ABSTRACT
Runoff generation and dynamics is an important issue in watershed and water resource management. Taking the Aksu River as a typical inland river, the spatial and temporal variations of δ18O and δD of the river water and its sources component pattern were investigated from May 2012 to May 2013. The results showed the following three main findings. Firstly, we analysed the runoff generation and mechanism over a longer time-scale in two tributaries of the Aksu River. Secondly, 46–54% of the runoff in the Aksu River was derived from groundwater, 31–36% from glacier meltwater, 5–8.8% from snow meltwater and 10% from precipitation. The third major finding was the significant inconsistency of the climate change impact on water resources. Specifically, our results showed that the Toxkan River is recharged by more glacier meltwater (36%), and responds to sensitive temperature changes. Autumn runoff is more sensitive to changes of precipitation and temperature.
Editor Z.W. Kundzewicz Associate editor Not assigned
1 Introduction
The combination of water shortage and inefficient usage has detrimentally affected the water supply in Northwest China. Based on previous studies, the loss of glaciers due to climate change will further deplete water supplies into the future (Chen et al. Citation2006). Therefore, when studying headwater and river water dynamics, it is important to understand the runoff generation mechanism especially the seasonal changes and streamflow sources and composition.
The separation of a hydrograph into its component parts is important for many types of water resources planning and management projects. Consequently, various approaches have been developed to estimate the contributions of different components in surface water as these cannot be directly measured. Stable isotope ratios of hydrogen and oxygen of water samples can provide essential information about water dynamics within a given watershed (Rock and Mayer Citation2007, Fasong et al. 2008, Kattan et al. Citation2008). These ratios are useful for identifying water sources via tracers of stable isotope hydrogen or oxygen, since different water sources act within a river at different times of the year.
In hydrograph separation analysis, the three-component model is widely used to compute the contributions of the various components of river water. Isotope hydrograph separation was first applied in the 1970s to define the baseflow component of total runoff. During the 1980s, the method gained in popularity because the variability of isotopes incorporated was involved in the entire process of the total runoff. Isotope hydrograph separation has undergone further improvements from the 1990s to the present.
Numerous studies have shown that components of surface water may include surface flow, subsurface water, meltwater and groundwater, and that their contribution is site-dependent (Wels et al. Citation1991, Xu et al. Citation2011, Jin et al. Citation2012). Burn (Citation2002) reviewed the development of isotope hydrology separation and pointed out that this method should be combined with new cutting-edge methods and an improved database, which can then be used to study hydrological processes with different climatic and human disturbance regimes. Qu et al. (Citation2008) reviewed the study of isotope hydrology separation in China, pointing out the importance of sampling in this method. Chen et al. (Citation2006) reported that the runoff in the Tarim River basin mainly came from groundwater, precipitation and meltwater. Kong and Pang (Citation2010) investigated the progress in isotope hydrology separation for alpine catchments, with an emphasis on its significance in reflecting the impact of global change on water resources, and explained the principle of isotope hydrology separation and its uncertainties. Kong and Pang (Citation2012) found that runoff in the Aksu River derived mainly from meltwater during the wet season.
The Aksu River is the main tributary of the Tarim River which is China’s largest inland river. The characteristics of the streamflow components of the Aksu River are still poorly understood. Previous studies of this area mainly focused on the spatial and temporal variations of stable isotopes in atmospheric precipitation and river water (Tian et al. Citation2007, Pang et al. Citation2011), but the runoff generation and mechanism over a longer time-scale is unclear and site-dependent, especially in the Aksu River. For this reason, in this study, the identification of water sources and the hydrograph separation analysis of two tributaries of the Aksu River from May 2012 to May 2013 were made. We attempted to: (1) compute the contribution of different water sources to streamflow during the entire year and (2) evaluate the sensitivity of the Aksu River to climate change. Our research will enhance the understanding of the hydrological cycle in semi-arid mountain-desert basins and help assess the effects of environmental and climatic changes on the hydrological cycle in Central Asia.
2 Data and methods
2.1 Study area
The Aksu River is located in the southwest of the Xinjiang Uygur Autonomous Region. The area with the river’s greatest runoff lies in the northwest edge of the Tarim River basin, which is bounded by latitudes 40°17ʹ–42°27ʹN and longitudes 75°35ʹ–80°59ʹE (), covering an area of 51.4 × 103 km2. The terrain declines gradually from north to south and from west to east, with distinct geomorphologic zoning from high to low. This is comprised, in turn, of low-middle mountains and hills, groups of piedmont pluvial fans, tilted alluvial plains, and alluvial plains (elevation 1000–1500 m).
Figure 1. Map showing the locations of sampling sites including geographical features in Xinjiang Autonomous Region. The Aksu River is located on the southern slopes of the Tianshan Mountains. The solid red star represents the hydrological station.
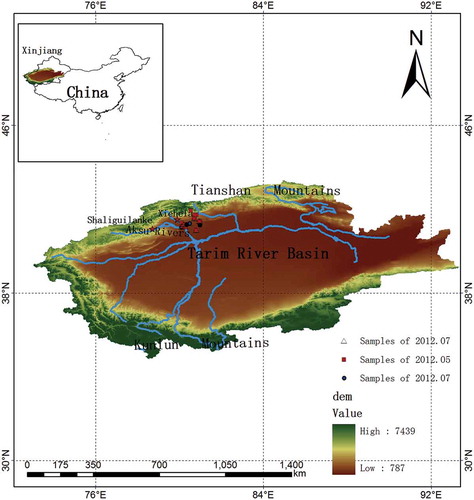
Because of its extreme inland location far from any sea, the Aksu River drainage area has a temperate continental arid climate characterized by drought, low rainfall, high evaporation intensity, and large temperature differences between both days and years (Xu et al. Citation2011). The multi-year average temperature, precipitation, and evaporation in the drainage area are 9.2–11.5°C, 64 mm, and 1890 mm, respectively; the annual extreme maximum and minimum temperatures are 40.2 and –27.6°C; and the multi-year mean sunshine duration is 2850 h. The two main tributaries, the Kumalak and Toxkan, join at Kaladuwei before flowing into the Aksu River. The catchment areas of the Kumalak and Toxkan (the Aksu’s northern and western branches, respectively) are 12 816 and 18 400 km2, while the lengths from its headwaters to the confluence point are 293 and 457 km, respectively (Ding Citation2007). Mountainous regions comprise the major areas of runoff-generation for the Aksu River, with runoff fluctuation resulting from a complex climatic condition and hydrological environment.
2.2 Data collection
Water samples (), including groundwater and surface water, were collected from the Aksu River from May 2012 to May 2013. Three sampling campaigns were undertaken in the entire Aksu River basin throughout September 2011, May 2012, and July 2012 (see ), and precipitation samples were collected at Xiehela and Shaliguilanke stations. Precipitation samples were also sampled (by event) from May 2012 to May 2013. However, as it was challenging to find suitable wells for sample collection in the mountainous watersheds, we collected groundwater from the two hydrological stations as well. Four glaciermelt samples were collected from the Aksu River basin during May 2012 and September 2012.
Table 1. Mean measurements of surface water and groundwater during different season in the Aksu River basin.
Prior to sampling, we washed a 5 mL glass sample vial three times using the sampled water, and then filled it with the water sample. Each vial was sealed, after sample collection, with Parafilm to prevent evaporation. All samples were then immediately sealed in a glass bottle and stored in a cold laboratory under −18°C. When the samples were analysed, they were stored at 4°C in the refrigerator to enable gradual melting and prevent evaporation. Meanwhile, the corresponding parameters, such as precipitation amounts, air temperature and humidity, were also measured at the meteorological stations during the sample collecting.
2.3 Methods
2.3.1 Stable isotope and chemical analysis
The δ18O and δD values for the surface water samples and groundwater samples were measured at the State Key Laboratory of Desert and Oasis Ecology, Xinjiang Institute of Ecology and Geography, Chinese Academy of Sciences. All samples were measured in 2012 using the Liquid Water Isotope analyser (LGR). The precision was ±0.1‰ and ±0.8‰ for δ18O and δD, respectively. Results were reported as relative to the standard V-SMOW (Vienna-Standard Mean Ocean Water). To avoid the memory effect associated with continuous-flow methods, measurements of each sample were repeated six times, and the first two values were discarded. The measured data were analysed using the LWIA Post software. This software determines the values of δ18O and δD by calculating differences between the known and measured values for standard water samples. By applying these corrections, extremely accurate measurements of the isotopic ratios can be achieved.
A 500-mL bottle was filled with river water to determine the major ions (Cl-, SO42-, HCO3-, Ca2+, Mg2+, Na+ and K+), and related chemical analyses were carried out at the State Key Laboratory of Desert and Oasis Ecology, Xinjiang Institute of Ecology and Geography, Chinese Academy of Sciences. The water samples were filtered through a 0.45-μ millipore membrane, using a high performance liquid chromatography (HPLC) method with a dual column instrument (model Dionex CD20) that has an analytical error of less than 1 mg/L. The water temperature, pH, and electrical conductivity (EC), together with total alkalinity values of all water samples, were measured in the field during sampling.
2.3.2 Hydrograph separation
We used three-component isotope hydrograph separation (Kong and Pang Citation2012) to calculate the ratio of various recharging sources in the Aksu River. Both the two and three-component methods can be described as a uniform equation:
where is total runoff discharge,
is the discharge of component m, and
is the tracer b incorporated into the component m. In isotope hydrograph separation, one of the tracers should be a kind of isotope. Several assumptions are required for this model (Sklash and Farvolden Citation1979, Turner and Macpherson Citation1990), as follows:
should be constant during the calculation period such as a rainfall–runoff process or snowmelt–runoff process.
should be different between each component.
A steady-state model adequately represents watershed conditions.
To meet these assumptions, the tracers were used variously in environmental tracers such as 18O, D, and d-excess, and in geochemical data such as TDS, EC, Si, and even DOC and temperature (Gibson et al. Citation2005, Kong and Pang Citation2012). In this study, we chose TDS and δ18O as tracers for their significant differences in recharging sources.
The measurement of mean contributions from several water sources in the Aksu River during different seasons was carried out by hydrograph separation analysis.
3 Analysis and results
3.1 Isotopic characteristics of surface water sample in the Aksu River basin
3.1.1 Kumalak River
During the monitoring period, δ18O values in precipitation varied between −23.1‰ and −1.54‰, with a weighted mean of −10.04‰. Obvious temporal variations for precipitation δ18O were observed. The value was especially high from May to June (), indicating that δ18O values of precipitation were significant affected by the regional evaporation. As the regional evaporation strengthened continuously, the precipitation δ18O was fractionating in the transport of water vapour, leading to high δ18O in precipitation in the Kumalak River basin.
The δ18O values of glacier meltwater ranged between −10.5‰ and −9.7‰, with an average of −10.1‰, suggesting an insignificant 18O signature for the meltwater ( and ). The sample which was collected in May (M-Q1) is more enriched than that in September (M-Q2). The groundwater has an opposite isotopic composition to local amount-weighted mean annual precipitation. Moreover, a seasonal trend is also observable in the groundwater, with the most isotopically enriched values in summer and positive values in winter (). In summer, the low groundwater δ18O value is due to the discharge from the soil water and seasonal frozen ground. According to previous studies (Zarei et al. Citation2014), water level fluctuations of piezometer wells in semi-arid basin groundwater level may increase by up to 16 m during the rainy season. This suggests a large soil recharge potential and that the storm flow reaches the stream largely through the soil by subsurface runoff mechanisms. New rainwater and glaciermelt water are able to recharge the aquifer, possibly through fractures, and mobilizes older water that has been residing in smaller fractures and pores within the catchment soil and bedrock.
Figure 2. Plots of δ18O versus δ2 H of surface water samples collected from (a) the Kumalak River and (b) the Toxkan River.
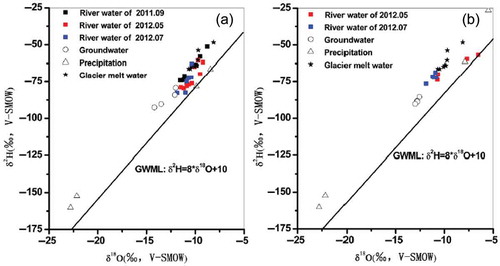
The overall δ18O composition of the Kumalak stream waters ranged between −13.82‰ and −3.92‰, with an average of −11.3‰ over the study period (). Significant seasonal variation can be observed in the Kumalak River basin, which suggested the pattern of streamflow components was inhomogeneous. In the snowmelt season (spring and late autumn), the δ18O values showed a larger variation and fluctuations, which is mainly because the snowmelt discharged into the river water. Throughout the evaporative enrichment of winter and early spring, the isotopic content of snow became more positive. When the temperature rose up, the snow meltwater drained into the river and caused the isotopic values of river water to increase. Due to the volatility of spring temperatures, the snowmelt in this period was not continuous, so the δ18O values of river water showed a huge fluctuation. In this period, the river water’s isotopic characteristic indicated that snow meltwater was the main source. As shows, low δ18O values were observed in summer with the large streamflow (May–July). In summer, increasing glaciermelt water and groundwater made the river water more negative and the river discharge of this period increased sharply. In autumn, with the river discharge decreasing, the isotope values of river water began to become higher.
3.1.2 Toxkan River
The Toxkan River has an isotopic pattern of surface water that is similar to the Kumalak River. The δ18O values in precipitation varied between −31.25‰ and 5.82‰, with a weighted mean of −11.30‰. Obvious temporal variations for precipitation δ18O were also observed. The value was especially high from May to June (). In winter, the isotope values of precipitation are similar to that of Kumalak River basin. But, during the summer, precipitation isotopic content of the Toxkan is more positive than that of Kumalak River basin. This implies that the summer rainwater of Toxkan River basin was affected by more intense evaporation than that of Kumalak River basin.
In Toxkan catchment, the mean 18O isotope value of groundwater was higher (−12.70‰) than that in Kumalak catchment (−12.90‰) especially in the summer. That indicated the groundwater of Toxkan catchment has a stable value and is weakly affected by the galaciermelt and precipitation.
In Toxkan catchment, the isotope values of streamwater showed a fairly consistent variation with the Kumalak catchment. The δ18O valley was observed in summer (May to July) and the δ18O peak was observed in the spring. The overall δ18O composition of the Toxkan stream waters ranged between −12.57‰ and −4.20‰, with an average of −10.95‰ (). Like the Kumalak River basin, the δ18O values of Toxkan River basin showed a large variation and fluctuations during the spring. But the samples showed a less scattered variation, which indicated the impact of snowmelt in the Toxkan River is less than that in the Kumalak River. As shown in , the δ18O value of the Toxkan River water is higher than that of the Kumalak River during the entire year except in spring.
3.1.3 Spatial variations of river water
The spatial distribution of the river water δ18O in the Aksu River basin is far from homogeneous owing to topographic gradients, land surface cover and snowpack distribution in the two tributaries. shows the attitude–δ18O relationship for the two tributaries. In the Kumalak, the river water δ18O had a rapidly increasing trend between 1000 and 2000 m, but above 2500 m, the river water attained a stable state. This indicated that the Kumalak River discharged from different sources between 1000 and 2000 m. In the upstream portion of the Kumalak River (above 2500 m), the runoff mainly derived from the glacier meltwater. The Toxkan River water δ18O also showed a rapid rising trend between 1000 and 2000 m, but this trend is smaller than that of the Kumalak River.
3.2 Hydrograph separation in the Aksu River
3.2.1 Isotopic time-series partitioning of streamflow components
The partitioning of streamflow using stable isotopes as conservation tracers is based on the classical three-component hydrograph separation, and is inferred from the natural cycle of seasonally active source water (snow, glacier, groundwater, precipitation) in streamflow, typical streams in alpine cold climates. shows considerable streamflow variations at Xiehela station from 2012 to 2013, with seasonal variations of isotopic data during the same period. As described above, the streamflow partitioning strategy we employed provides considerable insight into the temporal controls on runoff sources and variability in a complex area. According to the river water mean δ18O values, the streamflow of the entire year was separated into four periods, as shown in . In winter, the river water had relatively stable isotopic composition. From November to mid-February, the mean δ18O values ranged from −11‰ to −12‰ for the Toxkan and Kumalak rivers, respectively. Streamflow during March and April tended to contain the highest proportion of surface water derived from snowmelt, and it therefore attained an isotopically enriched signature during this period of time. There is a significant change that could be observed during mid-May, after which the TDS and the river water δ18O became stable. From Sepetember to November, the river water δ18O showed a fluctuation trend. A one-way analysis of variance (ANOVA) of the temporal variations of the river water isotopes was performed in order to test the significance of the variations. The result showed that the mean river water isotopes of four periods had significant differences. We used Q1, Q2, Q3 and Q4 to represent these periods. However, as the streamflow of Q4 was mainly discharged by groundwater, we did not separate the streamflow component for this period. Q1 represents the snowmelt season, which is from March to May (the snowmelt period for the Kumalak River is longer than that for the Toxkan River). Period Q2 runs from 15 May to 1 September, when glacier meltwater and precipitation were the main sources. Period Q3 runs from 1 September to 1 November.
Some efforts to characterize the isotopic composition of streamflow and the identification of its primary source waters in the Aksu River basin have established an in-depth understanding of seasonal variability in the streamflow signal, as illustrated in . Some general features of the stable isotope pattern are as follows:
Figure 6. Relationships between TDS–δ18O of river water, groundwater, glaciermelt water, snowmelt water and precipitation during three periods along the Aksu River basin.
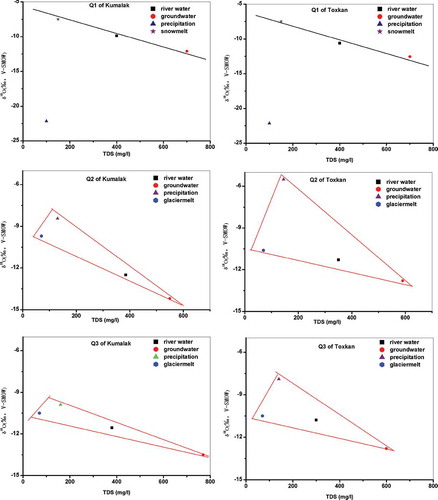
In spring, the δ18O value was volatile as the snow began to melt. The isotopic composition became extremely enriched with both δ18O and δD because the old snow underwent strong evaporation from the melt. However, the meltwater did not cause the runoff to obviously change (). Large fluctuations in spring temperature caused the river water’s isotope value to be hugely volatile. shows the river water, groundwater and snow meltwater distributed on a line during this period. This further proved the river water is mainly affected by the groundwater and snowmelt water. Using the average TDS and δ18O of groundwater, direct snowmelt water and precipitation as parameters, we can obtain the ratios of each component contributing to the Aksu River basin.
The low δ18O values during the wet season (May to September) were more positive than during the other periods when the rising temperature caused large areas of the glacier to melt. At the same time, the natural groundwater reservoirs were filled and the water was pushed out by direct snow meltwater and frozen groundwater. The lower δ18O groundwater and glacier meltwater levels caused the streamflow δ18O values to decrease. The samples of river just distributed in a triangle were formed by precipitation, glaciermelt water and groundwater (). Using the average TDS and δ18O of groundwater, glaciermelt water and precipitation as parameters, we can get the ratios of each component contributing to the Aksu River based on equation (1).
Steady δ18O increased with decreasing flow in autumn, along with rapid δ18O and flow response to rainfall in the wet season. The progressive reduction of surface water sources and the increase in the proportion of groundwater flow and runoff from snowmelt led to a gradual increase in isotopic signatures towards winter. Though the isotope values of river water had changed, the river water seemed to maintain the same water sources as Period 2. As illustrates, the river samples (average values) were distributed in a triangle formed by precipitation, glaciermelt water and groundwater. We calculated the ratios of each component contributing to the Aksu River basin using the same method as for Period 2.
3.2.2 Contribution of different water sources in the Aksu River
Using the isotopic content of different sources, the source ratios of three periods were calculated. shows the proportion of the various water sources in the Kumalak River during different periods. Results from the isotope separation analysis showed that groundwater made the greatest proportional contribution to the streamflow of the Kumalak River throughout the summer and autumn of 2012. In spring, snow meltwater played an important role in the streamflow components. More than 52% of the streamflow was discharged by the snowmelt water, while precipitation was only a minor contributor to the streamflow (2%).
Table 2. Estimated fractions of water sources as a result of hydrograph separation for the Kumalak River.
In summer, the main source was the groundwater, with glacier meltwater also being a large contributor to the streamflow (28.9%). In Period 3, groundwater also made the greatest proportional contribution (>40%) to the streamflow, followed by glacier meltwater and precipitation. The maximum fractional contribution of glacier meltwater and precipitation in Period 3 was 35 and 24.9%, respectively. It is worth mentioning that the proportions of both precipitation and glacier meltwater in Period 3 were larger than that in Period 2.
The Toxkan River had similar streamflow components to the Kumalak River (). In spring, the greatest proportional contribution to the streamflow was snowmelt. More than 48% of the streamflow was discharged by snow meltwater, followed by groundwater (45.9%) and precipitation (5.4%). In summer, glacier meltwater became an important source, contributing to about 40.6% of the streamflow, whereas precipitation had a relatively minor contribution (8.3%). Period 3, Results from the isotope separation analysis showed that, throughout Period 3, groundwater made the greatest proportional contribution (38.8%) to the streamflow, followed by glacier meltwater (36.7%) and precipitation (24.5%).
Table 3. Estimated fractions of water sources as a result of hydrograph separation of the Toxkan River.
Comparing the two main tributaries, the contribution of groundwater to the Kumalak River was larger than that of the Toxkan River. The recharge from glacier meltwater and precipitation in the Toxkan River was much greater than that in the Kumalak River, especially in summer.
4 Discussion
4.1 Variation of streamflow components’ response to snowmelt and precipitation
In most watershed areas, rainwater and snowmelt rapidly pass through catchments and comprise the major portion of the streamflow (Arvid Citation1981, Li et al. Citation2012). However, in some arid watersheds, increasing discharge during storms and snowmelt comes from old and pre-event water, because these catchments store a large volume of water and then release it to streams just as stormwater and snowmelt recharge the catchment from above (Sklash et al. Citation1986, Neal et al. Citation1988, Neal and Rosier Citation1990, Wels et al. Citation1991).
The results of summer streamflow component analysis underline this pattern. In the warm rainy season, the average fractional contribution of precipitation in the Kumalak and Toxkan were 7.7–8.3%. The rainfall–runoff transformation is a complex and nonlinear process. The precipitation of the Aksu River basin mainly occurs in May–August. However, the proportion of rainfall to the summer streamflow is rather small. The most important cause of nonlinearity is likely represented by the effect of antecedent conditions (Zarei et al. Citation2014). The low runoff ratios indicate that only a small fraction of the rainfall was directly translated to stream flow during the individual precipitation event, especially during an first phase of the summer. There are three reasons that could contribute to this pattern. The first is that precipitation at elevations above 2500 m arrives, and accumulates, as snow and hence most of catchment does not contribute to stream water during the period considered. However, even if the rain or snow accumulation part of the catchment is significant, it is not large enough to explain the observed pattern. The second factor is that following the long time of drought conditions most of the early precipitation results in a recharge of the catchment soil and only a small fraction of the riparian area closest to the river will contribute to streamflow. As the soils in the study catchment overlying the bedrock are porous with relatively high infiltration rates, most of the precipitation will infiltrate the soils, resulting in little overland flow. New rainwater or snowmelt water is able to recharge the aquifer, possibly through fractures, and mobilizes older water that has been residing in smaller fractures and pores with in the catchment soil and bedrock. When the soil is saturated, the rainfall–runoff generation mechanism gradually returns to a linear process. This is the reason why the proportion of precipitation to streamflow becomes larger in Period 3. The same result is also in line with the results from other studies (Zarei et al. Citation2014) which found that precipitation runoff ratios were low for most carchments with comparable semi-arid conditions. From the rainfall–runoff response analysis and isotopic content of the stream water, it can be concluded that the runoff generation was dominated by subsurface flows.
As the results of isotopic hydrology separation show, the isotopic composition of the Toxkan River is more homogeneous than that of the Kumalak River especially in spring. The mainly possibility of this pattern is the homogenization of snow meltwater during temporary surface or shallow storage. The difference in isotopic composition may be due to the different residence time of snowmelt water and groundwater. The mixing of varying ratios of snowmelt runoff and groundwater causes temporal variations in isotopic values. To reduce such variations in snowmelt runoff and precipitation, standing water in lakes, ponds, wetlands and reservoirs may mix water from multiple sources and homogenous isotopic compositions. The headwaters of the Toxkan River comprise a sizeable reservoir. The uniform isotopic values of streamwater during snowmelt and baseflow may reflect the dampening of temporal variations of snow inputs when snow meltwater is mixed with other water in the reservoir.
4.2 Evaluating the sensitivity of the Aksu River to climate change
The changes in processes of hydrology and water resources and the climate conditions are sensitive to global climate change (Kankam et al. Citation2013, Thompson et al. Citation2014). Numerous studies (Chen et al. Citation2006, Xu et al. Citation2010, Citation2011, Li et al. Citation2012) have attempted to clarify runoff and climate change trends and to apply newly emerging principles of their relationship to typical rivers in the arid region of Northwest China. In arid areas, Chen (Citation2010) found the correlations between runoff and temperature, and also discovered that precipitation may be related to runoff recharge proportions from glacial meltwater and precipitation. The hydrological response to climate change in the Tarim River basin has been proved by many researchers (Chen et al. Citation2006, Liu et al. Citation2006, Li et al. Citation2012).
Recently, many studies have paid more attention to the relationship of climate change and hydrograph process. Rasouli et al. (Citation2014) studied the hydrological sensitivity of a northern Canadian mountain basin to change in temperature and precipitation and found that mountain hydrology is extremely sensitive to warming especially during the melt season. Some studies (Dahlke et al. Citation2014, Zarei et al. Citation2014) found only a small proportion of rainfall was translated into stream water in the mountain arid basin during events especially during the first phase of the wet season. The changes in precipitation may partly modulate the streamflow. However, the streamflow, especially the summer streamflow of an arid mountain basin, is not significantly sensitive to changes in precipitation.
Isotope hydrograph separation results proved that precipitation is the important factor in the autumn runoff and more than 24% runoff is discharged from precipitation. In summer, due to the smaller ratio of precipitation (less than 10%), increasing summer precipitation may not cause large changes in summer runoff. Over the past 50 years, the autumn mountain precipitation has significantly increased, at a rate of 4.1–5.2 mm per decade. If this trend continues, the autumn streamflow will increase greatly, and the proportion of precipitation in the streamflow component also will increase. Rainfall-flood should be paid more attention to in the autumn runoff. The autumn runoff in the Aksu River basin is more sensitive to precipitation change.
The release of water as snowmelt and ice in high elevation catchments has significant social and economic impacts for populations living in mountain areas. The Aksu River is a typical glacier river and the extent of glacier input to a water system governs its sensitivity towards climate change (Kong and Pang Citation2012). This is even more critical under the current conditions of glacier retreat as the result of global warming. Therefore, it is important to understand the role of glacier meltwater on runoff dynamics and groundwater recharge in glacierized environments. We quantitatively determined the ratio of glacier meltwater and found that the Kumalak River is recharged by 31% of glacier meltwater, while the Toxkan River contains more than 36% of glacier meltwater in its discharge. Large ratio of meltwater to runoff indicated the temperature is a key factor in the hydrological process. On the basis of the metrological data of Aksu River basin, we found that annual temperature over past 50 years has risen significantly. If the temperature raising trend is continuous, the summer streamflow and autumn streamflow will rapidly increase. The recharge from glacier meltwater in the Toxkan River is much greater than that in the Kumalak River, especially in summer. This indicates that the Toxkan River is affected by temperature change. These results showed that rising temperature has an important influence on the Aksu River, and the Toxkan River is more sensitive to climate change than the Kumalal River.
Glaciermelt and glacier flash floods play a vital role in runoff supply and flood control security for the Aksu River. Over the past few decades, the Tianshan glacier has shown rapid melting due to rising temperatures. This has led to the formation of an increasing number of glacier-fed lakes. The total discharge of glacier flash floods has significantly increased from 0.1 × 109 m3 in the 1960s and 1970s to 0.3–0.4 × 109 m3 in the 1990s, while the total flood volume has risen to 0.45 × 109 m3 (Shen et al. Citation2009). Similarly, the peak discharge has increased 32% from the 1950s to the 1990s. The glacier flash floods drain into Merzbacher Lake and then, via the river, flow downstream. The flood period can last anywhere from a few days to more than two weeks (Shen et al. Citation2009). In addition, climate change especially rising temperature has great influence on the runoff of the Aksu River.
5 Conclusions
Isotopic compositions of streamwater in the Aksu River basin are presented in this study. The overall δ18O composition of Kumalak streamwaters from May 2012 to May 2013 ranged between −13.82‰ and −3.92‰, with an average of −11.30‰, while the Toxkan stream waters ranged between −12.57‰ and −4.20‰, with an average of −10.95‰. Furthermore, a significant seasonal variation of the river-water isotope value was observed in the Aksu River. The water has a significant spatial variation of isotope values at elevations ranging from 1000 m to 2000 m during the wet season, but no significant spatial variation above 3000 m.
Based on the river water mean δ18O values, and using hydrograph separation, the streamflow of the Aksu River basin was divided into four periods over the course of entire year. Groundwater, snowmelt water, precipitation and glacier meltwater are the main sources of the river water. The results of our study revealed that the glaciermelt contributed a significant proportion (31–36%) to the streamflow in the Aksu River basin, followed by precipitation (10%) and snow meltwater (5–8.8%). There is also a large proportion of groundwater in the Aksu River runoff which is mainly discharged from glacier meltwater and mountain precipitation.
The component of the streamflow in the Aksu River has obvious seasonal variations. The contribution of snow meltwater varied from 48.7% to 52.5% during the spring, whereas from May to August, glacier meltwater and groundwater were the main sources. The contribution of glacier meltwater varied from 28.9% to 40.6%, and that from groundwater varied from 51.1% to 63.5. In this period, precipitation accounted for only a small proportion (7.7–8.3%). From September to November, the two rivers’ streamflow components were similar, with 36% runoff discharged from glacier meltwater. The proportion of precipitation in the streamflow varied from 24.6% to 24.9%.
We found that the Kumalak and Toxkan rivers have similar streamflow components. In the Kumalak River, snow meltwater accounts for a larger proportion of the streamflow than in the Toxkan River. The role of glacier and snow meltwater should be taken into consideration in water resource management in the alpine rivers of the Tarim River basin. Glacier meltwater contributed a significant proportion to streamflow in the Kumalak and Toxkan Rivers. The recharge from glacier meltwater in the Toxkan River is much greater than that in the Kumalak River, especially in summer. This indicates that the Toxkan River is affected by temperature change.
Disclosure statement
No potential conflict of interest was reported by the authors.
Additional information
Funding
References
- Arvid, S., 1981. Relationships between precipitation chemistry, hydrology, and runoff acidity. Nordic Hydrology, 12 (2), 65–80.
- Burns, D.A., 2002. Stormflow-hydrograph separation based on isotopes: the thrill is gone? What’s next? Hydrological Processes, 16 (7), 1515–1517. doi:10.1002/hyp.5008
- Chen, X., 2010. Physical geography of arid land in China. Beijing: Science Press, Vol. 226, 289.
- Chen, Y.N., et al., 2006. Regional climate change and its effects on River runoff in the Tarim basin, China. Hydrological Processes, 20 (10), 2207–2216. doi:10.1002/hyp.6200
- Dahlke, H.E., et al., 2014. Isotopic investigation of runoff generation in a glacierized catchment in northern Sweden. Hydrological Processes, 28 (3), 1383–1398. doi:10.1002/hyp.9668
- Ding, Y.H., 2007. China’s national assessment report on climate change. Beijing: Science Press, 265–269 (in Chinese).
- Gibson, J.J., et al., 2005. Progress in isotope tracer hydrology in Canada. Hydrological Processes, 19 (1), 303–327. doi:10.1002/hyp.5766
- Jin, L., et al., 2012. Identifying streamflow sources during spring snowmelt using water chemistry and isotopic composition in semi-arid mountain streams. Journal of Hydrology, 470–471, 289–301. doi:10.1016/j.jhydrol.2012.09.009
- Kankam-Yeboah, K., et al., 2013. Impact of climate change on streamflow in selected River basins in Ghana. Hydrological Sciences Journal, 58 (4), 773–788. doi:10.1080/02626667.2013.782101
- Kattan, Z., et al., 2008. Estimation of evaporation and irrigation return flow in arid zones using stable isotope ratios and chloride mass-balance analysis: case of the Euphrates River, Syria. Journal of Arid Environments, 72 (5), 730–747. doi:10.1016/j.jaridenv.2007.10.011
- Kong, Y. and Pang, Z., 2012. Evaluating the sensitivity of glacier Rivers to climate change based on hydrograph separation of discharge. Journal of Hydrology, 434–435, 121–129. doi:10.1016/j.jhydrol.2012.02.029
- Kong, Y.L. and Pang, Z.H., 2010. Isotope hydrograph separation in alpine catchments: a review. Journal of Glaciology and Geocryology, 32 (3), 619–625.
- Li, B.F., et al., 2012. Trends in runoff versus climate change in typical Rivers in the arid region of northwest China. Quaternary International, 282, 87–95. doi:10.1016/j.quaint.2012.06.005
- Liu, S.Y., et al., 2006. Impact of the glacial change on water resources in the Tarim River basin. Acta Geogr Sin, 61 (5), 482–490.
- Neal, C. and Rosier, P.T.W., 1990. Chemical studies of chloride and stable oxygen isotopes in two conifer afforested and moorland sites in the British uplands. Journal of Hydrology, 115 (1–4), 269–283. doi:10.1016/0022-1694(90)90209-G
- Neal, C., et al., 1988. Chloride in precipitation and streamwater for the upland catchment of River severn, mid-wales; some consequences for hydrochemical models. Hydrological Processes, 2 (2), 155–165. doi:10.1002/hyp.3360020206
- Pang, Z., et al., 2011. Processes affecting isotopes in precipitation of an arid region. Tellus B, 63 (3), 352–359.
- Qu, S.M., et al., 2008. Isotope tracer in watershed hydrological modeling. Advances in Water Science, 19, 138–147.
- Rasouli, K., et al., 2014. Hydrological sensitivity of a northern mountain basin to climate change. Hydrological Processes, 28, 4191–4208. doi:10.1002/hyp.10244
- Rock, L. and Mayer, B., 2007. Isotope hydrology of the Oldman River basin, southern Alberta, Canada. Hydrological Processes, 21, 3301–3315. doi:10.1002/hyp.6545
- Shen, Y.P., et al., 2009. Changes in Merzbacher Lake of Inylchek glacier and glacial flash floods in Aksu River basin, Tianshan during the period of 1903–2009. Journal of Glaciology and Geocryology, 31 (6), 993–1002.
- Sklash, M.G. and Farvolden, R.N., 1979. The role of groundwater in storm runoff. Journal of Hydrology, 43 (1–4), 45–65. doi:10.1016/0022-1694(79)90164-1
- Sklash, M.G., Stewart, M.K., and Pearce, A.J., 1986. Storm runoff generation in humid headwater catchments: 2. A case study of Hillslope and low-order stream response. Water Resources Research, 22 (8), 1273–1282. doi:10.1029/WR022i008p01273
- Thompson, J.R., et al., 2014. Climate change uncertainty in environmental flows for the Mekong River. Hydrological Sciences Journal, 59 (3–4), 935–954. doi:10.1080/02626667.2013.842074
- Tian, L. et al., 2007. Stable isotopic variations in west China: a consideration of moisture sources. Journal of Geophysical Research: Atmospheres, 1984–2012 (112(D10)), 185–194.
- Turner, J.V. and Macpherson, D.K., 1990. Mechanisms affecting streamflow and stream water quality: an approach via stable isotope, hydrogeochemical, and time series analysis. Water Resources Research, 26 (12), 3005–3019.
- Wels, C., et al., 1991. Streamflow generation in a headwater basin on the Precambrian Shield. Hydrological Processes, 5 (2), 185–199. doi:10.1002/hyp.3360050206
- Xu, C.C., et al., 2010. Hydrology and water resources variation and its response to regional climate change in Xinjiang. Journal of Geographical Sciences, 20 (4), 599–612. doi:10.1007/s11442-010-0599-6
- Xu, J.H., et al., 2011. The nonlinear trend of runoff and its response to climate change in the Aksu River, western China. International Journal of Climatology, 31 (5), 687–695. doi:10.1002/joc.2110
- Yuan, F. and Miyamoto, S., 2008. Characteristics of oxygen-18 and deuterium composition in waters from the Pecos River in American Southwest. Chemical Geology, 255, 220–230. doi:10.1016/j.chemgeo.2008.06.045
- Zarei, H., et al., 2014. Runoff generation processes during the wet-up phase in a semi-arid basin in Iran. Hydrology and Earth System Sciences Discussions, 11 (4), 3787–3810. doi:10.5194/hessd-11-3787-2014