Abstract
Discharge in the Warta River in Poland has been analysed based on long series of measurements at the Gorzów Wielkopolski gauge station (covering the whole catchment area) and at Poznań (middle and upper catchment area), and the Noteć River is characterized by the gauge station at Nowe Drezdenko. The annual mean discharge of the Warta River for the period 1981–2010 was equal to the average value for the last 163 years (209 m3 s-1), and there was no significant change in comparison with the ratio of runoff in the summer and winter half-years. In the driest region of Poland, the climate has been described on the basis of precipitation and air temperature. The annual mean precipitation for 1981–2010 (544 mm) in the Warta River catchment area was the same as that for the period 1848–2010. The precipitation has been increasing in spring and winter, and decreasing in summer. There is a positive and very significant correlation (r = 0.705) between the annual discharge and annual precipitation totals. The annual mean air temperature has risen by 0.6°C between the periods 1848–1980 and 1981–2010.
Editor D. Koutsoyiannis
Citation Ilnicki, P., Farat, R., Górecki, K., and Lewandowski, P., 2014. Impact of climatic change on river discharge in the driest region of Poland. Hydrological Sciences Journal, 59 (6), 1117–1134. http://dx.doi.org/10.1080/02626667.2013.831979
Résumé
Les débits de la rivière Warta en Pologne ont été analysés sur la base de longues séries de mesures à la station de jaugeage de Gorzow Wielkopolski (contrôlant l’ensemble du bassin versant) et à celle de Poznań (zone intermédiaire et supérieure du bassin versant). La rivière Noteć a été étudiée à partir des mesures de la station de jaugeage de Nowe Drezdenko. Le débit moyen annuel de la rivière Warta pour la période 1981–2010 était égal à la valeur moyenne pour les 163 dernières années (209 m3 s-1), et il n’y avait pas de changement significatif du rapport de l’écoulement hivernal à l’écoulement estival. Dans la région la plus sèche de la Pologne, le climat a été décrit sur la base des précipitations et de la température de l’air. Les précipitations moyennes annuelles pour la période 1981–2010 (544 mm) dans le bassin de la rivière Warta sont les mêmes que pour la période 1848–2010. Les précipitations ont augmenté au printemps et en hiver, et ont diminué en été. Il existe une corrélation positive et très significative (r = 0,705) entre le débit annuel et les totaux annuels de précipitations. La température annuelle moyenne de l’air a augmenté de 0,6°C entre les périodes 1848–1980 et 1981–2010.
1 INTRODUCTION
The aim of this study is the presentation of Warta and Noteć river discharge variability, trends and correlation in the driest Polish river basin for the period 1822–2010. Discharge variability was compared with precipitation and air temperature variability in different periods. Air temperature determines the potential and actual evapotranspiration, while human activity has little influence on the river flow and thus has not been taken into account as an alternative explanation.
In recent years, there has been intense discussion about the global water balance, particularly concerning its response to changing climate and social use (Milliman et al. Citation2008). The runoff of rivers is the effect of many factors, among which the most crucial are the climatic ones. River flow in a specific geographical region is affected by precipitation, evaporation, topography, lithology, subsurface flow systems, vegetation heterogeneity and other factors, including regional and global climatic fluctuations. However, the land morphology, land use (agriculture, forest), watercourse network, existing lakes and soil retention have to be considered too. As a general rule, however, the impacts of land-use activities on hydrological processes can only be verified on a smaller scale (up to some tens of square kilometres), where they can be distinguished from natural processes and other sources of degradation (FAO 2000, Blöschl et al. Citation2007). Therefore, no formula exists which could describe the relationship between discharge and all the other mentioned factors.
There is an increasing need for observational data which may help to discern any emerging trends in river flows so as to compare with future projections of climate models (Hannaford et al. Citation2011). Estimation of river flow variability is therefore important in water resources management (Sen and Niedzielski Citation2010). This approach consists of trend analyses in local and regional climate characteristics related to the basis of long historical records (Blöschl et al. Citation2007). Trends in hydrological data are analysed by many researchers and, on their basis, the effects of climate changes by the 2050s or 2100s in hydrological regimes at global and continental scales have been realized (Arnell Citation1999, Kundzewicz et al. Citation2008). To minimize the impact of anthropogenic disturbances, some studies considered only annual streamflow, and in addition models were applied in order to fill gaps or establish a time series of comparable lengths longer than 30 years (Milly et al. Citation2005, Dai et al. Citation2009).
There are a large number of analyses referring to discharge in various river basins, regions, countries and continents worldwide. Probst and Tardy (Citation1987) selected 50 major rivers distributed worldwide, and concluded that the global runoff has been fluctuating, but as an average it has only increased about 3% during the period 1910–1975. Mitosek (Citation1995) studied 176 flow data time series across the world. For the annual discharge in 46 European rivers, he found significant trends in only 45.7% of cases. Labat et al. (Citation2004) analysed records from 231 rivers accounting for about 51% of global runoff, using time series from 1880 to 1994. Their results show significant decadal to multi-decadal variations in continental runoff, indicating a 4% increase of global runoff per 1°C of global surface warming. At the regional scale, both increasing and decreasing runoff has been identified. This corresponds to more intense evaporation by oceans coupled with continental precipitation increase or continental evaporation decrease. This process leads, finally, to an increase in the global continental runoff.
An analysis of 925 rivers (of the 24 largest rivers in the world) for the period 1948–2004, accounting for 73% of global runoff, was presented by Dai et al. (Citation2009). Large multi-year variations were seen in most of the time series records. The effects of human activity (dams, reservoirs, withdrawal of stream water) on the yearly river flow were rather small and they mostly affected the annual cycle. Compared with the effect of climatic variations, this change is small. Only about one-third of the top 200 rivers showed statistically significant trends for the period 1948–2004. Out of these, 45 rivers showed downward trends, while 19 rivers demonstrated upward trends. In 195 rivers, a significant positive trend of runoff was only found in 31 rivers (Kundzewicz et al. Citation2005). In 137 representative world rivers, in the period 1951–2000, the cumulative discharge remained statistically unchanged (+0.4% ΔQ), but in 97 rivers the discharge increased by >10% and in 48 rivers it was greater than 30%. Only the last group of rivers showed a statistically significant (p > 0.05) trend. Most North European rivers also showed little change, whereas many Mediterranean rivers declined considerably. At the same time, precipitation remained statistically unchanged (+1% ΔP). The runoff trends in normal rivers are assumed to primarily reflect the climatic response to oceanic drivers (such as the North Atlantic Oscillation, NAO), and they do not reflect long-term changes (Milliman et al. Citation2008).
The regional variability of the runoff in the Nordic countries (Denmark, Estonia, Finland, Greenland, Iceland, Norway and Sweden) has been examined by analysing 160 long-term series of daily discharge. An increased trend was found in southwestern Norway and in Denmark, and a decreased trend in Sweden, especially since 1980. The increase in the maritime exposed areas is most significant in the autumn and early winter. The increase of runoff in southwest Norway is reflected in a similar increase of the precipitation in the same period (Roald et al. Citation1997). In Estonia, Lithuania and Latvia, for all three investigated periods (1923–2003, 1941–2003, 1961–2003), a significant positive trend for the winter and annual discharge time series was found, while no trend was observed for the spring, summer and autumn seasonal streamflows (Reihan et al. Citation2007). In Sweden, in the period 1901–2002, in 61 time series the average runoff increased by 5%, but the trend was statistically insignificant (Lindström and Bergström Citation2004). In 12 rivers of the Baltic Sea east coast, the discharge increased, but significantly in only five rivers (Timuhins et al. Citation2010).
Recently, several countries in Europe have established the so-called reference sites near natural rivers with a small catchment area (<1000 km2), constituting a basis for the investigation of climate and hydrological processes (Stahl et al. Citation2010). In 15 mainly western and northern countries, in 411 small river catchments, the streamflow trends for the period 1962–2004 were examined. On maps of European runoff trends, the authors show a large “white space” in Eastern and Central Europe. In that area, in the period 1963–2000, runoff trends have been observed using only model simulation. In annual runoff, there were strong negative trends in Iberia, in the Mediterranean and in Eastern Europe, from the Black Sea to the Baltic Sea. Monthly runoff trends reflect a wetting trend in the winter period (December–April) and a widespread drying pattern from February to August in the southern and some eastern parts of Europe. In Poland, during all the months, a negative trend dominates (Stahl et al. Citation2012). In the catchments of the 15 countries studied, analysis of the observed runoff and corresponding long time series of precipitation and air temperature showed that the variance of runoff is, on average, greater than the variance of precipitation and temperature; however, it is correlated with the mean climatic conditions. It was shown that the space–time patterns of long time series of runoff can be described by a few modes of oscillations having their direct counterparts in precipitation and temperature. These are directly driven by large-scale climatic variability and are unlikely to be altered by catchment processes (Gudmundsson et al. Citation2011). The occurrence of high and low flow across northern Europe in the period 1968–1997 is associated with large-scale patterns of temperature and precipitation variation (Kingston et al. Citation2009).
In Western and Central Europe, the trend analysis does not show any significant tendencies of the annual mean river discharge change during the period 1810–1990 in representative large rivers. However, in southeastern European rivers (Dnepr, Don, Volga), a decrease of runoff was found for the period 1881–1990 (Pekárová et al. Citation2003). Long-term runoff trends of 27 small Slovak rivers for the period 1931–2000 show a high variability. A constant trend can be observed in Slovakia in the north and east, a slow decrease in the centre, and a rapid decrease in the south. For the Danube Bratislava station, no increase or decrease of runoff is observed, but at Nagymaros station (Hungary) a decrease was detected in 1972–1992 compared to 1902–1922. The Slovak territory is divided by a line (from Marseille to St Petersburg) between northwestern Europe, where there is no decrease of runoff, and southeastern Europe, where the runoff trend was decreasing until 1995 (Miklanek and Pekárová 2004).
The mean long-term runoff of Czech rivers (50 gauge stations) from 1931–1980 to 1961–2005 showed an average increase of 1.3%, but the low-flow values indicated a significant increase. In the north and west, the increasing trend of mean annual discharge prevailed, while decreased discharge dominated in the south and east. The patterns were statistically insignificant. In January, February and March, increasing trends prevailed, while in the following 3 months, decreasing trends dominated (Fiala Citation2008). The closest connection could be observed between NAO indices for winter and the spring monthly runoff. The most significant increase in monthly runoff of Belarusian rivers in the Baltic Sea basin was observed after 1961. There was a positive trend of runoff at the beginning of the year, but a negative one in summer and autumn months (Danilovich et al. Citation2007). In the mountain catchment area of the Upper Rhine River in Switzerland, for the period 1808–2007, the most remarkable changes in distributions were found in winter and spring, when runoff, precipitation and air temperature had significantly increased. The distribution of runoff and precipitation is significantly correlated only in winter and spring (Hänggi and Weingartner Citation2011). The decadal variability of the Danube River flow in Romania was analysed for the period 1931–1995. A decreasing trend in annual mean discharge was visible for the last two decades. The flow was lower than normal when the NAO was in the positive phase, and vice versa (Rimbu et al. Citation2002).
On other continents, trend analyses of river streamflow values were presented in 26 river basins in Turkey (Kahya and Kalaycı Citation2004), in three river basins in Tanzania (Valimba Citation2008), six great rivers in the Arctic Ocean (Peterson et al. Citation2002), in China (Xiong and Guo Citation2004) and in Siberia (Smith et al. Citation2007). Trends in the runoff in 395 streamgauge stations for the period 1914–1993 were evaluated in the United States. The trends were most prevalent in the annual minimum (Q0) to medium (Q50) flow categories and were the least prevalent in the annual maximum (Q100) category (Lins and Slack Citation1999).
Studies of the river discharge in Poland were conducted by Stachý (Citation1970, Citation1972). His conclusion referred to the insignificant, negative trend in the discharge of the Warta River at Gorzów Wlkp for the period 1901–1965 (y = −0.117x + 208.2). Another conclusion was that the level of water discharge in the Oder and Vistula catchment areas depended on atmospheric circulation conditioning the climatic factors, while the influence of other factors decreased with the increase in catchment surface area. The number of days with the western type of circulation exerts a positive influence, while the southern and eastern circulation types were found to have a negative influence on the mean discharge. Descriptions of discharge and precipitation periodicity were given by Jeż et al. (1987), who showed that in Central Europe it is evident that there are both 3- to 4- and 12- to 14-year cycles in river discharge. In series of annual precipitation totals, 2- to 4-year cycles are dominant. Time series of annual maxima, mean and minimum flow of the River Warta in Poznań for the period 1822–1994 are presented by Graczyk et al. (Citation2002) and Kundzewicz et al. (Citation2004). This complete time series does not provide convincing evidence for the existence of a significant long-term trend in annual flow records. In the period 1901–2000, no significant changes in the mean annual runoff of the Oder and Vistula rivers were measured (Fal and Bogdanowicz Citation2002). Wrzesiński (Citation2009) showed his own analysis of 91 rivers in Poland, including the Warta River for the years 1951–2000, whereas, for the period 1951–1980, a significant positive trend in the discharge can be observed. In the Warta and Noteć rivers, this trend was significant (mainly at the 0.01 confidence level) both for the whole year and for all seasons. However, in the period 1971–2000, a non-significant negative trend of discharge was dominant in the Warta and Noteć rivers. During the whole 50-year period, an insignificant positive trend of the annual and seasonal discharge dominated in Poland, and this is also characteristic of the Oder, Vistula, Warta and Noteć rivers. Wrzesiński (Citation2009) stressed the existing dependence between the discharge in winter, as well as the positive and negative phases of the NAO. Sen and Niedzielski (Citation2010) examined the daily discharge time series in 15 gauge stations along the Upper Oder basin from November 1971 to October 2006, and found a statistically significant decreasing trend in the daily discharge data for all sites. Michalczyk and Paszczyk (Citation2011) concluded that global climatic changes exerted no significant influence on water discharge in the river, based on the water balance of Oder River catchment area for the 1901–2000 period.
An increase in the global mean air temperature has occurred since the mid-20th century, and it was clearly visible from 1980. In the last 100 years, the global air temperature has increased by 0.074°C/10 years, and in the last 25 years by 0.177°C/10 years (IPCC 2007, Kundzewicz and Döll Citation2009). A consistent correlation is highlighted between global annual temperature and the runoff, suggesting a 4% global runoff increase for 1°C global temperature rise; however, at the regional scale, both an increase and a decrease in temperature have been identified (Labat et al. Citation2004). In Poland’s lowland, for 1951–2000, the 10-year annual average air temperature rose by about 0.6°C, but this increase only occurred in the last two decades (Żmudzka Citation2004). A statistically significant increase in global land precipitation during the 20th century of 0.5% to 1% per decade has been noted in mid and high latitudes of the Northern Hemisphere, particularly in autumn and winter (IPCC Citation2001). There have been a lot of studies about precipitation and air temperature variability in Poland, but research on long time series is rare (Hellmann Citation1906, Smosarski Citation1925, Schubert Citation1941, Hohendorf Citation1966, Lorenc Citation2000, Degirmendžić et al. Citation2004, Bryś and Bryś Citation2010, Woś Citation2010, Łabedzki et al. Citation2011, Ilnicki et al. Citation2012).
2 STUDY AREA
The Warta River is the largest right-bank tributary of the Oder River and most of its catchment area (54 519 km2) lies below 200 m a.m.s.l. The upland starts above 580 km of the river length, and its total length is 795.1 km. The catchment area is divided by two proglacial stream valleys: the Toruń-Eberswalde Valley includes the lower and middle Noteć River and the lower Warta River, and the Warsaw-Berlin Valley includes the middle part of the Warta River. In this context, as far as the basin is concerned, it is important to note that the hydrographic net is well developed. The rivers with big longitudinal slopes are in the northern and southern parts of the catchment area. The studies of specific runoff in the whole Warta River catchment area for 1951–1970 showed that it can be divided into three clearly differentiated sections: the largest (66%) in the middle, with a specific runoff of 2–4 dm3 s-1 km2, includes the middle and lower Warta River catchment area with the rivers Prosna, Obra and Noteć. In the southern part, below the Wieluń–Łódź line, and in the northern part in the Noteć River right-bank tributaries, the specific runoff rises to 4–7 dm3 s-1 km2. The driest region lies between Poznań, Bydgoszcz and Lake Gopło. For the period 1921–1970, the specific mean runoff was 4 dm3 s-1 km2, which equalled 127 mm, and the runoff coefficient was 23.9% (Pasławski et al. 1972). Most of the Warta River basin is situated in the driest region of Poland (). The climatic water balance of the vegetation period (April–September) for 1970–2004 ranged between −200 and −250 mm, showing the lowest data in the area lying to the east of Poznań (Łabędzki et al. Citation2011). In the second part of the 20th century, the mean annual precipitation total at Poznań was 543 mm and the mean annual air temperature 8.3°C (Woś Citation2010).
Fig. 1 Map of the Warta River catchment area showing the location of meteorological stations and gauges, and the climatic water balance (April–September) in 1970–2004 (according to Łabędzki Citation2011).
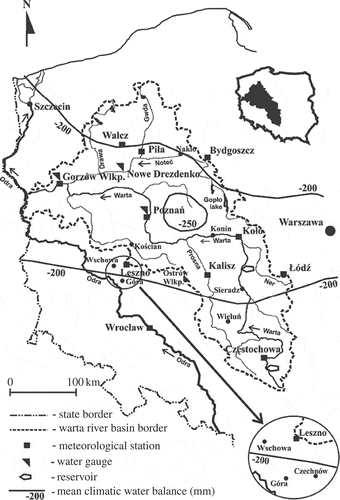
In the whole Warta River basin, sandy soils cover 45% of the land, loamy soils 41%, and organic and alluvial soils 14%. At the end of the 19th century, forests in the upper part of the Warta River catchment area covered only 20% of the land, in the middle part 15.6%, and in the lower part 25.4%. In this respect, the data for the Noteć River catchment area recorded only 14.4% forest in the upper part, but as much as 34.9% in the lower part (Wasser-Ausschuss Citation1896). In the Warta River basin, arable land constitutes 48%, grassland 10.3% and forests 30.2%, and “other” occupies 11.5% of the area, it being a region of intensive agriculture (Ilnicki et al. Citation2008).
In the 17th–19th centuries, the Warta River was intensively used for water transport. The drainage and regulation of the lower Warta (below Skwierzyna) and lower Noteć (below Drawa estuary) were introduced in the 18th century. After 1773, the middle Warta was also regulated. By the year 1880, the river was regulated to the point below Poznań, and by the end of the 19th century, it ended near Pyzdry, below the Prosna estuary. In the years 1890–1895 and 1905–1906, von Neumann measured the water runoff, which permitted regulations for adapting the river to 400-tonne barges. This was the basis for rebuilding the Warta in the part belonging to Prussia at that time, in the 348-km section below the Prosna estuary. The Polish project of the river regulation between Sieradz and the Prosna estuary (172 km) was realized in the part below Konin between 1936 and 1970, and in the part above Konin in the period 1949–1972. The lower and middle sections (406 km) of the Warta River constitute a waterway and, as a result, there are no weirs. After 1975, the water reservoirs Jeziorsko (483.4 km of the river; storage capacity: 224 hm3) and Poraj (764.5 km of the river; storage capacity: 25 hm3) were built (Arkuszewski et al. Citation1985).
The Noteć is the largest Warta tributary (388 km) with a catchment area of 17 302 km2. Regulation of the River Noteć was started in the middle of the 18th century, while the channelling of the Oder-Vistula waterway was undertaken at the end of that century. Consequently, training of the middle part of the river was undertaken later and, finally, land reclamation of the Noteć River valley took place. The works were continued in the middle of the 19th century and, in the periods 1863–1896 and 1905–1915, channelling of the lower and the middle Noteć River was carried out. At the end of the 19th century, the upper Noteć above Lake Gopło was regulated, and in the middle of the 20th century, this lake was connected with the Warta River near Konin by the Ślesiński Canal.
3 DATA
3.1 Discharge
Discharge data for the Warta and Noteć rivers are characterized and based on three gauges with a long series of observations starting at the beginning of the 19th century. The gauge station at Gorzów Wlkp, established in 1809, characterizes the whole Warta River basin (52 365 km2), while the gauge at Poznań (25 907 km2) describes its middle and upper parts. The gauge at Nowe Drezdenko characterizes the whole Noteć River, with a catchment area of 15 917 km2. The last two gauges were installed in 1818. All the stations concerned have always been in the same place. In the 19th century, up to 1945, the data at the Gorzów Wlkp and Nowe Drezdenko gauge stations, and until 1918 those at the Poznań gauge station were measured by the Prussian and German water authorities; later measurements were done by the Polish State Institute for Hydrology and Meteorology (PIHM) and the Institute for Meteorology and Water Management (IMGW). These institutes filled the short gaps in 1939 and 1945.
The total length of the analysed periods includes 163 years (1848–2010) at Gorzów Wlkp, 189 years (1822–2010) at Poznań and 148 years (1863–2010) at Nowe Drezdenko. However, monthly mean discharge data are available for the entire period (1896–2010) only at Gorzów Wlkp, for 1822–2010 at Poznań and for 1901–2010 at Nowe Drezdenko. The data on Noteć River discharge at Nowe Drezdenko for 1863–1892 should be regarded as uncertain and overestimated. As a result of river training carried out at that time, the river water level was reduced by 0.6 m. The long time series of annual mean discharge was calculated for 1901–2010 or 1896–2010. Schubert (Citation1941) published the monthly and annual mean values of water level and its fluctuation in the Warta River at Poznań only as means for some periods: 1818–1894, 1848–1893, 1896–1915 and at Gorzów Wlkp for 1810–1893, 1848–1893 and 1896–1935.
Rundo (Citation1949) supplied detailed data for the Noteć River. For the gauge station at Nowe Drezdenko and for the periods 1836–1892, 1863–1892, 1896–1915 and 1896–1910, he also quoted the annual and monthly mean levels. In the period 1893–1894, at the Nowe Drezdenko gauge, 23 discharge measurements were carried out (von Normann Citation1895, Keller Citation1902) and 13 measurements were conducted between 1897 and 1911. For the first period, Keller made a rating curve. For the intervals of −0.25 < H < + 1.83, the valid formula was the following: Q = 23.3863(H + 1.50)1.66 368, where H is the river water level above the gauge datum (26.39 m NN) in metres. The mean, low, middle and high levels, as well as the half-year and the annual mean values, and their frequency at the Nowe Drezdenko gauge for the years 1863–1892 and 1873–1892 had been published (Wasser-Ausschuss Citation1896).
For the period 1901–2010, the annual mean discharge data at all three examined gauge stations were available (Wasser-Ausschuss Citation1896, Schubert Citation1941, Rundo Citation1949, PIHM Citation1952, Citation1972, PIHM/IMGW 1961–1983, Olejnik Citation1991, PIHM Citation2000, Fal and Bogdanowicz Citation2002). The annual mean discharge at the Gorzów Wlkp gauge (1896–1900), and the monthly mean discharge at Gorzów Wlkp (for 1848–1893 and 1896–1915) and at Nowe Drezdenko (for 1863–1892, 1896–1910 and 1941–1950) were calculated based on river water levels and on the above-mentioned formulas.
3.2 Precipitation
All climatic data come from the measurements carried out at the meteorological stations in Prussia (Preussisches Meteorologisches Institut), Germany (Reichsamt für Wetterdienst) and Poland: at the State Institute for Hydrology and Meteorology (PIHM) and later at the Institute for Meteorology and Water Management (IMGW). The Warta River basin is represented by nine meteorological stations with long time series: Bydgoszcz, Częstochowa, Gorzów Wlkp., Kalisz (Ostrów Wlkp), Koło, Leszno (Czechnów, Góra, Wschowa), Łódź, Piła (Wałcz) and Poznań () and a regular distribution. Because of the absence of data for some years, the data taken from the nearby meteorological stations were used and their names are given in brackets, which allows for evaluation of mean precipitation in the Warta River basin at various time intervals. The aim, therefore, was to establish the mean precipitation in the basin, not at specific stations. Precipitation was measured at Leszno and Poznań (since 1848), Bydgoszcz (since 1861), Gorzów Wlkp (since 1875), Piła (since 1883), Częstochowa (since 1891), Łódź (since 1904), Kalisz and Koło (since 1921). For every year, the monthly means of precipitation total were available only for Leszno and Poznań (since 1848), Bydgoszcz (since 1861), and for all stations for the period 1981–2010. For the other six stations, the monthly means were available only as means for the following periods: 1891–1930, 1931–1960, 1931–1950 and 1951–1980. Annual, half-year and decadal means were calculated from monthly means. Mean annual precipitation (rainfall and snowfall) was presented for all stations for the total measured period and as a weighted mean for the Warta River basin.
3.3 Air temperature
Temperature was measured at Bydgoszcz, Leszno and Poznań (since 1848); Gorzów Wlkp., Kalisz and Piła (since 1891); Częstochowa and Łódź (since 1921); and Koło (since 1931). For every year, the monthly means were available for only three stations (Bydgoszcz, Leszno, Poznań) and for all stations for the period 1981–2010. For the other six stations, the monthly means were available only as means for the following periods: 1881–1930, 1931–1950 and 1951–1980. Their distribution permitted the calculation of the weighted mean air temperature for the Warta River catchment area. Data were only collected for three stations for the period 1848–1880, for seven stations in 1881–1930 and for eight stations in 1931–1950, whereas the data were obtained from nine stations in the period 1951–2010. Thus, the total research includes 163 years divided into periods of 20–50 years. Mean values for air temperature for the Warta River basin were calculated for the periods 1848–1880, 1881–1930, 1931–1950, 1951–1980 and 1981–2010, and weighted means for the period 1848–2010. The differences for periods in the 19th century are due to the accessibility of data. Annual, half-year and seasonal temperatures are presented for the aforementioned periods.
4 METHODS
We applied the linear regression gradient trend model (y = ax + b) and a 5% significance level recommended by Kundzewicz and Robson (Citation2004) for hydrological studies, which is commonly used in meteorological studies. The analysed data are normally distributed, with the exception of the runoff at the Gorzów Wlkp gauge station. This test is based on the assumption of the normal probability distribution of data and the trend line illustrates the results (Lindström and Bergström Citation2004). There is generally a good agreement between the results of the trend analysis using the linear regression method and the Mann-Kendall method (Svensson et al. Citation2005). Significant trends in many rivers have been found in some studies, but no globally homogenous trend has been reported (Kundzewicz and Döll Citation2009). Standard deviation and coefficient of variation were calculated for discharge and precipitation trends. Using the linear regression method, the trends of the annual mean discharge were determined and illustrated for the Warta River for the periods 1896–2010 and 1901–2010 at Gorzów Wlkp station, 1822–2010 and 1901–2010 at Poznań station, as well as for 1901–2010 for the Noteć River at Nowe Drezdenko station. Also, the changes in the ratio of discharge in the winter water half-year to that in the summer half-year were analysed for selected periods. For the period 1981–2010, the amount of monthly and annual discharge in the water gauges was compared and the trends presented graphically.
The analysis of discharge variability at three basic gauge stations of the Warta and Noteć rivers requires an investigation of the basic climatic elements (precipitation and air temperature) in the whole catchment area over the longest possible time. The study was limited to a comparison of the annual and decadal precipitation, as well as the precipitation ratio of the winter half-year to the summer half-year taken from nine stations. The weighted mean values for the whole catchment area for 163 years (1848–2010) were calculated for these stations. For the stations with different lengths of observation series (90–163 years), linear trends were calculated. The decadal mean values of precipitation for nine meteorological stations were compared in order to visualize the variability of the annual precipitation in the Warta River basin and their cyclical variability. For the Poznań station, the variation in the ratio of precipitation between the winter and the summer water half-years has been determined and presented as a figure. The air temperature of the Warta River basin was presented by the mean seasonal and annual air temperatures for nine meteorological stations in different periods between 1848 and 2010. Due to the availability of data, the lengths of the periods are different before the year 1951. For Poznań, the variability of annual mean values and the running 10-year average temperatures, as well as the ratio of the mean air temperature of the winter to the summer half-year for 1848–2010 have been presented, specifying the trends.
For the 1981–2010 period, the correlation between the ratio of the discharge of the Warta at Gorzów and of the ratio of annual precipitation in the whole Warta catchment area in the winter and summer half-years were presented graphically. The above-mentioned trends in discharge, precipitation totals and air temperature were compared for the periods 1848–2010 and 1981–2010.
5 RESULTS AND DISCUSSION
5.1 Discharge in the Warta and Noteć rivers
The variability of annual mean discharge was analysed for the period 1896–2010 at the Gorzów Wlkp gauge station (), for 1822–2010 at the Poznań station () and for 1901–2010 at the Nowe Drezdenko station (). The trends of river flow in these gauges were compared for the same period of time (1901–2010). The above analysis showed that there was always a small, statistically insignificant, positive trend in the Warta River, while in the Noteć the trend was positive and significant, showing a value of 1.14 m3 s-1/10 years (). At the Gorzów Wlkp and Nowe Drezdenko gauges, the variability (coefficient of variation, CV) of annual mean discharge was moderate, while at Poznań gauge it was high. In the Warta River, the discharge was 119–360 m3 s-1 at Gorzów Wlkp and 39.9–235 m3 s-1 at Poznań, while in the Noteć River it was 31.2–112 m3 s-1. The Warta River discharge at Gorzów Wlkp was more than twice as high as that at Poznań. The annual mean specific runoff in the longest examined periods of time was as follows:
Gorzów Wlkp: 4.02 dm3 s-1 km2,
Poznań: 3.75 dm3 s-1 km2 and
Nowe Drezdenko: 4.38 dm3 s-1 km2.
Fig. 2 Long-term variability of annual mean discharge of the Warta River (Gorzów Wlkp gauge) for 1896–2010.
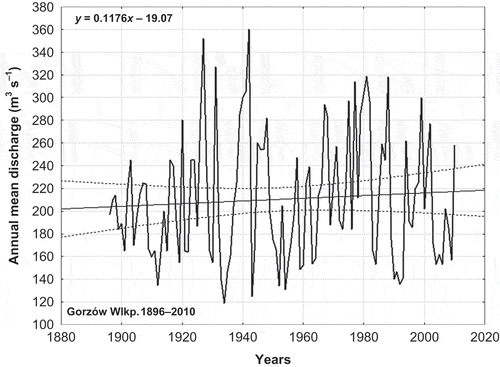
Fig. 3 Long-term variability of annual mean discharge of the middle and upper Warta River (Poznań gauge) for 1822–2010.
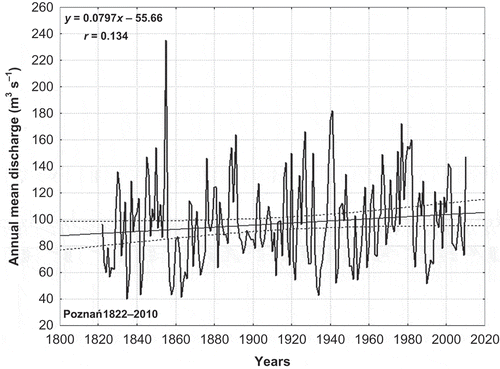
Fig. 4 Long-term variability of annual mean discharge of the Noteć River (Nowe Drezdenko gauge) for 1901–2010.
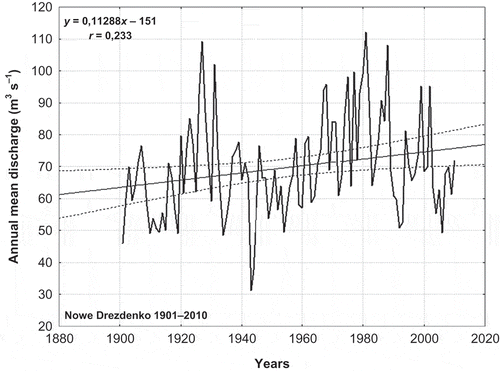
Table 1 Annual mean discharges in the Warta and Noteć rivers in 1822–2010.
Table 2 Mean monthly and annual discharge (m3 s-1) of the Warta River at Gorzów Wlkp for selected years between 1848 and 2010.
For all gauges, the monthly mean discharge was also compared over selected long time periods, with a focus on the three last decades (1981–2010), which had been under the “global warming” influence (). The length of the investigated periods was different before 1920 due to the availability of data from that time. At Gorzów Wlkp, the highest annual mean discharge occurred in the period 1848–1893 (231 m3 s-1). In the other examined periods the discharge fluctuated in the range of 195–215 m3 s-1. The annual mean discharge of 1981–2010 (209 m3 s-1) corresponded with the mean value from the whole examined period (); in the last three decades, it decreased from 228 to 197 m3 s-1. In 1981–1990, the discharge values were significantly higher than the long-term mean values. The highest runoff took place in March and April (327 and 317 m3 s-1), while it was lowest in the July–September period (approx. 140 m3 s-1). In the last 30 years at Poznań, the annual mean discharge of the Warta River was about 8% higher than in the years 1822–1970, and this increase took place in the summer half-year (). The highest runoff was in March and April, the lowest in the July–October period.
Table 3 Mean monthly and annual discharge (m3 s-1) of the Warta River at Poznań for selected years between 1848 and 2010.
The highest river water levels were recorded at Poznań mainly in February, March and April, while the lowest ones took place in the July–September period. In 1981–2010, the discharge in all three gauges showed a slightly negative trend, which was only statistically significant for the Noteć River (). This is opposite to the positive trend in the 1951–1980 period. This was caused by a significant decrease in the discharge in the upper part of the catchment area, where brown coal had been exploited for 10 years, as indicated by Pakość gauged discharge, which decreased from 6.30 m3 s-1 (1951–1990) to 4.18 m3 s-1 (1991–2010) (Ilnicki et al. Citation2012).
Fig. 5 Variability of annual mean discharge of the Warta and Noteć rivers for water years 1981–2010.
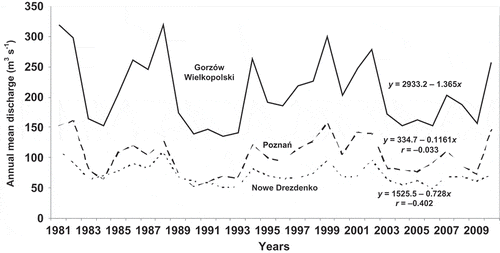
In the last 30 years, the annual mean discharge of the Warta River at Gorzów Wlkp (209 m3 s-1) was equal to the average for 1848–2010 (), being 8% higher than in the years 1822–2010 at Poznań (), and 4% higher than at Nowe Drezdenko (). In the following decades, 1981–2010, the discharge values for all gauges for the 1991–2010 period were slightly lower than for 1981–2010.
Table 4 Mean monthly and annual discharge (m3 s-1) of the Noteć River at Nowe Drezdenko for selected years between 1848 and 2010.
The changes in the ratio of the winter half-year to the summer half-year discharge showed a clear fluctuating pattern over the study periods. Spatially, this ratio is highest at Poznań and lowest at the Nowe Drezdenko gauge, except for the 1991–2000 period, when the ratio was comparable between the three catchments (). This ratio changed differently in the last three decades (), but a small increase of the discharge in the winter half-year was visible.
Fig. 6 Relationship of mean discharge of the Warta River at Gorzów Wlkp and precipitation in the catchment area between the winter half-year (November–April) and summer half-year (May–October) in the period 1981–2010.
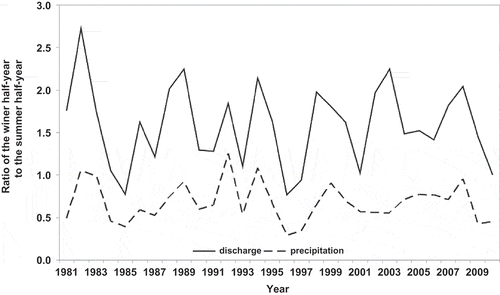
Table 5 Relationship of mean discharge in the Warta and Noteć rivers between the winter half-year (November–April) and summer half-year (May–October).
Stachý (Citation1970) had predicted that, by the year 2001, due to weaker sun activity followed by western circulation, the Oder basin discharge would gradually increase. The present studies confirm that hypothesis in the Warta River catchment area (, ). Model simulations of East European monthly runoffs for the period 1963–2000 show that in all months a negative trend dominated (Stahl et al. Citation2012). According to our study of the Warta River, there was a positive trend in the period 1896–2010, but for the periods 1950–1980 (positive) and 1981–2010 (negative), the trends were opposite. Also, Wrzesiński (Citation2009) showed that in 91 rivers in Poland, including the Vistula, Oder and Warta, for the years 1951–2000, an insignificant, positive trend of the annual and seasonal discharge dominated, which is also characteristic of the Warta and Noteć rivers. Michalczyk and Paszczyk (Citation2011) concluded that global climatic changes exerted no significant influence on water discharge in the Oder River in the period1901–2000.
5.2 Precipitation
The decadal precipitation in the Warta River basin (average of nine meteorological stations) indicates a fluctuating pattern of variability, with low precipitation in the periods 1871–1890, 1951–1960 and 1981–1990, and the highest precipitation in 1901–1920, 1961–1970 and 2001–2010 (). The weighted mean annual precipitation total in the Warta River basin (544 mm) ranged between 510 and 579 mm at nine stations in the period 1848–2010; it was higher (648.6 mm) only in the upper upland part of the catchment area (Częstochowa). In the 20- to 40-year periods analysed, the mean annual precipitation totals in the Warta River basin oscillated between 516 mm (1861–1890) and 558 mm (1891–1930). The variability (CV) of annual mean precipitation was moderate. All calculated trends of annual mean precipitation total were small and statistically insignificant (). For Bydgoszcz, Gorzów Wlkp, Koło and Poznań, the trends were positive (0.12–5.24 mm/10 years), and for Częstochowa, Kalisz, Leszno, Łódź and Piła, they were negative (0.48–7.66 mm/10 years). Therefore, in the Warta River catchment area we find both increasing and decreasing mean annual precipitation totals over a very long time.
Table 6 Annual precipitation totals (mm) in the analysed stations in the Warta River basin.
A typical feature of the climate was a significant fluctuation in the annual mean precipitation (). The share of precipitation total in spring was gradually increasing, while in summer it was decreasing. In the period 1981–2010, in the Warta River basin, the annual mean precipitation showed a positive but statistically insignificant correlation. Positive trend was observed in all seasons, but it was only statistically significant in the spring (r = 0.423). Cyclical fluctuation was observed in all seasons.
In 1848–2010, in Poznań, the ratio of precipitation in the winter half-year (November–April) in relation to the summer half-year (May–October) did not show any significant trend (y = 0.54x + 0.548; r = 0.01), but there were significant fluctuations in the particular years (). The mean ratio of the winter to the summer half-years was 0.65 at Poznań. However, it showed a very wide range (0.35–1.70). Extreme values appeared in 1881–1890 (0.54) and in 1910–1920 (0.78). Relatively low ratio values (0.55–0.59) in 1931–1980 showed a significant increase in 1961–1970 (0.68) and in 1981–2010 (0.69–0.71), indicating a return to the values from the years 1861–1880 (0.66–0.70) and 1891–1930 (0.64–0.78). During 163 years, there was no significant trend in this ratio. However, a periodicity of changes was observed.
5.3 Air temperature
In the 1848–2010 period in the Warta River basin, the annual mean air temperature in the examined 20- to 50-year periods varied within the range 7.8–8.6°C, with a mean value of 8.1°C. In the whole river basin, the annual mean air temperature has risen by 0.6°C in comparison with the years 1848–1980 and 1981–2010. The warmest periods were in 1981–2010, and the coldest occurred in the previous years (1951–1980). The annual mean air temperature in the period 1848–1980 equalled 8.0°C, and for 1981–2010 it rose to 8.6°C. The increase of the air temperature in the period 1981–2010 was most visible during spring (March–May) and winter (December–February). The summer mean air temperature for 1981–2010, in comparison with 1848–1980, showed an increase of only 0.34°C, but in winter it increased by 0.91°C and in spring by 1.03°C ().
Table 7 Annual and seasonal air temperatures (°C) in the Warta River basin in 1848–2010.
At Poznań, situated in the centre of the catchment area, the annual mean air temperature over 163 years showed a slightly positive significant trend (y = 0.0044x − 7.9373; r = 0.252). This reveals a periodicity of colder and warmer periods (). The ratio of mean air temperatures in the winter water half-year to the summer half-year at Poznań in the last 163 years equalled 0.12 (in the range from −0.13 to +0.36). At Poznań, this showed a statistically insignificant (y = 0.0004x + 0.0861; r = 0.222) positive trend (). In the period 1861–1970, the above ratio fluctuated at about 0.10–0.13, and it was lower (0.10) during the successive 40 years. In the period 1971–1990, it rose again to 0.15, to reach the level of 0.18–0.19 in the following 20 years. This indicates a gradual increase of the winter air temperature part in the annual sum.
5.4 Summary and discussion
The study presents the river flow, precipitation and air temperature variability in the Warta River basin over longer periods starting from 1822 (discharge) and 1848 (precipitation and air temperature). In shorter periods of time, there are very high differences. The trends of river flow at three gauges were compared over the same period of time (1901–2010). There was always a small, statistically insignificant positive trend for the Warta River, while for the Noteć River the trend was positive and significant. For the Warta River basin, we find both an increasing and a decreasing trend of annual precipitation, but the trends were small and statistically insignificant. The ratio of the winter half-year discharge to the summer half-year discharge in the period 1981–2010 in the Warta River at Gorzów Wlkp station increased to 1.59, and a small increase of the discharge in the winter half-year was visible. For the period 1981–2010, the annual precipitation showed a positive trend in all seasons, but it was statistically significant only in spring (r = 0.423). In the Warta River basin, the annual mean air temperature for 1848–1980 equalled 8.0°C and for 1981–2010 it increased to 8.6°C. These increases were most visible during spring and winter.
The study periods for discharge, precipitation and air temperature used in this study were not always the same. This was due to there being different start dates for measurements, and to the availability of data for different periods, and not for every year. Therefore, it was difficult to compare the three variables, so we compared the results of their trend analysis and the data for different 20- to 40-year periods, and analysed the correlation between annual discharge and precipitation and between the ratio of discharge and the precipitation total in winter and summer half-years.
In the period 1901–2010, the trend of annual discharge in the Warta River at Gorzów Wlkp is positive and insignificant. In this city, the trend of annual precipitation and air temperature for the period 1875–2010 is positive and insignificant (Ilnicki et al. Citation2012). In the Warta River basin the trend of annual precipitation is always insignificant: positive in northern and central parts and negative in southern and eastern parts. The trend of air temperature in the basin for the 1891–2010 period was small and insignificant (0.06–0.10°C/10 years), and for 1981–2010, it rose to 0.30–0.44°C/10 years. In the last 30 years, the trend in the northwestern part of the basin was significant. A comparison of annual precipitation totals in the different long periods analysed showed that the differences were small and differ from the 163-year mean of 544 mm by no more than 5%. The ratio between the precipitation in the winter half-year to that in the summer half-year in the period 1848–2010 does not show a significant trend, but for the period 1981–2010, it rose (0.63) compared with the period 1951–1980 (0.57).
Based on the collected data, covering the period 1981–2010, a comparison of the ratio of the Warta River discharge at Gorzów Wlkp with the ratio of annual precipitation totals for the whole Warta River basin in winter and summer water half-years was carried out. This ratio changed in a similar way (). There is a positive and significant correlation between the annual discharge at Gorzów Wlkp and the annual precipitation (y = 0.342x + 0.134; r = 0.705). The impact of the North Atlantic Oscillation on the extent of atmospheric precipitation and that of river discharge in Europe is practically identical. The former negative correlation in Poland is clearly higher and consequently more important in terms of statistical records, the effect of air temperature being decisive for a given rate of evaporation (Wrzesiński Citation2010).
The new large Jeziorsko Reservoir (storage capacity 224 hm3) resulted in a smaller annual mean runoff at the Poznań gauge in the period 1988–1997 (87.4 m3 s-1) compared to the period 1822–1987 (97.2 m3 s-1). In these same periods, the mean annual lowest value was higher (39.5 m3 s-1 compared to 32.1 m3 s-1), and the mean of the annual highest recorded values was evidently smaller (201 m3 s-1 cf, 449 m3 s-1) (Miller Citation1997). In the period 1988–1997, the mean annual discharge of the Warta River at Gorzów was only 91.2%, and at Poznań only 90.7% of the long-term average. This is similar to the difference (89.9%) between the above-mentioned annual mean runoff in the two compared periods. Thus, the influence of the Jeziorsko Reservoir is visible only in the lowest and highest runoff.
Many of the studies of runoff time series mentioned show that, in Central Europe, there is a dividing line from Bratislava (Slovakia) along the eastern border of the Vistula basin, over Minsk to St Petersburg. This line divides Western and Central Europe, where there is an increased or stable runoff, and southeastern Europe, where the runoff trend was decreasing in the Dnepr, Danube, Don and Volga rivers basins. This is opposite to the results from simulations presented by Stahl et al. (Citation2012).
The higher air temperatures in winter and spring, causing the melting of snow, result in the reduction and disappearance of the snow cover, as well as the absence of snow or soil freezing. Consequently, this will enable a higher percolation rate of the precipitation. With the detected increase of precipitation in the winter half-year, this creates the conditions of higher water retention in the soil, an increase of the groundwater level and finally a decrease of the spring water runoff. The annual runoff does not change visibly. An increase of precipitation in spring and winter as well as a decrease in summer are observed, and one can see that this causes a decrease of effective evapotranspiration and an increase of soil water retention. Peterson et al. (Citation2002) found in 12 long discharge records (1935–2002) in southern and central Russia that the recent minimum flow keeps increasing from about 1985. Because the low flow is sensitive to groundwater and unsaturated zone inputs to river discharge, some authors suggest that the reduced intensity of seasonal ground freezing, together with precipitation increases, might drive much of the well-documented but poorly understood increases in river discharge to the Arctic Ocean (Peterson et al. Citation2002, Smith et al. Citation2007). It could be similar for the Warta River basin.
6 CONCLUSIONS AND RECOMMENDATIONS
The above analysis showed that there was always a small, statistically insignificant, positive trend in Warta River discharge, while for the Noteć River discharge, the trend was positive and significant, with a value of 1.14 m3 s-1/10 years. For all gauges, the variability of annual mean discharge was high. The annual mean discharge in the lower part of the Warta River in the period 1981–2010 showed the same value for the last 163 years (209 m3 s-1), and the ratio of the mean winter and summer half-year discharge does not show any significant changes. Between the annual discharge and annual precipitation total, there is a positive and very significant correlation.
The annual precipitation total in the period 1981–2010 (544 mm) in the Warta River catchment area is equal to the average for the years 1848–2010. Increases of winter half-year precipitation and a decrease in summer were identified. This fact may suggest an evapotranspiration decrease and an increase of soil water retention.
The climatic changes in the period 1981–2010 did not exert any significant influence on the water discharge in the Warta River basin. The decreasing water runoff in the upper Noteć is caused by the negative influence of brown coal excavation in the region of Konin. Based on the above facts, there is no concern about a substantial threat for water resources and agriculture in the Warta River basin, this being the driest part of the country. There are no threats resulting from discharge changes and the influence of climate variability on agriculture production.
Acknowledgements
The authors thank the referees Claudine Dieulin and Patrick Valimba, as well as Professor Demetris Koutsoyiannis, HSJ Co-editor, for their constructive and useful comments, which indeed helped improve the article.
ARCHIVE SOURCES
- Hellmann, G., 1906. Die Niederschläge in den Norddeutschen Stromgebieten. Vol. 2. Berlin: D. Reimer. (in German) Table I.
- Jeż, G., Jokiel, P., and Kożuchowski, K., 1987. Cykliczne zmiany przepływów w rzekach europejskich na tle wahań opadów atmosferycznych. Wiadomości IMGW, 10 (31), 4, 3–19. (in Polish).
- Keller, H., 1902. Hydrographische Arbeiten in Preussen und Norddeutschland. In: IX Int. Schiffahrtskongress Düsseldorf. Münster: J. Bredt. (in German).
- PIHM, 1952. Materiały do bilansu wodnego Polski. In: IX. Przepływ Warty i Noteci w latach 1921–1940. Prace PIHM 28. Warszawa: Wydawnictwo Komunikacji i Łączności. (in Polish).
- PIHM, 1972. Przepływy charakterystyczne rzek w dorzeczu Warty w latach 1951–1965. Warszawa: PIHM.
- PIHM, 2000. Przepływy charakterystyczne rzek polskich w latach 1951–1995. Warszawa: Wydawnictwo Komunikacji i Łączności.
- PIHM/IMGW (Państwowy Instytut Hydrologiczno-Meteorologiczny–State Institute for Hydrology and Meteorology/Instytut Meteorologii i Gospodarki Wodnej–Institute for Meteorology and Water Management), 1961–1983. Roczniki hydrologiczne wód powierzchniowych. Dorzecze Odry i rzek Przymorza między Wisłą a Odrą. Ed. Warszawa: Wydawnictwo Komunikacji i Łączności (in Polish).
- Rundo, A., 1949. Charakterystyka przepływu Noteci na podstawie pomiarów z lat 1890–1924. Wiadomości Służby Hydrologiczno-Meteorologicznej I, 5, 331–344. (in Polish).
- Schubert, J., 1941. Der Wasserhaushalt im Warthegebiet. Eberswalde: R. Müller Verlagsgesellschaft. (in German).
- Smosarski, W., 1925. Temperatura i opady atmosferyczne w Wielkopolsce. Prace Komisji Matematyczno-Przyrodniczej PTPN, Seria A, 2, 75–176. (in Polish).
- von Normann, 1895. Wassermengenmessungen im Warthegebiet 1893–1895. Posen: Ministerium der öffentlichen Arbeiten, Landesanstalt f. Gewässerkunde u. Hauptnivellements, Posen. (in German).
- Wasser-Ausschuss, 1896. Der Oderstrom, sein Stromgebiet und seine wichtigsten Nebenflüsse. Vol. I–III. Berlin: D. Reimer. (in German) Tables.
REFERENCES
- Arkuszewski, A., et al., 1985. Monografia dróg wodnych śródlądowych w Polsce. Warszawa: Wydawnictwo Komunikacji i Łączności. (in Polish).
- Arnell, N.W., 1999. The effect of climate change on hydrological regimes in Europe: a continental perspective. Global Environmental Change, 9, 5–23. doi:10.1016/S0959-3780(98)00015-6.
- Blöschl, G., et al., 2007. At what scales do climate variability and land cover change impact on flooding and low flows? Hydrological Processes, 21, 1241–1247. doi:10.1002/hyp.6669.
- Bryś, K. and Bryś, T., 2010. Reconstruction of the 217-year (1791–2007) Wrocław air temperature and precipitation series. Bulletin of Geography – Physical Geography Series, 3, 121–171. Toruń: Nicolaus Copernicus University.
- Dai, A., et al., 2009. Changes in continental freshwater discharge from 1948 to 2004. Journal of Climate, 22, 2773–2792. doi:10.1175/2008JCLI2592.1.
- Danilovich, I., Wrzesiński, D., and Nekrasova, L., 2007. Impact of the North Atlantic Oscillation on river runoff in the Belarus part of the Baltic Sea basin. Nordic Hydrology, 38 (4–5), 413–423. doi:10.2166/nh.2007.025.
- Degirmendžić, J., Kożuchowski, K., and Żmudzka, E., 2004. Changes of air temperature and precipitation in Poland in the period 1951–2000 and their relationship to atmospheric circulation. International Journal of Climatology, 24, 291–310. doi:10.1002/joc.1010.
- Fal, B. and Bogdanowicz, E., 2002. Zasoby wód powierzchniowych Polski. Wiadomości IMGW, 25 (46), 2, 3–37. (in Polish).
- FAO (Food and Agriculture Organization), 2000. Land–water linkages in rural watersheds – electronic workshop. Conclusions and Recommendation. Rome: FAO of the United Nations.
- Fiala, T., 2008. Statistical characteristics and trends of mean annual and monthly discharges of Czech Rivers in the period 1961–2005. Journal of Hydrology and Hydromechanics, 56 (2), 133–140.
- Graczyk, D., Kundzewicz, Z.W., and Szwed, M., 2002. Variability of change in the flow of the river Warta 1822–1994. In: Z.W. Kundzewicz and M. Radziejewski, eds. Detecting changes of climate and hydrological processes. Sorus: Research Center for Agricultural and Forest Environment Poznan, 55–65. (in Polish).
- Gudmundsson, L., et al., 2011. Low-frequency variability of European runoff. Hydrology and Earth System Sciences Discussions, 8, 1705–1727. doi:10.5194/hessd-8-1705-2011.
- Hänggi, P. and Weingartner, R., 2011. Inter-annual variability of runoff and climate within the Upper Rhine River basin, 1808–2007. Hydrological Sciences Journal, 56 (1), 34–50. doi:10.1080/02626667.2010.536549.
- Hannaford, J., et al., 2011. Decadal-scale river flow variability in Europe: evidence from long hydrometric record spanning 1900–2004. Geophysical Research Abstracts [online], 13, EGU201112053. Available from: http://meetingorganizer.copernicus.org/EGU2011/EGU2011-12053.pdf [Accessed 21 March 2014].
- Hohendorf, E., 1966. Opady atmosferyczne w ostatnim stuleciu w Bydgoszczy. Bydgoskie Towarzystwo Naukowe, Seria B, 5 (2), 169–269. (in Polish).
- Ilnicki, P., Górecki, K., and Melcer, B., 2008. Eutrofizacja cieków wodnych zlewni Warty w latach 1992–2002. ed. Poznań: University of Life Sciences. (in Polish).
- Ilnicki, P., et al., 2012. Mit stepowienia Wielkopolski w świetle wieloletnich badań obiegu wody. ed. Poznań: University of Life Sciences. (in Polish).
- IPCC (Intergovernmental Panel on Climate Change), 2001. Climate change: impacts, adaptation and vulnerability. Contribution of the Working Group II to the Third Assessment Report of the Intergovernmental Panel on Climate Change. Cambridge: Cambridge University Press.
- IPCC (Intergovernmental Panel on Climate Change), 2007. Climate change. Fourth Assessment Report. Synthesis report. Summary for policymakers. (R.K. Pachauri and A.J. Reisinger, eds). Geneva: IPCC Secretariat.
- Kahya, E. and Kalaycı, S., 2004. Trend analysis of streamflow in Turkey. Journal of Hydrology, 289, 128–144. doi:10.1016/j.jhydrol.2003.11.006.
- Kingston, D.G., et al., 2009. Climate–river flow relationships across montane and lowland environments in northern Europe. Hydrological Processes, 23, 985–996. doi:10.1002/hyp.7202.
- Kundzewicz, Z.W. and Döll, P., 2009. Will groundwater ease freshwater stress under climate change? Hydrological Sciences Journal, 54 (4), 665–675. doi:10.1623/hysj.54.4.665.
- Kundzewicz, Z.W. and Robson, A.J., 2004. Change detection in hydrological records—a review of the methodology. Hydrological Sciences Journal, 49 (1), 7–19. doi:10.1623/hysj.49.1.7.53993.
- Kundzewicz, Z.W., et al., 2004. Detection of change in world-wide hydrological time series of maximum annual flow. Global Runoff Data Centre, Report Series, Report, 32, 1–36.
- Kundzewicz, Z.W., et al., 2005. Trend detection in river flow series: 1. Annual maximum flow Débit maximum annuel. Hydrological Sciences Journal, 50 (5), 797–810. doi:10.1623/hysj.2005.50.5.797.
- Kundzewicz, Z.W., et al., 2008. The implications of projected climate change for freshwater resources and their management. Hydrological Sciences Journal, 53 (1), 3–10.
- Labat, D., et al., 2004. Evidence for global runoff increase related to climate warming. Advances in Water Resources, 27, 631–642. doi:10.1016/j.advwatres.2004.02.020.
- Łabędzki, L., et al., 2011. Estimation of reference evapotranspiration using the FAO Penman-Monteith method for climatic conditions of Poland. In: L. Łabędzki, ed. Evaporation [online]. Rijeka: InTECH Europe. Available from: http://www.intechopen.com/articles/show/title/estimation-of-reference-evapotraspiration-using-thefao-penman-monteith-method.for.climatic.conditi [Accessed 31 Mar 2011].
- Lindström, G. and Bergström, S., 2004. Runoff trends in Sweden 1807–2002. Hydrological Sciences Journal, 49 (1), 69–83. doi:10.1623/hysj.49.1.69.54000.
- Lins, H.F. and Slack, J.R., 1999. Streamflow trends in the United States. Geophysical Research Letters, 26 (2), 227–230. doi:10.1029/1998GL900291.
- Lorenc, H., 2000. Studia nad 220-letnią (1779–1998) serią temperatury powietrza w Warszawie oraz ocena jej wiekowych tendencji. Materiały Badawcze IMGW, Seria Meteorologia, 31, 1–104. (in Polish).
- Michalczyk, Z. and Paszczyk, J., 2011. Zmiany składników bilansu wodnego w zlewniach Wisły i Odry, 1901 2000. Czasopismo Geograficzne, 82 (1–2), 49–68. (in Polish).
- Miklanek, P. and Pekárová, P., 2013. Long-term runoff changes in region of Slovakia, FY Republic of Macedonia, Ohrid, 25–29 [online]. Available from: http://balwois.com.
- Miller, A., 1997. Przepływy Warty w Poznaniu w 10-letnim okresie eksploatacji zbiornika Jeziorsko na tle przepływów w ponad 150-letnim okresie poprzedzającym. In: Z. Przedwojski, ed. Konferencja nauk. techn. Eksploatacja i oddziaływanie dużych zbiorników nizinnych na przykładzie zbiornika wodnego Jeziorsko. Poznań: Wydawnictwo Akademia Rolnicza, 95–103. (in Polish).
- Milliman, J.D., et al., 2008. Climatic and anthropogenic factors affecting river discharge to the global ocean, 1951–2000. Global and Planetary Change, 62, 187–194. doi:10.1016/j.gloplacha.2008.03.001.
- Milly, P.C.D., Dunne, K.A., and Vecchia, A.V., 2005. Global pattern of trends in streamflow and water availability in a changing climate. Nature, 438 (7066), 347–350. doi:10.1038/nature04312.
- Mitosek, H.T., 1995. Climate variability and change within the discharge time series: a statistical approach. Climatic Change, 29, 101–116. doi:10.1007/BF01091641.
- Olejnik, K., 1991. Przepływy Warty w Poznaniu 1822–1988. Poznań: Fundacja Warta. (in Polish).
- Pasławski, Z., Koczorowska, J., and Olejnik, K., 1972. Odpływ średni na obszarze dorzecza Warty. Gospodarka Wodna, 6, 214–218. (in Polish).
- Pekárová, P., Miklánek, P., and Pekár, J., 2003. Spatial and temporal runoff oscillation analysis of the main rivers of the world during the 19th–20th centuries. Journal of Hydrology, 274, 62–79. doi:10.1016/S0022-1694(02)00397-9.
- Peterson, B.J., et al., 2002. Increasing river discharge to the Arctic Ocean. Science, 298 (5601), 2171–2173. doi:10.1126/science.1077445.
- Probst, J. and Tardy, Y., 1987. Long range streamflow and world continental runoff fluctuations since the beginning of this century. Journal of Hydrology, 94, 289–311. doi:10.1016/0022-1694(87)90057-6.
- Reihan, A., et al., 2007. Changes in water discharges of the Baltic states rivers in the 20th century and its relation to climate change. Nordic Hydrology, 38 (4-5), 401–412. doi:10.2166/nh.2007.020.
- Rimbu, N., et al., 2002. Decadal variability of the Danube river flow in the lower basin and its relation with the North Atlantic Oscillation. International Journal of Climatology, 22, 1169–1179. doi:10.1002/joc.788.
- Roald, L.A., et al., 1997. Historical runoff variation in the Nordic countries. In: A. Gustard, et al. eds. FRIEND’97—Regional hydrology: concepts and models for sustainable water resource management. Wallingford: IAHS Press, 87–96.
- Sen, A.K. and Niedzielski, T., 2010. Statistical characteristics of riverflow variability in the Odra River Basin, Southwestern Poland. Polish Journal of Environmental Studies, 19 (2), 387–397.
- Smith, L.C., et al., 2007. Rising minimum daily flows in northern Eurasian rivers: a growing influence of groundwater in the high-latitude hydrologic cycle. Journal of Geophysical Research, 112, G04S47. doi:10.1029/2006JG000327.
- Stachý, J., 1970. Wieloletnia zmienność odpływu rzek polskich. Warszawa: PIHM, 1–42. (in Polish).
- Stachý, J., 1972. Z zagadnień wieloletniej zmienności odpływu rzecznego w Polsce. Wiadomości Służby Hydrologiczno-Meteorologicznej, 8 (20), 17–27. 3–4 (in Polish).
- Stahl, K., et al., 2010. Streamflow trends in Europe: evidence from a dataset of near-natural catchments. Hydrology and Earth System Sciences, 14, 2367–2382. doi:10.5194/hess-14-2367-2010.
- Stahl, K., et al., 2012. Filling the white space on maps of European runoff trends: estimates from a multi-model ensemble. Hydrology and Earth System Sciences, 16, 2035–2047. doi:10.5194/hess-16-2035-2012.
- Svensson, C., Kundzewicz, W.Z., and Maurer, T., 2005. Trend detection in river flow series: 2. Flood and low-flow index series. Hydrological Sciences Journal, 50 (5), 811–824. doi:10.1623/hysj.2005.50.5.811.
- Timuhins, A., Rodinovs, V., and Klavinš, M., 2010. Wavelet analysis of the Baltic Region river runoff long-term trends and fluctuations. Proceedings of the Latvian Academy of Sciences B, 64 (5/6), 229–235.
- Valimba, P., 2008. Temporal flow variations: a challenge for water management in Tanzania. In: 13th IWRA World Water Congress [online]. Montpellier. International Water Resources Association, 1–10. Available from: http://www.iwra.org/congress/2008/resource/authors/abs624_article.pdf.
- Woś, A., 2010. Klimat Polski w drugiej połowie XX wieku. Poznań: Wydawnictwo Naukowe A. Mickiewicz University Poznań. (in Polish).
- Wrzesiński, D., 2009. Tendencje zmian przepływu rzek Polski w drugiej połowie XX wieku. Badania Fizjograficzne nad Polską Zachodnią, 60, 147–162.
- Wrzesiński, D., 2010. Przestrzenne zróżnicowanie stabilności reżimu odpływu rzek europejskich. Poznań: Bogucki Wydawnictwo Naukowe. (in Polish). Seria Studia i Prace z Geografii i Geologii 3.
- Xiong, L.H. and Guo, S.L., 2004. Trend test and change-point detection for the annual discharge series of the Yangtze River at the Yichang hydrological station. Hydrological Sciences Journal, 49 (1), 99–112. doi:10.1623/hysj.49.1.99.53998.
- Żmudzka, E., 2004. Tendencje zmian i zróżnicowanie przestrzenne elementów klimatu w Polsce w drugiej połowie XX wieku. In: Z. Michalczyk, ed. Badania geograficzne w poznawaniu środowiska. Lublin: Wydawnictwo UMCS, 452–458.