Abstract
The hydrological response of a small agroforestry catchment in northwest Spain (Corbeira catchment, 16 km2) is analysed, with particular focus on rainfall events. Fifty-four rainfall–runoff events, from December 2004 to September 2007, were used to analyse the principal hydrological patterns and show which factors best explain the hydrological response. The nonlinearity between rainfall and runoff showed that the variability in the hydrological response of the catchment was linked to the seasonal dynamics of the rainfall and, to a lesser extent, to evapotranspiration. The runoff coefficient, estimated as the ratio between direct runoff and rainfall volume, on an event basis, was analysed as a function of rainfall characteristics (amount and intensity) and the initial catchment state conditions prior to an event, such as pre-event baseflow and antecedent rainfall index. The results revealed that the hydrological response depends both on the soil humidity conditions at the start of the event and on rainfall amount, whereas rainfall intensity presented only a significant correlation with discharge increment. The antecedent conditions seem to be a key point in runoff production, and they explain much of the response. The hydrographs are characterized by a steep rising limb, a relatively narrow peak discharge and slow recession limb. These data and the observations suggest that the subsurface flow is the dominant runoff process.
Editor Z.W. Kundzewicz; Associate editor T. Wagener
Citation Rodríguez-Blanco, M.L., Taboada-Castro, M.M. and Taboada-Castro, M.T., 2012. Rainfall–runoff response and event-based runoff coefficients in a humid area (northwest Spain). Hydrological Sciences Journal, 57 (3), 445–459.
Résumé
La réponse hydrologique d'un petit bassin versant agroforestier du nord-ouest de l'Espagne (bassin versant de Corbeira, 16 km2) est analysée, avec un accent particulier sur les événements pluvieux. Cinquante-quatre événements pluie-débit, de décembre 2004 à septembre 2007, ont été utilisés pour analyser les traits hydrologiques principaux et montrer quels facteurs expliquent le mieux la réponse hydrologique. La non-linéarité entre les pluies et le ruissellement montre que la variabilité de la réponse hydrologique du bassin versant est liée à la dynamique saisonnière de la pluie et, dans une moindre mesure, à l'évapotranspiration. Le coefficient de ruissellement, estimé comme le rapport entre le volume de ruissellement direct et celui des pluies, sur une base événementielle, a été analysé en fonction des caractéristiques des pluies (quantité et intensité) et les conditions initiales des états du bassin avant événement, telles que l'écoulement de base pré-événement et l'indice des pluies antérieures. Les résultats ont révélé que la réponse hydrologique dépend à la fois des conditions d'humidité du sol au début de l'événement (indirectement déduites de l'écoulement de base au début de chaque épisode de pluie) et de la quantité de pluie, tandis que l'intensité des précipitations n'a présenté une corrélation significative qu'avec les incréments de débit. Les conditions antérieures semblent être un point clé dans la production de ruissellement, et elles expliquent en grande partie la réponse. Les hydrogrammes sont caractérisés par un montée rapide, un débit de pointe relativement étroit et une récession lente. Ces données et les observations suggérent que l'écoulement souterrain est le processus d'écoulement dominant.
INTRODUCTION
The hydrological response of a catchment in humid regions is the combination of many complex hydrological processes, depending on the catchment characteristics (e.g. vegetation, land use, soil properties), but it is also a function of the antecedent conditions and rainfall characteristics. The traditional perception is that runoff generation is dominated by subsurface stormflow and by saturation excess overland flow (Cappus Citation1960, Hewlett and Hibbert Citation1967). However, process-based studies at both hillslope and catchment scales have demonstrated that in these environments runoff may be also produced as the result of infiltration excess overland flow, especially in areas regarded as impervious or of low permeability because of human activities (Jordan Citation1994). The hydrological systems are complex and show significant seasonality, such that the dominant processes may experience changes throughout the year (Kirkby Citation2005).
For several decades, systematic studies have been conducted extensively worldwide to ascertain the processes involved in the hydrological response (Hewlett and Hibbert Citation1967, Merz and Blöschl Citation2009). Most of the studies indicate that, independently of antecedent moisture conditions and rainfall characteristics, only one runoff-generating process is dominant in the catchment studied (Hewlett and Hibbert Citation1967). Few studies show the simultaneous occurrence of various runoff processes (Bonell et al. Citation1990, Jordan Citation1994, Latrón et al. Citation2008), given most of the studies are done in small catchments (a few hectares) with relatively homogenous characteristics and, in other cases, the recording periods are relatively short and do not include a broad spectrum of situations. Large river catchments tend to comprise a mosaic of different land uses and management practices, with heterogeneous geology, topography and soils. This spatial variability, in combination with spatial and temporal differences in rainfall patterns, will moderate the integrated river basin hydrological response. It is therefore not certain that conclusions drawn from small-scale studies are directly applicable to larger catchments (Cerdan et al. Citation2004). Several types of runoff-generating process are present in a medium-sized catchment, and the source areas of each type may amount to only a small fraction of the catchment (Dunne and Leopold Citation1978).
The study of hydrograph characteristics and the runoff volumes associated with rainfall events has been the classical approach in catchment hydrology for several decades. For instance, Hewlett and Hibbert (Citation1967) studied the rainfall–runoff relationship at the event scale for a larger number of catchments in the USA to define a factor of catchment hydrology response, whereas Hewlett et al. (Citation1977) used this methodology to demonstrate the negligible effect of rainfall intensity on runoff volume and peak discharge from a forest catchment. In Europe, to understand the runoff generation processes, several studies were conducted at the event scale in different types of catchments (Mulholland Citation1993, Jordan Citation1994, Meyles et al. Citation2003, Burns et al. Citation2005, Latrón et al. Citation2008). Cerdan et al. (Citation2004) analysed 345 rainfall–runoff events in three catchments of different sizes in France to study scale effects in the runoff generation process. García-Ruíz et al. (Citation2008) used rainfall and runoff data to study the influence of land use on the magnitude of the hydrological response in the central Pyrenees.
Most of the above-mentioned studies have shown that the rainfall–runoff transformation is a nonlinear process. The most important cause of nonlinearity is represented by the effect of antecedent conditions. It is well known that soil water content is one of the most important factors for the generation of runoff. It affects infiltration capacity and the capability of soils to store new rainfall water. Hence, the different runoff generating processes are highly regulated by soil characteristics and especially by soil moisture, and not by rainfall characteristics (Jordan Citation1994, Pfister et al. Citation2002, Merz and Blöschl Citation2009).
In Spain, many hydrological studies have been conducted to understand the hydrological response to individual rainfall events; most of these were conducted in small Mediterranean mountain research catchments and in arid and semi-arid environments. Through the study of the rainfall–runoff relationship, Martínez-Mena et al. (Citation1998) identified the runoff generation mechanisms and their controlling factors in Mediterranean semi-arid micro-catchments with different types of soils. Using 260 rainfall–runoff events, Martínez-Fernández et al. (Citation2005) demonstrated the importance of saturated areas in the generation of runoff in an experimental forestry catchment of the Spanish Central System. Latrón et al. (Citation2008) and Nadal-Romero et al. (Citation2008) used runoff volumes and coefficient to investigate the spatial and temporal variability of the hydrological response in Mediterranean areas as well as the influence of small badland areas on runoff production. Through the study of rainfall–runoff events, Serrano-Muela et al. (Citation2008) demonstrated that the depth to the water table was the most important factor in the relationship between precipitation and discharge in a well preserved mountain environment.
In wet Spain, which is the context of this study, there is a lack of information on the hydrological response of the catchments. Most routine measurements of water level are carried out by river basin authorities on a daily or weekly basis to monitor the discharge volume of medium-large catchments. However, daily discharge measurements are not sufficiently detailed to capture runoff events. In Galicia (a humid temperate region of northwest Spain), a few examples of detailed hydrological studies using sub-daily information exist. These studies are focused more on understanding the effects of fires (Fernández et al. Citation2006) and reforestation (Soto et al. Citation2005) on the water yield than on analysing the hydrological response of the catchments to rainfall events. The only studies that analysed rainfall–runoff events at the catchment scale in Galicia focus on a limited number of events (Alvarez et al. Citation2001, Varela Citation2002). This highlights the need for more concentrated efforts to establish a hydrological monitoring system so that the processes governing runoff generation for small-sized catchments in northwest Spain may be better understood.
In this paper, data recorded in the Corbeira catchment (16 km2) during the period October 2004–September 2007 are analysed and studied in detail. The main objectives of this study are to demonstrate the variability in hydrological behaviour in this catchment and to explore the role of controlling factors. Rainfall–runoff relationships and relationships between several hydrological variables are examined at the event scale.
DATA DESCRIPTION
This study was conducted in a small headwater tributary of the Mero River, located near the city of La Coruña in northwest Spain (). The main morphological characteristics of the catchment are shown in .
Table 1 General characteristics of the Corbeira catchment
The most abundant soils are Cambisols and Umbrisols (FAO Citation2006), with silt and silt-loam texture developed on a basic schist of “Ordenes Complex” (IGME Citation1981). Approximately 65% of the catchment is occupied by forests, the most frequently occurring species being Eucalyptus globulus and Pinus pinaster; the remainder consists of pasture and cultivated land, at 26% and 3.8%, respectively. The imperviousness ratio is very low, as the area covered by villages and the road network does not exceed 5% of the total catchment surface. The population density is about 35 inhabitants per square kilometre.
The climate regime can be considered as humid temperate with a mean annual rainfall over the 25 hydrological years (October–September) from 1983 to 2007 of 1042 mm, distributed mainly between October and March (about 66% of the total annual rainfall). The maximum mean monthly precipitation occurs in October (143.3 mm) and the minimum in July (27 mm). Mean minimum and maximum temperatures occur during January (8.4°C) and August (18.6°C), respectively. Annual reference evapotranspiration, estimated using the Penman-Monteith FAO method (Smith et al. Citation1991), is about 620 mm.
The Corbeira is a permanent river. The flow regime of the Corbeira is “pluvial oceanic”; the minimum discharge is from July to September due to less rainfall and more potential evapotranspiration (ETP) during summer, while high discharge is observed from November to March. Based on rainfall and streamflow seasonality in this area, a hydrological year was defined as 1 October to 30 September.
Climate variables: temperature and rainfall
For an overall picture of the weather during the study period, the evolution of monthly air temperature and rainfall, as well as the number of rainy days per month, are shown in . The temperature maximum was in July–August and the minimum in February, except in the last year when it was in December. The rainfall distribution throughout the year is typical of the wet region of Spain, showing a clear seasonal pattern: autumn is the wettest period (about 50% of annual rainfall), whereas the summer is always the driest. Yet, rainfall was still significant in this latter season, with approximately 12% of the annual rainfall.
Fig. 2 Top: Monthly mean of daily mean (solid line), maximum (dotted line) and minimum (discontinuous line) air temperatures during the period 2004–2007. Middle: monthly rainfall (bars) and number of rainy days per month (dots connected by a solid line) during the period 2004–2007. Bottom: monthly total runoff during the period 2004–2007.
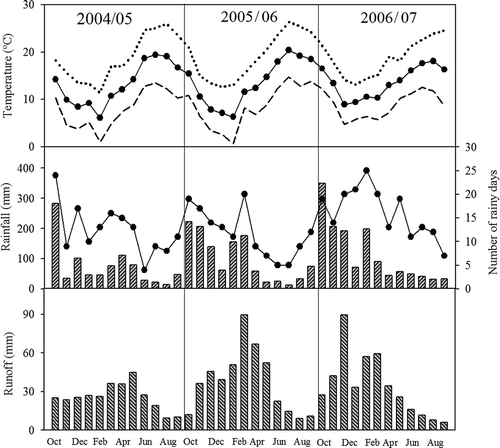
The inter-annual variability of the rainfall was quite high, with the minimum observed in 2004/05 (895.0 mm) and the maximum in 2006/07 (1397.2 mm, approx. 35% more than the mean rainfall of the last 24 hydrological years), reaching 1191.6 mm in 2005/06. The magnitude of this variability is also evident when we place these data into the scheme used by Romero-Díaz and López-Bermúdez (Citation1989) to classify the different years, depending on the precipitated water volume. By using this classification of annual rainfall amounts, the different hydrological years under study in the Corbeira catchment can be described as dry (2004/05), humid (2005/06) and very humid (2006/07). This fact is very important for this work because it illustrates the rainfall variability of the study area.
METHODOLOGY
Rainfall in the Corbeira catchment was recorded with three tipping-bucket raingauges (0.2 mm resolution) distributed across the catchment, at intervals of 10 min. Areal rainfall was calculated using Thiessen polygons.
At the catchment outlet, discharge was calculated from measurements of water level using a pressure transducer (ISCO720) connected to an automatic sampler (ISCO 6712-FS). Mean water level values (measured every 1 min) were recorded every 10 min. The gauging site did not include a control structure; therefore, the empirically-derived level–discharge rating curve equation relating logQ to log level was developed over a range of discharge values. Discharge measurements were undertaken for many different flow conditions using the velocity–area technique (WMO Citation1980). Flow velocity measurements were taken at 60% of the water depth (0.6D) at regular intervals along the cross-section by means of a current meter.
Soil water reserve data were derived by the MAFF method (MAFF Citation1991). Accumulated soil water deficit and surplus was calculated from the balance between rainfall, potential evapotranspiration (PET) and the soil's available water-holding capacity (AWC). First, the rainfall minus PET was used to represent the water available for each day. Secondly, the soil water reserve for each day was calculated to give either a surplus or a deficit.
Selection of individual hydrological events
To separate rainfall–runoff events, it is necessary to decide when a runoff event starts and ends. In this study, runoff events were identified as increases in discharge exceeding 1.5 times the discharge at the start of the rainfall event. Rainfall events separated by a minimum of 10 h without rainfall were considered separate events. These criteria allowed us to segregate 54 rainfall–runoff events during the entire monitoring period (October 2004–September 2007). The hydrograph was separated into two components (direct or quick runoff, and baseflow) using the constant slope method (Hewlett and Hibbert Citation1967). The original value was modified and a less steep line (1.83 L s-1 km-2 d-1; cf. Latrón et al. Citation2008) was used to ensure the cessation of runoff, because if the direct runoff at the end of a runoff event is significantly higher than zero the runoff coefficients are underestimated (Blume et al. Citation2007, Merz and Blöschl Citation2009).
For each rainfall–runoff event, several variables of the hyetograph and hydrograph were considered (). The analysis is based on the comparison of hydrograph characteristics, runoff volumes and runoff coefficients. The relationships between rainfall characteristics (rainfall amount and intensities) and the hydrological response of the catchment were studied by regression analysis.
Table 2 Name, abbreviations and units for the variables used to characterize rainfall–runoff events
RESULTS AND DISCUSSION
Overview of hydrological response
The three hydrological years considered in this study showed pronounced differences in the hydrological response () due to the inter-annual variability of the rainfall (). Runoff showed a higher trend for variability than rainfall, indicating the nonlinear relationship between rainfall and runoff rates in the study area, as described by numerous authors (Jordan et al. Citation1994, Merz and Blöschl Citation2009, Penna et al. Citation2011). This means that water yield from this catchment depends not only on the rainfall amount but also on its temporal distribution, as other catchment characteristics, such as land use and management, have remained unchanged during the study period.
Table 3 Annual and mean hydrological values for the study period
At the monthly scale, although there is a statistically significant relationship (r = 0.42, p < 0.01) between rainfall and runoff, data dispersion is high, so the same rainfall could produce different runoff. The absence of a straightforward relationship between monthly values of rainfall and water yield is not particular to the data shown here, and a similar effect has already been reported for humid temperate catchments (Endale et al. Citation2006, Merz et al. Citation2006, Penna et al. Citation2011) and for Mediterranean catchments (García-Ruíz et al. Citation2008), in particular. A possible factor explaining this nonlinearity is related to the number, the succession and the characteristics of the rainfall events, as well as to the seasonal dynamics of rainfall and evapotranspiration, which then affect the antecedent conditions for individual rainfall events. This seasonality promotes the succession of two periods throughout the year:
a. | The period of water deficit, in which evapotranspiration exceeds the monthly rainfall amount, extends generally from April to September. During this period, rainfall amounts are not very high and the hydrological response of the catchment is scarce, since a substantial proportion of the incident rainfall is lost as evaporation, or is used to supply the soil water demand. | ||||
b. | From October to March, the rainfall amount is greater than the loss by evapotranspiration, a surplus of moisture existing in the soil. Since the demand is smaller than the supply, the groundwater resources and the surface streams are fed with this excess water. Although the largest surplus of water is recorded in October (), the highest discharges are registered in December (), showing that water yield not only depends on the rainfall amount but is also heavily influenced by the antecedent soil moisture conditions. |
Baseflow is responsible for approximately 75% of the annual water yield. This value is comparable to that obtained in other agroforestry catchments of Galicia, despite their different drainage areas (Varela Citation2002, Rial et al. Citation2005), but very high in comparison with ratios from other streams in northeast Spain (García-Ruíz et al. Citation2008, Latrón et al. Citation2008). The scarce direct runoff is concentrated basically in very wet periods, specifically after a sequence of closely-occurring rainfall events. Thus, for the hydrological year 2006/07, in the periods 10–30 October, 15 November–15 December and 10–28 February, the direct runoff accounted for 4.5, 9.8 and 4.7%, respectively, of the water originating from the catchment.
The annual runoff coefficient (estimated as the ratio between total annual runoff and areal catchment rainfall) can vary considerably from year to year in the same catchment in response to differences in the rainfall amount and distribution throughout the year, as illustrated in many case studies worldwide (e.g. Martínez-Fernández et al. Citation2005, Macrae et al. Citation2007). However, in the Corbeira catchment, variations in the runoff coefficient have been limited, despite the notable differences in annual rainfall during the study period. This coefficient fluctuated between 29% in 2006/07 and 37% in 2004/05, reaching a value of 36% in 2005/06. These values are substantially lower than those measured in other catchments with a similar hydroclimate and land use, where runoff coefficients are usually above 50% (e.g. Rial et al. Citation2005), and could be related to the low water supply capacity of soils of the catchment. In fact, Martínez-Cortizas et al. (Citation1994) provided for the whole Mero basin, which is a tributary of the Corbeira stream, water supply coefficients of 30–40% rainfall, values close to those obtained in the present study.
Hydrological response at the event scale
To investigate the variability of the hydrological response of the Corbeira catchment, an analysis at the event scale was carried out, applying a methodology analogous to that used by other authors (e.g. Pearce Citation1990). Summary statistics of the rainfall and hydrological variables for the 54 events are presented in and . The 54 events in our study were caused by very different rainfall amounts (4.20–110.40 mm, mean = 27.76 mm) and showed large differences in antecedent discharge (Qb = 0.03–0.73 m3 s-1), 5-day antecedent rainfall (0–107.80 mm) and antecedent rainfall index (4.85–132.34 mm), suggesting a wide range of catchment moisture conditions. Owing to the diversity of rainfall events and prior hydrological conditions, observed hydrological responses were also variable. The runoff coefficients were low, less than 3% for most events and only 19% for an event with a total precipitation of 71.60 mm in 58 h, after 215 mm in the 15 preceding days (event of 7–13 December 2006). This is probably due to the high soil infiltration capacity, interception (about 65% of catchment is covered by forest) and the lack of anthropogenic influences such as soil compaction (<4% of the catchment is dedicated to cultivated land).
Table 4 Statistic summary of the main characteristics of rainfall–runoff events
The direct runoff percentage varied between 5 and 66% of the total flow of each event with a mean of 28%, and it was higher during the wet season. In contrast, direct runoff was generally below 20% of the total runoff volume during spring and summer. In our study catchment, as in the case of many forested and well-vegetated catchments, only a fraction of the direct runoff can be classified as stream interception and overland flow, since much direct runoff from catchments like this is subsurface flow (Hewlett and Hibbert Citation1967).
The relationship between rainfall and runoff is shown in . Note that, at the event scale, the relationship is significant (r 2 = 0.49, p < 0.01), despite the data scatter, especially for high rainfall. Thus, within the study period, similar runoff amounts (0.52 mm) were generated by two different rainfall events of 22.40 and 39.20 mm. Conversely, a 63.40 mm rainfall event generated 3.33 mm of runoff, whereas one of 98.40 mm only produced 1.54 mm. includes one point that did not fit the general trend, which is highlighted in grey. This point represents abundant runoff produced during floods. If this point is removed, the trend line r 2 value is 0.69 (p < 0.01), i.e. it is in the same order of magnitude as that reported by Varela (Citation2002) for a set of 22 events in the River Carballas catchment (also located in Galicia) and that referred to by Jordan (Citation1994) in the forest Haute-Mentue catchment (Switzerland) under humid temperate climate, for rainfall events of more than 10 mm.
Fig. 5 Relationship between rainfall and runoff amount at the event scale. The straight line shows the linear relationship between the two variables.
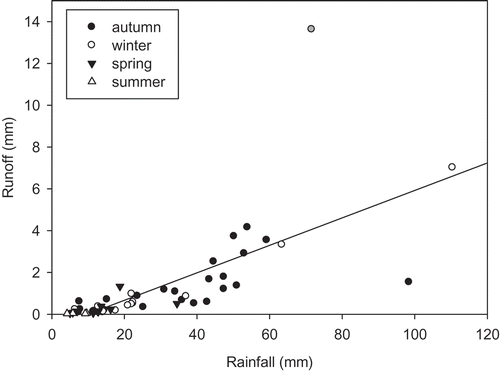
In the relationship between rainfall amount and runoff, two different trends can be identified: for small rainfall events (P < 15 mm), runoff response was limited (R < 0.25 mm), whereas for large rainfall events, a significant increase in runoff was observed. However, in the Corbeira catchment, the stream response is less marked than in other areas, where a clear threshold between small and large events exists (e.g. Jordan et al. Citation1994).
When the distinction between seasons was considered, the linear relationship between rainfall and runoff improved notably for winter events (r 2 = 0.96, p < 0.01), suggesting that, during this time of year, the hydrological response of the catchment is strongly influenced by rainfall magnitude. In autumn, when the highest number of runoff events is registered and the number of large rainfall episodes is greater than in the other seasons (12 out of 28 episodes with P > 30 mm), runoff is more variable (r 2 = 0.32, p < 0.01). In spring, both the rainfall and runoff decreased significantly in relation to the wet season (autumn and winter). In fact, only one episode recorded rainfall and runoff above 16 and 0.50 mm, respectively. The relationship between rainfall and runoff, although significant (r 2 = 0.74, p < 0.01), shows a less pronounced slope, indicating that, for a given rainfall event, runoff will be lower than in the wet season. Finally, in summer, the catchment response is limited, as demonstrated both by the reduced number of rainfall–runoff events (five) and by the low runoff generated (R < 0.06 mm). In addition, it is independent of rainfall, since the relationship between rainfall and runoff was not significant in summer.
The mean value of runoff coefficient of events was 3%, which implies that most of the rainfall infiltrates into the soil. The runoff coefficient ranged from 0.3 to 19%, 78% of the events showing runoff coefficients of less than 5%. The runoff coefficient only exceeded 10% on one occasion, corresponding to a floods period. A similar result was found by Alvarez et al. (Citation2001), who analysed the hydrological response in a small agroforestry catchment in Galicia. They found that only heavy rainfall events (P > 35 mm) produced runoff coefficients higher than 5%, and runoff coefficients of 15% were reached only exceptionally.
The seasonal evolution of runoff coefficient for the 54 events recorded throughout the year is shown in . The lowest values were obtained in summer (when the runoff coefficient never exceeded 1%) and the highest at the end of autumn and winter. Many authors have described a similar seasonal pattern for other humid catchments (Hewlett and Hibbert Citation1967). For instance, Hewlett and Hibbert (Citation1967) observed that runoff coefficients tended to be significantly higher in winter than in summer due to the higher contribution of summer rainfall to soil-moisture recharge and to the high evapotranspiration in this season.
In the Corbeira catchment, several factors could be involved in the temporal variability of the runoff coefficients of the events. During the summer period there is a relative reduction in rainfall. Mean rainfall between May and September in the three hydrological years studied amounted to 192 mm, which is less than 20% of annual rainfall. This decrease in rainfall, coupled with greater evapotranspiration (about 65% of the 620 mm of annual ETP are recorded in these five months) and water extraction by the vegetation, leaves the soil with a lower water reserve in summer, as shown in . Under these circumstances, the catchment has a reduced capacity to cause runoff during rainfall events, even when these are generated by rainfall amounts similar to those during wet periods. Thus, it has been shown that a rainfall event of 34 mm recorded in spring resulted in a runoff coefficient of only 1%, while rainfall events of the same order occurring in mid-autumn were generally associated with runoff coefficients higher than 4%. At the beginning of the hydrological year, the response is also limited, even when rainfall amounts are relatively high, because part of the rainfall is used to recharge the soil water reserves. From it is clear that, as the catchment becomes wet, the hydrological response increases and, consequently, it represents a greater percentage of rainfall. Probably the antecedent soil moisture state plays an important role in the hydrological response of the catchment, as has been often suggested for temperate humid catchments (Dunne et al. Citation1975, Pfister et al. Citation2002, Merz et al; Citation2006, Norbiato et al. Citation2009, Penna et al. Citation2011). However, over the study period, the observed seasonality of the runoff coefficients was also possibly affected by rainfall characteristics, such as large and long rainfall events occurring in 2006.
To identify factors that might explain the variability of runoff coefficients, these were compared with runoff, rainfall, maximum 10-min rainfall intensity and baseflow at the start of the events. The results are depicted in .
Fig. 7 Relationship between runoff coefficient and (a) runoff, (b) rainfall, (c) maximum rainfall intensity and (d) baseflow at the start of the events.
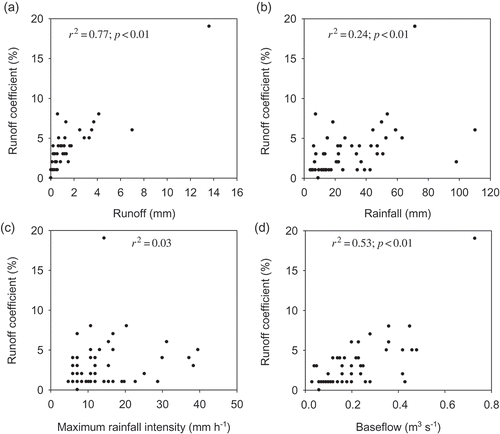
The runoff coefficient showed a significant relationship with runoff, as expected, suggesting a positive trend between these two variables, which explained 77% of the variance. The increase in runoff coefficient with runoff was subject to some scattering; for example, runoff coefficients around 8% were associated with runoff of 0.61 and 4.16 mm. Conversely, runoff around 0.5 mm led to runoff coefficients of 1 and 8%. These results reflect the nonlinearity of the rainfall–runoff processes in the Corbeira catchment. This nonlinearity is further evident in the relationship between runoff coefficient and rainfall. The linear fit of runoff coefficient on rainfall was significant (p < 0.01), but only explained 24% of the variance. During the study period, similar runoff coefficients (about 3%) were generated by 7.8 and 34.0 mm of rainfall. The absence of a strong relationship between rainfall and runoff coefficient shows the role of other factors in controlling the magnitude of the hydrological response.
In general, there is a strong consensus among many authors to note the negligible effect of rainfall intensity on well-vegetated catchments (Hewlett et al. Citation1977, Serrano Muela et al. Citation2008), although sometimes the rainfall intensity appears slightly correlated with the peak discharge. The results from our study confirm this assessment, since the regression analysis of runoff coefficient against maximum rainfall intensity (10 min) was not statistically significant ().
Finally, the linear regression of runoff coefficient against baseflow at the start of the event (considered as an indicator of the wetness of the catchment) reveals a relatively strong positive relationship between the two variables, explaining 53% of the variance of runoff coefficient. However, the scatter was relatively high, since similar runoff coefficients (around 3%) were associated with baseflow varying from 0.05 to 0.24 m3 s-1 ().
Although neither of these relationships is particularly strong, the above results showed that baseflow variability appears to be more influential than rainfall amount in controlling the hydrological response of the Corbeira catchment, as observed in many temperate humid catchments (e.g. Cappus Citation1960, Jordan Citation1994). However, the values of baseflow cannot, by themselves, sufficiently explain the runoff coefficients in this catchment, suggesting the combined influence of baseflow and rainfall amount on the observed runoff coefficients.
Initial conditions are not always found to closely drive the runoff response. There are examples in the literature where runoff volumes seem to be insensitive to antecedent soil moisture conditions and they are controlled mainly by event rainfall. An example is the study of Nadal-Romero et al. (Citation2008), who analysed the runoff response of a mountainous experimental catchment affected by gully dynamics in the central Pyrenees (Spain). They suggested that the lack of sensitivity of runoff response to soil moisture is related to the role of the badland areas in runoff generation. Scherrer et al. (Citation2007) argued that the sensitivity of runoff coefficient on antecedent soil moisture depends on the dominant runoff process. Hortonian overland flow is hardly affected, while catchments dominated by subsurface flow react very sensitively to antecedent wetness. However, it is probable that the initial conditions affect runoff volume for most runoff generation types: (a) in Hortonian runoff by reducing infiltration capacity, (b) in saturation excess runoff by expanding contributing areas, and (c) in macropore flow and subsurface runoff by connecting preferential flow paths (Zehe and Blöschl Citation2004).
For a more detailed analysis of the influence of the different variables on the magnitude of the hydrological response, a correlation analysis was performed with runoff coefficient and discharge increment (defined as the difference between the discharge peak and the discharge at the start of the event) measured for each event. Two groups were differentiated based on the rainfall associated with the events. A rainfall threshold of 15 mm was chosen, as this corresponds to the change in the rainfall–runoff relationship observed in . The results listed in reveal that the correlation between runoff coefficient and rainfall was only significant for large events (rainfall > 15 mm). In contrast, the correlation between runoff coefficient and baseflow and antecedent rainfall index was always significant. This suggests that, for small events, the response of the catchment depends mainly on baseflow at the start of the event and antecedent rainfall index, while, for large events, both antecedent conditions (baseflow and antecedent rainfall index) and rainfall amount affect the magnitude of the response. These results are similar to those derived by Hewlett et al. (Citation1977) for the Coweeta catchment, especially with respect to the rainfall. However, baseflow appears to be more significant for the Corbeira catchment, as occurs in the catchments studied by Jordan (Citation1994) in Switzerland. Likewise, García-Ruíz et al. (Citation2008), studying the hydrological response in an experimental forested catchment (San Salvador) in the central Pyrenees, also concluded that the main factors that explain the hydrological response are antecedent rainfall and baseflow, while rainfall amount and intensity only became important when the water table was close to the surface.
Table 5 Results from the Pearson correlation coefficients between runoff coefficients (RC), increase in discharge (ΔQ) and different variables for the observed rainfall–runoff events
Considering the increase in discharge, significant correlations were found with rainfall, antecedent rainfall index and baseflow for the two groups of events (). The correlation between rainfall and discharge increment was stronger than the correlation with runoff coefficient. In addition, for larger events, maximum rainfall intensity was unexpectedly correlated with the discharge increment. The influence of rainfall amount on the discharge increment is in concordance with the results described in other humid temperate catchments (Hewlett et al. Citation1977, Jordan et al. Citation1994), whereas the effect of rainfall intensity on the discharge increment is usually observed in Mediterranean and semi-arid areas (e.g. Martínez-Mena et al. Citation1998, Nadal-Romero et al. Citation2008), where infiltration excess runoff occurs in areas with low permeability and, on the contrary, has not been usually reported under wetter conditions.
To complete the analysis of the hydrological response in the Corbeira catchment, an analysis of the shape of the hydrographs was performed. It is well known that the duration of the hydrographs and the shape of their recession curves are an expression of the type of runoff, and give some insight into the hydrological processes operating in the catchment (Boughton and Freebairn Citation1985). Most of the events presented a steep ascending limb, narrow peak discharge and a gentle recession limb. This led to values of intensity index (IVI), on average, three times higher than the values of recession index (IVR). shows four examples of hydrographs for the Corbeira catchment with their corresponding rainfall distributions.
Fig. 8 Hydrographs and hyetographs for four selected events of contrasting magnitude within the Corbeira catchment. The dotted line indicates the separation between direct runoff and baseflow.
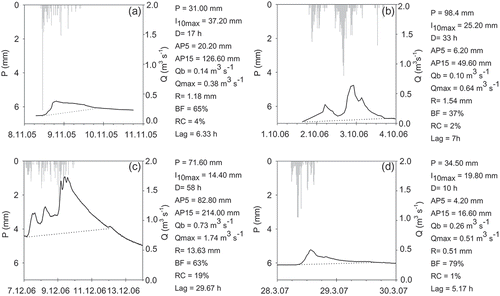
The first event (8–10 November 2005, ) followed a rainy period with more than 125 mm of rainfall during the previous 15 days. Under these circumstances, a 31.00-mm rainfall event of high intensity (37.20 mm h-1; rainfall intensities for the Galicia region are usually lower than 10 mm h-1) produced significant runoff (1.18 mm) and a significant runoff coefficient (4%). This event was characterized by a relatively small time lag in relation to the rainfall event, moderate reaction during the rising limb, absence of true peak flow, and slow recession limb.
The event of 28–30 March 2007 () was generated by a rainfall amount (34.50 mm) similar to that of the event described above, but under dry antecedent conditions (AP5 = 4.20 mm and AP15 = 16.60 mm). Under such dry conditions, the catchment yielded a peak discharge (0.51 m3 s-1) 1.3 times higher than the peak discharge of the 8–10 November 2005 event, but generated runoff (R = 0.51 mm) almost three-times lower than that event. Moreover, during this event, the catchment stored 99% percent of the rainfall, possibly due to the relatively low soil moisture state prior to the rainfall–runoff event.
These two events show the nonlinear effect of rainfall amount on runoff generation and the importance of antecedent moisture conditions in the hydrological response of the catchment: the same amount of rainfall can produce low runoff when the soil is relatively dry, but can result in large runoff response when the soil is already wet.
The event of 1–3 October 2006 () occurred at the beginning of the hydrological year following the low-discharge summer period; hence, the low antecedent rainfall (AP15 = 49.60 mm) and baseflow (Qb = 0.10 m3 s-1) recorded. This runoff event was generated by a high-magnitude rainfall event of 98.4 mm (an unusual event for this area) that caused a fast response of stream discharge, especially during the second peak discharge. In terms of volume, it showed a large proportion of direct runoff (63% of total) due to the very low baseflow, an unusual fact in this catchment and in the vast majority of the humid climate catchments (e.g. Hewlett and Hibbert Citation1967, Bonell et al. Citation1990). The high rainfall intensity (44 mm in 4 h) during the first hours of the second rainfall episode suggests that surface runoff during this period was most likely due to the infiltration excess mechanism. The high suspended sediment concentrations associated with this rainfall–runoff event (data not shown here) also seem to confirm the importance of the infiltration excess overland flow over impervious areas, and especially over unprotected croplands during this event. Although this event generated a large portion of direct runoff, the runoff coefficient was very low because a substantial part of the rainfall was diverted to soil water storage.
The last event (7–13 December 2006, ), indicated with a filled circle in , occurred after an extremely wet period (antecedent rainfall in the 15 days prior to the event of 214 mm); hence, the high baseflow (0.73 m3 s-1) at the beginning of the rainfall event. The rainfall pattern is characteristic of the rainfall that causes floods in Galicia, i.e. rainfall of long duration, significant rainfall amount and low intensity (Rodríguez Martínez-Conde et al. Citation2000). Under these wet conditions, the stream response was higher and steeper than for other events and presented a slow recession limb. Moreover, the stream response was immediate and the shape of the hydrograph is similar to that of the rainfall distribution. Considering that the rainfall intensity does not exceed the infiltration capacity, and that it was lower during this event than in other events, it is surprising that discharge presents a similar distribution to that of rainfall. The direct rainfall onto saturated areas, a small portion of the drainage catchment, namely, in the lower part of the catchment, could explain the similarity between the hyetograph and hydrograph. The high runoff (R = 13.63 mm) and the runoff coefficient (RC = 19%) associated with this event could confirm these findings. In any case, the long discharge recession limb, indicative of a prolonged response after the rainfall event, and the long time lag imply that subsurface flow is also the predominant mechanism for this particular event. Finally, it is also noted that, in terms of volume, this runoff event was one of the highest recorded at the outlet of the Corbeira catchment in recent years.
The shape of the hydrographs in the Corbeira catchment, characterized by a quick increase and slow recession, suggests that the subsurface flow is the main process explaining the discharge response of the catchment to a rainfall event. The importance of subsurface runoff in the generation of runoff in humid areas has been well documented in the southern Appalachians by Hewlett et al. (Citation1967) at the Coweeta Forest Experiment Station. This process also dominates hydrographs in Galicia, as has been observed in other studies carried out in this area (Varela Citation2002).
CONCLUSIONS
The study conducted in the Corbeira catchment in northwest Spain, to examine the hydrological response in an agroforestry catchment under temperate humid climate, revealed, on the one hand, that baseflow dominated the stream response and, on the other hand, that there was not a linear relationship between rainfall amount and runoff at the event scale.
The analysis of the relationship between stream response and other hydrological variables pointed out that antecedent moisture conditions (indirectly deduced from the baseflow at the beginning of each rainfall event) and rainfall amount are the key variables in terms of runoff generation. Seasonal variability of the runoff coefficients can be observed, and the runoff coefficients tend to be high in autumn and winter when soil moisture is high, while in summer, when the catchment is dryer, the runoff coefficients tend to be lower. The rainfall seasonality influenced the distribution of runoff coefficients, not only through the characteristics of the runoff-generating rainfall events, but also by controlling the variability of the initial conditions.
The shape of the hydrographs, with a quick increase and slow recession, suggested that the subsurface flow was probably the dominant process explaining the discharge response of the catchment to rainfall events.
Acknowledgements
This investigation was carried out within the projects REN2003-08143 funded by the Spanish Ministry of Education and Science and PGIDIT05RAG10303PR financed by the Xunta of Galicia. The first author was awarded a research contract/grant (Ángeles Alvariño Programme) funded by the Xunta de Galicia. The authors would also like to acknowledge the helpful comments made by J. Parajka and another anonymous reviewer in an earlier version of this paper.
REFERENCES
- Alvarez , M. , Varela , C. , Soto , B. , López , E. and Díaz-Fierros , F. 2001 . “ Análisis de la respuesta hidrológica en una cuenca fluvial y su relación con la precipitación ” . In Avances sobre el estudio de la erosión hídrica II , Edited by: Paz-González , A. and Taboada-Castro , M.T. 21 – 32 . Coruña , , Spain : Universidade da Coruña .
- Blume , T. , Zehe , E. and Bronstert , A. 2007 . Rainfall runoff response, event-based runoff coefficients and hydrograph separation . Hydrological Sciences Journal , 52 ( 5 ) : 843 – 862 .
- Bonell , M. , Pearce , A.J. and Steward , M.K. 1990 . The identification of runoff-production mechanisms using environmental isotopes in a tussock grassland catchment, Eastern Otago, New Zealand . Hydrological Processes , 1 : 15 – 34 .
- Boughton , W.C. and Freebairn , D.M. 1985 . Hydrograph recession characteristics of some small agricultural catchments . Australian Journal of Soil Research , 23 : 373 – 382 .
- Burns , D. 2005 . Effects of suburban development on runoff generation in the Croton River basin, New York, USA . Journal of Hydrology , 311 : 266 – 281 .
- Cappus , P. 1960 . Bassin expérimental d'Álrance. Etude des lois de l´écoulement. Application au calcul et à la prevision des debits . La Houille Blanche A , : 493 – 500 .
- Cerdan , O. 2004 . Scale effect on runoff from experimental plots to catchments in agricultural areas in Normandy . Journal of Hydrology , 299 : 4 – 14 .
- Dunne , T. and Leopold , L.B. 1978 . Water in environmental planning , New York : W.H. Freeman & Co .
- Dunne , T. , Moore , T.R. and Taylor , C.H. 1975 . Recognition and prediction of runoff-producing zones in humid regions . Hydrological Sciences Bulletin , XX : 305 – 327 .
- Endale , D.M. , Fisher , D.S. and Steiner , J.L. 2006 . Hydrology of a zero-order southern Piedmont watershed through 45 years of changing agricultural land use. Part 1. Monthly and seasonal rainfall–runoff relationships . Journal of Hydrology , 316 : 1 – 12 .
- FAO (Food and Agriculture Organization) . 2006 . World reference base for soil resources , Rome , , Italy : FAO World Soil Resources Reports 103 .
- Fernández , C. 2006 . Changes in water yield after a sequence of perturbations and forest management practices in an Eucalyptus globulus Labill. watershed in northern Spain . Forest and Ecological Management , 234 : 275 – 281 .
- García-Ruíz , J.M. 2008 . Flood generation and sediment transport in experimental catchments affected by land use changes in the central Pyrenees . Journal of Hydrology , 356 : 245 – 260 .
- Gravelius , H. 1914 . “ Flusskunde. Gosche'sche Verlagshandlung Berlin ” . In Morphometry of drainage basins , Edited by: Zavoianu , I. Amsterdam : Elsevier . 1985
- Gregory , K.J. and Walling , D.E. 1973 . Drainage basin form and processes. A geomorphological approach , London : Edwards Arnold .
- Guy , H.P. 1964 . “ An analysis of some storm-period variables affecting stream sediment transport ” . In US Geological Survey Professional Paper 462 , 1 – 46 . Washington , DC : US Government Printing Office .
- Hewlett , J.D. and Hibbert , A.R. 1967 . “ Factor affecting the response of small watersheds to precipitation in humid areas ” . In Forest hydrology , Edited by: Sopper , W.E. and Lull , H.W. New York : Pergamon .
- Hewlett , J.D. , Fortson , J.C. and Cunningham , G.B. 1977 . Effect of rainfall intensity on storm flow and peak discharge from forest land . Water Resources Research , 13 : 259 – 266 .
- Horton , R.E. 1932 . Drainage basin characteristics . Transactions of the American Geophysical Union , 13 : 350 – 361 .
- IGME (Instituto Tecnológico Geominero de España) . 1981 . Mapa geológico de España, 1:50 000 , Madrid : Servicio de Publicaciones del Ministerio de Industria y Energía . Hoja 45. Betanzos
- Jordan , J.P. 1994 . Spatial and temporal variability of stormflow generation processes on a Swiss catchment . Journal of Hydrology , 153 : 357 – 382 .
- Kirkby , M.J. 2005 . “ Organisation and process ” . In Encyclopedia of hydrological sciences , Edited by: Anderson , M.G. volume 1 , Chichester : Wiley .
- Latron , J. 2008 . Spatial and temporal variability of the hydrological response in a small Mediterranean research catchment (Vallcebre, eastern Pyrenees) . Hydrological Processes , 22 : 775 – 787 .
- Macrae , M.L. 2007 . Capturing temporal variability for estimates of annual hydrochemical export from a first-order agricultural catchment in southern Ontario, Canada . Hydrological Processes , 21 : 1651 – 1663 .
- MAFF (Ministry of Agriculture, Fisheries and Food) . 1991 . Code of good agricultural practices for the protection of water , London : MAFF .
- Martínez-Cortizas , A. , Castillo-Rodríguez , F. and Pérez-Alberti , A. 1994 . Factores que influyen en la precipitación y el balance de agua en Galicia . Boletin de la Asociación de Geológos Españoles , 18 : 79 – 96 .
- Martínez-Fernández , J. 2005 . Procesos hidrológicos en una cuenca forestal del Sistema Central: Cuenca experimental de Rinconada . Cuadernos de Invesigación Geográfica , 31 : 7 – 25 .
- Martínez-Mena , M. , Albaladejo , J. and Castillo , V. 1998 . Factors influencing surface runoff generation in a Mediterranean semiarid environment: Chicamo watershed, SE Spain . Hydrological Processes , 12 : 741 – 754 .
- Meyles , E. 2003 . Runoff generation in relation to soil moisture patterns in a small Dartmoor catchment, Southwest England . Hydrological Processes , 17 : 251 – 264 .
- Merz , R. and Blöschl , G. 2009 . A regional analysis of event runoff coefficients with respect to climate and catchment characteristics in Austria . Water Resources Research , 45 : W0145 doi: 10.1029/2008WR007163
- Merz , R. , Blöschl , G. and Parajka , J. 2006 . Spatio-temporal variability of event runoff coefficients . Journal of Hydrology , 331 : 591 – 604 .
- Mulholland , P.J. 1993 . Hydrometric and stream chemistry evidence of three storm flowpaths in Walker Branch Watershed . Journal of Hydrology , 15 : 291 – 316 .
- Nadal-Romero , E. 2008 . Temporal variability in hydrological response within a small catchment with badland areas, central Pyrenees . Hydrological Sciences Journal , 53 : 629 – 639 .
- Norbiato , D. 2009 . Controls on event runoff coefficients in the eastern Italian Alps . Journal of Hydrology , 375 : 312 – 325 .
- Pearce , A.J. 1990 . Streamflow generation processes: an Austral view . Water Resources Research , 26 : 3037 – 3047 .
- Penna , D. 2011 . The influence of soil moisture on the threshold runoff generation processes in an alpine headwater catchment . Hydrology and Earth System Sciences , 15 : 689 – 702 .
- Pfister , L. 2002 . Use of regionalized stormflow coefficients with a view to hydroclimatological hazard mapping . Hydrological Sciences Journal , 43 : 479 – 491 .
- Rial , M.E. , Alvarez , M.A. and Díaz-Fierros , F. 2005 . “ Water balance and observed flows in the Anllóns river basin (NW Spain) ” . In Fourth Inter-Celtic Colloquium on Hydrology and Management of Water Resources Guimaräes , , Portugal
- Rodríguez Martínez-Conde , R. 2000 . Las inundaciones recientes en Galicia . Serie Geográfica , 9 : 187 – 210 .
- Romero-Díaz , M.A. and López-Bermúdez , F. 1989 . “ Avenidas fluviales e inundaciones en la cuenca del Mediterráneo. Relación entre escorrentías superficiales y características físicas y ambientales en pequeñas cuencas fluviales (Alto Guadalquivir) ” . Alicante : Instituto Universitario de Geografía de la Universidad de Alicante. Caja de Ahorros del Mediterráneo .
- Scherrer , S. 2007 . Formation of runoff at the hillslope scale during intense precipitation . Hydrology and Earth System Sciences , 11 : 907 – 922 .
- Serrano-Muela , M.P. 2008 . Forest and their hydrological effects in Mediterranean mountains . Mountain Research and Development , 28 : 279 – 295 .
- Smith , M. 1991 . Report on the expert consultation on procedures for revision of FAO methodologies for cropwater requirements , Rome : FAO, Land and Water Development Division .
- Soto , B. 2005 . “ Influence of 7-year old Eucalyptus globulus plantation in the low flow of a small basin ” . In Fourth inter-Celtic Colloquium on Hydrology and Management of Water Resources Guimarâes , , Portugal
- Strahler , A.N. 1964 . “ Quantitative geomorphology of drainage basins and channel networks ” . In Handbook of applied hydrology , New York : McGraw-Hill . Section 4-II
- Varela , C. 2002 . Control, diagnosis y prevención de la contaminación difusa en una cuenca agraria , Spain : Tesis Doctoral. Universidad de Santiago de Compostela .
- Viessman , W. , Lewis , G.L. and Knapp , J.W. 1989 . Introduction to hydrology , New York : Harper-Collins .
- WMO (World Meteorological Organization) . 1980 . Manual on stream gauging fieldwork , volume I , Geneva : WMO, Operational. Hydrology Report no.13 .
- Zehe , E. and Blöschl , G. 2004 . Predictability of hydrological response at the plot and catchment scale: role of initial conditions . Water Resources Research , 40 : W10202 doi: 10.1029/2003WR002869