ABSTRACT
A geochemical approach using stable oxygen isotopes was used to understand streamflow generation processes in the highly peaty catchment of the Rokytka Brook in the headwaters of the Vltava River, Czech Republic. The contribution of water from peat bog areas to the total surface runoff was assessed using a hydrological time series, as well as geochemical, hydrochemical and isotope-hydrological approaches for unit hydrogram separation by means of anion deficiency. Using data from the hydrological year 2008, the role of an existing peat bog in the runoff formation dynamics of the Rokytka Brook catchment was determined, and the hydrological cycle was described and assessed using stable 18O/16O isotopes. The research findings strongly support the fact that peatland areas within the studied catchment do not significantly communicate hydraulically with surface streams, and their hydrological function in this region is insignificant.
Editor M. C. Acreman; Associate editor not assigned
1 Introduction
There are several obstacles to defining the environment in which hydrological processes take place. The surface runoff concept is insufficient, and determination of basic hydrological processes using information about the qualitative composition of water is inadequate. Hydrochemical and geochemical approaches may be used to explain the mechanisms of water retention and runoff formation in headstream areas. Solving the question of runoff formation in river headstream areas requires detailed information on the basic elements of runoff mechanisms. Since the theory of so-called “effective precipitation” (Horton Citation1933) was accepted, the hydrological responses of runoff to causal rainfall have been extensively studied. Despite this, the real mechanisms of water behaviour underground have not been so clearly described (McDonnell Citation2003). The absence of detailed measurements results in simplified assumptions and insufficient description of complicated processes such as the causal aspects of runoff formation. Rainfall–runoff transformation requires additional data, which can be obtained using a natural tracer. This information can be provided by a combination of isotope and geochemical approaches (Hoeg et al. Citation2000, Šanda Citation2010). This new dimension to hydrological studies has proven extremely simple and superior to previous methods (McDonnell Citation2003, Klaus and McDonnell Citation2013).
Using information about isotope concentrations in the soil and subsurface water, as well as in the causal precipitation, it is possible to determine the proportions of these phases in extreme runoff, based on the isotope concentration in the outflow. However, the processes causing this exchange are not completely known (Kendall and McDonnell Citation1999, Šanda Citation2010). Water can often move through different isotopically and geochemically specified spaces and channels, or be retained (Kirchner Citation2003). These spaces are not space-homogenous and their contribution over time to the proportion of runoff is not necessarily constant (Šanda et al. Citation2009).
The hydrological function of peat bogs remains a matter of debate; so far, no-one has fully solved this problem, despite a number of research projects in different countries. Opinions on the function of peat bogs vary, and it is evident in the literature that questions raised in the second half of the 20th century will not be easy to answer. A detailed analysis of the various approaches to these questions was made by Ferda (Citation1960) for the Šumava Mountains. The so-called “theory of sponge”, evident in the literature from the late 1960s, suggested that peatland was important for (a) its significant water retention and discharge regulating capability during high rainfall totals, and (b) its discharge heightening and runoff balancing ability in dry periods. From the late 1970s, studies appeared that confirmed the retention capacity of peat bogs. Authors asserted that the only possible way to increase the retention capacity was to lower groundwater levels by means of drainage. Since that time, the problem of hydraulic communication between peat bog complexes and draining streams, including the procedures of drainage, such as diking of former drainage channels, has become a field of broad debate within the literature (e.g. Conway and Millar Citation1960, Burke Citation1967, McDonald Citation1973, Moklyak et al. Citation1975, Baird Citation1997, Holden et al. Citation2001). An interesting and detailed review of the literature covering opinions on both sides can be found in Holden et al. (Citation2004). Conflicting results were presented in the papers mentioned, depending on the different physical-geographical conditions. However, in general, the results proved that water courses draining areas of peatland show significant discharge variability, and that the influence of peatland on runoff regime balance had been overestimated in the past.
The same result – a significantly negative influence of peatland on the runoff process from the runoff variability point of view – was reached in a study area in the Upper Vltava River basin (Janský and Kocum Citation2008, Kocum et al. Citation2010, Čurda et al. Citation2011, Kocum Citation2012, Vlček et al. Citation2012). Peatland represents a very significant component of the hydrological regime in central parts of the Šumava Mountains. The existence of large peat bogs in this area is due to a humid climate and optimal relief configuration (Pošta Citation2004). The influence of peatland on water quality in water courses has been assessed as unambiguously negative, while the intensity of the effect is related to the area and volume in a catchment.
The influence of peat bogs on hydrological processes has also been discussed with respect to the effect on water quality, especially the ionic structure of water in periods of high or low discharges (Novák Citation1955, Citation1959, Onderíková and Štěrbová Citation1956, Freeman et al. Citation2001, Worrall et al. Citation2003). In dry periods, runoff from peat bogs decreases or becomes almost intermittent. This results in improvement in the quality of the water in streams draining the peat bog. This was confirmed by studies carried out by Ferda et al. (Citation1971) and others (Hruška et al. Citation1996, Citation1999, Oulehle and Janský Citation2003). However, during summer rainfall periods and spring snowmelt, there is a decline in water quality as peat bog complexes are fully saturated and water flows over the stream bed. In the case of water release during dry periods, this would be expected to result in decreased water quality.
A geochemical approach, as a complement to hydrological approaches, can be used to assess the contribution of the peat bog water to total runoff formation through determination of the intensity of hydraulic communication between the hydrological conditions of a peat bog complex and surface runoff. With respect to the significant proportion of peatland areas within the Vltava River headwaters (southwestern Czech Republic), the local retention potential and runoff formation, in relation to the existence of highly peaty areas, could be assessed. Limited hydraulic connectivity between peat bogs and surface streams is related to specific geomorphological conditions in the Vltava River headwaters.
The Šumava Mountains have the largest peat bog areas in the Czech Republic, as well as in Central Europe, due to their specific geology and morphology. Similar conditions for upland peat bog development can also be found in Scotland and Scandinavia. Waterlogged areas in Central Europe are formed mostly in flat areas or valleys, for example in Biebrza, Poland (Wassen Citation1995), or in western Slovakia (Juráková and Klementová Citation2003), where hydrological and climatic conditions are different from those of mountainous peat bogs. Therefore, it is better to compare hydrological processes within the Šumava Mountains peat bogs to those in Scottish or Scandinavian peaty areas.
Based on hydrological time series analysis carried out within the Vltava River headstream area, Kocum (Citation2012) determined the significant dependence of runoff variability on peatland proportion. Continual records of water level and the corresponding discharge values offer an extraordinary database for detailed analyses of the ascending and descending phases of flood waves, and for assessment of peat bogs and the influence of peat-forming soils on hydrological processes during dry periods. Through detailed statistical analysis of daily, monthly and yearly time series, slightly higher runoff variability was identified in the Vydra River basin (representing a quite peaty area) compared to the non-peaty Křemelná River basin. Runoff variability in experimental sub-catchments (including the Rokytka Brook catchment) was assessed by means of peak flow frequency analysis with respect to the different rates of discharge of the two compared streams, and by analysis of runoff reaction to causal rainfall during several rainfall events. The analyses of extreme runoff phases showed a higher frequency of peak flows and their shorter reaction to causal rainfall (lower water retention potency) in the case of highly peaty areas (Rokytka Brook catchment in particular); therefore, there is more distinct runoff variability of streams draining peatlands and peat-forming soils (Kocum and Janský Citation2009, Kocum Citation2012).
Stable oxygen isotopes are among the elements that occur in the natural hydrological cycle in various concentrations. Their concentrations can be used to give us unique information about water that enters a catchment in the form of precipitation, is retained in the catchment (e.g. in soil and underground water, saturated areas, rivers, lakes) and is released from the catchment in the form of runoff (Kendall and McDonnell Citation1999). Due to the uniqueness of the proportion of 18O/16O isotopes in each source over time (precipitation, subsurface water, surface water), the principle of 18O/16O fractionation is used for runoff formation modelling through the unit hydrogram separation method by means of anion deficiency.
The main goals of this study were:
to assess the hydrological cycle in a highly peaty catchment using stable 18O/16O isotopes and to determine the role of an existing peat bog in the runoff formation dynamics;
to evaluate the correlation between the surface water chemistry and instantaneous discharge in the highly peaty catchment; and
to determine the total balance of ecologically significant elements (N, C, S, Ca, Mg, K) in the highly peaty catchment.
To achieve these goals, the experimental catchment of the Rokytka Brook, situated in the headstream area of the Vltava River and representing a highly peaty watershed, was chosen.
2 Study area
The upper Vltava River basin, including the area where our experimental research was undertaken, the Rokytka Brook catchment (see ), represents an area with a highly significant proportion of peatland. The catchment of interest is located in the most exposed part of the Šumava Mountains. The Rokytka Brook is the left tributary of the Roklanský Brook, one of two sources of the Vydra River, which becomes the Otava River. The experimental catchment, down to the closing profile with installed water level gauge, has an area of 3.86 km2. According to the ZABAGED (the basic platform for geographical data of the Czech Republic: digital terrain model of the Czech Republic area) underlayer of bogs and marshes, and to the water streams and basins of the TGM Water Research Institute DIBAVOD (digital basis for water management data: thematic water management upgrade of ZABAGED layer) layers, bogs make up 0.892 km2, which corresponds to about 23.1% of the total area.
Figure 1. Location of the studied catchment including the CHMI (Czech Hydrometeorological Institute) and FS CU (Faculty of Science, Charles University in Prague) water stage recorders and automatic water level and precipitation gauges within the Vltava River headwaters (Vydra-Modrava closing profile). (a) Vydra River headwaters and location of the Rokytka Brook experimental catchment; (b) location of sampling profiles and the main peat bogs. Sampling profiles, 1: stream water at the outflow; 2: the largest peat bog lake in the catchment; 3: tributary.
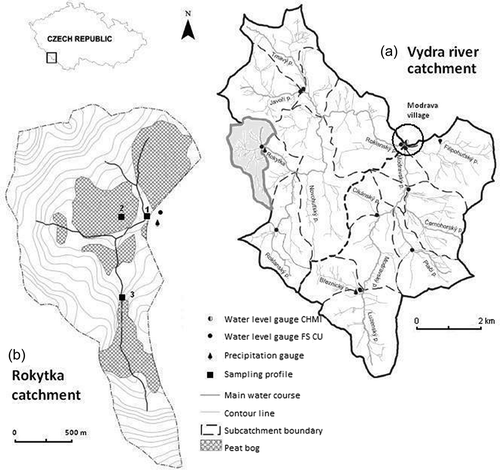
Although the studied catchment is mountainous, its exposure in the plateau part of the Šumava Mountains gives it flat watershed characteristics favourable for the existence of high moorland. The catchment is formed of a typical old-aligned surface with an average slope of about 6° and an altitude varying between 1090 and 1230 m a.s.l. The bedrock is composed of weathered rocks, mainly granite. Soil conditions in the studied area include the features of on-site Organosols, as described by Šefrna (Citation2004). Local soils represent typical examples of Šumava Mountain soils, where a vertical sequence of several types of soil, with Histosols on the ridges and basins, is typical. The basin is mostly covered by entic Podzol. The second most common type of soil is Histosol, which covers about 26% of the catchment area. In the lower part of the catchment, close to the closing profile, a relatively broad peat bog complex, with significant cubic capacity (up to 7.2 m depth), and several peat bog lakes can be found (see ). In certain lower parts of the basin, Gleysols are distributed. From a runoff conditions point of view, water-saturated Organosols represent extreme runoff accelerators. Even if Organosols have a broad capacity for retention of water, which is successively delivered to streams, their retention effect does not dominate in the state of full water saturation.
The vegetation can be divided between peat bog and forest (other). Within the peat bog vegetation, bogs of mountainous type are easily distinguishable. They are surrounded by waterlogged spruce forest and minerotrophic sedge peat soils (Bufková Citation2009). The rest of the forest vegetation is mainly composed of spruce with the addition of fir and beech, and is present predominantly on the south-facing slope. The forest has been influenced by the spruce bark beetle calamity.
3 Methods
3.1 Hydro-climatic monitoring
The existing systems of automatic ultrasound and hydrostatic pressure water-stage recorders (with data transmission through the GPRS network using a GSM module), climatic stations and shuttle precipitation gauges (; located in the closing profile of the Rokytka Brook catchment) and other modern equipment and methods were used in the chosen experimental catchments to assess the landscape retention potential and to determine rainfall–runoff relations in this area. The experimental profiles chosen also contained sensors for observation of physical-chemical parameters. This network, together with state profiles within the Czech Hydrometeorological Institute (CHMI) limnographic stations, produced a very good basis for analysis of the headwater runoff regime. In the profiles given, periodic discharge measurements using a hydrometric propeller were carried out in order to construct accurate consumption curves with high confidence coefficients. Using the above measurements, a detailed analysis of peatland hydrological function was carried out. The influence of peat bogs on runoff conditions was assessed by thorough comparison of runoff regimes in sub-catchments with different peatland proportions and other physical-geographical conditions. Processes of streamflow generation and present peat bog revitalization activities were studied primarily using hydrological statistics, and then by means of ion, carbon and oxygen isotope balance analyses, with particular attention to periods of high or low discharge rates.
3.2 Precipitation sampling
Precipitation sampling for isotope analyses was carried out once a month initially, and then twice a month after 3 months, in the study catchment. The precipitation amount and its isotopic composition (δ18O-H2O) were measured (). Precipitation chemistry and deposition were measured at monthly intervals in the adjacent catchments of Roh and Doupě, which have very similar characteristics and are close to the study area.
3.3 Surface water sampling
Surface water sampling was carried out to determine chemistry and isotopic composition in monthly and two-weekly time steps, respectively, in three different sampling profiles: outflow profile (water level gauge, 1), bog profile (organogenous lake, 2) and inflow profile (tributary, 3) (see ). The sampling profile represented the study locality (sites) where water was sampled. The study catchment was closed by the automatic ultrasound hydrological gauge for continual water level and discharge monitoring. Instantaneous discharge in the studied profiles during water sampling for laboratory analyses is shown in . Sampling episodes were chosen with respect to the whole discharge range in order to obtain data from extreme episodes such as thaw, snowmelt, rainfall and drought.
3.4 Chemical analyses
Samples were kept in the dark and cold (4°C) and analysed in accredited laboratories of the Czech Geological Survey (ISO 9001). The pH values and concentrations of the following species were stated: NO3−, SO42−, Cl− determined by ion exchange chromatography, F− potentiometrically by ion-selective electrode, and NH4+ by Indofenol blue colorimetry; Ca, Mg, Na, K, Al, Fe and Si measured by flame atomic absorption spectrometry (FAAS); alkalinity measured by titratably strong acid (0.1 M HCl); dissolved organic carbon (DOC) and total nitrogen (TN) obtained by high-temperature oxidation-catalysed platinum, as for CO2 and NO2; total organic nitrogen (TON) calculated as the difference between TN and N-NO3 + N-NH4; and δ18O-H2O determined using standard methods with an analysis error ±0.1% (Bůzek Citation1984).
3.5 Water body apportionment using 18O
In nature, oxygen (O) occurs as three stable isotopes 18O, 17O and 16O. The H216O molecule is lighter and needs less energy to evaporate than the H218O molecule. During condensation it behaves in the opposite way. The tracing method uses the fact that the proportion of heavier and lighter isotopes changes at a phase interface (usually between liquid and vapour phases). The extra energy needed to transfer molecules with heavier isotopes to the vapour phase results in a relative deficiency of molecules with heavier isotopes in water vapour than in liquid water (Kendall and McDonnell Citation1999). Because this process (fractionation) is temperature related (Craig and Gordon Citation1965), precipitation in colder areas and during colder periods has a lower 18O/16O ratio compared to warmer areas and warmer periods. Due to the uniqueness of the 18O/16O isotope ratio of each source (precipitation, subsurface water, surface water) at a particular time, the principle of 18O/16O fractionation could be used for runoff formation modelling. The symbol “delta” is used to express the 18O/16O isotope ratio. It represents the relative proportion of measured 18O/16O to a standardized 18O/16O proportion (in this case SMOW – Standard Mean Ocean Water) (Hoeg et al. Citation2000, Klaus and McDonnell Citation2013).
To calculate the contribution of the bog to the Rokytka Brook runoff, a simple model, including the inputs from the bog and from the tributary, was used. It was not possible to assess the input of direct precipitation separately due to the similar signals of δ18O-H2O in the bog and the precipitation (see ). The water balance of the Rokytka Brook experimental catchment stems from the mass balance (Bůzek Citation1984). The contribution of the bog to the Rokytka Brook runoff was therefore calculated on the basis of the following equations:
Figure 4. Profile of δ18O-H2O in surface water and precipitation in the Rokytka Brook catchment for the hydrological year 2008; the y-axis shows the relative balance contribution of bog water to the total runoff from the watershed.
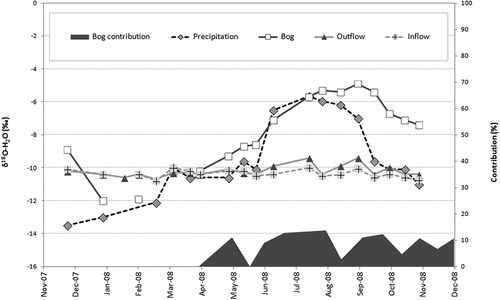
where δ18OO is the outflow isotopic composition, δ18OT is the tributary isotopic composition, δ18OB is the bog isotopic composition, p is the relative contribution of bog water (%) and Q is the discharge in observed profiles.
4 Results
4.1 Precipitation chemistry and deposition
The total amount of precipitation in the Rokytka Brook catchment in the hydrological year 2008 was 1485 mm. Deposition of each element in the nearby Roh and Doupě catchments is presented in . Precipitation amounts and δ18O-H2O profile are visualized in . The seasonal course of δ18O-H2O was very consistent.
Table 1. Deposition (kg ha−1 year−1) and precipitation amount (mm) in the Roh and Doupě experimental catchments of the Czech Geological Survey, located very close to the Rokytka Brook catchment.
4.2 Hydrological analyses
The Rokytka Brook represents typical hydrological behaviour of streams in the central Šumava Mountains, with peak flows occurring in April and May during snowmelt (). The annual discharge was 0.18 m3 s−1, so the studied year, 2008, showed a slightly below-average value ().
Figure 5. Mean monthly discharge in the outflow from the Rokytka Brook catchment (FS CU 2007–2014 dataset).
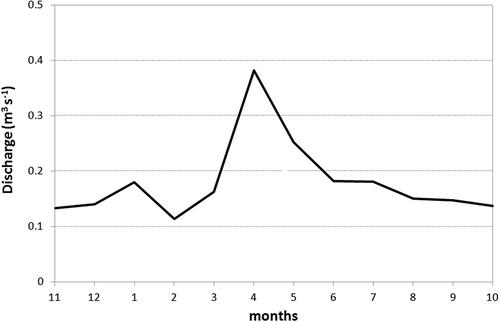
Figure 6. Annual discharge in the outflow from the Rokytka Brook catchment (FS CU 2007–2014 dataset). Dashed line represents mean annual discharge.
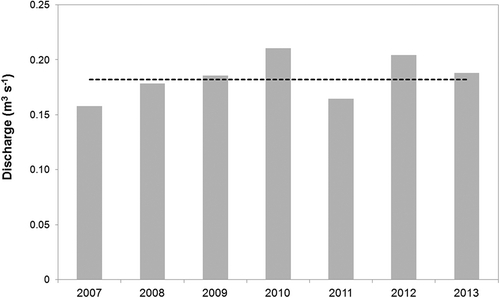
Potential evapotranspiration was calculated using the Penman-Monteith equation (Penman Citation1948) from the set of 2007–2014 data. Evapotranspiration data varied little within the year, with a maximum movement of around 100 mm month−1, see . The precipitation amount did not include water from snow during winter, so it seems quite low compared to the discharge.
Figure 7. Monthly mean precipitation, specific discharge and potential evapotranspiration (pot. ET).
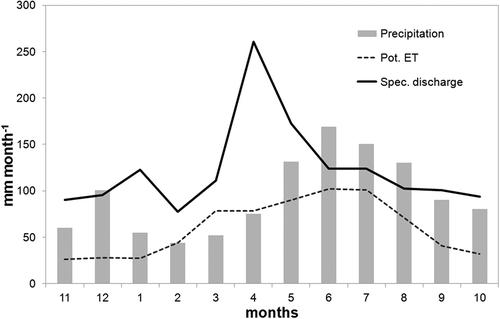
The 2008 hydrological year analysed in the Rokytka Brook profile was, from a runoff and precipitation point of view, quite average and typical for a region with such physical-geographic conditions (see ). Data from the 2008 hydrological year were homogenized and deemed representative and sufficient for detailed analyses. The mean daily and monthly discharges were calculated and the average discharge for 2008 was determined in order to assess general features of the runoff regime in the studied year (). The time series showed a typical course except for the first week of December 2007, when a strong thaw occurred. The actual discharge values during each sampling term are shown in . Sampling of precipitation and of surface water was focused on different levels of discharge (especially on periods of low or high surface runoff) and thus provided sufficient data for partial analyses. Results from hydrological analyses had to be verified using other approaches including geochemical methods (see Section 4.3).
Total runoff (1437 mm) was comparable to the measured amount of precipitation (1485 mm). Such low evapotranspiration is improbable; thus, the input of atmospheric water is apparently undervalued. Horizontal precipitation such as fog or frost probably contributed to the higher rate of total precipitation. The contribution of horizontal deposition in the Šumava Mountains could be estimated at a minimum of 10%. Most elevated locations, including the Rokytka Brook catchment, had a higher horizontal deposition of around 15% (Eliáš et al. Citation1995, Tesař et al. Citation1995, Pavlásek et al. Citation2010).
4.3 Runoff chemistry and balance
4.3.1 Bog
Water in the Rokytka peat bog had low concentrations of dissolved solids and a seasonal δ18O-H2O profile () similar to that for precipitation, which represents the main source of water in the bog. Hydrogen ion concentration (pH) in the bog water is regulated largely by the total organic carbon (TOC) concentration (). TOC showed a strong seasonal profile related to evaporation and organic matter production (low TOC concentration in winter and high concentration in summer). Inherently higher contents of organic acids together with low total mineralization result in low pH and low water alkalinity. Nitrates occur in the bog solely during the winter period, while their source is represented by winter precipitation.
4.3.2 Rokytka Brook tributary
The Rokytka Brook catchment is fed by many tributaries; however, two of them are the most significant. Due to the fact that both affluents showed very similar chemistry, data from the one with higher discharge were analysed. Total mineralization of the Rokytka Brook was higher than that of a bog and its δ18O-H2O profile was more balanced (). The δ18O-H2O balance is a consequence of the prevailing supply from groundwater. The Rokytka Brook can contain water from shallow soil horizons with a higher TOC content, but only during periods of higher precipitation (). The hydrogen ion concentration (pH) of the Rokytka Brook was significantly dependent on discharge ( and ) and the profile of affluent discharge was very similar to that of the brook itself. Increased TOC concentration was related to the production of organic matter during the summer period. No significant correlation between TOC and pH occurred.
4.3.3 Rokytka Brook outflow
The chemistry of the Rokytka Brook in the closing profile is similar to the chemistry of the main tributary. As mentioned previously, the pH of the Rokytka Brook is dependent on actual discharge ().
From the results (), it is evident that the contribution of bog water to the Rokytka Brook runoff was insignificant, moving at the most only around 10% of total runoff outside the winter period. During winter months the bog contribution was negligible and the runoff was formed solely by the tributaries, thus by groundwater. The general character of Rokytka Brook chemistry is derived primarily from sources of water that have been in contact with mineral soils, also during the period of increased runoff (see stable δ18O-H2O, ). A strong argument for the claim that the main sources of Rokytka Brook runoff are represented by its tributaries, supplied mainly by groundwater, is that there was also a high concentration of cations in the brook compared to the bog (). Periodically increased TOC concentrations most likely originated from the riparian zone, from where TOC is washed away in periods of increased runoff. Production of seasonal organic matter would also have had some influence.
5 Discussion
The Šumava Mountains encompass the largest peat bog area in not only the Czech Republic, but in Central Europe, largely due to the specific geology and morphology. However, it is quite complicated to compare data acquired from this type of peat bog with those situated in other parts of Europe, or the rest of the world. Although some of the requirements needed for peat bog development are present in Central Europe, all the necessary conditions for upland peat bog formation are found in Scotland and Scandinavia. Waterlogged areas in Central Europe are found mostly in flat areas or valleys, for example in Biebrza in Poland (Wassen Citation1995) or in western Slovakia (Juráková and Klementová Citation2003). However, the hydrological and climatic conditions in these regions are different from those in mountainous bog regions. This paper presented new and unique findings about the role of peat bogs in the specific conditions typical of Central Europe. It is crucial to be aware of this fact and to take it into consideration.
Opinions on the role of peat bogs in rainfall–runoff processes in the Šumava Mountains have changed dramatically during the last 150 years. A general consensus has appeared in the last five decades. The first hydrological survey on this topic was carried out by Ferda et al. (Citation1971) and followed by Janský and Kocum (Citation2008). Čurda et al. (Citation2011) then provided more accurate results comparing two sources of the Vydra River with different peat proportions in their catchments, confirming previous research. Importantly, they showed that the role of peat bogs in rainfall–runoff processes starts to be observed from about 15 mm rainfall during drought and about 20 mm during maximum saturation. What is more, groundwater level measurements showed that peat bogs generate high outflow in the case of full saturation, as Vlček et al. (Citation2012) claimed.
On the basis of the current observations of surface runoff, we have undertaken detailed analyses of ascending and descending phases of extreme runoff, and minimum discharges in profiles closing several sub-catchments with different physical-geographic conditions, including the Rokytka Brook catchment. In general, our studies showed higher peak flow frequency and shorter reaction to causal amounts of precipitation in the highly peaty areas. In other words, the studies showed greater runoff variability of streams draining peatland localities (Janský and Kocum Citation2008, Čurda et al. Citation2011, Vlček et al. Citation2012). The Rokytka Brook experimental catchment represents a highly peaty area with a runoff regime of significant variability compared to other watersheds. Data from the 2008 hydrological year proved it to be a representative year, with sufficient information for detailed analyses.
The exactness of the hydrological balance calculation in such a small experimental catchment can be affected by some possible errors. On the basis of the acquired data, the input data presented by precipitation could be undervalued, the outflow data could be overvalued due to an inaccurate consumption curve, or there is an underground inflow, which is not probable. Amounts of total runoff and measured precipitation (see Section 4.2; 1437 mm) point to the fact that the input of atmospheric water is apparently undervalued. Although it represents a less important component of wet deposition, from the quantitative point of view, horizontal precipitation (fog and frost) probably contributes to a higher rate of total precipitation. Tesař et al. (Citation1995) stated that the contribution of horizontal deposition in the Šumava Mountains could be estimated as at least 10%. Most elevated areas, including the Rokytka Brook catchment, accounted for even higher horizontal deposition of around 15% (Eliáš et al. Citation1995, Pavlásek et al. Citation2010).
On the basis of the findings using a hydrological approach, a more detailed hydrochemical and geochemical analysis was undertaken. Geochemical data showed no significant hydraulic connection of the studied bog to the Rokytka Brook bed. The contribution to surface runoff by water from the bog was very insignificant, moving a maximum of around 10% outside the winter period. The predominance of underground water (forced out due to the pressure gradient) in total runoff was also confirmed by separation of each runoff component according to geochemical parameters. Several experts (Conway and Millar Citation1960, Burke Citation1967, McDonald Citation1973, Moklyak et al. Citation1975, Baird Citation1997, Holden et al. Citation2001) have undertaken detailed studies of peatland hydrological function, including drainage procedures such as diking of former drainage channels, in various regions with different physical-geographic backgrounds. The findings are often very discrepant. Accordingly, the problem of hydraulic communication between peat bog complexes and draining streams needs to be solved strictly with respect to local physical-geographic conditions.
From a hydrological point of view, in these physical-geographic conditions peatland acts as a negative element for runoff transformation and its hydrological features are not favourable. Findings from our geochemical analyses therefore confirmed our primary hydrological assumption of a negative and insignificant impact of peatland on runoff dynamics, especially during flood and drought episodes. The weak influence of the peat bog is also demonstrated by a high concentration of cations in the surface runoff compared to the bog. Groundwater from the catchment is a much greater contributor to the surface runoff of the Rokytka Brook. In general, a very close correlation between pH and actual discharge in the experimental profiles located on the Vltava River headwaters was found, corresponding to Worrall et al. (Citation2003). A reasonably close relationship was also observed in the closing profile of the Rokytka Brook catchment.
Detection of natural tracers is a useful method to provide key information in hydrological observation studies of catchment runoff formation. Studies of water dynamics by means of natural tracers typically use oxygen (18O) and hydrogen (2H) isotopes (Kendall and McDonnell Citation1999). This approach is very favourable for the study of the hydrological balance under Central European conditions. Stable oxygen and hydrogen isotopes are elements that occur naturally, in variable concentrations, in the hydrological cycle. The isotope ratio provides unique information about the water that enters a catchment in the form of precipitation, the water that is retained in the catchment and the water that flows out in the form of runoff. The approaches of Hoeg et al. (Citation2000) and Klaus and McDonnell (Citation2013) showed the potential of using natural tracers, such as oxygen isotopes, to give good insight into the mechanism of runoff creation, especially in the studied area. Subsequent analyses need to be carried out in a field laboratory such as the Rokytka Brook catchment.
6 Conclusions
Using a combination of modern hydrochemical and geochemical methods it is now possible to investigate hydrological processes in experimental catchments in much more detail. These methods complement classical hydrological statistics and open up new opportunities to perform detailed studies of the “big picture” of streamflow generation processes under specific conditions. Statistical analysis of a hydrological time series, specifically the rate of variability of the runoff regime, for the catchment chosen in this study, correlated with results carried out using ion, carbon and oxygen isotope balance observations. Hydrological function in the study area appeared to be insignificant. Considerable runoff variability in highly peaty areas, together with the minimum hydraulic connectivity of peat bog complexes with water stream beds, is of fundamental importance for our understanding of the behaviour of peatland areas in the highly exposed Vltava River headwaters. It is even more crucial that the area of the Vydra River headwaters is being monitored in terms of flood protection, drought prevention, and use as a potential reserve of freshwater. Proper understanding of the mechanisms of runoff generation processes in this area would certainly improve prediction of extreme hydrological episodes.
Disclosure statement
No potential conflict of interest was reported by the authors.
Additional information
Funding
References
- Baird, A.J., 1997. Field estimation of macropore functioning and surface hydraulic conductivity in a fen peat. Hydrological Processes, 11, 287–295. doi:10.1002/(ISSN)1099-1085
- Bufková, I., 2009. Ochrana rašelinišť na Šumavě aneb byly Rokytecké slatě první? In: D. Černý and L. Dvořák, eds. Weitfällerské slatě. Vimperk: Správa NP a CHKO Šumava, 12–23.
- Burke, W., 1967. Principles of drainage with special reference to peat. Irish Forestry, 24, 1–7.
- Bůzek, F., 1984. A Rapid Procedure for Preparing Oxygen-18 Determination in Water Samples. Isotopenpraxis, 19, 70–72.
- Conway, V.M. and Millar, A., 1960. The hydrology of some small peat-covered catchments in the northern Pennines. Journal of the Institute of Water Engineers, 14, 415–424.
- Craig, H., Gordon, L.I., 1965. Deuterium and oxygen-18 variations in the ocean and marine atmosphere. In: E. Tongiorgi, ed. Proceedings of conference on stable isotopes in Oceanic Studies and Paleotemperatures. Spoleto: Labouratory of Geology and Nuclear Science, 9–130.
- Čurda, J., Janský, B., and Kocum, J., 2011. Vliv fyzickogeografických faktorů na extremitu povodní v povodí Vydry. Geografie – Sborník ČGS, 116 (3), 335–353.
- Eliáš, V., Tesař, M., and Buchtele, J., 1995. Occult precipitation: sampling, chemical analysis and process modelling in the Šumava Mts. (Czech Republic) and in the Taunus Mts. (Germany). Journal of Hydrology, 166, 409–420. doi:10.1016/0022-1694(94)05096-G
- Ferda, J., 1960. Hydrologický význam horských vrchovištních rašelinišť. Sborník ČSAZV – Lesnictví, 10, 835–856.
- Ferda, J., et al., 1971. Odtokový režim a chemismus vod v povodí Horní Otavy se zaměřením na výskyt rašelinišť. Sborník Prací HMÚ, 17, 22–126.
- Freeman, C., et al., 2001. Export of organic carbon from peat soils. Nature, 412, 785–786. doi:10.1038/35090628
- Hoeg, S., Uhlenbrook, S., and Leibundgut, C., 2000. Hydrograph separation in a mountainous catchment - combining hydrochemical and isotopic tracers. Hydrological Processes, 14, 1199–1216. doi:10.1002/(SICI)1099-1085(200005)14:7<1199::AID-HYP35>3.0.CO;2-K
- Holden, J., Burt, T.P., and Cox, N.J., 2001. Macroporosity and infiltration in blanket peat: the implications of tension disc infiltrometer measurements. Hydrological Processes, 15, 289–303. doi:10.1002/(ISSN)1099-1085
- Holden, J., Chapman, P.J., and Labadz, J.C., 2004. Artificial drainage of peatlands: hydrological and hydrochemical process and wetland restoration. Progress in Physical Geography, 28 (1), 95–123. doi:10.1191/0309133304pp403ra
- Horton, R.E., 1933. The role of infiltration in the hydrologic cycle. Transactions American Geophysical Union, 14, 446–460. doi:10.1029/TR014i001p00446
- Hruška, J., et al., 1996. Role of organic solutes in the chemistry of acid-impacted bog waters of the western Czech Republic. Water Resources Research, 32 (9), 2841–2851. doi:10.1029/96WR01104
- Hruška, J., Kohler, S., and Bishop, K., 1999. Buffering processes in a boreal dissolved organic carbon - rich stream during experimental acidification. Environmental Pollution, 106 (1), 55–65. doi:10.1016/S0269-7491(99)00061-5
- Janský, B. and Kocum, J., 2008. Peat bogs influence on runoff process: case study of the Vydra and Křemelná River basins in the Šumava Mountains, southwestern Czechia. Geografie - Sborník ČGS, 113 (4), 383–399.
- Juráková, M. and Klementová, E., 2003. Status and trends of the wetlands in west Slovakia. Slovac Journal of Civil Ingeneering, 3, 1–8.
- Kendall, C. and McDonnell, J.J., 1999. Isotope tracers in catchment hydrology. Amsterdam: Elsevier Science B.V.
- Kirchner, J.W., 2003. A double paradox in catchment hydrology and geochemistry. Hydrological Processes, 17, 871–874. doi:10.1002/(ISSN)1099-1085
- Klaus, J. and McDonnell, J.J., 2013. Hydrograph separation using stable isotopes: Review and evaluation. Journal of Hydrology, 505, 47–64. doi:10.1016/j.jhydrol.2013.09.006
- Kocum, J., 2012. Tvorba odtoku a jeho dynamika v pramenné oblasti Otavy. Thesis (PhD). Charles University in Prague.
- Kocum, J. and Janský, B., 2009. Retence vody v pramenných oblastech Vydry a Křemelné – případová studie povodí Rokytky. In: D. Černý and L. Dvořák, eds. Weitfällerské slatě. Vimperk: Správa NP a CHKO Šumava, 26–48.
- Kocum, J., et al., 2010. Extremita odtoku v pramenné oblasti Otavy (řešení negativních dopadů klimatické změny). In: M. Vrabec, I. Durčanský, and J. Hladný, eds. Hydrologické dny 2010 - Voda v měnícím se prostředí. Praha: Nakladatelství ČHMÚ, 597–604.
- McDonald, A., 1973. Some views on the effects of peat drainage. Scottish Forestry, 27, 315–327.
- McDonnell, J.J., 2003. Where does water go when it rains? Moving beyond the variable source area concept of rainfall–runoff response. Hydrological Processes, 17, 1869–1875. doi:10.1002/(ISSN)1099-1085
- Moklyak, V.I., Kubyshkin, G.P., and Karkutsiev, G.N., 1975. The effect of drainage works on streamflow. Hydrology of marsh-ridden areas, Minsk, USSR, 1972. IAHS Studies and Reports in Hydrology, 19. Paris: Unesco Press, 439–446.
- Novák, M., 1955. Huminové vody ve vodách údolních nádrží. Vodní Hospodářství, 5 (4), 127–128.
- Novák, M., 1959. Výzkum kvality vody v údolní nádrži Lipno. Vodní Hospodářství, 9 (9), 378–383.
- Onderíková, V. and Štěrbová, A., 1956. Prispevok k biologii a chemizmu Oravskej nádrže. Vodní Hospodářství, 6 (2), 46–51.
- Oulehle, F. and Janský, B., 2003. Limnologie a hydrochemismus v NPR Rejvíz. In: B. Janský and M. Šobr, eds. Jezera České republiky (Současný stav geografického výzkumu). Prague: Charles University in Prague, 93–108.
- Pavlásek, J., et al., 2010. Ten years of hydrological monitoring in upland microcatchments in the Bohemian Forest, Czech Republic. IAHS-AISH Publication, 336, 213–218.
- Penman, H.L., 1948. Natural evaporation from open water, bare soil and grass. Proceedings of the Royal Society A: Mathematical, Physical and Engineering Sciences, 193, 120–145. doi:10.1098/rspa.1948.0037
- Pošta, P., 2004. Organogenní jezera v České republice. Thesis (Master). Charles University in Prague.
- Šanda, M., 2010. Přirozené stabilní izotopy kyslíku a vodíku v hydrologii experimentálního povodí. In: M. Vrabec, I. Durčanský, and J. Hladný, eds. Hydrologické dny 2010 - Voda v měnícím se prostředí. Praha: Nakladatelství ČHMÚ, 255–261.
- Šanda, M., Kulasová, A., and Císlerová, M., 2009. Hydrological processes in the subsurface investigated by water isotopes and silica. Soil and Water Resources, 4, 83–92.
- Šefrna, L., 2004. Pedologická charakteristika povodí Otavy ve vztahu k povodním [online]. Charles University in Prague. Available from: http://hydro.natur.cuni.cz/zmeny_povodni/pdf/sefrna.pdf [Accessed 8 July 2009].
- Tesař, M., Eliáš, V., and Šír, M., 1995. Preliminary results of characterization of cloud and fog water in the mountains of Southern and Northern Bohemia. Journal of Hydrology and Hydromechanics, 43 (6), 412–426.
- Vlček, L., et al., 2012. Retenční potenciál a hydrologická bilance horského vrchoviště: případová studie Rokytecké slatě, povodí horní Otavy, JZ. Geografie - Sborník ČGS, 117 (4), 371–395.
- Wassen, M.J., 1995. Hydrology, water chemistry and nutrient accumulation in the Biebrza fens and floodplains (Poland). Wetlands Ecology and Management, 2, 125–137.
- Worrall, F., Burt, T.P., and Adamson, J.K., 2003. Controls on the chemistry of runoff from an upland peat catchment. Hydrological Processes, 17, 2063–2083. doi:10.1002/(ISSN)1099-1085