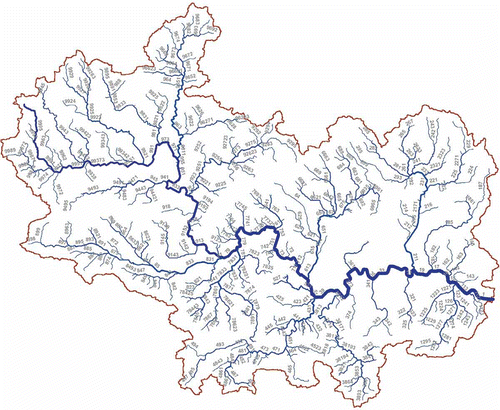
Fig. 10 Sub-basins with Level 2 Pfafstetter codes for the Thames River basin (UK) (from Vogt et al., Citation2007a).
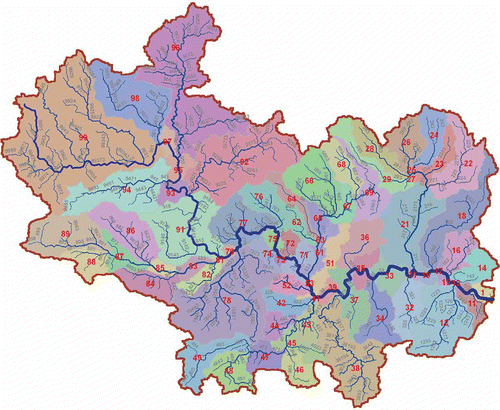
Final considerations
The advantage of this coding mechanism is that a unique identifier can be generated automatically and that a user can read the relative position of any hydrological feature with respect to the river basin, landmass and sea from the hydrological code. Due to the usage of numbers in the code a logical ordering can be achieved allowing identification of gaps or double records in a database. For data exchange functions it is further important to define a uniform format for the code. This can be done by defining fixed field lengths using leading zeros for short codes.
The Pfafstetter code is a coding system that reflects defined rules based on the length of the flow path and the size of the drained area. The longest flow path does, however, not always correspond to the known or agreed source of the main river. The coding system should be regarded as a topologically-smart coding system only and not as necessarily reflecting geographical conventions. A very striking example is found in the Rhone basin, where the Saone forms the longest flow path. As a consequence, the upper branch of the Rhone is regarded as a tributary to the Saone in the Pfafstetter system. Another example is the Wisla. Its source has traditionally been defined on the basis of greatest discharge volume and not on the criterion of longest flow path as in our database. The topological information embedded in the proposed codes does, however, not lose any of its usefulness for hydrological analysis, independent of these possible differences with respect to geographical traditions.
The sorting of islands can take a long time if small rocks are not excluded in archipelagos like the Aland Islands, for example. In our examples we created a sorting identifier for islands larger than 2.5 km2 only. Such a definition is arbitrary and depending on the application. Similar considerations apply to the replications (iterations) for deriving the commencement and Pfafstetter codes. In Europe it was sufficient to replicate in river basins up to five times and in the river systems up to 12 times. The number depends largely on the complexity of the hydrological system and the topography. Thus the length of the code can also be seen as an indication of the complexity of the hydrological system. A long Pfafstetter code signals a mature river system and a long commencement code a young system (Strahler, Citation1952).
The hydrological code presented here is logic for areas were the water flows according to surface flow rules. Areas like polders, irrigated plains, karstic areas, and postglacial landscapes do not necessarily follow this logic. One can generate a hydrological code for such areas just for the sake of uniquely identifying features.
INTERPRETING THE HYDROLOGICAL FEATURE CODE
The proposed hydrological feature code is built up of five components. Each component reflects the position and function of a hydrological feature within the complex hydrological cycle. The first letter of the code signals the hydrological system to which the water flows. The second number defines the sea into which a river basin drains. Then the landmass on which the river basin lies is identified. This is done by reserving the number 0 for the continental landmass and a sequence numbers for the islands along and at a certain distance off the continental coastline. For each landmass a commencement code is then determined per river basin whilst within a river basin the Pfafstetter code is used to identify any river stretch and its drainage area (catchment). provides an overview of the different components.
Table 6 Overview of the components of the proposed hydrological feature code
The complete code can be read as in the following example:
A5 0 8 9999: River stretch in the headwaters of a large (8 is one digit only) river system on the continent (0) flowing into the North Sea (5), being part of the Atlantic Ocean (A). With some experience of the system it is also clear that the river stretch belongs to the Rhine system draining into the southern part of the North Sea.
The proposed coding system can be used, for example, to analyse topological relationships (e.g. upstream–downstream relationships) within a river network and its sub-basins or also in relation to a sea. Within the river system, the analysis is based on simple larger–smaller and odd–even comparisons of the individual digits of the code. illustrates the concept for identifying river stretches affected by a point source pollution event entering the system in the branch with code 464. Note that the code is not to be understood as a number (i.e. four hundred and sixty four) but that the individual digits of the code have to be considered (i.e. four-six-four). The hypothetical example in shows point source pollution that enters the drainage system at branch 4–6–4 of a hypothetical river basin with code 4. By simple larger–smaller and odd–even comparisons of the different digits, potentially polluted stretches can easily be identified; detailed explanation is given in . The same applies for the identification of upstream river stretches and their catchments. An example of the latter case would be the identification of potential source areas for pollution measured at a given point in the system.
The simplicity of the code and the fact that it implicitly encodes the topology of the system allows for the development of automatic queries on a database without even displaying the river system graphically.
Commencement codes and Pfafstetter codes can also be used to group hydrological data in a manner that makes sense with respect to the coastline ordering. If we explore this option it implies that one can use the coding technique also as a hydrologically-relevant grouping mechanism for data.
A topologically-structured data set like CCM2, containing topological connections such as direction of water flow, allows generation of the proposed coding in an automated and repeatable manner. In addition, one can consider transferring the code from CCM2 geometric data to other geometric data using classical geometric intersection techniques (Burrough, Citation1986). We made various tests to code lakes, rivers, beaches or even features that do not follow surface hydrological flow. The main consideration for transferring a code from CCM2 geometries to other geometries is to set a clear rule that allows the creation of an automatic procedure for transferring the code. As an example we coded lakes with the Pfafstetter code of the river stretch draining the lake. Appendix lists several online examples that give more insight to the drawbacks and advantages of such coding applications. Furthermore, readers can download CCM2 data containing the described hydrological coding at http://ccm.jrc.ec.europa.eu/ in order to analyse the data and implement individual applications.
SUMMARY AND CONCLUSIONS
The proposed hydrological feature coding system (ocean/endorheic code, sea code, island code, commencement code, and Pfafstetter code) has been developed in order to provide for a consistent coding of all hydrological features throughout the European continent. Based on its structure, it could relatively easily be extended to other continents and the entire globe. An important feature is the possibility to implement it automatically within a structured and complete database. Thus the system is repeatable and can be updated if more detailed data become available.
The generation of the code follows a Pfafstetter logic in all its parts (i.e. assigning even numbers to the four biggest river basins draining to a given sea and odd numbers to the five inter-basins, replicated to lower levels as necessary). As a result, the system code, the sea code, the commencement code and the Pfafstetter code together provide a unique and structured hydrological feature code for each river reach and its corresponding catchment.
Lakes are treated in such a way that they carry the code of the river reach draining the lake. Thus they can be located within the system and both the downstream flow path as well as all upstream river reaches draining into the lake can be identified. Small lakes that are not linked to any river reach in the system will receive the code of the primary catchment in which they fall or, in the case where several primary catchments intersect with a lake, the code of the primary catchment furthest downstream.
This code carries important topological information, allowing for the analysis of topological relationships within the system (e.g. upstream–downstream analysis).
Acknowledgements
The hydrological code evolved from discussions within the GIS Working Group and the Hydrological Coding Working Group under the Common Implementation Strategy for the Water Framework Directive. These working groups included representatives from EU Member States and the European Commission. Special thanks are due to Peter Britton for his excellent review of hydrological feature coding systems, as well as to Joachim D'Eugenio and Albrecht Wirthmann, European Commission, for their support to this activity. Thanks are further due to two anonymous reviewers whose comments helped to improve an earlier version of the manuscript.
Notes
1Throughout this paper we use the term “river basin” to denote the area drained by an entire river system. A river basin thus drains to the sea or an endorheic system. A “catchment” is a hydrological sub-unit (i.e. an area draining to a given point such as a river confluence). Catchments exist at various hierarchical levels within a river basin. A “primary catchment” is the smallest entity in this hierarchy and is drained by one river segment.
2CCM: Catchment Characterisation and Monitoring. A project run by the European Commission, Joint Research Centre, Institute for Environment and Sustainability. CCM2 refers to version 2 of the CCM River and Catchment Database for Europe from 2007. For further details see http://ccm.jrc.ec.europa.eu
References
- Arge , L. , Danner , A. , Haverkort , H. and Zeh , N. 2006 . “ I/O-efficient hierarchical catchment decomposition of grid terrain models ” . In Process in Spatial Data Handling , Edited by: Riedl , A. , Kainz , W. and Elmes , G. 825 – 844 . Berlin, Heidelberg : Springer . Ined. by
- Brilly, M. & Vidmar, A. (1995) Catchment coding of large river basins, modelling and management of sustainable basin-scale water resource systems. In: Modelling and Management of Sustainable Basin-Scale Water Resource Systems (ed. by S. P. Simonovic, Z. Kundzewicz, D. Rosbjerg & K. Takeuchi), 347–351. IAHS Publ. 231. Wallingford: IAHS Press. http://www.iahs.info/redbooks/231.htm (http://www.iahs.info/redbooks/231.htm) (Accessed: 11 May 2010 ).
- Britton, P. (2002) Review of existing river coding systems for river basin management and reporting. In: WFD GIS working group: European coding systems task group http://ftp.ei.jrc.it/pub/dejager/wise/Britton-River-Coding-Review-2002s.pdf. (http://ftp.ei.jrc.it/pub/dejager/wise/Britton-River-Coding-Review-2002s.pdf.)
- Burrough , P. A. 1986 . Principles of Geographical Information Systems for Land Resources Assessment , Oxford : Oxford University Press .
- Colombo , R. , Vogt , J. V. , Soille , P. , Paracchini , M. L. and de Jager , A. 2007 . Deriving river networks and catchments at the European scale from medium resolution digital elevation data . Catena , 70 ( 70 ) : 296 – 305 .
- De Jager , A. , Rimaviciute , E. and Haastrup , P. 2007 . “ A water reference for Europe ” . In Environmental Data Exchange Network for Inland Water , Edited by: Haastrup , P. and Würtz , J. 259 – 286 . Amsterdam, , The Netherlands : Elsevier .
- EC (European Communities) . 2003 . Implementing the Geographical Information System Elements (GIS) of the Water Framework Directive , Luxembourg : Office for Official Publications of the European Communities . Common implementation strategy for the water framework directive, guidance document no. 9ISBN 92–894–5129–7
- FGDC (Federal Geographic Data Committee) (2003) Federal standards for delineation of hydrologic unit boundaries. http://www.fgdc.gov (http://www.fgdc.gov) (Accessed: 11 May 2010 ).
- International Hydrographic Organization . 1953 . Limits of Oceans and Seas , 3rd , Monegasque, Monte Carlo : International Hydrographic Organization . Special Publication no. 23. Imp
- Nõges , P. and de Jager , A. 2009 . Clustering large lakes and reservoirs in Europe on the basis of their morphometry, catchment, and climatic conditions . Verh. Int. Ver. Limnol. , 30 ( 30 ) : 993 – 996 .
- Pfafstetter , O. 1989 . “ Classification of hydrographic basins: coding methodology ” . In Unpublished manuscript. Departamento Nacional de Obras de Saneamento , Sioux Falls, SD : US Geological Survey, EROS Data Center . 18 August 1989, Rio de Janeiro; Available from: J. P. Verdin
- Scharf , F. and de Jager , A. 2006 . Delineation of Potential Locations for Intercalibration Sites for Rivers , Ispra, , VA Italy : Joint Research Centre, Report EUR 22628 .
- Soille , P. , Vogt , J. V. and Colombo , R. 2003 . Carving and adaptive drainage enforcement of grid digital elevation models . Water Resour. Res. , 29 ( 29 ) : 1366 doi:10.1029/2002WR001879.
- Strahler , A. N. 1952 . Hypsometric (area-altitude) analysis of erosional topography . Geol. Soc. Am. Bull. , 63 : 1117 – 1142 .
- Verdin , K. L. and Verdin , J. P. 1999 . A topological system for the delineation and codification of the Earth's river basins . J. Hydrol. , 218 : 1 – 12 .
- Vogt, J. V., Soille, P., de Jager, A., Rimaviciute, E., Mehl, W., Foisneau, S., Bodis, K., Dusart, J., Paracchini, M. L., Haastrup, P. & Bamps, C. (2007a) A pan-European River and Catchment Database. Luxembourg: Office for Official Publications of the European Communities, Report EUR 22920 EN. ISBN: 978–92–79–06941–3. http://ccm.jrc.ec.europa.eu (http://ccm.jrc.ec.europa.eu) (Accessed: 11 May 2010 ).
- Vogt, J. V., Soille, P., de Jager, A., Rimaviciute, E., Mehl, W., Haastrup, P., Paracchini, M. L., Dusart, J., Bodis, K., Foisneau, S. & Bamps, C. (2007b) Developing a pan-European database of drainage networks and catchment boundaries from a 100 meter DEM. In: Proc. 10th AGILE Int. Conf. on Geographic Information Science, 8–11 May 2007, Aalborg, Denmark http://www.agile-online.org/ (http://www.agile-online.org/) (Accessed: 11 May 2010 ).
APPENDIX
Online examples of the hydrological code in sorted lists
-
The named River Rhine:
-
http://desert.jrc.ec.europa.eu/water/ccm/php/jrc_river.php?action=view&id=204850
-
Example of island coding using a hexadecimal sorting number:
-
http://desert.jrc.ec.europa.eu/action/php/index.php?action=view&id=38
-
Rivers draining an area larger than 400 km2 with a code proposal:
-
http://desert.jrc.ec.europa.eu/action/php/index.php?action=view&id=39
-
Named lakes per catchment and a proposed hydrological code:
-
http://desert.jrc.ec.europa.eu/action/php/index.php?action=view&id=44