ABSTRACT
Understanding the isotopic composition of precipitation in a forested catchment is critical for ecohydrological studies. Changes in the water isotopes of rainfall were assessed during its passage through the canopy in throughfall, and the effect of different forest stands on the isotope composition of throughfall. In a cool temperate forest in Korea, rainfall and throughfall samples collected under Pinus densiflora (red pine), Castanea crenata (chestnut), Robinia pseudoacacia (black locust) and mixed stands (mix of these three species) were analysed for oxygen and hydrogen isotopes. Throughfall δ18O and δD were enriched compared to rainfall. A difference of δ18O and δD among throughfall may be related to the difference in interception–storage capacity of different species due to dissimilar canopy characteristics. Since isotopic composition of throughfall and rainfall are different due to canopy isotopic effects, use of rainfall isotopic signatures for ecohydrological studies in forested ecosystem can lead to biases.
Editor M.C. Acreman Associate editor M.D. Fidelibus
Introduction
Forest ecohydrology is intimately tied to the relationship between trees and incident precipitation (Pypker et al. Citation2005, McGuire and McDonnell Citation2007, Carlyle-Moses and Gash Citation2011, Levia and Germer Citation2015). Oxygen (18O) and hydrogen (2H or D) isotopes of water are widely used to constrain the hydrology and water budgeting of various forested ecosystems (Bayard et al. Citation2005, Cui et al. Citation2009, Sprenger et al. Citation2016). Knowledge of oxygen and hydrogen isotope fractionation and their subsequent spatial and temporal variabilities among different water reservoirs has helped to highlight the hydrological relationship between precipitation, vegetation, soil water and groundwater (Clark and Fritz Citation1997, Kendall and McDonnell Citation1998, Lee et al. Citation2003, Liu et al. Citation2008).
The presence of forested landscape causes isotopic changes in the infiltrating water (Brodersen et al. Citation2000, Hsueh et al. Citation2016, Allen et al. Citation2017). Depending upon vegetation types, a plant canopy can intercept and temporarily store a substantial amount of precipitation (Pypker et al. Citation2005). Interception losses range from as low as 6.2% of total annual rainfall in the savanna, to as high as 45% in coniferous forests (Carlyle-Moses and Gash Citation2011). Thus, the interaction between three processes: (1) interception manifested in the form of a canopy storage/selection effect (Ikawa et al. Citation2011, Xu et al. Citation2014, Hsueh et al. Citation2016); (2) re-evaporation between precipitation events (Gat and Matsui Citation1991, McGuire and McDonnell Citation2007); and (3) the isotopic mixing of intercepted precipitation with the water vapour and pre-event water trapped in tree canopies (Saxena Citation1986, Allen et al. 2014, 2017), can cause throughfall and stemflow water, collected in the sub-canopy, to be isotopically different from water collected above the canopy (DeWalle and Swistock Citation1994, McGuire and McDonnell Citation2007). When determining rainfall and throughfall/stemflow 18O and D isotopic composition, it is important to identify the factors that govern the final isotopic composition of water in the canopy. The isotopic signatures of precipitation traversing the tree canopy are altered by vegetation type, canopy architecture and leaf-to-branch ratio. Apart from this, intensity and duration of precipitation, and other regional to local meteorological conditions also influence isotopic signatures (Brodersen et al. Citation2000, Allen et al. Citation2017).
There exist several studies that characterize the behaviour of 18O and D isotopes in incident precipitation. However, far fewer studies exist that examine the composition of 18O and D isotopes in throughfall and stemflow (McGuire and McDonnell Citation2007, Zhang et al. Citation2010, Ikawa et al. Citation2011, Xu et al. Citation2014, Hsueh et al. Citation2016). Despite the importance of the forest’s role in influencing the hydrology of a region, throughfall isotopic studies for most forest ecosystems are not prioritized. Isotopic signatures of rainfall rather than throughfall are used to strengthen understanding about the various ecosystem processes, including forest ecosystems. The canopy-related effect of interception, storage and re-evaporation on the isotopic concentration of rainfall has often been neglected for forested regions (Kubota and Tsuboyama Citation2003). In forested ecosystem, it is necessary to determine the contribution of the canopy in altering throughfall isotope signatures, so that water isotope-based studies can take note of the modified 18O and D isotopic ratios in forest ecohydrology. Such understanding will help in interpreting the results of various ecosystem-based studies that use 18O and D isotopes (Brodersen et al. Citation2000, Allen et al. Citation2017). In this context, an improved understanding of the control of the seasonal and spatial distribution of water in the rainfall–throughfall interface is desirable, to provide scientific input for better planning of forest ecohydrological strategies.
In this study, inter- and intra-storm 18O and D (or 2H) variations in rainfall and throughfall were used to compare differences in their isotopic compositions in the cool temperate forest stands of different canopy characteristics. The objectives of the study are to (1) quantify the spatial isotope variability of throughfall in relation to differing species-specific stands characterizing different structures, and (2) then compare the isotopic composition of rainfall with throughfall, and the local meteoric water line (LMWL) to understand the role of vegetation in this forested catchment.
Materials and methods
Study site
The study was carried out in the cool temperate forests of Mt Mokryeong, South Korea (33°43ʹN latitude and 127°26ʹE longitude) (). The cool temperate forests growing in this region are secondary in origin, being about 40 years old. Pinus densiflora Siebold and Zucc. (red pine) (conifer), Castanea crenata Siebold and Zucc. (chestnut) (broadleaved with whole leaf) and Robinia pseudoacacia L. (black locust) (broadleaved with compound leaf) were planted during the reforestation programme of the 1980s. The total stem density of red pine was 654 ha−1 and the average tree height was 15.5 m. The total stem density and average height of chestnut were 354 ha−1 and 12.5 m, and those of black locust were 310 ha−1 and 18.5 m. The average tree density in mixed stands was 530 ha−1.
The altitude of the study area ranged between 85 and 248 m above mean sea level. The annual mean temperature during the study period was 12°C; with a mean minimum temperature of 6.5°C in January and mean maximum temperature of 17.3°C. The annual rainfall in the area during the study period was 1448 mm, which was distributed into 1079 mm summer rainfall and 202 mm winter rainfall. These values are identical to the long-term mean average values for the region (Korea Meteorological Administration, www.kma.go.kr). During winter, snow starts accumulating in the mountainous regions in mid-December and the study area remains covered by snowpack until mid-spring.
Sampling and analysis
Thirteen throughfall and precipitation events were collected from 23 April 2012 to 4 February 2013. Four different stands within the forests were selected, characterized by three species, red pine, chestnut and black locust, and a fourth mixed plantation. In each stand, three trees were selected, differing in diameter, size and height, based on ocular estimation. Under each tree, throughfall apparatuses (three in number) were placed midway between the main stem and the canopy edge. To collect rainfall, apparatuses were placed in the open area, outside the forests. A polyethylene funnel of 21 cm diameter (Nalgene™) fitted with 2-mm polyethylene mesh at the bottom of the mouth was used to channel throughfall and rainfall into a 10-L fluorinated, high-density polyethylene container (Nalgene™). All the containers were wrapped in aluminium foil to mask them from direct sunlight. For snow collection, 50-L, wide-mouth collection buckets were used. After every event, funnels and collection apparatuses were washed with deionized water (>18 MΩ) (Pure Power II+ water purification system, Human Corporation, Seoul, Korea) and air dried.
Collected samples were brought to the laboratory immediately after the end of every storm event and filtered using 0.45-µm pore size filter paper (Advantec® mixed cellulose membrane 47 mm size). Filtrate was collected in a 1-L polystyrene filterware (Nalgene™) and subsequently stored in a refrigerator at 2°C until isotopic analysis. In the case of snow, samples were retrieved immediately in large Ziploc® bags after the snowfall to prevent melting-related losses, brought to the laboratory, and immediately stored in a refrigerator at −20°C until isotopic analysis. Two samples were lost due to funnel breakage and one sample was discarded because it was contaminated with bird droppings.
For oxygen isotopic analysis, about 2 mL of each rainfall/throughfall sample was equilibrated with tank carbon dioxide gas at 25°C. The gas was then extracted and cryogenically purified. For deuterium analysis, metallic chromium was used to produce hydrogen gas using an automatic on-line sample preparation system (GV Instruments, Euro PyrOH, UK). The oxygen and hydrogen isotope compositions were measured using two isotope ratio mass spectrometers (IRMS; GV Instruments IsoPrime and Micromass Optima, UK) at the Korea Basic Science Institute. The stable isotopic compositions were expressed in δ notation relative to Vienna Standard Mean Ocean Water (VSMOW) for oxygen and hydrogen isotopes:
where R = D/H or 18O/16O. The analytical reproducibility for each standard and sample was ±0.1‰ for δ18O and ±0.5‰ for δD. During the sample analysis, the laboratory standards for each isotope were run after every nine samples.
Data analysis
Volume-weighted means were calculated for rainfall and throughfall using the equation δWA = Σ(Pi δi)/ΣPi, where δi is measured isotopic value during the precipitation event and Pi is precipitation (mm) during the period (Lee et al. Citation2003). The relationship of δD versus δ18O for all the waters, and the local meteoric water line (LMWL) were obtained using linear regression analyses. Afterwards, LMWLs were then compared with the global meteoric water line (GMWL) of Craig (Citation1961), which shows the global relationship between δD and δ18O of different waters. If the slope of the LMWL is lower than 8, it suggests the role of evaporation. We have calculated the deuterium excess value (d-excess value, d) following Dansgaard (1964) as d = δD – (8 × δ18O), to identify the processes that contribute to the variations in the isotopic composition of precipitation. Deviation of d-excess values from the LMWL (higher or lower than 10‰) shows the effect of source air masses, humidity, temperature and evaporation (Gat Citation1996, Lee et al. Citation2003). The isotopic results for rainfall and throughfall samples were subjected to ANOVA using XLSTAT-pro v. 13.2.1.0 (Addinsoft, New York, USA). Graphical visualization of the lines of best fit was produced using Sigmaplot 10.0 (SYSTAT, Chicago, USA).
Results
During the study period, the isotopic compositions of rainfall and throughfall varied among events (Fig. 2). Rainfall δ18O values range from −14.36‰ to −4.16‰ with a weighted mean value of −9.20‰ (±2.59). And δD values range from −84.7‰ to −18.8‰, with a weighted mean value of 62.4‰ (±18.4) (). In the case of throughfall, mean δ18O values (weighted) range from −8.91‰ (±2.50) in black locust to −8.69‰ (±2.22) in red pine. Annual mean δD range from −58.1‰ (±18.2) in chestnut to −56.6‰ (±17.8) in red pine. Comparison of individual throughfall events with corresponding rainfall events shows species-specific differences in δ18O values ().
Table 1. Average stable isotopic composition and d-excess values of water by type in the cool temperate region.
The adjusted r2 for all the δD–δ18O best-fit LMWLs ranges from 0.82 to 0.87. The slope values for throughfall in this study range from 6.89 in black locust to 7.46 in red pine, with a slope of 6.65 for rainfall. Seasonally, the LMWLs for summer and winter precipitation differ significantly (). The slopes and intercepts (y) of best-fit lines are higher in summer LMWLs compared to winter LMWLs (). Summer LMWLs lie right of the GMWL, whereas winter LMWLs lie left of the GMWL (). The isotopic compositions of winter precipitation exhibit a greater scatter than summer precipitation (). The slopes of summer and winter precipitation (except winter rainfall) almost match the GMWL. In the case of the intercept (y), winter precipitation (except rainfall) LMWL intercepts approximate the GMWL intercept of 10. However, for the summer precipitation, intercepts here are much higher than the GMWL intercept of 10, ranging from 11.6 (mixed stand) to 16.4 (red pine). The slope of winter rainfall at 6.38 is lower, including the slopes of winter throughfall precipitations, though not considerably, than that of GMWL.
Table 2. Seasonal oxygen-18, deuterium, d-excess values, and seasonal local meteoric water line (LMWL) showing δ18O–δD relationship of various precipitation types.
Figure 1. Map of Korean peninsula (a) showing sampling locations (b) and (c). Inset shows the plan of sample collection at each site. Each point shows the placement of the three throughfall apparatuses used for sample collection at each site.
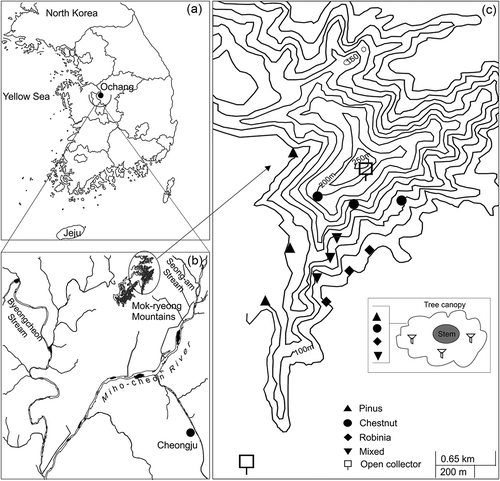
Figure 2. Oxygen (δ18O) and deuterium (δD) isotopic compositions and d-excess values (‰) (primary y-axis) of rainfall (●) (a), and red pine (□), black locust (○), chestnut (×) and mixed stand (∆) throughfall events (b) collected in cool temperate forests, South Korea. Secondary y-axis shows the amount of rainfall and throughfall (mm). Horizontal dashed lines show weighted-average isotopic values. Shaded area shows snowfall samples.
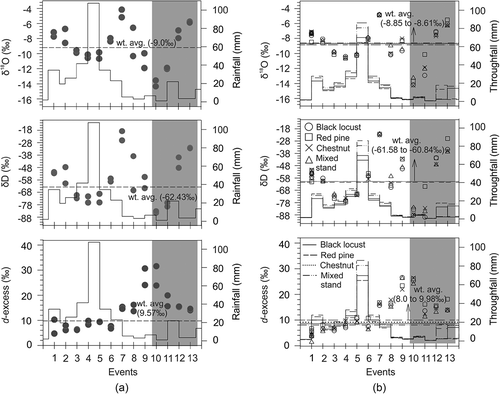
Figure 3. Differences in δ18O isotopic composition between throughfall (δ18OTh) and rainfall (δ18ORf) (primary y-axis; black closed columns) and throughfall amount recorded during events (secondary y-axis, downward grey columns) in (a) red pine, (b) mixed stand, (c) chestnut and (d) black locust stands. The values above the x-axis (upward black columns) indicate throughfall enriched compared to rainfall and those below the x-axis (downward black columns) indicate throughfall less enriched compared to rainfall. Shaded area shows snowfall samples.
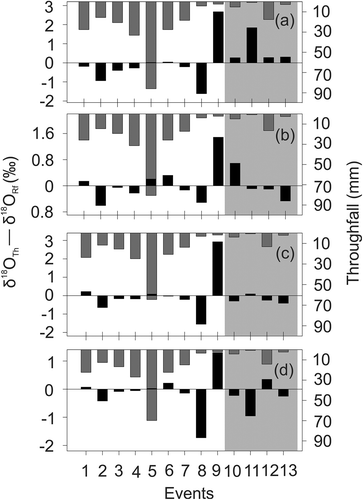
Figure 4. Covariation plots of δD–δ18O of all (a) and seasonal (b) and (c) precipitation samples collected in the study from cool temperate forests, South Korea. Global meteoric water line (GMWL) (grey dashed line) with δD = (8 × δ18O) + 10 (Craig Citation1961) is plotted for reference. Local meteoric water line (LMWL) is based on the sample collected in the study.
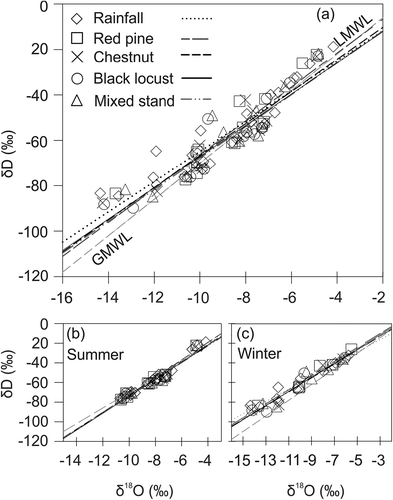
Weighted mean d-excess values for rainfall and all throughfall lie close to 10‰ of the GMWL ( and ). Both rainfall and throughfall d-excess values vary significantly between various events (ANOVA P < 0.0001). Among throughfall, weighted d-excess values differ from each other and range from 8.0‰ (±7.2) in the mixed stand to 10.0‰ (±6.6) in red pine. Seasonally, mean d-excess values for summer precipitation (throughfall and rainfall) (≤10‰) differ substantially from those for winter precipitation (>17‰) ().
Discussion
Our δ18O rainfall isotopic results are in agreement with the long-term average rainfall isotopic values of the IAEA-GNIP station in Korea (Cheongju) for the period of 1998–2009 (−2.65 to −16.70‰, with an average of −8.13‰). However, the average δD isotopic value of rainfall over the cool temperate forests in this study on average is −5.3‰ higher than the long-term IAEA-GNIP results (−11.3 to −124.4‰ with an average of −52.9‰) (IAEA Citation2012).
Oxygen and hydrogen isotopic values vary spatially among different throughfall, though only by a small amount and non-significantly. The differences of 0.1–0.25‰ for δ18O and −0.7 to +0.7‰ for δD noticed among different throughfalls are consistent with Brodersen et al. (Citation2000). Seasonally also, different species showed differences in throughfalls. Differences in δ18O among different species in summer range from 0.02 to 0.25‰ and in winter the range is 0.10–0.88‰. Differences in the isotopic composition of different throughfalls are more visible in the case of δD. Differences in the isotopic signatures of deuterium are 0.2–0.4‰ in summer and 2.2–8.0‰ in winter. This could be tied to the differences in the canopy activities, rate of evaporation and moisture source between the two seasons. Among all the species, winter throughfall of red pine is generally enriched in δ18O and δD as compared to other species. This may be because of the winter green canopy surface of red pine, which allows selective storage even in winter and offers longer residence time for snow to stay in its canopy compared to other species.
Overall throughfall in the study is enriched by 0.17–0.36‰ in δ18O and by 0.9–1.6‰ in δD over rainfall, and the results of this study are consistent with the pattern of enrichment observed elsewhere (Dawson and Simonin Citation2011, Allen et al. Citation2017 and references therein). Seasonally, summer throughfall is 0.52–0.77‰ enriched, whereas winter throughfall is 0.08–0.62‰ enriched (except for black locust, −0.26‰ depleted) compared to rainfall. In a forested ecosystem, a significant quantity of incident precipitation is intercepted and held in the tree canopies (i.e. canopy storage), and subsequently evaporates. Commonly suggested processes of canopy effects, i.e. selective canopy storage (DeWalle and Swistock Citation1994, Brodersen et al. Citation2000, Dawson and Simonin Citation2011, Xu et al. Citation2014), molecular exchange processes of atmospheric water vapour with intercepted water due to nonequilibrium conditions resulting from dissimilar isotopic values (Brodersen et al. Citation2000), and pre-event effects (Allen et al. Citation2014), during and after rainfall events, lead to the alteration in the isotopic composition of throughfall (as well as stemflow) collected below tree canopies.
Small increases in throughfall isotopic ratios compared to rainfall suggest that selective canopy storage of rainfall from the end of an event, when rainfall is typically depleted in heavy isotopes and may completely evaporate, produces enriched throughfall compared to rainfall. In our study, relatively enriched isotopic signals and lower d-excess value as compared to rainfall are consistent with an evaporation effect (Allen et al. Citation2017). All the events plot close to the LMWL and the slopes of throughfall δ18O–δD regressions are close to 8. These conditions indicate that intercepted water has undergone limited evaporation during the precipitation events. Deuterium excess values calculated as d = δD − (8 × δ18O) (Dansgaard 1964), if they exceed that of the LMWL, suggest the role of evaporation and mixing of evaporated water with throughfall (Liu et al. Citation2007).
We notice d-excess values close to 10‰, which suggest small intra-event evaporation (Clark and Fritz Citation1997, Lee et al. Citation2003). Therefore, we assume that the isotopic patterns in our study probably indicate the effect of selective canopy storage on the throughfall isotopic composition in cool temperate forests. However, storage describes the net capacity of a canopy to intercept water rather than processes that generate effects on throughfall isotopic signatures. In addition to this, isotopic data that suggest evaporation are also suggestive of a selection effect (Allen et al. Citation2017). Two consecutive precipitation events separated by 24 hours (events 9 and 10) indicate a pre-event canopy storage effect, as suggested by Allen et al. (Citation2014). Though a difference in the isotopic composition exists in rainfall also for these two events (3.02‰ for δ18O and 23.8‰ for δD), the isotopic difference between the aforementioned two events in the throughfalls is much higher compared to rainfall. The isotopic difference was 5.42‰ for δ18O and 40.7‰ for δD in P. densiflora, 4.54‰ for δ18O and 37.7‰ for δD in R. pseudoacacia, 3.81‰ for δ18O and 32.7‰ for δD in the mixed stand, and 6.24‰ for δ18O and 45.4‰ for δD in C. crenata. This establishes the potential role of residual moisture from previous precipitation events in altering the isotopic composition of throughfall.
The individual TF events of different species show considerable variations in enrichment and depletion relative to RF. The highest δ18O enrichment ranges from 1.49 to 2.93‰, whereas the highest depletion ranges from −0.61 to −1.75‰ (). This suggest that the magnitude of isotopic effects differs among species, possibly due to the difference in canopy architecture and its effect on the flow path (of water), the within canopy environment, evaporation and storage.
The patterns of δD and δ18O change among events are almost identical for various throughfall and rainfall (). However, individual events in this study show considerable variations of enrichment and depletion in isotopic composition (), similar to the differences observed by Brodersen et al. (Citation2000). These differences are noticeable seasonally. We notice that mean δ18O values of winter rainfall and throughfall are lower than the mean δ18O values of the summer rainfall and throughfall. This is because of the relatively depleted isotopic composition of snow precipitation.
Generally, δ18O and δD values of precipitation decrease with increasing rainfall (amount effect) and decreasing air temperature (temperature effect) (Clark and Fritz Citation1997, Sharp Citation2007). A comparison of individual events reveals the lowest δ18O and δD during winter, corresponding to snowfall, which coincides with the lowest air temperature (≈ −10°C). Such highly depleted isotopic composition corresponding to snow has been reported elsewhere (Koeniger et al. Citation2008), including Korea (Lee and Kim Citation2007, IAEA. Citation2012). However, one winter non-snow sample shows relatively enriched δ18O and δD values. In summer, the lowest δ18O and δD values in the study overlap with the events where precipitation amounts are highest. Previous stable isotopic studies of the precipitation for the region also showed such a pattern (Araguás-Araguás et al. 1998, Lee et al. 1999).
A number of studies suggest that the d-excess value is a better tracer of isotopic changes during water channelling than δD and/or δ18O (Cui et al. Citation2009, Dawson and Simonin Citation2011). In this study, mean d-excess values for both rainfall and throughfall lie close to 10‰ of the GMWL. And differences in the isotopic values between rainfall and various throughfall are small and non-significant. However, that is not the case with the d-excess values. The d-excess values of throughfall and rainfall differ significantly (P < 0.01). The throughfall d-excess value is 0.4‰ (chestnut) to 1.6‰ (mixed stands) higher than the rainfall d-excess values, except for red pine (). This could possibly be because of the canopy effect on the interception–evaporation interplay (Cui et al. Citation2009, Zhang et al. Citation2010, Dawson and Simonin Citation2011). Gat and Matsui (Citation1991) also observed an increase of 3‰ in the d-excess value of the isotopic composition of precipitation over Amazon forests. They interpreted this increase in d-excess value to the cumulative effect of re-evaporation from the canopy of the rainforests.
Furthermore, d-excess values differ significantly among different throughfalls (P < 0.001). The d-excess values are in the decreasing order of red pine > chestnut > black locust > mixed stands. We recorded 0.6–2.0‰ difference in the d-excess values among different throughfalls. This indicates that during precipitation events different amounts of water re-evaporate from the canopies through species-specific differential evaporation rates, due to heterogeneous canopy characteristics, and related processes (Zhang et al. Citation2010). Differences in the d-excess values also exist when we binned all the throughfall events into two different seasons. In the summer, d-excess values range from 8.7 to 10.1‰ in the order of red pine > chestnut > black locust > mixed species, with differences of 0.1–1.4‰ among the four different throughfalls. In the winter, d-excess values are higher than summer, and are in the range of 17.2–19.3‰ in the decreasing order of red pine > black locust > mixed species > chestnut. The winter-specific d-excess value differences among the four different throughfalls are in the range of 0.4–2.1‰.
Here, results indicate that vegetation can modify the d-excess values of the precipitation and the change is higher in winter than in summer. The d-excess values are generally regulated by the meteorological conditions of the sampling region, i.e. temperature, relative humidity and wind regime (Gat Citation1996, Lee et al. Citation2003). In a forested ecosystem, tree stands moderate below-canopy air temperature and relative humidity, and create a specific understory microclimate that differs from the surrounding local climate. This moderation differs from species to species due to the complex interplay of several stand characteristics and physiographic settings (von Arx et al. Citation2012). The differences in the d-excess values among the four different throughfalls can be related to the specific microclimatic conditions created by the species-specific canopy studied here.
The d-excess values of this study overlap with seasonal and annual d-excess values reported by Lee et al. (Citation2003) for Korean precipitation. Throughfall during the winter season is relatively depleted in δ18O, with a relatively low intercept compared to throughfall during the rainy summer season. In addition, secondary re-evaporation of water in winter is unlikely in our region due to sub-zero temperatures. Hence, the d-excess values >10‰ cannot be ascribed to secondary evaporation. The slopes of winter throughfall near 8 show that some other process is responsible for high d-excess values of winter throughfall, with evaporation playing either no role or only a small role. It is important to note that in our study region most of the winter precipitation is snowfall coupled with the leafless period, leaving limited canopy areas for evaporation, except for red pine. Therefore, the slope of winter rainfall is lower than 8 (6.38‰), with a high d-excess value (19.5‰). Snow interception is often characterized by an increase in d-excess values and the factors responsible for such changes are sublimation and partial snowmelt (Gat Citation2010). The average difference of ~9.5‰ in d-excess value that we recorded between water collected in the summer and the winter precipitation (throughfall and rainfall) reflects differences in the humidity over the region during summer and winter (Lee et al. Citation2003).
If the δD–δ18O slope is different between rainfall and throughfall and among the four different throughfalls, we can suggest that a particular species has affected the precipitation differently compared to the other species. Here, rainfall δD–δ18O slope (6.65‰) is about 0.24–0.81‰ lower than that of throughfall. Different throughfall LMWLs result in δD–δ18O slopes in the range of 6.89–7.46‰ in the order of red pine > chestnut > mixed species > black locust. The difference in the δD–δ18O slopes among the four throughfalls is in the range of 0.10–0.57‰.
Seasonally also, summer and winter throughfall LMWLs differ from each other. The δD–δ18O slope difference among different throughfalls is higher in the summer (0.02–0.33‰) compared to the winter (0.03–0.14‰). This indicates that although differences are non-significant here, small mean overall as well as seasonal isotopic differences exist among different throughfalls. However, when rainfall and throughfall are compared seasonally, differences between the two are higher in the winter (0.71–0.85‰) than in the summer (0.14–0.19‰). The summer throughfall LMWL lies close and to the right of the GMWL. Relatively high humidity (>85% at the study site in summer) and minimal evaporation are the two factors that favour a δD–δ18O slope of near 8. The winter throughfall LMWL also lies close to the GMWL, but it is positioned towards the left of the GMWL. Re-evaporative water tends to cause δD and δ18O isotopic values to co-deviate away from slope 8 towards a lower slope (Clark and Fritz Citation1997). However, re-evaporation in the sub-zero winter temperatures is improbable; and winter d-excess values exceeding 17‰ reinforce this (). Lee et al. (Citation2003) suggested that trajectories of cold dry polar air masses passing over the Yellow Sea and arriving in continental Korea in winter are responsible for the δD–δ18O slope lower than 8 for the region. Lower δD–δ18O slopes can also be related to the low relative humidity (mean 60%) during the winter season observed for the study region.
Conclusions
We noticed alterations of H and O isotopes as canopy interception effects are transmitted in the throughfall. Individual events showed considerable variations of enrichment and depletion in the isotopic composition of both rainfall and throughfall. Overall δ18O and δD in throughfalls were about 0.35–0.59‰ and 0.85–1.95‰ different from rainfall, and these differences between the two forms of water are substantially larger than the 0.1‰ and 0.5‰ analytical uncertainty for both the isotopes in this study. Dissimilar throughfall d-excess and slope values show that structure of the vegetation may be one of the determinants of throughfall δ18O and δD isotopic composition. We hypothesize that higher δD–δ18O slope values of throughfalls compared to rainfall in winter precipitation may be related to the difference in the humidity inside forests (higher) and open locations. The differences in the δD–δ18O slopes among different throughfalls can be related to the differences in leaf packing (number of leaves per branch), crown architecture, and canopy packing in a stand (species specific). These conditions can greatly affect the processing of water traversing the tree stand as throughfall and stemflow, as well as humidity levels inside each forest (due to differences in the rate of evapotranspiration). To conclusively address the role of the microclimate in moderating d-excess values in a forested ecosystem, future research is needed to quantify the effect of stand(species)-specific microclimate vis-à-vis local climate on deuterium excess values.
Since even a 0.5‰ difference in δ18O can produce a >15% change in calculation of the contribution of new water (Xu et al. Citation2014) , it is critical not to discount the canopy isotopic effects in a forested ecosystem, otherwise this could lead to bias in the interpretation of results for ecohydrological studies. To this end, knowledge of the effects of interception and other canopy-related processes on the isotopic composition of incident rainfall and stemflow collected below the canopy is desirable.
Apart from throughfall, another most important process that influences rainfall distribution in the wooded ecosystem is stemflow. There are marked differences in the routing of intercepted precipitation to the forest floor via stemflow, due to notable differences in the vegetation surfaces, which result in notable differences in the flow path and residence time of intercepted water (Levia Citation2011). The stemflow amount varies greatly depending upon species, their branching pattern, and bark properties (i.e. smoothness and wettability). Various precipitation studies have suggested that, percentage-wise, stemflow is relatively smaller than throughfall, but its proportion can still be as high as 15% of the total rainfall (Crockford and Richardson Citation2000). In some cases, it even reaches 30% (Xu et al. Citation2005). One weakness of our study is that we did not include the isotopic composition of stemflow. Several studies have suggested that isotopic composition of stemflow is different from both the rainfall and the throughfall. It is therefore important that future research on H and O isotopic studies should include stemflow hydrology to better model the changes in catchments dominated by forests.
Compliance with ethical standards
Study does not infringe any bioethical principles and no damage to the biodiversity was inflicted while carrying out the study.
Conflict of interest
The authors declare that they have no conflict of interest.
Acknowledgements
This work was supported by the National Agenda Program (NAP) of the National Research Council of Science and Technology and partly by the Korea Basic Science Institute grant (C34700). We are thankful to four anonymous reviewers for correcting the manuscript. We also extend our thanks to handling editor Dr Maria Dolores Fidelinus, who helped in improving the manuscript with constructive comments.
Disclosure statement
No potential conflict of interest was reported by the authors.
Additional information
Funding
References
- Allen, S.T., et al., 2014. The role of pre-event canopy storage in throughfall and stemflow by using isotopic tracers. Ecohydrology, 7, 858–868. doi:10.1002/eco.1408
- Allen, S.T., et al., 2017. The role of stable isotopes in understanding rainfall interception processes: a review. Wiley Interdisciplinary Reviews: Water, 4 (1). doi:10.1002/wat2.1187
- Araguás-Araguás, L., Froehlich, K., and Rozanski, K., 1998. Stable isotope composition of precipitation over Southeast Asia. Journal of Geophysical Research, 103, 28721–28742. doi:10.1029/98JD02582
- Bayard, D., et al., 2005. The influence of seasonally frozen soil on the snowmelt runoff at two Alpine sites in southern Switzerland. Journal of Hydrology, 309, 66–84. doi:10.1016/j.jhydrol.2004.11.012
- Brodersen, C., et al., 2000. Influence of vegetation structure on isotope content of throughfall and soil water. Hydrological Processes, 14, 1439–1448. doi:10.1002/1099-1085(20000615)14:8<1439::AID-HYP985>3.0.CO;2-3
- Carlyle-Moses, D.E. and Gash, J.H., 2011. Rainfall interception loss by forest canopies. In: D.F. Levia, D.E. Carlyle-Moses, and T. Tanaka, eds. Forest hydrology and biogeochemistry. The Netherlands: Springer, 407–423.
- Clark, I. and Fritz, P., 1997. Environmental isotopes in hydrology. New York: Lewis Publishers.
- Craig, H., 1961. Isotopic variations in meteoric waters. Science, 133, 1702–1703. doi:10.1126/science.133.3465.1702
- Crockford, R.H. and Richardson, D.P., 2000. Partitioning of rainfall into throughfall, stemflow and interception: effect of forest type, ground cover and climate. Hydrological Processes, 16–17, 2903–2920. doi:10.1002/1099-1085(200011/12)14:16/17<2903::AID-HYP126>3.0.CO;2-6
- Cui, J., et al., 2009. Using deuterium excess to determine the sources of high-altitude precipitation: implications in hydrological relations between sub-alpine forests and alpine meadows. Journal of Hydrology, 373, 24–33. doi:10.1016/j.jhydrol.2009.04.005
- Daansgard, W., 1964. Stable isotopes in precipitation. Tellus, 16, 436–468. doi:10.1111/j.2153-3490.1964.tb00181.x
- Dawson, T.E. and Simonin, K.A., 2011. The role of stable isotopes in forest hydrology and biogeochemistry. In: D.F. Levia, D.E. Carlyle-Moses, and T. Tanaka, eds. Forest hydrology and biogeochemistry. The Netherlands: Springer, 137–162.
- DeWalle, D.R. and Swistock, B.R., 1994. Differences in oxygen-18 content of throughfall and rainfall in hardwood and coniferous forests. Hydrological Processes, 8, 75–82. doi:10.1002/hyp.3360080106
- Gat, J.R., 1996. Oxygen and hydrogen isotopes in the hydrologic cycle. Annual Review of Earth and Planetary Science, 24, 225–262. doi:10.1146/annurev.earth.24.1.225
- Gat, J.R., 2010. Isotope hydrology: a study of the water cycle. Series on environmental science and management. London: Imperial college press, 70–80.
- Gat, J.R. and Matsui, E., 1991. Atmospheric water balance in the Amazon Basin: an isotopic evapotranspiration model. Journal of Geophysical Research: Atmospheres, 96 (D7), 13179–13188. doi:10.1029/91JD00054
- Hsueh, Y.-H., et al., 2016. Fine-scale spatial variability of throughfall amount and isotopic composition under a hardwood forest canopy. Hydrological Processes, 30, 1796–1803. doi:10.1002/hyp.10772
- IAEA., 2012. WISER: Water isotope system for data analysis, visualization and electronic retrieval. Available from: http://www.univie.ac.at/cartography/project/wiser/ [ Accessed 4 July 2013].
- Ikawa, R., et al., 2011. Temporal variations of isotopic compositions in gross rainfall, throughfall, and stemflow under a Japanese cedar forest during a typhoon event. Hydrological Research Letters, 5, 32–36. doi:10.3178/hrl.5.32
- Kendall, C. and McDonnell, J.J., 1998. Isotope tracers in catchment hydrology. Amsterdam: Elsevier Science.
- Koeniger, P., et al., 2008. Isotopic variation of snow cover and streamflow in response to changes in canopy structure in a snow-dominated mountain catchment. Hydrological Processes, 22, 557–566. doi:10.1002/hyp.6967
- Kubota, T. and Tsuboyama, Y., 2003. Intra- and inter-storm oxygen-18 and deuterium variations of rain, throughfall, and stemflow, and two-component hydrograph separation in a small forested catchment in Japan. Journal of Forest Research, 8, 179–190. doi:10.1007/s10310-002-0024-9
- Lee, K.S., et al., 2003. Climatic controls on the stable isotopic composition of precipitation in North-east Asia. Climate Research, 23, 137–148. doi:10.3354/cr023137
- Lee, K.S. and Kim, Y., 2007. Determining the seasonality of groundwater recharge using water isotopes: a case study from the upper North Han River basin, Korea. Environmental Geology, 52, 853–859. doi:10.1007/s00254-006-0527-3
- Lee, K.S., Wenner, D.B., and Lee, I., 1999. Using H-and O-isotopic data for estimating the relative contributions of rainy and dry season precipitation to groundwater: example from Cheju Island, Korea. Journal of Hydrology, 222, 65–74. doi:10.1016/S0022-1694(99)00099-2
- Levia, D.F. 2011. Throughfall and stemflow in wooded ecosystems. In: D.F. Levia, et al. eds. Forest hydrology and biogeochemistry. The Netherlands: Springer, 425–443.
- Levia, D.F. and Germer, S., 2015. A review of stemflow generation dynamics and stemflow‐environment interactions in forests and shrublands. Reviews of Geophysics, 53, 673–714. doi:10.1002/2015RG000479
- Liu, W.J., et al., 2007. Using stable isotopes to determine sources of fog drip in a tropical seasonal rain forest of Xishuangbanna, SW China. Agricultural and Forest Meteorology, 143, 80–91. doi:10.1016/j.agrformet.2006.11.009
- Liu, W.J., et al., 2008. Isotope variations of throughfall, stemflow and soil water in a tropical rain forest and a rubber plantation in Xishuangbanna, SW China. Hydrology Research, 39, 437–449. doi:10.2166/nh.2008.110
- McGuire, K. and McDonnell, J., 2007. Stable isotope tracers in watershed hydrology. In: R. Michener and K. Lajtha, eds. Stable isotopes in ecology and environmental science. Boston: Blackwell Scientific, 334–365.
- Pypker, T.G., et al., 2005. The importance of canopy structure in controlling the interception loss of rainfall: examples from a young and an old-growth Douglas-fir forest. Agricultural and Forest Meteorology, 130, 113–129. doi:10.1016/j.agrformet.2005.03.003
- Saxena, R.K., 1986. Estimation of canopy reservoir capacity and oxygen-18 fractionation in throughfall in a pine forest. Nordic Hydrology, 17, 251–260.
- Sharp, Z., 2007. Principles of stable isotope geochemistry. New Jersey: Pearson education.
- Sprenger, M., et al., 2016. Illuminating hydrological processes at the soil vegetation atmosphere interface with water stable isotopes. Reviews of Geophysics, 54, 674–704. doi:10.1002/2015RG000515
- von Arx, G., Dobbertin, M., and Rebetez, M., 2012. Spatio-temporal effects of forest canopy on understory microclimate in a long-term experiment in Switzerland. Agricultural and Forest Meteorology, 166–167, 144–155. doi:10.1016/j.agrformet.2012.07.018
- Xu, X., Guan, H., and Deng, Z., 2014. Isotopic composition of throughfall in pine plantation and native eucalyptus forest in South Australia. Journal of Hydrology, 514, 150–157. doi:10.1016/j.jhydrol.2014.03.068
- Xu, X.N., Wang, Q., and Hirata, E., 2005. Precipitation partitioning and related nutrient fluxes in a subtropical forest in Okinawa, Japan. Annals of Forest Science, 62, 245–252. doi:10.1051/forest:2005016
- Zhang, W., et al., 2010. Using stable isotopes to determine the water sources in alpine ecosystems on the east Qinghai-Tibet plateau, China. Hydrological Processes, 24, 3270–3280. doi:10.1002/hyp.7754