Abstract
The runoff regime of glacierized headwater catchments in the Alps is essentially characterized by snow and ice melt. High Alpine drainage basins influence distant downstream catchments of the Rhine River basin. In particular, during the summer months, low-flow conditions are probable with strongly reduced snow and ice melt under climate change conditions. This study attempts to quantify present and future contributions from snow and ice melt to summer runoff at different spatial scales. For the small Silvretta catchment (103 km2) in the Swiss Alps, with a glacierization of 7%, the HBV model and the glacio-hydrological model GERM are applied for calculating future runoff based on different regional climate scenarios. We evaluate the importance of snow and ice melt in the runoff regime. Comparison of the models indicates that the HBV model strongly overestimates the future contribution of glacier melt to runoff, as glaciers are considered as static components. Furthermore, we provide estimates of the current meltwater contribution of glaciers for several catchments downstream on the River Rhine during the month of August. Snow and ice melt processes have a significant direct impact on summer runoff, not only for high mountain catchments, but also for large transboundary basins. A future shift in the hydrological regime and the disappearance of glaciers might favour low-flow conditions during summer along the Rhine.
Citation Junghans, N., Cullmann, J. & Huss, M. (2011) Evaluating the effect of snow and ice melt in an Alpine headwater catchment and further downstream in the River Rhine. Hydrol. Sci. J. 56(6), 981–993.
Résumé
Le régime hydrologique des bassins versants amont glaciaires dans les Alpes est essentiellement caractérisé par la fonte de neige et de glace. Les hauts bassins versants alpins influencent les débits du Rhin loin en aval. Avec le changement climatique, des faibles débits seront possibles en période estivale en raison de la forte diminution de la fonte de neige et de glace. Cette étude tente de quantifier les contributions actuelles et futures de la fonte de neige et de glace dans l'écoulement estival à différentes échelles spatiales. Le modèle HBV et le modèle glacio-hydrologique GERM sont appliqués au petit bassin versant du Silvretta (103 km2), situé dans les Alpes Suisses et ayant une surface englacée de 7%, afin de calculer l'écoulement futur suivant différents scénarios climatiques régionaux. Nous évaluons l'importance de la fonte de neige et de glace dans le régime d'écoulement. Une comparaison des modèles indique que le modèle HBV surestime fortement la contribution future provenant de la fonte des glaciers dans le débit total, le modèle considérant les glaciers comme des éléments statiques. En outre, nous fournissons des estimations, pour le mois d'août, de la contribution actuelle des glaciers en eau de fonte pour plusieurs bassins versants du Rhin. Les processus de fonte de neige et de glace ont un impact direct sur les débits estivaux, non seulement pour les bassins versants de haute montagne, mais aussi pour les grands bassins transfrontaliers. Un décalage futur dans le régime hydrologique et la disparition des glaciers pourraient favoriser des conditions de faible débit en été sur le Rhin.
SETTING THE SCENE
Global warming already has a commensurable effect on the runoff regime of Alpine drainage basins (e.g. Zierl and Bugmann Citation2005, Koboltschnig et al. Citation2009). Glacierized catchments are highly vulnerable and are particularly affected. The runoff processes in these glacial or nivo-glacial regimes differ considerably from the predominantly pluvial runoff regimes in downstream catchments. Hence, the changes in the runoff characteristics triggered by global warming also impact on runoff in areas located further downstream. The River Rhine, originating mainly in the Swiss Alps, and running through Austria, Liechtenstein, Germany, France and The Netherlands on its way to the North Sea, serves as an example for these rather complex processes.
Glacierized catchments are characterized by temporarily storing precipitation and then releasing it with a time delay. This feature significantly impacts on runoff processes in glacierized regions and downstream. Winter precipitation is stored as snow and ice and released during spring and summer melting seasons. This is also reflected in the shape of the runoff hydrograph. Hence, runoff in winter is low, as almost the total amount of precipitation is stored. In summer, in contrast, maximum discharge is reached due to snow and ice melt.
Given their distinctive runoff behaviour, glacial and nivo-glacial regimes can have a considerable influence on downstream regions. Snow melt provides additional flow for downstream river sections with pluvial characteristics during the summer period. Without the impact of snow melt, a more distinct low flow can be expected for many gauges along the River Rhine during summer months. The present study assesses this context, as it may entail consequences for various branches of the economy.
Future changes in the runoff regime of glacierized watersheds have been analysed in detail in a number of studies using different models (e.g. Horton et al. 2006, Schaefli et al. Citation2007, Hagg et al. Citation2007, Stahl et al. Citation2008, Huss et al. Citation2008). However, most studies have focused on rather small and highly glacierized drainage basins. The importance of glacier melt for larger catchments, and its contribution during low-flow conditions, have rarely been quantified. Weber et al. (Citation2010) present a comprehensive hydrological modelling framework for the upper Danube watershed. They find a relatively small contribution of ice melt on an annual scale and project a fast decrease of glacial meltwater under climate change conditions. However, the resolution of their glacier model is coarse; thus, several processes determining glacier mass balance are difficult to consider in detail.
The impact of meltwater from glaciers on the runoff regime can be quantified based on two different concepts: (1) the separation of snow and ice melt runoff using a hydrological model, or (2) the glacier storage change, ΔS over a given time period. Approach (1) requires the application and the careful calibration of a distributed hydrological model and provides process-based portions of melt originating from the snow pack or from bare ice. This has a considerable advantage when analysing changes in the runoff regime of small drainage basins, because the individual components of the changes are revealed. However, approach (2) is well suited for first-order estimates of glacier contribution to runoff in large catchments. This approach is purely based on the closure of the water balance. The monthly glacier storage change, ΔS, is made up by snow accumulation in winter, snow and ice melt in early summer, and mostly ice melt in late summer. In the present study, we use approach (1) for detailed hydrological modelling of a small-scale Alpine catchment, and approach (2) is applied to estimate the importance of glacial meltwater for transboundary basins of the River Rhine.
This paper investigates the impacts of climate change on Alpine runoff regimes by using regional climate scenarios. We present a comparison between the HBV model (Lindström et al. Citation1997) and the glacio-hydrological model GERM (Huss et al. Citation2008). For a small, moderately glacierized Alpine drainage basin, we evaluate the future contribution of snow and ice melt to total runoff using the two models and different climate change scenarios. Furthermore, we estimate the current contribution of glaciers to the August runoff of large watersheds of the River Rhine. This allows an estimate of the impacts of climate change-induced shifts in the runoff regime due to changes in the cryosphere on large transboundary watersheds.
STUDY SITE AND FIELD DATA
The study site is the catchment upstream of the Klosters gauge on the River Landquart, located in eastern Switzerland in the canton of Grisons, being part of the Silvretta group mountain range. The catchment area is 103 km2, and it is located at an altitude between 1300 and 3300 m a.s.l. (). In addition to the Silvretta Glacier, with an area, currently, of 2.8 km2, the region features other smaller glaciers; approximately 7% of the catchment area is currently glacierized.
Fig. 1 Catchment of River Rhine with selected gauges and the study site; the inset shows the topography of the catchment of gauge Klosters (detail).
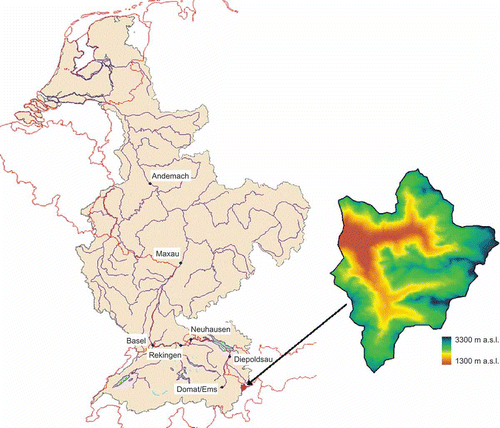
Field data from the study site comprise:
-- | meteorological data (air temperature and precipitation at daily resolution for Davos and Klosters, 1961–2008); | ||||
-- | daily discharge measured at the Klosters gauge, River Landquart (1976–2004); | ||||
-- | seasonal glacier mass balances observed for the Silvretta Glacier (since 1960, Huss et al. Citation2009a); and | ||||
-- | digital terrain models (DTM), with 50 m × 50 m resolution (six DTMs since 1959). |
To assess the impact of snow and ice melt on the River Rhine, we select seven gauges located in the region extending from the Alpine Rhine to the Middle Rhine (). These gauges are listed in . For all gauges, daily discharge data from 1970–2003 are used.
Table 1 Selected Rhine River gauges (sources: BAFU Citation2008 and BfG)
Climate change scenarios
Future climate change will affect all parts of the world and lead to strong impacts on earth surface processes (e.g. IPCC Citation2007). In Switzerland, as well as in the entire Alpine region, climate change will be characterized primarily by a perennial rise in air temperature, an increase in winter precipitation and a decrease in summer precipitation (Christensen and Christensen Citation2007). These changes are expected to have a strong impact on snow and ice melt, and thus on streamflow runoff (e.g. Zappa et al. Citation2003, Horton et al. Citation2006, Schaefli et al. Citation2007). A general decrease in the amount of snow generated and melting is projected (OcCC Citation2008). An initial increase in glacier melt due to the release of water from glacial storage is expected to be followed by a decrease due to shrinking of the glacierized area (Huss et al. Citation2008, Schädler Citation2008).
In this paper, the effects of climate change on melting processes are explored in a small test catchment using regional climate scenarios at seasonal resolution. The climate studies of Switzerland according to Frei (Citation2007), based on the results of the PRUDENCE project (Christensen and Christensen Citation2007), serve as the starting point for the climate scenarios used. Proceeding on these results, Huss et al. (Citation2008) defined three climate scenarios that are used in the present study. Scenario 2 represents the median of the 16 regional climate model (RCM) results of the PRUDENCE project. The RCMs were driven using four different global circulation models. Two supplementary scenarios were defined corresponding to the 2.5% and 97.5% quantiles, respectively, of the statistical evaluation of the 16 RCMs (Frei 2007). Both Scenario 1 (cold and wet) and Scenario 3 (warm and dry) represent extreme climate evolutions. Changes in other atmospheric variables, such as global radiation and vapour pressure, were not taken into account. A temperature rise of 1.8°C in winter and 2.8 C in summer, as well as an increase in winter precipitation of 11% and a decline in summer precipitation of 19% are expected for the year 2050, according to the median scenario (). Due to a significant uncertainty in future climate evolution the spread between the scenarios is considerable, and we used the extreme scenarios 1 and 3 to provide lower and upper bounds on our modelling.
Table 2 Expected change in seasonal air temperature and precipitation for the northern flank of the Alps for 2050 relative to 1990, according to Frei (2007)
METHODS
Hydrological models
Two different hydrological models have been applied to calculate daily runoff from the study area (Silvretta catchment).
HBV The HBV model (Lindström et al. Citation1997), widely used at present, was developed for rainfall runoff modelling at the Swedish Meteorological and Hydrological Institute. It is a semi-distributed, conceptual model incorporating individual routines for snow (and ice), soil moisture and runoff formation and a simple routing procedure. HBV operates on daily time steps. Daily air temperature and precipitation are needed as input data. The basic calculation units are zones, built from land use and altitude. The discharge is calculated for sub-basins and then routed along the river.
Calculations considering glacier processes are contained in the snow routine. Snow and ice melt calculation is based on a simple temperature-index model with constant day-degree factors for snow and ice (Hock Citation2003). For water routing, an upper nonlinear and a lower linear reservoir are used. Actual evaporation is calculated using a simple empirical approach. No updating of the ice volume of glaciers is included. The glacier module of HBV is thus static, i.e. changes in the ice-covered area cannot be simulated. This entails problems for evaluating ice melt in the future, as glaciers are expected to shrink considerably, thus providing less meltwater.
GERM The GERM model (Glacier Evolution Runoff Model, Huss et al. Citation2008) is a combined glacio-hydrological model designed for calculating runoff from highly glacierized catchments and for the simulation of glacier mass balances. The model is grid-based, processing the same meteorological input data as the HBV model (daily temperature and precipitation). Snow and ice melt are calculated using a distributed temperature-index model (Hock Citation1999). Degree-day factors are varied as a function of potential solar radiation that is computed based on the DTM. This allows a spatially-distributed calculation of melting processes, while taking into account topographic effects such as slope and exposure. Actual evaporation is determined based on a simple empirical approach, and water is routed through several linear reservoirs. The initial ice volume in the catchment is estimated from an inversion of glacier surface topography (Farinotti et al. Citation2009), and is updated annually. This is done by redistributing calculated surface mass balance according to a spatial pattern observed in the past (see Huss et al. Citation2008 for details on all modules of GERM). This mass-conserving simulation of the glacier ice volume thus allows the analysis of the changes in glacial runoff under conditions of strong glacier retreat as a consequence of global warming.
Calibration and validation of models
Both HBV and GERM are forced using the same meteorological time series of daily temperature and precipitation. The calculated discharge at the catchment outlet was compared to the discharge measured at the Klosters gauge in order to calibrate and validate both models. Calculated and measured glacier mass balances of the Silvretta Glacier were used for calibration of the glacier modules.
The discharge values calculated using the HBV model ((b), R2 = 0.91) provide a slightly better adjustment to the measured discharge values than those of the GERM model ((a)). The glacier modules ((c)) do not show any significant differences in terms of portraying the dynamics of the glacier mass balance. For glacier mass balance calculated with GERM we find a Nash-Sutcliffe efficiency (Nash and Sutcliffe Citation1970) of R2 = 0.62, and for glacier mass balances modelled with HBV, R2 = 0.53.
Fig. 2 Gauge Klosters: model performance: monthly discharge values calculated using (a) GERM, and (b) HBV. (c) Comparison of measured and calculated annual mass balances of the Silvretta Glacier. R2 is the Nash-Sutcliffe efficiency criterion.
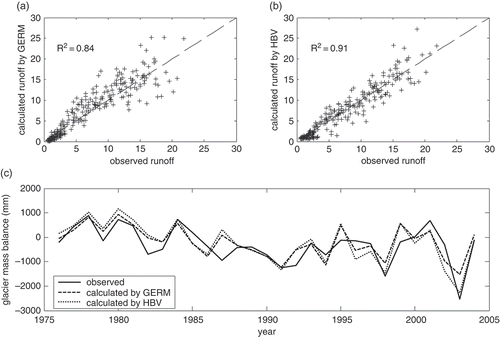
In general, both models are able to reproduce past processes in the catchment with sufficient accuracy. The question whether the models are also able to supply valid results for future developments will be explored in the following section.
Both HBV and GERM were run over the entire 21st century. In order to include year-to-year variability in weather conditions, randomly chosen years in the past were superimposed on the expected seasonal trends in meteorological variables (see ). Thus, continuous daily meteorological time series for the period 2008–2100 were generated that include variability consistent with past observations. For each of the three scenarios we performed 20 simulations using different meteorological time series with random variability. For the analyses we used the mean of these 20 model runs.
RESULTS
Model reliability in terms of scenario calculations
Assessing the reliability of both models for climate change impact studies is based on simulating the impact of three climate scenarios (Frei 2007, Huss et al. Citation2008) on the runoff regime at the Klosters gauge and the mass balance of Silvretta Glacier ( and ). For visualization of future runoff trends, the runoff hydrographs are depicted at intervals of 20 years, including the observed average discharge in the past (1976–2004).
Fig. 3 Results of the scenario calculations obtained at gauge Klosters: (a)–(c) using GERM; and (d)–(f) using the HBV model.
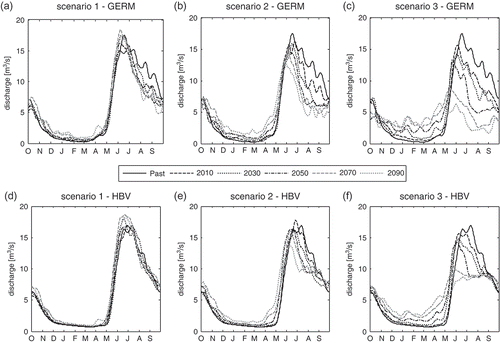
Fig. 4 Calculated future annual and seasonal glacier mass balances obtained: (a)–(c) by GERM, and (d)–(f) by HBV. Glacier mass balance is undefined after 2076 for Scenario 3 (GERM) as no ice is left by that time.
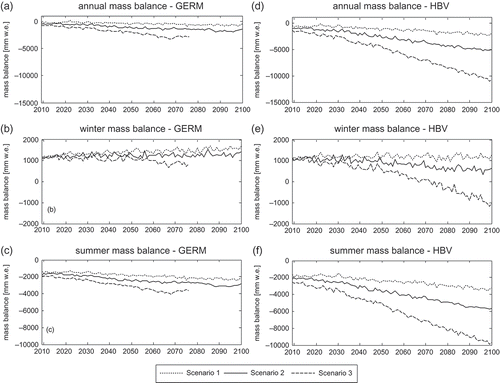
Evaluating the cold-wet Scenario 1, the results of both GERM and HBV show hardly any long-term trends in future runoff. For Scenario 2 (median) and Scenario 3 (warm-dry) the results obtained with GERM and HBV showed considerable differences (). In scenarios 2 and 3, GERM predicts a steadily decreasing peak discharge that additionally shifts towards an earlier date within the year. At the same time, runoff increases in winter. These effects are—as expected—much more pronounced for Scenario 3. According to this scenario, a distinct summer low-flow period is expected to occur, starting in the month of July, after 2050. These findings also correspond to previous studies of future runoff from high mountain catchments (Braun et al. Citation2000, Schaefli et al. Citation2007, Stahl et al. Citation2008, Huss et al. Citation2008). The results of HBV differ from this observation (): Although a reduction and shift of the peak discharge take place, runoff in late summer does not recede, but remains at a relatively high level (approx. 10 m3/s). This indicates that HBV yields similar general changes to GERM (shift in peak discharge, runoff increase in winter), but future low-flow conditions during summer months cannot be simulated.
The differences in future runoff calculated using HBV and GERM are mainly explained by the representation of glaciers in the modelling framework. In order to evaluate this component, we analysed time series of glacier mass balance given by both models for all scenarios (). We evaluated annual mass balance (hydrological year), as well as winter balance (1 October–30 April) and summer balance (1 May–30 September).
In comparison to the results of HBV, GERM shows significantly less negative mass balances ((a)–(c)). Glaciers are allowed to change their size, and ice volume is modelled in a transient and mass conserving way. Thus, glaciers continuously adapt to the given climate conditions by decreasing their size and retreating to higher elevation.
Glacier mass balances calculated by HBV become extremely negative ((d)–(f)). By 2100, HBV predicts annual glacier mass balances of –11 m w.e. for Scenario 3. This rate of ice thickness change would be sufficient to make the current ice volume of Silvretta Glacier disappear in a period of only 5 years. As the glaciers are not allowed to change their size in the model framework of HBV, they become an infinite source of water, which is especially significant in a warmer climate. Cumulated over the 21st century, runoff from ice melt is up to 10 times higher than the glacier ice volume currently stored in the region. For the month of August, this leads to high runoff ((d)–(f)), which is mostly due to runoff provided by melting of substantially too large glaciers.
As it is the aim of this study to evaluate the future contribution of snow and ice melt to runoff with a focus on future low-flow conditions in summer due to changes in the meltwater input, we decided to base our further analysis on GERM, as it reproduces glacier melt for the coming decades more realistically.
Proportions of meltwater and future changes
For the following analysis we only consider Scenario 2. This scenario represents the median of 16 RCMs and is assumed to yield the most likely climatic evolution for the 21st century. shows hydrographs of the total discharge, and the proportions resulting from snow and ice melt, for different years.
Fig. 5 Proportions of snow and ice melt in the total runoff, obtained using GERM at the Klosters gauge, for Scenario 2, averaged: (a) for the past, (b) for 2010, and (c)–(f) at 20-year intervals thereafter.
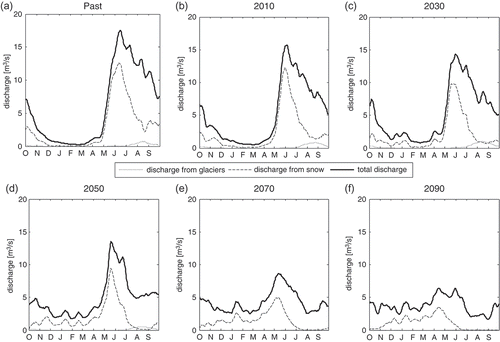
Fig. 6 Mean runoff hydrographs (1970–2003) of selected Rhine River gauges (data sources: BfG, and Global Runoff Data Center).
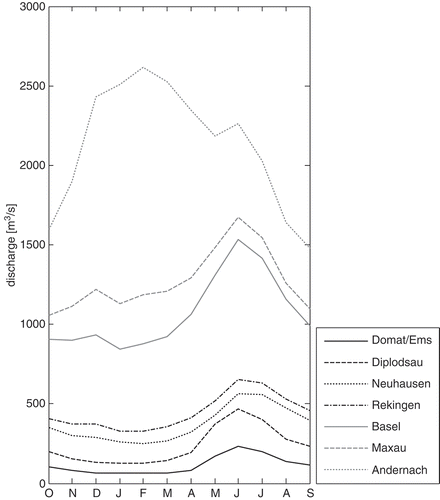
Proportions of snow melt for the past As shown in (a), snowmelt is the predominant runoff component in the Klosters catchment, primarily contributing to runoff from May to July, and its share in the total runoff of a hydrological year amounts to approx. 50%. The snow melt peak is reached in June. Basically, snow melt may occur throughout the year, as soon as energetic conditions allow for the melting process. However, these are usually very low in the months December–March.
In the study area, the level of glacierization is relatively small (approx. 7%). According to our simulations (GERM), the proportion of ice melt in the total annual runoff was 2%, on average, between 1976 and 2004. Ice melt only occurs if parts of the glacier are not snow-covered and bare ice appears at the surface. This generally applies in the months of July–September. Ice melt reaches its peak in August and provides about 5% of the runoff in this month.
Future development of melting processes according to Scenario 2 According to the simulations, runoff will decline in the summer months until 2090. Moreover, peak runoff will occur earlier, while winter runoff is expected to increase. The total annual discharge might decrease by 10–15% until 2090 in the Klosters region, according to the results of Scenario 2. As discussed below, this changed runoff behaviour is largely due to the changes in melt-induced runoff.
Over the 21st century, the proportion of snow melt, compared to total runoff is projected to decrease (). According to Scenario 2, total snow melt decreases by approximately 40% until the end of the century (). Also, the maximum of snow melt runoff in summer decreases. Owing to a rise in air temperatures, peak snow melt will generally shift towards earlier dates. Higher temperatures in winter will also increase the fraction of rainfall, consequently reducing snow accumulation in wintertime and enhancing direct runoff. Moreover, positive temperatures will prevail in winter with increasing frequency, causing additional snow melt-induced runoff ().
Table 3 Changes in the relative contribution of discharge components, precipitation and evapotranspiration over time, calculated by GERM. Results refer to Scenario 1 / Scenario 2 / Scenario 3
According to the results for Scenario 2, ice melt could initially double its value (compared to the past), followed by a subsequent strong decrease in melt flow as a result of receding glaciers—no ice will be left. Our results indicate a runoff peak from ice melt around 2050 (Fig. 5). In 2090, however, ice melt only accounts for one third of the amount observed in the past, and less than 1% of the total discharge. Unlike snowmelt, the timing of peak ice melt will not shift markedly (Fig. 5).
The results of scenarios 1 (wet and cold) and 3 (warm and dry) can be interpreted as the lower and upper ranges of the expected change (uncertainty bounds). Scenario 1 indicates no significant changes in annual precipitation and discharge. The amount of snow decreases slightly, while ice melt increases by one fourth until 2090. According to Scenario 3, in contrast, annual precipitation and discharge decrease by one third until the end of the century. The initial increase in ice melt is much stronger than in the median Scenario 2, but also the subsequent decrease is more pronounced. After 2070, ice melt is projected to drop to zero as the glaciers will have completely disappeared. Also, snow melt is expected to show a highly significant decrease, by two thirds.
Current contribution of glaciers to August runoff along the River Rhine
The proportions of snow and ice melt largely depend on the share of glaciers in the respective region, as well as on the catchment topography (altitude) and the temperature dynamics. This section aims to evaluate whether glacier storage change in August has currently a recognizable impact on the runoff in large transboundary catchments, and whether the probable decrease in meltwater yield during this month (see e.g. (a)–(c)) towards the end of the 21st century might favour low-flow conditions in late summer.
The long-term hydrographs (1970–2003) of the selected Rhine River gauges are shown in Fig. 6. The gauges at Domat/Ems, Diepoldsau, Neuhausen and Rekingen display a typical nivo-glacial regime. At the gauges Basel and Maxau the same regime type is shown, but there is a secondary peak in December indicating pluvial influences. The hydrograph at the Andernach gauge has a pluvial regime, but the secondary peak in June underlines the nival influences in the catchment.
Our simple estimate of current glacier contribution to runoff during the month of August is based on glacier mass balance data from 10 glaciers in the Rhine River catchment. Monthly glacier storage changes, ΔS, are available from the combination of a wide array of mass balance field data and observed ice volume changes, combined with distributed modelling (Huss et al. Citation2010). The 10 glaciers in the data set cover about 20% of the glacierized area in the Rhine River catchment. As they are spread over several sub-catchments, and include different sizes and exposures, they are assumed to be a representative sample, allowing extrapolation of glacier storage change to the entire Rhine River catchment. We evaluate the 10-glacier arithmetic mean of specific glacier storage changes in the month of August for a 20-year period (1989–2008), as well as for the year 2003.
In the catchment of the River Rhine at Basel (), the total glacierized area is 431 km2 according to glacier inventory data (Müller et al. Citation1976). Averaged over the last two decades, glaciers in the Rhine River catchment exhibited specific storage changes in August of –0.68 m w.e. (Huss et al. Citation2010). Thus they contributed 2.9 × 108 m3 of water to the hydrological cycle only during the month of August. If this water volume is evenly distributed across the entire month, we find a discharge of 110 m3/s originating solely from glacier storage change, ΔS. The year 2003, with its extreme European summer heat waves, has been interpreted as a precursor of the coming decades (Schär et al. Citation2004). Glaciers in the Rhine River basin have experienced specific storage changes of –1.20 m w.e. in August 2003, leading to a runoff contribution of 193 m3/s.
We now compare these total glacier discharges for August (mean of 1989–2008, and 2003) to observed runoff at several gauges along the River Rhine (). During the two last decades, August discharge at Basel is enhanced by as much as 10% by contribution from glacier storage change (). For the catchment of Andernach (area: 140 000 km2 and glacierization of only 0.3%), we still find a contribution of 7% of glacier storage change to August runoff. According to our estimate, contribution from glaciers was even more pronounced in the year 2003. We estimate the contribution of glacier storage change to the August runoff measured at Basel and at Andernach as 31% and 22%, respectively ().
Table 4 Measured August runoff in 1989–2008 and 2003, at all Rhine gauges shown in Fig. 1 and Table 1, compared to runoff attributed to glacier storage change in August. The percentages refer to the fraction of observed discharge explained by glacier storage change. Note that only the three largest catchments include all glaciers of the Rhine River basin, while the other gauges obtain less discharge from glacier storage change
These results indicate that the contribution of glaciers to summer runoff is important not only for Alpine catchments, but also in macro-scale basins with a glacierization of 1% or even less. This can be explained by the generally low runoff volumes, in the summer months in basins with a pluvial regime, which are further reduced by high rates of evapotranspiration. For example, August runoff increases by a factor of less than 1.5, going down the River Rhine from Basel to Andernach, whereas catchment size becomes four times larger. As both basins are fed by the same quantity of water contribution from glaciers, the impact of glacier meltwater is still clearly recognizable at Andernach. The contribution of glaciers to August runoff in 2003 was particularly pronounced (see also Koboltschnig et al. Citation2009). This is attributed to two factors: (a) strongly below-average streamflow runoff in non-glacierized catchments (), due to little precipitation and high evapotranspiration, and (b) an almost doubled rate of glacier storage change. The combination of these conditions led to a surprisingly high percentage of August discharge that can be attributed to the influence of glaciers (22% for a catchment with a glacierization of only 0.3%). In the Alpine Silvretta catchment, the glacier contribution in August 2003 is found to be only about twice as high as that at Andernach (). Because of relatively high overall precipitation and low evapotranspiration in the Alpine catchment, compared to the transboundary basin, mean discharge per unit area is higher, and the importance of glacier melt is moderate.
Strong glacier retreat in response to fast global warming might have a significant positive impact on the summer runoff of the River Rhine; however, only for a limited time. Our results based on Scenario 2 show that meltwater provided by ice melt could strongly decrease and converge to zero towards the end of the 21st century (), as small glaciers disappear completely and larger ice masses lose up to 90% of their current area (e.g. Zemp et al. Citation2006, Huss et al. Citation2008). Simultaneously, a shift in peak snow melt discharge by one or two months is likely to occur (see ). Thus, we expect a significant increase in low flows for summers in the second half of the 21st century, due to the cessation of the storage effect of snow and ice. Additionally, summer low-flow conditions could possibly be aggravated by higher evapotranspiration which accompanies the global warming, and lower summer precipitation which is indicated by climate models (e.g. Christensen and Christensen Citation2007).
As opposed to the contribution of glacier storage change, the proportion of snow meltwater in the River Rhine is much more difficult to quantify. Snow melt is not limited to the high Alpine sub-catchments, but also is an important factor for the Alpine foothills and uplands (i.e. in part also in Germany and France). In order to evaluate the fraction of snow melt in the River Rhine, further detailed studies are necessary.
Discussion of uncertainties
The evaluations and projections presented in this paper are subject to important uncertainties that are briefly discussed below. Therefore, our results should be understood as projections of future runoff from Alpine catchments downstream into transboundary watersheds, rather than definite forecasts of future discharge.
The accuracy of simulated runoff strongly depends on the input data, as well as on uncertainties in the modelling procedure. Projections of future climate change by different climate models largely differ. To some extent, this is due to unknown future emission of greenhouse gases, as well as their impact on the climate system. Thus, input data for our model, for example future air temperature and precipitation, cannot be taken as crisp and reliable forecasts. In particular, the evolution of precipitation, and its spatial and temporal distribution, are subject to important uncertainties. Our simulation of future runoff builds on these input data and, therefore, shows a large spread between the three different climate change scenarios defined to capture the range of possible future climate change.
The temperature-index method is used to calculate snow and ice melt in both GERM and HBV. However, the melt factors calibrated in the past might not be constant over longer periods with strong changes in the climate system. Huss et al. (Citation2009b) report changes in the melt factors in the range of ±10–20% over the last century. For modelling future runoff, the factors calibrated for the last decade (until 2007) are applied, resulting in additional uncertainties for the simulation of melt and discharge. Furthermore, many processes, such as melting, evapotranspiration and runoff routing, are implemented with simple approaches in both models. Some back-coupling effects might thus not be captured adequately. For example, changes in vegetation dynamics and future soil moisture need to be mentioned. A joint quantification of the total uncertainties in the runoff projections for the 21st century is difficult because of the number of processes involved. The different climate scenarios used allow the determination of a kind of uncertainty estimate given by the climate evolution. It can be stated that the spread in the climate scenarios dominates over modelling uncertainties for more remote future time horizons.
Estimates of the current contribution of glacier storage change to August discharge in macro-scale watersheds are relatively uncertain, due to the high degree of simplification and extrapolation that is required. Our data set contains only 10 glaciers in the drainage basin of the River Rhine. All other glacier surfaces in the catchment were assumed to yield the same average mass balance. This is recognized as an important simplification, but, in the context of this rough estimate, it is the only way possible to perform the calculation. The signal of glacier runoff into the river system is further blurred by the presence of hydropower reservoirs that often capture important fractions of runoff from glacierized catchments and only release it time-delayed. Also, the effects of large lakes along the Rhine (e.g. Lake Constance) are not considered. Furthermore, the transit time of water from the source down the River Rhine is not considered. The time delay might be of the order of a few weeks from the Alps to Andernach. Nevertheless, our methodology provides an order-of-magnitude estimate of the present contribution of glacier storage change to runoff in large-scale catchments.
CONCLUSION
We caution against using the static HBV glacier module for simulating the impacts of global warming on glacierized catchments. As HBV, in the version that was used in this study, does not take into account changes in glacier volume and area, runoff induced by ice melt is overestimated in the long term in the warming climate. A module allowing dynamic change in glacier ice volume and extent should be implemented in the HBV model in order to use it for simulations of future runoff from currently glacierized basins. The glacio-hydrological model GERM contains a module for calculating changes in ice volume and glacier extent. This allows more plausible results to be obtained for ice melt and glacier storage change. The drawback of GERM is the considerable computing time needed, due to the higher spatial resolution required to resolve the processes considered.
Our results show that runoff originating from bare ice melt is relatively small compared to annual runoff volume, also in an Alpine catchment with non-negligible glacierization. However, considering the glacier storage change, ΔS, in August, that also includes snow melt over ice-covered areas, glaciers are found to be important also at larger scales. In the month of August meltwater originating from glaciers currently enhances runoff at Basel (glacierization about 1%) by 9%. Moving away from the Alps, this fraction only decreases slowly. Generally less precipitation and higher evapotranspiration in the low-lands lead to smaller effective runoff. Thus, glacier storage change contributes significantly to summer runoff also in large transboundary watersheds.
In the future, the amount of meltwater will decrease, especially in the second half of the 21st century. This will be reflected in decreasing summer discharge values in the River Rhine, and may, for instance, aggravate water shortages in the summer months. Hence, a change in the runoff generation process in Switzerland would have a direct impact on water management in the downstream regions, e.g. The Netherlands. Furthermore, lower flow rates and consequently reduced discharge rates in the River Rhine might impact on the navigability of the waterway, which may, in turn, have economic impacts. The KLIWAS project is currently exploring options to secure timely connections to export and import routes during future low-discharge periods in the summer months (Krahe et al. Citation2009). In conclusion, it can be generalized that the expected changes in the hydrological regime of Switzerland will directly impact on the downstream riparian states of Germany, France, and The Netherlands. As regards the Rhine River basin, this topic will be dealt with by the intergovernmental organizations, such as the International Commission for the Protection of the Rhine (ICPR) and the International Commission for the Hydrology of the Rhine basin, which have proven effective cooperation in the past, continuing in the present, and can be regarded as examples of efficient trans-national river basin organizations.
Acknowledgements
We thank the Federal Office for the Environment (BAFU) for discharge data from the Klosters gauge; MeteoSwiss provided meteorological data; and VAW-ETHZ is acknowledged for digital terrain models of the Silvretta Glacier. We thank the Bundesanstalt für Gewässerkunde for their help in the implementation of the HBV model. We also thank the Global Runoff Data Centre for providing discharge data of Rhine River gauges. Finally, we thank the reviewers for their helpful comments.
REFERENCES
- Braun , L. , Weber , M. and Schulz , M. 2000 . Consequences of climate change for runoff from Alpine regions . Annals of Glaciology , 31 ( 1 ) : 19 – 25 .
- BAFU (Bundesamt für Umwelt) . 2009 . “ Hydrologisches Jahrbuch der Schweiz 2008 ” . In Umwelt-Wissen Nr. 0921. Bern , Switzerland : Bundesamt für Umwelt .
- BfG (Bundesanstalt für Gewässerkunde) . Deutsches Gewässerkundliches Jahrbuch, Rheingebiet , Teil I und Teil III. Koblenz, Germany : Bundesanstalt für Gewässerkunde .
- Christensen , J. H. and Christensen , O. B. 2007 . A summary of the PRUDENCE model projections of changes in European climate by the end of this century . Climatic Change , 81 ( 1 ) : 7 – 30 .
- Farinotti , D. , Huss , M. , Bauder , A. and Funk , M. 2009 . An estimate of the glacier ice volume in the the Swiss Alps . Global and Planetary Change , 68 ( 3 ) : 225 – 231 . doi: doi:10.1016/j.gloplacha.2009.05.004
- Frei, C., 2007. Die Klimazukunft der Schweiz. In: Klimaänderung und die Schweiz 2050—Erwartete Auswirkungen auf Umwelt, Gesellschaft und Wirtschaft. Beratendes Organ für Fragen der Klimaänderung (OcCC), 12–16, http://www.occc.ch. (http://www.occc.ch.)
- Hagg , W. , Braun , L. , Kuhn , M. and Nesgaard , T. 2007 . Modelling of hydrological response to climate change in glacierized Central Asian catchments . Journal of Hydrology , 332 : 40 – 53 .
- Hock , R. 1999 . A distributed temperature index ice and snow melt model including potential direct solar radiation . Journal of Glaciology , 45 ( 149 ) : 101 – 111 .
- Hock , R. 2003 . Temperature index melt modelling in mountain areas . Journal of Hydrology , 282 : 104 – 115 .
- Horton , P. 2006 . Assessment of climate-change impacts on alpine discharge regimes with climate model uncertainty . Hydrology and Earth System Sciences , 20 ( 10 ) : 2091 – 2109 .
- Huss , M. , Farinotti , D. , Bauder , A. and Funk , M. 2008 . Modelling runoff from highly glacierized alpine drainage basins in a changing climate . Hydrological Processes , 22 : 3888 – 3902 .
- Huss , M. , Bauder , A. and Funk , M. 2009a . Homogenization of long-term mass balance time series . Annals of Glaciology , 50 ( 50 ) : 198 – 206 .
- Huss , M. , Funk , M. and Ohmura , A. 2009b . Strong Alpine glacier melt in the 1940s due to enhanced solar radiation . Geophysical Research Letters , 36 : L23501 doi: doi:10.1029/2009GL040789
- Huss , M. , Hock , R. , Bauder , A. and Funk , M. 2010 . 100-year glacier mass loss in the Swiss Alps linked to the Atantic Multidecadal Oscillation . Geophysical Research Letters , 37 : L10105 doi: doi: 10.1029/2010GL042606
- IPCC (Intergovernmental Panel on Climate Change) . 2007 . “ Climate change 2007. The scientific basis ” . In Contribution of Working Group I to the Fourth Assessment Report of the Intergovernmental Panel on Climate Change , Cambridge : Cambridge University Press .
- Krahe , P. 2009 . Wirkungsabschätzung von Unsicherheiten der Klimamodellierung in Abflussprojektionen – Auswertung eines Multimodell-Ensembles im Rheingebiet . Hydrologie und Wasserbewirtschaftung , 53 ( 5 ) : 316 – 331 .
- Koboltschnig , G. R. , Schöner , W. , Zappa , M. and Holzmann , H. 2009 . Glaciermelt of a small basin contributing to runoff under the extreme climate conditions in the summer of 2003 . Hydrological Processes , 23 : 1010 – 1018 . doi: doi:10.1002/hyp.7203
- Lindström , G. 1997 . Development and test of the distributed HBV-96 hydrological model . Journal of Hydrology , 201 : 272 – 288 .
- Müller , F. , Caflisch , T. and Müller , G. 1976 . Firn und Eis der Schweizer Alpen , Zürich : Geographisches Institut der ETH Zürich, Gletscherinventar no. 57 .
- Nash , J.E. and Sutcliffe , J.V. 1970 . River flow forecasting through conceptual models part I – A discussion of principles . Journal of Hydrology , 10 ( 3 ) : 282 – 290 .
- OcCC (Organe consultatif sur les changements climatiques) . 2008 . Das Klima ändert – was nun? Der neue UN-Klimabericht (IPCC 2007) und die wichtigsten Ergebnisse aus Sicht der Schweiz , Bern, Switzerland : Organe consultatif sur les changements climatiques . ISBN: 978‐3‐907630‐33‐4
- Schädler , B. 2008 . Klimawandel . Gas Wasser Abwasser , 10 : 763 – 769 .
- Schaefli , B. , Hingray , B. and Musy , A. 2007 . Climate change and hydropower production in the Swiss Alps: quantification of potential impacts and related modelling uncertainties . Hydrology and Earth System Sciences , 11 ( 3 ) : 1191 – 1205 .
- Schär , C. 2004 . The role of increasing temperature variability in European summer heatwaves . Nature , 427 : 332 – 336 .
- Stahl , K. 2008 . Coupled modelling of glacier and streamflow response to future climate scenarios . Water Resources Research , 44 : W02422 doi: doi:10.1029/2007WR005956
- Weber , M. , Braun , L. , Mauser , W. and Prasch , M. 2010 . Contribution of rain, snow- and icemelt in the upper Danube discharge today and in the future . Geografia Fisica e Dinamica Quartenaria , 33 : 221 – 230 . IT ISSN 0391‐9838
- Zappa , M. 2003 . Seasonal water balance of an Alpine catchment as evaluated by different methods for spatially distributed snow melt modelling . Nordic Hydrology , 34 : 179 – 202 .
- Zierl , B. and Bugmann , H. 2005 . Global change impacts on hydrological processes in Alpine catchments . Water Resources Research , 41 : W02028 doi: doi:10.1029/2004WR003447
- Zemp , M. , Haeberli , W. , Hoelzle , M. and Paul , F. 2006 . Alpine glaciers to disappear within decades? . Geophysical Research Letters , 33 : L13504 – 026319 . doi: doi:10.1029/2006GL