Abstract
Water resource use limits ensure protection of environmental values and define the availability and reliability of water supply for out-of-channel use. We examined how three types of scientific tools (environmental flow setting methods, hydrological analyses for setting total allocations and spatial frameworks) have been used to define limits across jurisdictional regions comprising multiple catchments in New Zealand. We found that recently developed minimum flow and total allocation setting tools are widely used. Spatial frameworks are increasingly used to discriminate and account for variation in environmental characteristics, thereby increasing the specificity of water resource use limits. The uptake of scientific tools has enabled improvements in the clarity of water management objectives and the transparency of limits defined by regional water management plans. We argue that more integrated use of scientific tools could improve the clarity and transparency of regional limits by explicitly demonstrating the trade-off between out-of-channel use and protection of environmental values.
Editor D. Koutsoyiannis; Guest editor M. Acreman
Citation Snelder, T.H., Rouse, H.L., Franklin, P.A., Booker, D.J., Norton, N., and Diettrich, J., 2014. The role of science in setting water resource use limits: case studies from New Zealand. Hydrological Sciences Journal, 59 (3–4), 844–859.
Résumé
Les limites dans l’utilisation des ressources en eau permettent d’assurer une protection de la valeur environnementale, et de définir la disponibilité et la fiabilité de l’alimentation en eau pour une utilisation hors du cours d’eau. Nous avons examiné comment trois types d’outils scientifiques (méthodes de détermination du débit environnemental, analyses hydrologiques pour fixer les allocations totales, et cadres spatiaux) ont été utilisés pour définir des limites entre des régions administratives comprenant plusieurs bassins versants en Nouvelle-Zélande. Nous avons constaté que les outils récemment mis au point pour la détermination du débit minimum et de l’allocation totale sont largement utilisés. Les cadres spatiaux sont de plus en plus utilisés pour discriminer et rendre compte des variations des caractéristiques environnementales, augmentant de ce fait la spécificité de limites d’utilisation des ressources en eau. L’adoption d’outils scientifiques a permis d’améliorer la clarté des objectifs de gestion de l’eau et la transparence des limites définies par les plans régionaux de gestion de l’eau. Nous soutenons que l’utilisation plus intégrée d’outils scientifiques pourrait améliorer la clarté et la transparence des limites régionales, en démontrant explicitement le compromis entre l’utilisation hors du cours d’eau et la protection de la valeur environnementale.
INTRODUCTION
Out-of-channel uses of stream and river water for human, agricultural and industrial use and power production are increasing globally, threatening both environmental values and security of human water use (Vörösmarty et al. Citation2010). Internationally, there has been considerable attention given to defining environmental flows: the quantity, timing and quality of water flows required to sustain freshwater and estuarine ecosystems and the benefits they provide to people (Poff et al. Citation2010). However, principles for effective water management, such as those set out for Integrated Water Resources Management (IWRM), include the consideration of social and economic goals (Biswas Citation2004). Managing the conflicting demand for water therefore requires the definition of water resource use limits to protect both environmental values (i.e. ecological, recreation, aesthetic and cultural) and to allocate reliable water to out-of-channel use (Gleick Citation1998, OECD Citation2008). In this paper, we define water resource use limits as regulatory criteria that determine how water resources are allocated to environmental flows and out-of-channel use. Water resource use limits are used to manage the consequences of competing demands for water for both the instream environmental values and out-of-channel uses.
The process of setting water resource use limits includes balancing different sets of values and is inherently normative (i.e. involves value judgements). Principles of water resources management suggest that limit setting needs to: consider and define environmental, economic and social objectives; use scientific knowledge to assess options; and involve stakeholder participation to establish the legitimacy of resulting management actions and regulatory criteria (Biswas Citation2004). The failure to establish effective limits has led to overallocation of water in many areas worldwide, resulting in environmental degradation and reduction in reliability of water for existing users (Postel and Carpenter Citation1997, Dudgeon et al. Citation2006). As a consequence, managers in some areas are now faced with the significant challenge of trying to “claw back” allocated water to restore ecologically sustainable river flows and to achieve an acceptable reliability for out-of-channel use (e.g. Arthington et al. Citation2006, Richter et al. Citation2006).
Ideally, water resource use limits are set strategically (i.e. defined for all locations within jurisdictional regions, often comprising multiple catchments, and in advance of management actions such as licensing of water use). Strategic water resource use limits provide certainty about the environmental outcomes that are sought and future resource availability and reliability. If limits are not set strategically, decision-making occurs on a case-by-case basis for individual projects, resulting in uncertain consequences for both environmental values and water users. In addition, in the absence of limits, the ability to consider cumulative effects of multiple users is poor.
The establishment of water resource use limits has become a significant issue in New Zealand, where per capita demand for water is two to three times higher than most other OECD countries (MFE Citation2007). Demand is continuing to rise, with water allocation increasing nationally by approximately 50% between 1999 and 2006 (MFE Citation2006). The greatest demand is for irrigation, which accounts for 78% of the permitted water abstractions in New Zealand (MFE Citation2006).
In New Zealand, the Resource Management Act (RMA) provides the principal legislative framework for water management (Memon Citation1997, Pyle et al. Citation2001). Under the RMA, substantial authority for water management decision-making is devolved to 16 regional councils (). One of the key regulatory mechanisms that a regional council can employ to manage water resources is a regional water management plan (RWMP). RWMPs set objectives for management and specify regulatory criteria that define how regional water resources can be used. In addition, RWMPs define the circumstances in which licences to take or use water are required, and the likely conditions that will be imposed on water users (Memon Citation1997, Snelder and Hughey Citation2005).
The Organisation for Economic Co-operation and Development’s review of New Zealand’s environmental performance (OECD Citation1996) criticized RWMP objectives, saying that ambiguity led to a lack of accountability by management agencies, both in terms of environmental outcomes and for the licensing of resource use. A subsequent review concluded that there remains a need to strengthen regulatory control of out-of-channel use of water (OECD Citation2007). Government reviews of RWMPs (Pyle et al. Citation2001, OCAG Citation2005) also found that the plans were often lengthy and confusing, but neither sufficiently specific nor clear. The need for clear (i.e. unambiguous and measurable) water resource use limits has now been recognized in New Zealand. In Citation2011, a national policy was introduced requiring councils to use RWMPs to set limits for all waterbodies in the country (New Zealand Government Citation2011). A significant technical challenge for setting regional water resource use limits is providing and using appropriate scientific information on which to base the decision-making process. These challenges are also likely to occur in other countries where increasing population and/or changes in farming practices are driving a rapid expansion of water resource exploitation.
In this article, we describe how scientific tools are being used to define water resource use limits at the regional level in New Zealand. We first describe three types of scientific tools that have been applied in New Zealand to support the process of setting water resource use limits (environmental flow setting methods, hydrological analyses for setting total allocation and spatial frameworks). We then discuss the concept of limits in water resources management and how water resource use limits combine to determine consequences for both environmental values and out-of-channel uses. We also discuss the importance of limits being associated with clear objectives. We then examine RWMPs in New Zealand and demonstrate how the use of scientific tools has increased the clarity of objectives, and the transparency and specificity of the limits that RWMPs define. Finally, we offer suggestions for further improving the process of setting effective regional water resource use limits.
SCIENTIFIC TOOLS USED FOR SETTING LIMITS IN NEW ZEALAND
Methods for setting environmental flows
Globally, many environmental flow setting tools and methods have been developed, reflecting a range of paradigms for establishing the ecological flow requirements of rivers (Poff et al. Citation1997, Citation2010, Tharme Citation2003, Acreman and Dunbar Citation2004). These methods can be categorized along a spectrum according to the trade-off between data requirements and effort involved in their application versus the specificity and rigour of their underlying analysis. At one end of this spectrum are easily applied “reconnaissance-level” methods for setting minimum flows (sensu Tharme Citation2003). Many reconnaissance-level methods are based on hydrological indices. For example, minimum flows may be defined based on hydrological indices such as the mean annual low flow (MALF) and exceedence percentiles, e.g. Q95 (Tharme Citation2003). These methods are not directed at protecting specific ecological values or processes, but are based on the assumption that the status quo will be maintained if flows are not reduced below historic natural low flows. The advantage of reconnaissance-level methods is that, provided observations or estimations of natural flows are available, they are easily applied (Acreman Citation2005). However, because they are generally not focused on particular environmental values, they do not allow the objective of the environmental flow to be clearly expressed. An additional limitation of these methods is that they do not account for variation in the relationship between flow and protection of environmental values between locations. Snelder et al. (Citation2011) showed that minimum flows based on a proportion of MALF had variable consequences for the protection of hydraulic habitat across New Zealand rivers. Minimum flows based on 80% or 90% of MALF were overly restrictive in some circumstances, but insufficiently protective in others.
At the other end of the spectrum are methods based on site-specific measurements, analysis and modelling of particular aspects of a system, for example, hydraulic habitat (Nehring and Anderson Citation1993), water quality (Gippel et al. Citation2002), or bio-energetics (Hayes et al. Citation2000, Booker et al. Citation2004). Typically, such studies produce a relationship between flow and the quantity and quality of some environmental state variable. An example is the physical habitat modelling component of the Instream Flow Incremental Methodology (IFIM), which describes environmental state in terms of the hydraulic habitat of target species and evaluates how this changes at a location as a function of flow (e.g. PHABSIM; Bovee et al. Citation1998). Such relationships provide one rationale for defining environmental flows (especially minimum flows).
A key benefit of methodologies such as physical habitat modelling is that they enable the objectives of environmental flows to be clearly expressed in terms of the quantity of habitat for relevant species at a given location. There has been considerable effort in New Zealand to develop microhabitat-suitability criteria for both native and non-native species, which are a key model input (e.g. Jowett and Richardson Citation1995). However, acceptance of the approach is not universal due to uncertainties in both habitat suitability indices and hydraulic models (Williams Citation1996), as well as criticisms of the underlying assumptions (e.g. Mathur et al. Citation1985, Booker and Dunbar Citation2004, Lancaster and Downes Citation2010).
An approach that sits midway along the spectrum of environmental flow setting methods is generalized instream habitat modelling (Lamouroux and Jowett Citation2005). These models have been developed by combining results from many individual habitat studies, and generalizing the relationship between flow and habitat for particular species in natural stream reaches based on reach-average hydraulic characteristics (Lamouroux and Jowett Citation2005, Lamouroux Citation2008). Generalized models require less data collection and therefore allow more rapid assessments of flow–habitat relationships for target species at a site than traditional habitat models, albeit with reduced certainty due to use of generalized relationships rather than site-specific data (Booker and Acreman Citation2007).
Hydrological analyses for defining total allocations
The total allocation defines the total quantity or rate at which water can be abstracted for out-of-channel use upstream of a point in a catchment. The key reasons for setting total allocations include minimizing the effects of abstraction on the low-flow regime, and specifying a reliability of supply for existing and potential out-of-channel uses (Lincoln Environmental Citation2000).
Simple methods for defining total allocations that are similar to reconnaissance-level approaches to setting minimum flows are based on hydrological indices such as MALF and the one-in-five-year low flow. Due to the spatial variation in flow regimes, using hydrological indices to set total allocation can result in spatially variable outcomes. Snelder et al. (Citation2011) showed that total allocations based on a proportion of MALF produce spatially variable patterns in reliability of water for out-of-channel use.
A more rigorous approach to setting total allocations for specific locations is based on hydrological analyses that simulate the effects of different allocation scenarios. Inputs are a time series of natural flows (e.g. daily mean flows), and rules for a specific scenario (e.g. minimum flow and total allocations). Outputs from the analysis are a time series of residual river flows and abstraction restrictions. The residual river flows time series can be used to assess environmental considerations, including the increase in the duration that flow is held at the minimum (“flat-lining”), or the reduction in the frequency and duration of high flows that may be required for environmental purposes, such as mobilization of sediment or removal of nuisance algal growth (Jowett and Biggs Citation2008). In addition, the abstraction restriction time series is assessed in terms of the acceptability of the frequency, duration and timing of restriction for water users (Jowett and Biggs Citation2008).
In terms of effort and rigour for defining total allocations, use of flow duration curves (FDCs) () may be considered to sit between the use of hydrological indices and hydrological time-series analysis. FDCs are derived by analysing a time series of flow data to represent the percentage of time that flows are equalled or exceeded at a given location (Vogel and Fennessey Citation1995). FDCs can be used to quantify the reliability of supply of a river water resource for any nominated minimum flow and total allocation (Snelder et al. Citation2011). The FDC approach has the advantage that it can be easily applied to estimate the overall reliability and the duration of flat-lining, given the minimum flow and total allocations. However, while defining limits on an FDC describes the overall risk of restriction and flat-lining, they provide no information about whether periods occur consecutively or are scattered in time (Acreman Citation2005). An important advantage of FDCs is that they can often be estimated for ungauged locations using regionalization techniques (Ganora et al. Citation2009). Empirical methods for estimating FDCs have recently been defined for all catchments in New Zealand to assist with water allocation decision-making (Booker and Snelder Citation2012).
Fig. 2 Flow duration curves (FDCs) for four REC classes (see for explanation of the class labels). The FDCs all express the percentage of time that flows (normalized by mean flow) are exceeded. Each FDC represents the mean of FDCs for several individual gauging stations (the number of gauges in each class is shown in parentheses on the plot legend).
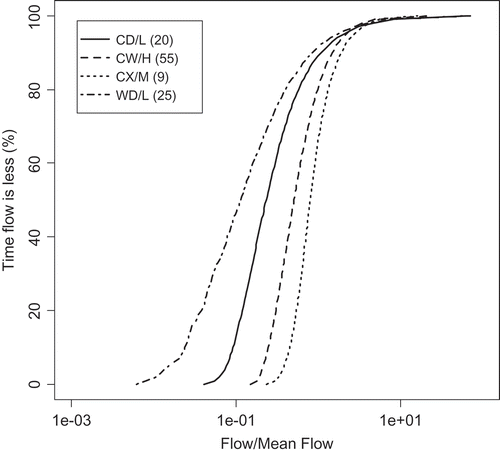
Spatial frameworks
Spatial frameworks can be described as systematic approaches to characterizing and mapping spatial variation in environmental (i.e. biotic and abiotic) characteristics. There are several approaches to defining spatial frameworks including hydrological and ecological regionalization (e.g. Omernik Citation1987, Harding and Winterbourn Citation1997), typologies and classifications (e.g. Kaika Citation2003), and species and community distribution models (e.g. Leathwick et al. Citation2005, Citation2011). Spatial frameworks are used in regional planning in three ways. First, they provide comprehensive inventories and stratifications of environmental characteristics (Omernik Citation1995). Second, spatial frameworks provide a basis for inferring characteristics of locations without data, thereby enabling the information derived from individual sites to be up-scaled (e.g. Dodds and Welch Citation2000). Third, spatial frameworks can be used to help identify management units, which are groups of locations that are likely to show similar responses to human activity or management actions and for which specific objectives and standards may apply (Omernik Citation1995, Snelder and Hughey Citation2005).
New Zealand encompasses large gradients in climatic, topographic and geological attributes, even at regional scales (Snelder and Biggs Citation2002). This environmental variation means rivers differ markedly with respect to their hydrological, geomorphic, water chemistry and ecological characteristics, and also their sensitivity to water resource development (Snelder et al. Citation2004a). Continued research has generated a strong appreciation of this variation with respect to hydrology (Pearson Citation1995), geomorphology (Booker Citation2010), fish (Crow et al. Citation2012), macro-invertebrate (Snelder et al. Citation2004b) and plant communities (Biggs Citation1995, Jowett and Richardson Citation2003, Leathwick et al. Citation2005), and the implications of this variability for large-scale management (Snelder and Hughey Citation2005).
Recognition of the need for spatial frameworks to support regional water resource management in New Zealand in the 1990s (Pyle et al. Citation2001) resulted in the development of a national classification of rivers known as the River Environment Classification (REC; Snelder and Biggs Citation2002). The REC comprises a digital representation of the New Zealand river network and classification system that are contained within a Geographic Information System. The river network is classified at six hierarchically organized levels of detail that stratify variation in hydrological, geomorphic, water chemistry and biological variation at a range of spatial scales. For example, the second level of the REC is referred to as Source-of-flow () and provides a broadscale hydrological regionalization that discriminates differences in hydrological characteristics such as the shape of FDCs (). The class of any network section is denoted by the combination of categories that describe the climate and topography of the watershed; for example, rivers located in the south-western coast of New Zealand () tend to be categorized as Cool-extremely wet climate and Mountain topography and, thus, belong to the CX/M Source-of-flow class ().
Table 1 Summary of the defining characteristics, categories and category membership criteria for the REC Source-of-flow classes included in this study.
Subsequent research has produced several more specific spatial frameworks that are based on the REC network and its attributes. Woods et al. (Citation2006) provided estimates of mean flow for each segment of the REC network. Leathwick et al. (Citation2005) used available biological data to produce comprehensive predictions of the distributions of fish species, and Leathwick et al. (Citation2011) produced predictions of the distribution of fish and invertebrate communities.
LIMITS IN WATER RESOURCE MANAGEMENT
Limits to river water resource use are typically applied for two reasons. First, limits are imposed to constrain human-induced alteration of river flows to levels that are judged to be sufficient to sustain environmental values (Richter et al. Citation2006, Acreman et al. Citation2008, Poff et al. Citation2010). Second, economic considerations require that limits on water resource use are specified so that the total availability of water resources and their reliability is quantified and understood.
In situations where environmental values are high or where water resource development is intense, for example, where there are facilities for significant water storage, detailed studies are commensurate with the environmental impact and scale of the resource use are required to inform the limit-setting processes. However, the more common situation is where there are multiple small run-of-river abstractions that are spread throughout a region with little, if any, facility for water storage. Environmental effects in this situation arise primarily due to the cumulative aggregation of abstractions, which can result in increased frequency and duration of low flows, but usually have a negligible impact on higher flows (Nilsson and Renöfält Citation2008). In these situations, the impact of abstraction can be controlled by defining two types of limit: minimum flow (the flow at which abstractions must cease) and total allocation (the total volume or rate at which water can be abstracted).
Broadly, higher minimum flows increase the protection of environmental values, but decrease the reliability of water resources available for out-of-channel uses. Higher total allocation increases the availability of water resources for out-of-channel use, but also reduces the reliability of that supply (i.e. the proportion of time that a river can supply the total allocation). Higher total allocation also increases the duration of low flows and decreases the frequency and magnitude of high flows, with subsequent potential impacts on environmental values. The minimum flow and total allocation interact with each other and the flow regime to determine the consequences for environmental protection (i.e. the magnitude and duration of low flows) and out-of-channel uses (i.e. the proportion of the time abstractions are partially and fully restricted). Because of these interactions, limits are only effective, and the consequences of those limits for instream values and out-of-channel use can only be established, if both minimum flow and total allocation are defined.
An example of how minimum flow and total allocation interact with the flow regime to determine consequences for instream and out-of-channel use at a site is illustrated in with reference to regulatory limits in New Zealand. Interim minimum flow and total allocations (i.e. to apply in the absence of RWMPs or other regulations) were proposed by the New Zealand Government in 2008 as part of a proposed National Environmental Standard (hereafter, proposed NES; MFE Citation2008). The proposed NES suggested a reconnaissance-level method be used for setting minimum flows based on proportions of MALF. Proportions of MALF of 90% and 80% were nominated, based on the opinions of experts, for small and large rivers (defined in the proposed NES as mean flows of less than and greater than 5 m3 s-1), respectively. The proposed NES also suggested that total allocations be 30% and 50% of MALF for small and large rivers, respectively (MFE Citation2008).
Fig. 3 Schematic diagram of aspects of limit setting based on FDC and flow–habitat relationship. The two plots are for a river with a mean flow of 11.7 m3 s-1 and a MALF of 2.6 m3 s-1. The top plot shows the flow–habitat relationship for adult brown trout estimated using generalized instream habitat models (Jowett et al. Citation2008) using the method described by Snelder et al. (Citation2011). The lower plot shows the FDC that was estimated using methods described by Booker and Snelder (Citation2012). The FDC shows the nominated minimum flow, management flow and proportion of the time that abstractions would be partially (G) and completely (E) restricted. Letters indicate key points that are referred to in the text.
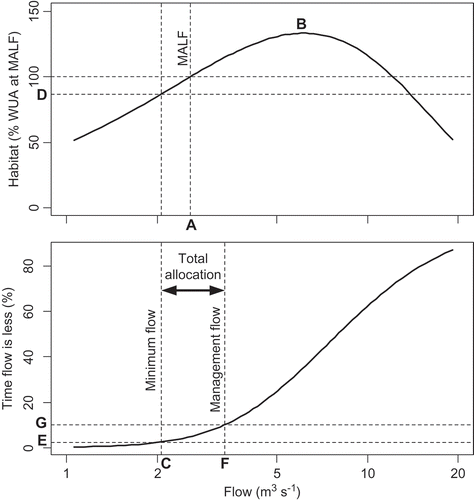
The top plot in shows how a measure of environmental state, in this case the availability of instream habitat for adult brown trout (Salmo trutta), varies with flow at a site. The mean flow at the site is 11.7 m3 s-1and the MALF is 2.6 m3 s-1 (Point A, ). The availability of suitable physical habitat for brown trout declines with both increasing and decreasing flow from an optimum at 6.2 m3 s-1 (Point B, ). The lower plot shows an FDC for the natural flow regime (i.e. the FDC that would have occurred if no abstractions were occurring).
Under the proposed NES, the minimum flow at the site would be 80% of MALF or 2.1 m3 s-1, (Point C, ), which equates to retention of 87% of the physical habitat available for adult brown trout at the MALF (Point D, ) (i.e. a decline of 13% relative to the amount of habitat available under average natural low-flow conditions). The minimum flow and the natural FDC can be used to define the proportion of time that complete restriction (cessation of abstractions) occurs. Complete restriction occurs for the proportion of time that the natural flow is at or below the minimum flow, equating to 2.4% of the time in this case (Point E, ).
The management flow is defined by adding the total allocation to the minimum flow, and is the flow below which partial restriction of abstractions would be required to maintain the river at the minimum flow. Under the proposed NES, total allocation at the site would be 50% of MALF or 1.3 m3s-1, resulting in a management flow of 3.4 m3 s-1 (Point F, ). Thus, when natural flows are below 3.4 m3 s-1 (i.e. 10.0% of the time, point G, ), the allowable abstraction is restricted to natural flow minus minimum flow. A consequence of this restriction is that residual flows (natural flows minus abstractions) are constant at the minimum flow (i.e. “flat-lining”) for the proportion of time that natural flows are between the management and minimum flows (i.e. between points G and E, ). Thus, in this example, the river would be flat-lined 7.6% of the time.
The example shows that the minimum flow and total allocation cannot be considered in isolation because they interact with each other, and the flow regime, to determine the environmental outcomes and availability and reliability of water for out-of-channel uses. The example demonstrates that the choice of limits involves a trade-off between instream and out-of-channel values. When simple reconnaissance-level methods such as the proposed NES are used to define limits, this trade-off is implicit, but may not be transparent. For example, choosing a lower minimum flow than the proposed NES would reduce protection of environmental values, but would increase reliability for out-of-channel use and vice versa. By considering a range of limits (i.e. minimum flows and total allocations) defined by differing proportions of MALF, a more complete range of potential outcomes can be generated for a site (). The range of limits and their consequences represent a “decision space”. If, for example, the objective was to maintain habitat change, reliability at the management flow and reliability at the minimum flow to ≤15%, ≥90% and ≥96%, respectively (areas enclosed by dashed outlines; ), the acceptable options for limits are defined by the grey shaded area in the bottom right panel (). Even within this more constrained set of options for limits, value judgments are required to define a final choice of limits. For example, options include: (a) maximize the volume of water available for allocation (minimum flow of 80% of MALF and total allocation of 50% of MALF); (b) maximize the reliability of allocated water (minimum flow of 70% of MALF and total allocation of 20% of MALF); or (c) maximize habitat protection (minimum flow of 90% of MALF and total allocation of 20% of MALF).
Fig. 4 “Decision space” diagrams for reliability of out-of-channel water use and adult brown trout habitat at a site for a range of options for limits (i.e. minimum flows and total allocations). Areas enclosed by dashed lines reflect the combinations of minimum flow and total allocation that meet defined objectives (i.e. reliability at the management flow and reliability at the minimum flow of ≥90% and ≥96% on top panel left and right, respectively) and that meet defined objectives for environmental state (i.e. a reduction of habitat available at MALF ≤15%, bottom left panel). The grey shaded area in the bottom right panel is the intersection of the three objectives and represents the combinations of minimum flow and total allocation that would satisfy all three objectives.
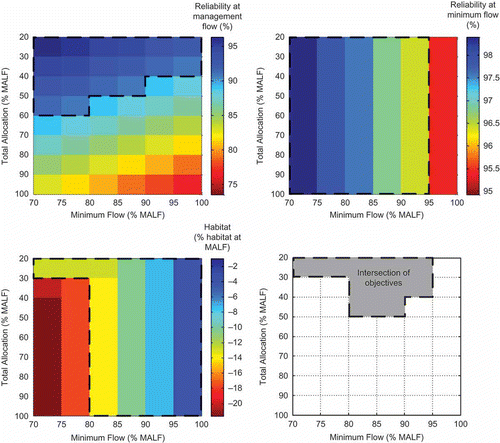
The example shows that, ideally, limits should be based on both environmental and water resource use objectives. If the objectives are clear (i.e. defined levels of protection for environmental values and availability and reliability of water for out-of-channel uses), decision-making is more transparent and limits are more easily justified. The role of scientific tools is to suggest options for objectives and to provide defensible criteria that will meet these objectives. A significant scientific challenge is the integration of the tools, so that the way minimum flows, total allocation and the flow regime interact is accounted for in defining a range of options for all objectives and associated limits.
When water resource development is intense, or the significance of environmental values is high, options for limits are often generated by detailed studies and analysis at catchment scales. However, this level of effort is often not justifiable when developing strategic water resource use limits over jurisdictional regions with sparse data, and where water resource use pressure is not high. In these situations, reconnaissance-level methods are often applied, with the disadvantage that objectives are unclear and the limits are not easily justified. Developing regional limits has the additional challenge of spatial variation in environmental characteristics. Spatial variation means that relevant objectives are likely to vary between catchments and, even in the absence of variable objectives, the use of a reconnaissance-level method is likely to produce limits with spatially variable consequences (Snelder et al. Citation2011). Spatial variation in environmental character means that, ideally, regional objectives and associated limits are spatially specific, i.e. applying to the particular set of circumstances in a geographically defined management unit (Snelder et al. Citation2004a, Snelder and Hughey Citation2005).
CASE STUDIES OF THE USE OF SCIENTIFIC TOOLS TO DEFINE REGIONAL WATER RESOURCE USE LIMITS IN NEW ZEALAND
The establishment of water resource use limits for all rivers has recently become a statutory requirement in New Zealand (New Zealand Government Citation2011). The legislative framework in New Zealand is inherently risk-based, and requires assessments of environmental effects to be scaled according to the environmental risk (Rouse and Norton Citation2010). The scientific basis for choosing appropriate tools for water allocation decision making can be linked to a risk-based framework (Acreman Citation2005). In this approach, methods are selected according to an assessment of the significance of the environmental values and the degree of hydrological alteration, the former representing “consequence” and the latter “likelihood of occurrence” as the key factors in a risk equation (). For example, in low-risk situations, i.e. both consequence and likelihood of adverse effects are low, the use of reconnaissance-level methods for setting minimum flows is justified. In situations where the risk is greater, a more resource-intensive and spatially specific assessment of the environmental flow requirements may be appropriate.
Fig. 5 Examples of methods used in the assessment of water resource use limits in relation to risk, as defined by the degree of hydrological alteration and significance of instream values (adapted from MFE Citation2008).
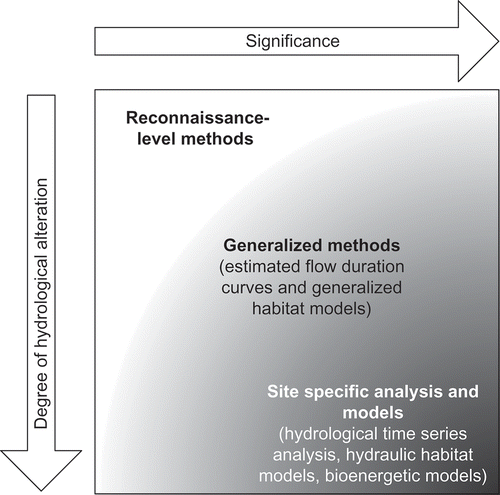
For water resource use projects in New Zealand where the potential environmental risk is high, such as large dams or diversions, environmental flows are generally developed using physical habitat modelling and other environmental flow setting methods (MFE Citation2008). However, the dominant water use comprises multiple spatially dispersed and small run-of-river abstractions spread throughout a region with little, if any, facility for water storage. Included in these abstractions may be river-linked groundwater abstractions, which are groundwater abstractions that cause immediate changes to river flow because of the high degree of hydraulic connection. Regional Water Management Plans (RWMPs) are the main mechanism for establishing strategic water resource use limits for these low-risk situations. RWMPs have the challenge of setting clear and transparent limits strategically (i.e. for all locations across jurisdictional regions and in advance of specific project proposals). We have therefore examined existing RWMPs to assess how limits were derived and the extent to which they define effective water resource use limits for these dominant water use situations.
We specifically assessed four aspects of the RWMPs. First, we assessed whether RWMPs set minimum flow and total allocations for all rivers in the region. We considered that regional limits were in place only if minimum flow and total allocations were specified for all rivers. Second, we assessed whether minimum flows and total allocations were transparently linked to clear objectives (i.e. unambiguous and measurable) for both environmental values and reliability of supply. Third, we assessed whether the limits were justifiable (i.e. derived using scientific assessments that are scaled appropriately to the level of environmental risk and in a replicable manner). We therefore looked for evidence whether the methods for setting minimum flows and total allocations had been chosen using a risk-based framework and whether tools had been integrated (used together) to quantify the consequences for environmental values and out-of-channel uses. Finally, we assessed whether the RWMP objectives and limits varied spatially. We looked for evidence of the use of spatial frameworks to define management units and whether RWMP objectives and limits varied by management units.
Our analysis used evidence from RWMP documents as well as available supporting documents such as technical reports and cost-benefit analyses that councils had undertaken to describe and justify their approach to regional water allocation. We also used evidence from other RWMP reviews (Lincoln Environmental Citation2000, OCAG Citation2005, SKM Citation2012). In some circumstances, councils have developed water management policies in documents other than RWMPs, including through the granting of individual water use licences. Our assessment focused on RWMP documentation and did not evaluate these additional documents. Our results are summarized in and are discussed below.
Table 2 Summary of review of early and current regional water plans, summarizing whether components of regional plans were generally absent (✗), sometimes present (✓) or generally present (✓✓).
Have RWMPs set minimum flows and total allocations for all rivers?
In the past, it was common for RWMPs to set minimum flows for rivers with high demand, but the definition of minimum flows for all rivers in a region was rare (Lincoln Environmental Citation2000). Total allocations were often not set in early RWMPs and few councils set total allocations for all rivers (Lincoln Environmental Citation2000). The examination of current RWMPs by SKM (Citation2012) found that of 16 plans, nine defined minimum flows and seven defined total allocations. However, only six current RWMPs defined limits (i.e. minimum flows and total allocations) for all rivers in the region.
Are minimum flows and total allocations linked to clear objectives for both environmental values and reliability of supply?
In early RWMPs, objectives were narrative statements such as “the avoidance of significant adverse effects on aquatic ecosystems” or “that people are able to take and use water for their social, economic and cultural wellbeing” (WRC Citation2004). These narrative objectives are often generic and, therefore, applicable to all waterbodies across a region. However, the disadvantage was that they were unclear (i.e. ambiguous and not measurable).
In current RWMPs, we found that objectives still tended to be narrative and unclear, but there was some increase in their transparency. For example, the Waikato region’s new RWMP contains an objective that is associated with reliability that states “there shall be no further allocation of water that results in a reduction in the benefits to be derived from the generation of renewable energy” (WRC Citation2012).
Current RWMPs also indicate that conscious decisions concerning the balance between environmental protection and water use have been made. However, these decisions were still generally not transparent. For example, the Waikato region’s current RWMP defines total allocations based on achieving reliability for out-of-channel uses. However, the total allocations are not linked to any objective in the RWMP that justifies a level of reliability or expresses a desired environmental outcome.
The Southland region’s RWMP had clear links between objectives and limits. To achieve this, the RWMP first specifies the critical environmental values for all locations (SRC Citation2010). The critical values are those having the highest flow requirements, which are highly valued (e.g. species with a threatened status) and that have a high probability of occurrence due to favourable habitat at the location. The RWMP then specifies objectives for protecting the critical values that are based on retaining a percentage of the habitat at MALF or a proportion of the maximum habitat if this occurs at a flow less than MALF. The RWMP also prescribes the methods to be used to determine minimum flows to protect these values.
Are the limits supported by scientific assessments?
Minimum flows in early RWMPs were generally defined using reconnaissance-level methods and, where RWMPs defined minimum flows for all rivers, this was always the case (Lincoln Environmental Citation2000). However, more detailed physical habitat modelling was used in the early RWMPs to derive minimum flows for specific catchments with high values. For example, the Manawatu-Wanganui region’s early RWMP used physical habitat modelling where target environmental values had been defined, while reconnaissance-level methods (i.e. percentage of MALF) were used for other rivers in the region (OCAG Citation2005). The early Waikato region’s RWMP also used a similar approach, with physical habitat modelling used for high-value rivers (e.g. trout fisheries), and a reconnaissance-level method based on the one-in-five-year low flow to set minimum flows for all other rivers in the region.
Current RWMPs have used a range of methods for setting minimum flows, but risk-based frameworks have been used to justify the approaches taken in different situations. For example, background documents supporting the Manawatu-Wanganui and Waikato regions’ current RWMPs refer to risk-based frameworks, with physical habitat modelling used to set minimum flows in higher-risk situations and reconnaissance-level methods used elsewhere (Hurndell et al. Citation2007, Environment Waikato Citation2008).
While total allocations were rarely set in the early RWMPs (Lincoln Environmental Citation2000), current RWMPs have frequently set total allocations and have used an integrated assessment of minimum flow and total allocations to quantify the consequences of limits for environmental values and out-of-channel uses. For example, the Manawatu-Wanganui region’s new RWMP defines total allocations for specific catchments that were derived from hydrological simulation analyses, which evaluated the reliability of supply under the proposed minimum flows (Hurndell et al. Citation2007). The approach taken by the Southland region’s RWMP has been to integrate minimum flow and total allocations based on the degree of hydrological alteration (i.e. based on risk) (SRC Citation2010). A default minimum flow of MALF applies when total abstractions are less than 10% of MALF. Where total abstraction is up to 30% of MALF, generalized habitat models are used to define minimum flows for the nominated critical species. In situations where hydrological alteration (i.e. total allocation) exceeds 30% of MALF, site-specific physical habitat modelling is used to define minimum flows for the critical species.
Are the objectives and limits location specific?
Early RWMPs made very limited use of spatial frameworks, but in current RWMPs spatial frameworks have been used to define management units and increase the specificity of objectives and limits. Four regions (Canterbury, Southland, West Coast and Manawatu-Wanganui regions) used the REC to provide spatial frameworks for their RWMPs (SKM Citation2012). For example, the Manawatu-Wanganui region’s current RWMP uses the REC as a spatial framework to develop water management zones (Hurndell et al. Citation2007, SKM Citation2012). Minimum flows are defined for the management zones based on specific values, and total allocations have frequently been defined based on hydrological analyses of flow records that are representative of the zone (Hurndell et al. Citation2007). The Southland region’s RWMP uses management units, based on the REC, to nominate the critical values to be used in establishing habitat–flow relationships for setting minimum flows. The critical values for each management unit were identified based on studies of species distributions (Jowett and Richardson Citation2003).
DISCUSSION
The definition of both minimum flows and total allocations are a minimum requirement for establishing effective limits for the dominant surface water resource use situation in New Zealand (i.e. multiple small spatially dispersed abstractions with little storage). We argue that setting limits involves balancing different sets of values and that, to establish the legitimacy of resulting management actions and regulatory criteria, the trade-offs need to be transparent. We have demonstrated a method for quantifying the trade-off between habitat at minimum flow and availability and reliability of water for out-of-channel use by integration of physical habitat models and FDC (). We have also described how a range of options can be presented so that the inherently normative nature of water management decisions can be made more transparent ().
We argue that, ideally, limits should be defined strategically (i.e. for all locations in jurisdictional regions and in advance of licensing water use), and that, to be accepted by stakeholders, the basis for water resource use limits must be transparent. Transparency is facilitated by objectives that clearly communicate the chosen level of protection for environmental values and the availability and reliability of water for out-of-channel uses. Furthermore, we argue that the limits (i.e. the minimum flow and total allocation) should clearly relate to these objectives and be based on scientific assessments.
The benefits of establishing water resource use limits include certainty for environmental protection and future out-of-channel resource availability and reliability of supply, and a basis for managing cumulative effects. A key risk associated with not setting limits to water use is overallocation. Overallocation has been identified as a risk in New Zealand, where some regional councils consider surface water resources to be overallocated, and others predict their water resources will be fully allocated within a few years (Aqualinc Citation2008). Overallocation is considered to have had detrimental environmental consequences and to be constraining economic opportunities (ME and MA Citation2009). The national policy introduced in 2011 also recognizes and defines overallocation and requires councils to take steps to “avoid any further overallocation of fresh water and phase out existing overallocation.” (New Zealand Government Citation2011, p. 8). An additional benefit of establishing clear objectives and limits is that it provides a basis for monitoring and future assessment of whether the objectives have been met (Holling Citation1980).
Regional water planning in New Zealand has struggled to establish clear objectives that are transparently linked to regulatory criteria (OECD Citation1996, Citation2007, Pyle et al. Citation2001, OCAG Citation2005). Many RWMPs still contain narrative objectives that are ambiguous and not measurable, and few RWMPs link their minimum river flow and total allocations to clear objectives that describe the level of environmental protection and reliability of water supply. This lack of clarity means that the RWMPs are not transparent about how out-of-channel water use and protection of environmental values have been balanced and, therefore, limits are not well justified. In addition, only six RWMPs have defined regional limits by establishing both minimum flows and total allocations for all rivers. The absence of limits means that decision-making for individual projects will occur on a case-by-case basis rather than at a regional policy level, which creates uncertainty for stakeholders and leads to overallocation (OECD Citation2007).
Previous work (ME and MA Citation2009, LAWF Citation2010) identified the need for resource use limits to strengthen management of water quantity and quality in New Zealand, and the setting of limits has recently become mandatory (New Zealand Government Citation2011). Technical inputs to defining limits must select and use the most appropriate scientific tools for setting environmental flows and total allocations, and account for spatial variation in environmental values and water resources. However, there is also a significant socio-political challenge to the policy development process associated with making normative decisions that balance environmental protection and resource use.
Our study suggests that regional councils in New Zealand are responding to these challenges and that water management planning is evolving, in part due to the uptake of new tools. The use of environmental flow setting methods has become more structured in current RWMPs and councils have used a risk-based framework to justify the methods they have used. RWMPs are reviewed and revised on a regular basis and the limits they establish can be challenged and altered if detailed studies show that objectives can be met with alternative limits. This justifies the use of generalized methods () in regions, or parts of regions, where water resource development pressure is not high. Councils are also considering the consequences of environmental flows and total allocations in an integrated manner, with hydrological simulations increasingly being applied to the problem of defining total allocations, although this is not yet widespread. Another key to developing limits on a region-wide basis has been the use of spatial frameworks to account for environmental variation within regions. Our analysis showed that RWMPs have used spatial frameworks to define management units and increase the clarity and spatial specificity of the plan objectives. The more recent RWMPs are therefore showing that the use of new scientific tools in a more integrated way makes setting clear objectives and justifiable water resource use limits for all rivers in jurisdictional regions achievable.
We argue that future RWMPs could improve in at least two key areas. First, we suggest that objectives in RWMPs should be expressed clearly for both environmental values and the availability and reliability of water for out-of-channel use. Measurable objectives would make normative decisions transparent for all stakeholders, and make limits more justifiable. We suggest that the translation of minimum flows and total allocations into objectives for reliability and retained habitat (e.g. and ) or potentially other ecological criteria would increase the transparency of the resulting limits. Second, we argue that the technical challenge of defining regional strategic limits can be assisted by more integrated use of scientific tools such as by linking FDC and generalized habitat models (). We acknowledge that detailed studies involving site-scale habitat modelling and analysis of hydrological time series will produce assessments with lower uncertainties and more detailed insights into the consequences for both environmental and water use outcomes. However, for regional assessments in situations where water resource use pressure is not high (i.e. low- to medium-risk situations; ), we suggest the use of generalized methods (i.e. estimated FDC and generalized habitat models) may be appropriate. Greater use of spatial frameworks will also assist in setting regional limits. Spatial frameworks can be used as a basis for up-scaling existing information and data and to infer environmental characteristics in low- to medium-risk situations. Spatial frameworks can also be used to stratify regional variability in values and flow regimes and these strata can be used to define management units for which specific objectives and regulatory criteria apply.
Finally, minimum flows, and to a lesser extent total allocations, have generally been considered as sufficient planning mechanisms to protect environmental values for run-of-river water use in New Zealand. However, as run-of-river allocation reaches limits imposed by regulation, water storage and harvesting is increasingly being considered (LAWF Citation2010). The effects of storage provide new challenges to defining limits to extractive water use due to their greater influence on components of the flow regime other than low flows. Consequently, RWMPs and the scientific methods used to set limits will need to continue evolving to meet these challenges.
CONCLUSIONS
We have shown that environmental flows are only one component of defining limits to water use and that socio-political decisions must ultimately determine how the balance between environmental protection and resource use will be struck. Therefore, we conclude that scientific input to defining water resource use limits needs to characterize the availability and reliability of water for out-of-channel use and to show how this varies with different levels of environmental protection. Water use limits are most justifiable if they are transparently linked to clear objectives for both environmental outcomes and water resource use. While defining justifiable limits at regional scales is a challenge, we suggest that it is achievable with the integration of at least three scientific tools that provide methods for defining environmental flows and total allocations, and accounting for spatial variation in environmental values and hydrological regimes.
Acknowledgements
We thank the two anonymous reviewers whose suggestions improved the original manuscript.
Funding
This research was funded by the New Zealand Ministry for Business, Innovation and Enterprise, New Zealand Foundation for Research, Science and Technology, Environmental Flows Programme (C01X0308) and Wheel of Water Programme (ALNC1102).
REFERENCES
- Acreman, M., 2005. Linking science and decision-making: features and experience from environmental river flow setting. Environmental Modelling and Software, 20, 99–109.
- Acreman, M., et al., 2008. Developing environmental standards for abstractions from UK rivers to implement the EU Water Framework Directive. Hydrological Sciences Journal, 53, 1105–1120.
- Acreman, M. and Dunbar, M.J., 2004. Defining environmental river flow requirements—a review. Hydrology and Earth System Sciences, 8, 861–876.
- Aqualinc. 2008. Sustainable freshwater management—towards an improved New Zealand approach. Christchurch, New Zealand: Aqualinc.
- Arthington, A.H., et al., 2006. The challenge of providing environmental flow rules to sustain river ecosystems. Ecological Applications, 16, 1311–1318.
- Biggs, B.J.F., 1995. The contribution of disturbance, catchment geology and landuse to the habitat template of periphyton in stream ecosystems. Freshwater Biology, 33, 419–438.
- Biswas, A.K., 2004. Integrated water resources management: a reassessment. Water International, 29, 248–256.
- Booker, D.J., 2010. Predicting wetted width in any river at any discharge. Earth Surface Processes and Landforms, 35, 828–841.
- Booker, D. and Acreman, M., 2007. Generalisation of physical habitat-discharge relationships. Hydrology and Earth System Sciences, 11, 141–157.
- Booker, D.J. and Dunbar, M.J., 2004. Application of Physical HAbitatSIMulation (PHABSIM) modelling to modified urban river channels. River Research and Applications, 20, 167–183.
- Booker, D., Dunbar, M., and Ibbotson, A., 2004. Predicting juvenile salmonid drift-feeding habitat quality using a three-dimensional hydraulic-bioenergetic model. Ecological Modelling, 177, 157–177.
- Booker, D.J. and Snelder, T.H., 2012. Comparing methods for estimating flow duration curves at ungauged sites. Journal of Hydrology, 435, 78–94.
- Bovee, K.D., et al., 1998. Stream habitat analysis using the instream flow incremental methodology. US Geological Survey, Biological Resources Division, Information and Technology Report.
- Crow, S.K., Booker, D.J., and Snelder, T.H., 2012. Contrasting influence of flow regime on freshwater fishes displaying diadromous and nondiadromous life histories. Ecology of Freshwater Fish, 22 (1), 82–94.
- Dodds, W.K.K. and Welch, E.B., 2000. Establishing nutrient criteria in streams. Journal of the North American Benthological Society, 19, 186–196.
- Dudgeon, D., et al., 2006. Freshwater biodiversity: importance, threats, status and conservation challenges. Biological Reviews, 81, 163–182.
- Environment Waikato, 2008. Proposed Waikato Regional Plan: proposed variation no. 6—water allocation. Analysis of alternatives, benefits and costs under s32 of the Resource Management Act 1991—Explanation of the approach taken in the variation. Hamilton, New Zealand: Environment Waikato. Available from: http://www.waikatoregion.govt.nz/ [Accessed March 2013].
- Ganora, D., et al., 2009. An approach to estimate nonparametric flow duration curves in ungauged basins. Water Resources Research, 45 (10), W10418.
- Gippel, C., Jacobs, T., and McLeod, T., 2002. Environmental flows and water quality objectives for the River Murray. Water Science and Technology, 45 (11), 251–260.
- Gleick, P., 1998. Water in crisis: paths to sustainable water use. Ecological Applications, 8, 571–579.
- Harding, J.S. and Winterbourn, M.J., 1997. An ecoregion classification of the South Island, New Zealand. Journal of Environmental Management, 51, 275–287.
- Hayes, J.W., Stark, J.D., and Shearer, K.A., 2000. Development and test of a whole-lifetime foraging and bioenergetics growth model for drift-feeding brown trout. Transactions of the American Fisheries Society, 129, 315–332.
- Holling, C.S., 1980. Adaptive environmental assessment and management. Bath, UK: John Wiley and Sons.
- Hurndell, R., Roygard, J., and Watson, J., 2007. Regional water allocation framework: technical report to support policy. Palmerston North, New Zealand: Horizons Regional Council. Available from: http://www.horizons.govt.nz [Accessed March 2013].
- Jowett, I.G. and Biggs, B., 2008. Application of the natural flow paradigm in a New Zealand context. River Research and Applications, 25, 1126–1135.
- Jowett, I.G., Hayes, J.W., and Duncan, M.J., 2008. A guide to habitat survey methods and analysis. NIWA Science and Technology Series. Wellington, New Zealand: NIWA. Available from: http://www.niwa.co.nz [Accessed March 2013].
- Jowett, I. and Richardson, J., 1995. Habitat preferences of common, riverine New Zealand native fishes and implications for flow management. New Zealand Journal of Marine and Freshwater Research, 29, 13–23.
- Jowett, I.G. and Richardson, J., 2003. Fish communities in New Zealand rivers and their relationship to environmental variables. New Zealand Journal of Marine and Freshwater Research, 37, 347–366.
- Kaika, M., 2003. The Water Framework Directive: a new directive for a changing social, political and economic European framework. European Planning Studies, 11 (3), 299–316.
- Lamouroux, N., 2008. Hydraulic geometry of stream reaches and ecological implications. In: H. Habersack, H. Piégay, and M. Rinaldi, eds. Gravel Bed Rivers 6: From process understanding to the restoration of mountain rivers. Amsterdam: Elsevier, 661–675.
- Lamouroux, N. and Jowett, I.G., 2005. Generalized instream habitat models. Canadian Journal of Fisheries and Aquatic Sciences, 62, 7–14.
- Lancaster, J. and Downes, B.J., 2010. Linking the hydraulic world of individual organisms to ecological processes: putting ecology into ecohydraulics. River Research and Applications, 26 (4), 385–403.
- LAWF (Land and Water Forum), 2010. Report of the land and water forum: a fresh start for freshwater. Wellington, New Zealand: Land and Water Forum. Available from: http://www.landandwater.org.nz/ [Accessed March 2013].
- Leathwick, J.R., et al., 2005. Predicting the distributions of New Zealand’s freshwater diadromous fish. Freshwater Biology, 50, 2034–2052.
- Leathwick, J., et al., 2011. Use of generalised dissimilarity modelling to improve the biological discrimination of river and stream classifications. Freshwater Biology, 56, 21–38.
- Lincoln Environmental. 2000. Information on water allocation in New Zealand. Christchurch, New Zealand: Lincoln Environmental. Available from: http://www.mfe.govt.nz/publications/water/water-allocation-apr00.html [Accessed March 2013].
- Mathur, D., et al., 1985. A critique of the instream flow incremental methodology. Canadian Journal of Fisheries and Aquatic Sciences, 42, 825–831.
- ME and MA 2009. Government Cabinet paper proposing a New Start for Fresh Water. Wellington, New Zealand: Offices of the Minister for the Environment and Minister of Agriculture (ME and MA). Available from: http://www.mfe.govt.nz/issues/water/freshwater/new-start-fresh-water.html [Accessed March 2013].
- Memon, P.A., 1997. Freshwater management policies in New Zealand. Aquatic Conservation: Marine and Freshwater Ecosystems, 7, 305–322.
- MFE (Ministry for the Environment), 2006. Snapshot of water allocation in New Zealand. Wellington, New Zealand: New Zealand Ministry for the Environment. Available from: http://www.mfe.govt.nz/publications/ser/snapshot-water-allocation-nov06/index.html [Accessed March 2013].
- MFE (Ministry for the Environment), 2007. Environment New Zealand. Wellington. New Zealand: Ministry for the Environment. Available from: http://www.mfe.govt.nz/publications/ser/enz07-dec07/ [Accessed March 2013].
- MFE (Ministry for the Environment), 2008. Proposed national environmental standard on ecological flows and water levels: discussion document. Wellington, New Zealand: New Zealand Ministry for the Environment. Available from: http://www.mfe.govt.nz/publications/water/proposed-nes-ecological-flows-water-levels-mar08/index.html [Accessed March 2013].
- Nehring, R. and Anderson, R., 1993. Determination of population-limiting critical salmonid habitats in Colorado streams using the Physical Habitat Simulation System. Rivers, 4 (1), 1–19.
- New Zealand Government. 2011. National Policy Statement for Freshwater Management 2011. New Zealand: New Zealand Government. Available from: http://www.mfe.govt.nz/rma/central/nps/freshwater-management.html [Accessed March 2013].
- Nilsson, C. and Renöfält, B., 2008. Linking flow regime and water quality in rivers: a challenge to adaptive catchment management. Ecology and Society, 13 (2), 18. Available from: http://www.ecologyandsociety.org/vol13/iss2/art18/ [Accessed March 2013].
- OCAG (Office of the Controller and Auditor General), 2005. Horizons and Otago Regional Councils: Management of freshwater resources. Wellington: Office of the Controller and Auditor General. Available from: http://www.oag.govt.nz/2005/water [Accessed March 2013].
- OECD (Office for Economic Co-operation and Development), 1996. New Zealand, Environmental Performance Review. Paris: Organisation for Economic Co-operation and Development. Available from: http://www.oecd.org/env/country-reviews/ [Accessed March 2013].
- OECD (Office for Economic Co-operation and Development), 2007. Environmental performance reviews: New Zealand. Paris: Organisation for Economic Co-operation and Development. Available from: http://www.oecd.org/env/country-reviews/ [Accessed March 2013].
- OECD (Office for Economic Co-operation and Development), 2008. Environmental outlook to 2030. Paris: Organisation for Economic Co-operation and Development. Available from: http://www.oecd.org/environment/indicators-modelling-outlooks/ [Accessed March 2013].
- Omernik, J.M., 1987. Ecoregions of the conterminous United States. Annals of the Association of American Geographers, 77, 118–125.
- Omernik, J.M., 1995. Ecoregions: a spatial framework for environmental management. In: W.S. Davis and T.P. Simon, eds. Biological assessment and criteria: tools for water resource planning and decision making. Boca Raton, FL: Lewis Publishers.
- Pearson, C.P., 1995. Regional frequency analysis of low flows in New Zealand. Journal of Hydrology (New Zealand), 33, 94–122.
- Poff, N.L., et al., 1997. The natural flow regime. A paradigm for river conservation and restoration. Bioscience, 47, 769–784.
- Poff, N.L., et al., 2010. The Ecological Limits of Hydrologic Alteration (ELOHA): a new framework for developing regional environmental flow standards. Freshwater Biology, 55, 147–170.
- Postel, S. and Carpenter, S., 1997. Freshwater ecosystem services. In: G.C. Daily, ed. Nature’s services: societal dependence on natural ecosystems. Washington, DC: Island Press.
- Pyle, E., et al., 2001. Establishing watershed management in law: New Zealand’s experience. Journal of the American Water Resources Association, 37, 783–792.
- Richter, B., et al., 2006. A collaborative and adaptive process for developing environmental flow recommendations. River Research and Applications, 22, 297–318.
- Rouse, H.L. and Norton, N., 2010. Managing scientific uncertainty for resource management planning in New Zealand. Australasian Journal of Environmental Management, 17, 66–76.
- SKM. 2012. Regional council practice for setting and meeting RMA-based limits for freshwater flows and quality. Christchurch, New Zealand: Sinclair Knight Merz.
- Snelder, T.H. and Biggs, B.J.F., 2002. Multi-scale river environment classification for water resources management. Journal of the American Water Resources Association, 38, 1225–1240.
- Snelder, T., Booker, D., and Lamouroux, N., 2011. A method to assess and define environmental flow rules for large jurisdictional regions. Journal of the American Water Resources Association, 47 (4), 828–840.
- Snelder, T.H. and Hughey, K.F.D., 2005. On the use of an ecological classification to improve water resource planning in New Zealand. Environmental Management, 36, 741–756.
- Snelder, T.H., Weatherhead, M., and Biggs, B.J.F., 2004a. Nutrient concentration criteria and characterization of patterns in trophic state for rivers in heterogeneous landscapes. Journal of the American Water Resources Association, 40, 1–13.
- Snelder, T.H., et al., 2004b. Is the River Environment Classification an improved landscape-scale classification of rivers? Journal of the North American Benthological Society, 23 (3), 580–598.
- SRC (Southland Regional Council), 2010. Regional water plan for Southland. Invercargill, New Zealand: Southland Regional Council. Available from: http://www.es.govt.nz/publications/plans/regional-water-plan/ [Accessed March 2013].
- Tharme, R.E., 2003. A global perspective on environmental flow assessment: emerging trends in the development and application of environmental flow methodologies for rivers. River Research and Applications, 19 (5–6), 397–441.
- Vogel, R.M. and Fennessey, N.M., 1995. Flow duration curves: a review of applications in water resources planning. Journal of the American Water Resources Association, 31, 1029–1039.
- Vörösmarty, C.J., et al., 2010. Global threats to human water security and river biodiversity. Nature, 467, 555–561.
- Williams, J.G., 1996. Lost in space: minimum confidence intervals for idealized PHABSIM studies. Transactions of the American Fisheries Society, 125, 458–465.
- Woods, R.A., et al., 2006. Estimating mean flow of New Zealand rivers. Journal of Hydrology (New Zealand), 45, 95–110.
- WRC (Waikato Regional Council), 2004. Proposed Waikato regional plan. Hamilton, New Zealand: Waikato Regional Council. Available from: http://www.waikatoregion.govt.nz/Council/Policy-and-plans/Rules-and-regulation/Regional-Plan/ [Accessed March 2013].
- WRC (Waikato Regional Council), 2012. Proposed Waikato regional plan: Variation No.6–water allocation. New Zealand: Waikato Regional Council. Hamilton. Available from: http://www.waikatoregion.govt.nz/Council/Policy-and-plans/Rules-and-regulation/Water-allocation- variation/ [Accessed March 2013].