ABSTRACT
Stable isotopes of water have been widely used in understanding the hydrological functions of alpine inland catchments. This study identifies dominant runoff generation mechanisms based on isotopic data (δ18O and δ2H) of 487 rainwater and river-water samples from three tributaries in the Tarim River Basin in China for the period May–September 2013. The isotope hydrograph separation results provide a comprehensive overview of the rainfall influence on hydrological processes. Stream water and groundwater have varied responses to different intensities of rainfall events. Only a small proportion of rainfall is directly transported to the stream during such events. An inconsistent temporal trend of event water contribution is observed in the three catchments. The average fractional contributions of rainfall for the Tizinafu, Kumalak and Huangshuigou rivers are 10.3% (±1.1%), 9.7% (±2.9%) and 8.7% (±2.4%), respectively.
Editor R. Woods; Associate editor F. Tauro
1 Introduction
Water resources strongly limit socio-economic development in arid Central Asia. Inland rivers in that region play a key role in human development through the provision of water for agriculture, industry and domestic use (Sun et al. Citation2016c). Understanding the hydrological functions of inland mountain river headwater catchments is therefore critical for scientific and management reasons including flood forecasting, water supply, irrigation water, and stream habitat protection (Hrachowitz et al. Citation2011, Zohrabi et al. Citation2011, Roshan and Grab Citation2012, Zarei et al. Citation2014, Penna et al. Citation2016). Studies (Chen et al. Citation2006, Citation2016, Immerzeel et al. Citation2010) have shown that nearly all mountainous regions of Central Asia have experienced a warming trend. This may result in feedback effects of reduced glacier/snow surface albedo that seriously alter the local water cycle. In inland river basins of Central Asia, runoff generation is controlled by the proportions of glacial meltwater, precipitation and groundwater. As a main water resource for arid mountainous Central Asia, precipitation has an important influence on the hydrological processes of inland river basins, and heavy rainfall events affect streamflow and may produce large peak flows, especially in summer (Mirus and Loague Citation2013, Sun et al. Citation2016a, Citation2016b). However, researchers have paid more attention to evaluating the proportion of glacial meltwater in Central Asia (Kong and Pang Citation2012, Li et al. Citation2015a, Sun et al. Citation2016a, Citation2016b, Citation2016c). As a result, streamflow variability as a response of inland rivers to summer rainfall is still unclear, especially in mountain areas.
Isotopic composition (δ18O and δ2H) of river water is a useful tracer of hydrological processes in catchments. Isotope tracer studies are generally used for hydrograph separation (Buttle Citation1994, Kong and Pang Citation2012). They provide additional information for identifying source areas and flow pathways under various flow conditions of a catchment (Shanley et al. Citation2002, McGuire and McDonnell Citation2006, Tekleab et al. Citation2013, Zarei and Akhondali et al. Citation2014, Sun et al. Citation2015). Recent studies in high-elevation catchments have shown that isotope hydrograph separation (IHS) based on isotopic and geochemical tracers is effective for exploring complex hydrological processes (Penna et al. Citation2016) and assessing streamflow sources at the annual (Li et al. Citation2014, Citation2015a, Citation2015b, Sun et al. Citation2015, Zhou et al. Citation2015) and seasonal (Kong and Pang Citation2012, Penna et al. Citation2016) scales. It is also useful in estimating the contributions of glacier/snowmelt, groundwater and precipitation to streamflow, and in determining the importance of various groundwater recharge sources (Joel et al., Citation2009, Pang et al. Citation2011, Tao et al. Citation2012, Wang Citation2014, Sun et al. Citation2016a). Applications of IHS techniques to better understand hydrological processes in alpine arid areas and their catchments with permafrost or glacier cover, however, are scarce and limited, mainly because of challenging monitoring conditions and sparse networks of research sites available in these catchments (Thayyen et al. Citation2007, Cable et al. Citation2011, Kong and Pang Citation2012, Carturan et al. Citation2016, Penna et al. Citation2016, Sun et al. Citation2016a, Citation2016b, Citation2016c).
In mountainous catchments of Central Asia, persistent and heavy precipitation increases glacier/snowmelt and groundwater recharge (Li et al. Citation2014). Despite the wide use of isotope tracers in hydrological studies, most studies have focused on single sampling events. Relatively few studies have concentrated on the examination of runoff generation processes and their responses to rainfall events, especially in arid areas of Central Asia (Kong and Pang Citation2012, Penna et al. Citation2016, Sun et al. Citation2016b, Citation2016c).
To gain more detailed knowledge of runoff generation for catchments, it is necessary to know the extent to which river-water isotopic composition is altered by rainfall, and how this alteration varies among inland river basins within Central Asia. In this paper, we use a large isotopic dataset supplemented by hydrological measurements to compare rain–streamflow processes for three rivers in China’s Tarim River Basin (TRB) during the rainy season. We investigate rainfall and pre-event water partitioning in order to gain insight into runoff generation processes in a typical Central Asia inland river basin.
2 Study area and methodology
2.1 Study area
The TRB is the largest inland river basin (more than 53 × 104 km2) in China. It is located north of the Qinghai-Tibetan Plateau, together with the surrounding mountains including the Tianshan and Kunlun mountains and the Altyn mountain ranges, and orographic effects create a barrier to warm and moist air penetrating into the basin (). As a result, the basin has an extremely dry desert climate with limited precipitation and high potential evaporation. Although the annual precipitation in the mountainous headwater regions can reach about 250 mm, it is only 40–70 mm in the remaining regions of the TRB. In the Taklamakan Desert and the Lop Nur basin, the average annual precipitation is only about 12 mm. In contrast, the annual potential evaporation of the entire TRB is about 1000–1600 mm, which makes the aridity index (ratio of annual potential evaporation to precipitation) of the basin one of the highest in the world.
Three inland rivers were selected to study the water isotope dynamics (see ): the Kumalak River in the west of the Tianshan Mountains, the Huangshuigou River in the east of the Tianshan Mountains, and the Tizinafu River in the Kunlun Mountains. The Kumalak River originates from Glacier No. 72, which flanks Tuomuer Peak, the highest peak in the Tianshan Mountains, with an elevation of 7435 m a.s.l. The total length of the river until Xiehela hydrological station (outlet station) is 208 km, with a drainage area of 12.86 × 103 km2 above Xiehela station. The Huangshuigou River originates from the south slope of Tianger Peak in the Tianshan Mountains and discharges into Bosten Lake. The total length of the Huangshuigou River is 162 km, with a drainage area of 0.4311 × 103 km2 above Huangshuigou hydrometric station, and the reach up to the outlet at Huangshuigou hydrological station is 110 km. The average annual runoff of the Huangshuigou River is 0.256 × 109 m3. The Tizinafu River Basin (36°31′N–38°54′N, 76°27′E–79°04′E), located in the Kunlun Mountains of western China, has a total area of 10.3 × 103 km2 above Jiangka station (). The main stream originates from the Kakrak Pass on the northern slopes of the Karakoram Mountains. This river has a total length of 335 km and the stream above Jiangka gauge station has a length of 190 km. Three meteorological stations were selected for collecting rainfall samples in the study area: Xiehela (Kumulak River), Baluntai (Huangshuigou River), and Xihexiu (Tizinafu River) ().
Table 1. Hydro-meteorological characteristics of investigated catchments. P, Q and E: catchment average precipitation, runoff, potential evaporation and actual evaporation, respectively.
2.2 Water sampling and laboratory analysis
To quantify the contributions of different potential source waters (i.e. end members) to streamflow, water samples were collected at different locations and times during the study period.
Rainwater was taken from a collector near the raingauge. Rainfall events were sampled (by event) from May to September 2013, as follows: 18 rainfall events at Xiehela station; 26 rainfall events at Baluntai station; and 16 rainfall events at Xihexiu station.
River-water samples were collected from the main stream at the outlet of the Kumalak (Xiehela station), Shuangshuigou (Huangshuigou station) and Tizinafu (Jiangka station) catchments. In order to describe the change of the water isotope clearly, we collected a river-water sample almost every day from 10 May to 30 September 2013: 144 samples were collected from Xiehela station, 143 water samples from Huangshuigou station and 141 samples from Jiangka station ().
Table 2. Isotope sample locations and total number of samples taken during the investigation period (May–September 2013).
To evaluate the response of river water and groundwater to different rainfall events, three observed rainfall events were selected, one in each river basin: an intense rainstorm on 29 July 2013 in the Kumalak River Basin; successive rainfall events during 15–20 June 2013 in the Huangshuigou River Basin; and light rainfall events during 3–4 August 2013 in the Tizinafu River Basin. Groundwater samples were collected using six piezometers (two per catchment) installed in the upper parts of the catchments. The holes for installation of the piezometers ranged from 1 to 1.6 m depth. The samples were collected as follows: six samples from Xihexiu station (1–5 August 2013), six samples from Baluntai station (15–20 June 2013) and five samples from Xihexiu station (27–31 July 2013). The δ18O and total dissolved solids (TDS) of pre-event water, river water, rainwater and groundwater were measured during the selected sample periods. Before sampling, we washed a 5-mL glass sample vial three times using sampled water, then filled it with the water sample. Each vial was sealed immediately with Parafilm to prevent evaporation. All sample bottles were immediately sealed and stored in a cold laboratory below −18°C. When the samples were analysed, they were stored at 4°C in a refrigerator to melt gradually and avoid evaporation. All water samples were analysed at the State Key Laboratory of Desert and Oasis Ecology, Xinjiang Institute of Ecology and Geography, Chinese Academy of Sciences, using a LGR (Model DLT-100; Los Gatos Research Inc., USA) liquid-water isotope analyser. The stable isotopic composition of oxygen-18 and deuterium are reported using the δ-notation, according to the Vienna Standard Mean Ocean Water (VSMOW), as δ18O and δ2H (‰), where δ = ((Rsample/Rstandard) – 1) × 1000, with R the ratio of the heavy and light isotopes (e.g. 18O/16O and 2H/1H). The accuracy of the LGR liquid-water isotope analyser measurements was 0.1‰ for δ18O and 0.4‰ for δ2H. River-water samples were collected in 500-mL bottles to determine the TDS. Related chemical analysis was carried out in the same laboratory. The water samples were filtered through a 0.45-μm millipore membrane using high performance liquid chromatography (HPLC) with a dual-column instrument (Model Dionex CD20), which had an analytical error of less than 1 mg/L.
2.3 Hydrograph separation techniques
The mass balance equation used for a time-based, two-component separation using δ18O as a tracer can be described by (Wels et al. Citation1991, Kong and Pang Citation2012, Sun et al. Citation2016a):
where Q is the discharge, C is the concentration of the tracer (the isotopic composition in this paper) and subscripts R, pre and e refer to stream water, pre-event water and event water, respectively. There are some assumptions for the isotope-based two-component hydrograph separation:
there is a difference between the isotopic composition of the event and pre-event water;
the isotope signature of event and pre-event water should be constant during the monitored period;
the contribution of water from the vadose zone is negligible, or the isotopic content of soil water is similar to that of groundwater; and
the contribution to streamflow from surface storage is negligible (Buttle Citation1994, Penna et al. Citation2016).
The δ18O was used as the tracer C to calculate the event water fraction based on the two-component hydrograph separations.
In this paper, the event water composition (Ce) used the incremental weighted mean of the isotopic composition of one-day rainfall. The isotopic composition of pre-event water (Cpre) was determined by the isotopic composition of stream water at the outlet station sampled a day prior to the onset of each rainfall event.
3 Analyses and results
3.1 Comparison of meteorology and hydrology
The hydrological responses to climate change in the TRB were investigated by analysing hydrological, temperature and rainfall data of the past 50 years. Climate change has resulted in streamflow changes in the TRB headwaters (Chen et al. Citation2006). From 1957 to 2010, the climate in the TRB became warmer and wetter. The average annual temperature in the Kumalak River Basin has had a significant increasing trend of 0.31°C/10 years, and an annual rainfall increase of 10.95 mm/10 years. Over the past 50 years, the average rate of runoff increase in the Kumalak Basin was 2.93 × 108 m3per decade. Seasonally, the rate of summer runoff increase in the basin was the greatest (2.19 × 108 m3per decade). During the past five decades, temperatures in the Huangshuigou River Basin have been increasing with fluctuations, with an average increase of 0.27°C per decade; annual rainfall has also increased 8.63 mm/10 years. As a consequence, the average runoff began to increase rapidly from 1986. In the Tizinafu River Basin, temperatures have been increasing with fluctuations, with an average increase of 0.24°C per decade over the past five decades. The average increase of rainfall in the basin was 4.62 mm/10 years. Over the past 40 years, annual runoff in the basin has shown a significant increasing trend. Seasonally, the summer runoff increase (0.034 × 109 m3/10 years) was the greatest.
Total precipitation at Xiehela, Baluntai and Xihexiu stations between 10 May 2013 and 1 October 2013 was 144.6, 115 and 39.4 mm, respectively. Average streamflow for the same period was 297.63, 9.69 and 20.96 m3/s for Xiehela, Huangshuigou and Jiangka stations, respectively. Maximum peak flow at Xiehela station occurred during an 8.5-mm rain event on 17 July. Peak flows at Huangshuigou and Jiangka stations occurred on 26 July and 11 July, respectively, without substantial precipitation.
3.2 Variability of isotopic composition in rainfall and river water
3.2.1 Isotopic composition of rainfall
Measured isotopic compositions of rainfall samples exhibited marked spatial and monthly variations (Fig. 2). Rainfall at Xihexiu (Tizinafu River) had the heaviest average δ18O values compared to the lighter isotopic composition at Xiehela (Kumalak River). During the monitoring period, δ18O of rainfall sampled at the Kumalak River (Xiehela station) varied between −11.01 and 3.12‰, with an average of −3.21‰. Temporal variations in rainwater δ18O were observed at this station. Maximum positive values appeared in the period from mid-July to early August. As expected, the isotopic composition of rainwater was highly variable () during continuous rainfall events on the Kumalak and Huangshuigou rivers. For example, for an event on 17–18 July 2013 at Xiehela station, δ18O decreased from −0.12‰ to −6.77‰. At Huangshuigou station, δ18O decreased from 0.24‰ to −11.51‰ during long continuous precipitation events over the period 14–20 June.
Figure 2. Rainfall hyetograph, mean daily air temperate, streamflow recorded and δ18O values of streamflow and precipitation samples for (a) Kumalak River, (b) Huangshuigou River and (c) Tizinafu River.
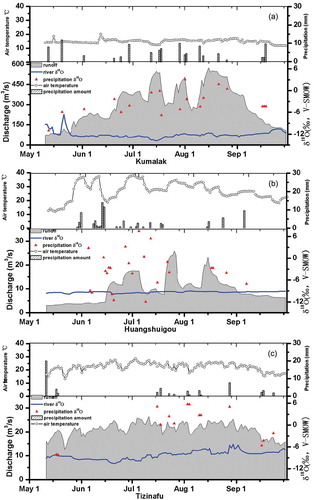
The δ18O and δ2H of rainfall samples from the three stations showed different patterns of variation. shows that the isotopic composition of rainfall in the Huangshuigou River Basin (Baluntai station) had a pronounced seasonal variation: δ18O varied between −11.96 and 7.45‰ (average:−3.49‰). The maximum positive value appeared in mid-June at Baluntai station. The large scatter of rainfall δ18O at that station corresponds to relatively strong air temperature fluctuations, indicating that rainfall isotopic composition in Huangshuigou Basin is affected by temperature. Rainfall δ18O for the Tizinafu River was between −8.14 and 5.74‰ (average: −0.33‰). The maximum positive value at Xiehexiu station was observed from mid-June to mid-July. Comparing the three stations, the maximum rainfall δ18O at Xiehexiu (5.74‰) was greater than that at Xiehela (3.12‰), but less than that at Baluntai (7.45‰). These larger maximum δ18O values indicate that the TRB has a unique climate pattern (high summer temperature and very strong evaporation).
3.2.2 Local meteoric water lines
The relationship between the δ18O and δ2H isotopic composition of rainfall and river water in the study area is shown in . The spatial distributions of δ18O and δ2H of rainfall composition on a south slope (Xihexiu station) and a north slope (Xiehela and Baluntai stations) varied considerably. Rainfall on the Huangshuigou River had a larger isotope value range. Some deviations from the global meteoric water line (GMWL: δ2H = 8δ18O + 10) might be related to the effect of evaporation of falling raindrops, condensation in clouds, and different moisture sources in different months (Dansgaard Citation1964, Tekleab et al. Citation2013). The local meteoric water line (LMWL) was determined from the three stations for the observation period.
LMWL of Kumalak: δ2H = 7.85δ18O + 9.51 (R2 = 0.96)
LMWL of Huangshuigou: δ2H = 6.12δ18O − 3.64 (R2 = 0.94)
LMWL of Tizinafu: δ2H = 6.82δ18O + 5.2 (R2 = 0.97)
All LMWLs in the TRB had a smaller slope and intercept than the GMWL. The differences among LMWLs reflect different sources of water vapour according to location and climate. Studies (Zhonghe and Yanlong et al. Citation2011, Li et al. 2016, Sun et al. Citation2016a) have found that water vapour in the TRB originates mainly from the westerly moisture vapour. The LMWL slopes showed a decreasing trend from west to east. The LMWL slope for the Kumalak Basin was similar to that of the GMWL. This suggests that moisture sources for the Kumalak Basin are mainly linked to atmospheric circulation patterns. Owing to strong evaporation, the LMWL of the Huangshuigou Basin in the TRB hinterland has a small slope. In the TRB, slopes and intercepts depend on elevation and distance from the vapour source. The LMWL of the western catchment had steeper and larger intercepts than that of the eastern margin and lower elevation areas.
3.2.3 Isotopic composition of stream water
shows the variations of stream-water δ18O for three rivers during the monitoring period. In the Kumalak catchment, isotope values of stream water were collected at the catchment outlet station Xiehela ()), and overall composition of Kumalak stream water ranged between −13.82 and −5.58‰ (average: −11.86‰). The maximum δ18O was observed in May after a 16-mm rainfall event, and the minimum was in mid-July, with stronger flow during a 7-mm rainfall event. In the study period, stream-water δ18O showed weak variations in the Huangshuigou River. The δ18O composition of river water varied between −9.86 and −9.01‰ (average: −9.38‰). Stream-water δ18O of the Tizinafu river varied between −10.58 and −6.44‰ (average: −8.26‰). The maximum δ18O was observed in mid-August and the minimum in mid-June.
There was substantial monthly variation in the Kumalak and Tizinafu rivers, suggesting that the main contributors to stream runoff were likely different. In the rainfall season, δ18O showed greater variations and fluctuations were observed in the Tizinafu River. This demonstrates that the streamflow components in that river vary greatly over time. Furthermore, there were no obvious seasonal variations of δ18O in river runoff with respect to rainfall at Baluntai station ()), suggesting that summer streamflow components of Huangshuigou River are relatively stable. A recent study (Sun et al. Citation2016b) found that the summer streamflow component of inland rivers on the south slope of Tianshan Mountain had a stable pattern and that streamflow in Huangshuigou was mainly sustained by groundwater (the isotopic value is stable), which infiltrates and discharges from glacier/snow meltwater and frozen soil water.
3.3 Hydrograph separation in three study areas
Numerous isotopic studies have focused on evaluating hydrological responses in humid regions around the world (Katz et al. Citation1997, Jones et al. Citation2000, Aquilina et al. Citation2006, Li et al. 2016), improving understanding of such responses during certain episodes such as the snowmelting period and the rainfall period. However, few hydrograph separation studies have been conducted in arid regions of Central Asia. In this study, we focus on an arid inland river basin during the rainy season (May–September), to improve the basic understanding of the hydrological responses to rain episodes and calculate partitioning between event and pre-event water. A number of hydrological events during the wet season were monitored and sampled for stable isotopic composition of δ18O and δ2H.
Using previous-day isotope values to represent pre-event water, we calculated fractions of rainfall and pre-event water. Temporal variations in stream-water isotopes sampled at the catchment outlet showed slight differences in event water contributions over all the catchments (). There were inconsistent temporal trends of event water contributions in the three catchments.
The event water contributions to streamflow remained stable during the rainy season, except for two intense rainfall events that had larger contributions to runoff in the Kumalak River. Most runoff from rainfall was concentrated in late July and early August. During this period, the rainfall was more intense and streamflow showed greater fluctuations. The results of IHS showed that the major resource in the period was the pre-event water, indicating that the streamflow change of the Kumalak River is not controlled by the contribution of rainfall events. In that river, the contribution of rainfall to total streamflow was between 2 and 89.5%, with the greatest contribution observed on 16 May 2012, when 11 mm of rainfall was recorded in two hours. In the Huangshuigou River, the oxygen isotope value of its water during the rainy season remained stable. Based on the IHS, rainfall had a weak effect on streamflow, and rainfall contributions to streamflow varied between 3.7 and 93.3%. The greatest contribution was on 20 June 2013, when 15.4 mm of rainfall was recorded in one hour. The IHS results showed that event water fractions were 1.5–70% and had the greatest contribution to streamflow on 17 May 2012, when 6.5 mm of rainfall was recorded in one hour.
Weighted by streamflow (observed on the sampling date), we calculated the contributions of various water sources to river runoff. shows the proportions of various event waters for the Tizinafu, Kumalak and Huangshuigou rivers in various months. A significant monthly difference was observed. In the Huangshuigou River, the rainwater contribution to streamflow was very small, especially during June 2013. In the Kumalak and Tizinafu rivers, the largest proportion of rainfall to runoff was observed in May 2013, and the contribution of rainwater in other months remained stable. The average fractional event water contribution in July and August was usually smaller than that of May and September. The average fractional contributions of event water in the Tizinafu, Kumalak and Huangshuigou rivers were 10.3% (±1.1%), 9.7% (±2.9%), and 8.7% (±2.4%), respectively.
4 Discussion
4.1 Influence of rainfall on runoff in inland river basin
For typical inland rivers in the high mountains of Central Asia, runoff from glacial meltwater and rainfall mainly occurs in summer. The rainfall−runoff transformation is a complicated and nonlinear process. However, the most important cause of this nonlinearity is likely antecedent conditions (Tekleab et al. Citation2013, Sun et al. Citation2016a, Citation2016b). Alpine catchments are important sources of freshwater, but our understanding of the hydrological functions of the catchments during rainfall events is still limited compared with lower altitude catchment. There are some evaluations of the precipitation contribution in inland river basins originating from the Tianshan Mountains. According to recent studies (Sun et al. Citation2016c), precipitation contributes 10–20% to river runoff in the source region of the Tianshan Mountains. Kong and Pang (Citation2012) found that glacier/snow meltwater and groundwater had a larger contribution than precipitation to flow in the Kumalak River. Through 2012, the proportion of precipitation in streamflow was only 17.6% in the Urumqi River (Sun et al. Citation2016b). In the Aksu River, 10% of runoff was derived from precipitation (Sun et al. Citation2015). Fan et al. (Citation2015) demonstrated that the contribution of precipitation to runoff was small in the inland river basin of the Kunlun Mountains. Many researchers have addressed dominant runoff generation mechanisms during rainfall–runoff events in alpine mountain catchments of Europe. Dahlke et al. (Citation2014) evaluated the summer rain contributions to streamflow in a glacierized catchment of northern Sweden and found that the average event water contribution to runoff was about 22%. Penna et al. (Citation2016) used isotope hydrograph separation to identify dominant runoff generation mechanisms in the 0.14-km2 Bridge Creek catchment in the Italian Dolomites over nine rainfall–runoff events. Their studies indicated average event water contributions were between 7 and 25% of total runoff during those events.
In this study, the IHS results also showed that only a little rainwater directly contributed to streamflow during individual events in the three catchments. New rainwater can recharge the aquifer, possibly through fractures, and mobilizes older water present in smaller fractures and pores within soil and bedrock. The TRB has a large area and is surrounded by high mountains. The source areas of alpine inland river basins always have strong relief and very steep slopes, and are covered by an abundance of loose debris (Chen et al. Citation2016). Surface runoff following rainfall is relatively short, but intense rainfall often mobilizes loose debris along inland river channels (Gregoretti et al. Citation2016). Studies (Penna et al. Citation2016, Sun et al. Citation2016b) have shown there were temporal variations of TDS in streamflow and groundwater after large events. In our study, TDS of stream water tended to slightly decrease and then increase above pre-event values during large rainfall events such as 29 July 2013 in the Kumalak River. A decrease in TDS of stream water at the onset of a major event is frequently attributed to streamflow dilution by overland flow and direct channel precipitation (with low TDS). However, groundwater TDS showed a significant increase and was maximized the next day, and then decreased to pre-event values (). The variation of groundwater TDS was likely caused by the leaching of soil salt during rain infiltration.
A relatively smaller rainfall influence on streamflow is also supported by mixing plots in the three study areas (). Hooper et al. (Citation1990) and Hooper (Citation2003) introduced end-member mixing analysis (EMMA), which is now often used for analysing possible source area contributions to streamflow. In this paper, the mixing model was used to identify variable source areas and mixing processes, and to quantify the contribution of each end member. There are obviously differences in the concentrations of TDS and δ18O for pre-event water, groundwater and rainwater in the three study basins. In this study, the pre-event component represents the fraction of stream discharge that was stored within the watershed, comprising a mixture of groundwater, snow and glacier melt, and/or rainwater that entered the stream prior to an event. The groundwater is defined as the shallow groundwater in the three stations (Xiehela, Xihexiu, Baluntai) after rainfall events. Accordingly, the concentrations of TDS and δ18O were selected for the analysis because this combination provides the best separation of sources.
As the mixing plots shown, stream-water samples collected during the rainfall event are similar to those of the pre-event, indicating that water in the latter event was the major source of recharge in the study areas. The TDS and isotopic composition of groundwater in Kumalak and Tizinafu rivers were different from those of pre-event stream water. However, the isotopic composition of groundwater in the Huangshuigou River is similar to that of pre-event water. Groundwater from three stations was characterized by higher TDS and low isotope concentrations. We do not know the reason for the differences in TDS concentrations of the groundwater for the piezometers installed. However, because groundwater was the only source with a high TDS and the increase in stream-water TDS occurred at the same time as the increase of groundwater levels, it is likely that the increase in TDS of streamflow during the events was caused by groundwater influx.
) shows that the stream water is located within the triangle spanning the three end-members, suggesting that the runoff is a mix of them. The EMMA analysis showed there were also large spatial and temporal variations in the concentrations of these components for the Tizinafu and Huangshuigou rivers. The stream-water location of these two rivers suggested that the runoff was also a mix of the three end-members. However, the stream-water location of the Kumalak River is closer to the groundwater points than that of the Tizinafu River, which suggests that the groundwater of the Kumalak River supplied a greater contribution for the streamflow than that of the Tizinafu River. Although the results indicate that the majority of streamflow was dominated by pre-event water, rainfall water and groundwater are also important sources for inland rivers of this study area. However, due to the lack of long-term monitoring data of shallow groundwater, we cannot further discuss the contribution of groundwater to the streamflow. Further evaluations of streamflow components are needed in the future.
Figure 4. The temporal variations of rainfall contribution in (a) Kumalak river, (b) Huangshuigou river and (c) Tizinafu river.
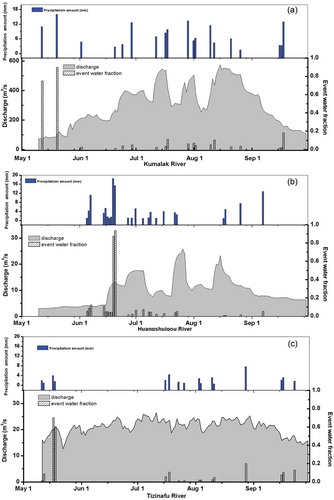
Figure 5. Estimated average contributions of water sources as a result of hydrograph separation in different months at three basins.
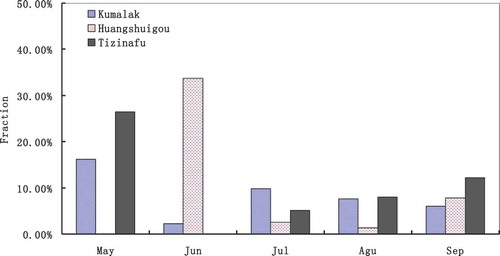
Figure 6. Time series of river δ18O and TDS (total dissolved solids), groundwater δ18O and TDS during rainfall events of (a) Kumalak River (27–31 July 2013), (b) Huangshuigou River (15–20 June) and (c) Tizinafu River (1–6 August 2013).
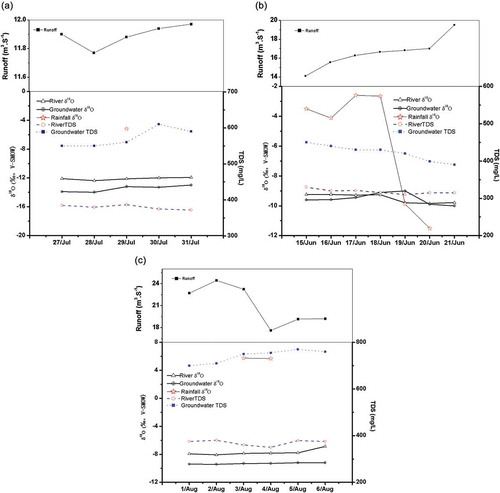
Figure 7. Mixing plots (δ18O vs TDS) for representative rainfall–runoff events of (a) Kumalak River (27–31 July 2013), (b) Huangshuigou River (15–20 June) and (c) Tizinafu River (1–6 August 2013). The dashed lines define the mixing space based on the rainfall samples, the pre-event water sample, and the dissolved solids (TDS) and isotopic composition of groundwater at three selected stations.
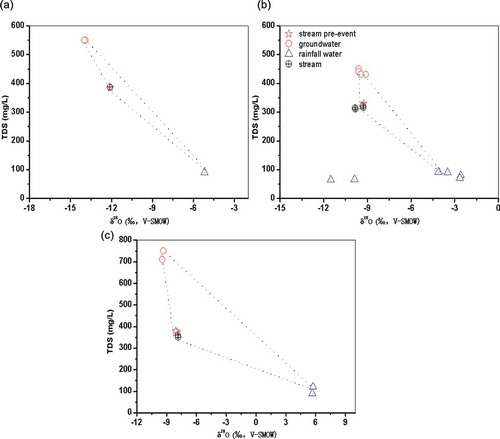
Precipitation in arid regions of western China is a complex process (Sun et al. Citation2016a, Citation2016b, Citation2016c). Zhao et al. (Citation2011) investigated the origin of moisture entering the headwaters of the Heihe River Basin, and found that atmospheric westerlies and polar water vapour were the main moisture sources in the arid region of northwestern China. Zhonghe and Yanlong et al. (Citation2011) concluded that seasonal changes in the moisture source and recycling in the Tianshan Mountains are responsible for the observed spatial and temporal variability of precipitation δ18O and δ2H in regional-scale hydrological conditions. These processes include equilibrium isotope fractionation, adiabatic ascent forming clouds during winter in mountainous terrain, sub-cloud evaporation, and the recycling of moisture from the ground (soil and surface water). In arid and semiarid regions, the recycling of terrestrial moisture via evapotranspiration has been recognized as an important component of atmospheric moisture balance. Strong evaporation in summer results in enriched isotopes in precipitation. Some studies have found (Zhao et al. Citation2011; Sun et al. Citation2016b) that summer precipitation events, which have more positive isotope values, are the main reason for significant variations in stream-water isotopes. In this study, the small variation of stream-water isotopes indicates that little rainfall contributes to stream water.
Observation of high mountain precipitation is very difficult, especially in the western Tianshan Mountains of Central Asia. In our study, there were few precipitation stations at high altitude and precipitation isotope data were limited, which may produce the small proportions of rainfall from IHS. The spatial variability of rainfall and rainwater isotopic composition is difficult to measure. The construction of a mountain precipitation observation network is a long and arduous task. Our future work will attempt to improve this situation.
4.2 Responses of streamflow water and groundwater to rainfall events
Some researchers have found that the chemical characteristics of stream water and groundwater vary substantially during rainfall events. To illustrate this variation, we selected three intense rainfall events in the three study catchments, and compared TDS and δ18O of stream water and groundwater before and after the events.
An initial decrease in stable isotopes of river water was observed after strong rainfall events (Penna et al. Citation2016, Li et al. 2016). The larger contribution of a strong rainfall event (with relatively negative δ18O and δD) should produce relatively negative stable isotope values of river water in the source region. However, in our study, stream-water isotopes did not change significantly (Kumalak River δ18O: −12.37 to −11.93‰, Huangshuigou River δ18O: −9.84 to −9.24‰, Tizinafu River δ18O: −8.08 to −6.87‰) under three different intensities of rainfall (). The limited variations of stream-water δ18O may be caused by a larger contribution from groundwater, which would dilute the isotopic composition of the rain and reduce its influence. Penna et al. (Citation2011) stated that rainwater contributions to streamflow were likely negligible and that rainwater was stored in the vadose zone during small events with dry antecedent moisture conditions.
The TDS of stream water tended to slightly decrease and then increase above pre-event values during the rain events. However, groundwater TDS showed different variations compared with the stream water. A significant increasing trend of groundwater TDS was observed during heavier rainfall, followed by a decrease to pre-event values ()). During persistent rainfall events, groundwater TDS showed a significant decreasing trend, observed during 15–21 July 2013 in the Huangshuigou River ()). However, a weaker rainfall event had no significant effect on groundwater TDS. After two light rainfalls during 3–4 August over the Tizinafu River, groundwater TDS had only a slight variation ()). The TDS variations in stream water and groundwater further proved that rainfall first recharged groundwater and then affected the stream over a larger area of the alpine inland river basin (Penna et al. Citation2016). Thus, detailed investigations of variability in TDS and isotopic composition of stream water and groundwater during different rainfall events are needed to distinguish the contributions to streamflow in various catchments.
5 Conclusions
The hydrometric, stable isotopic and TDS data collected during rainfall−runoff events in the three tributaries of the TRB helped improve our understanding of runoff generation processes in alpine headwater environments of Central Asia. The rainfall and streams showed noticeable spatial and temporal variations in stable δ18O and δD compositions across the study area. Temporal variations of TDS in streamflow and groundwater were observed after major events.
We used the IHS technique to determine the runoff components of three inland rivers. The results illustrate that the event water contribution was generally small, demonstrating that only a small proportion of rainfall is transported into stream water during events in the wet season (May–September). New rainwater can recharge the aquifer, possibly through fractures, and mobilize older water present in smaller fractures and pores within soil and bedrock. Responses of stream water and groundwater varied with rainfall event. The results showed the average fractional contribution of event water in the Tizinafu, Kumalak and Huangshuigou rivers was 10.3% (±1.1%), 9.7% (±2.9%), and 8.7% (±2.4%), respectively. The south slope (Tizinafu River) of the TRB had a larger rainfall ratio during the rainy season. The north slope (Huangshuigou River) of the TRB had a smaller rainfall ratio in the study period.
Disclosure statement
No potential conflict of interest was reported by the authors.
Additional information
Funding
References
- Aquilina, L., Ladouche, B., and Dörfliger, N., 2006. Water storage and transfer in the epikarst of karstic systems during high flow periods. Journal of Hydrology, 327, 472–485. doi:10.1016/j.jhydrol.2005.11.054
- Buttle, J.M., 1994. Isotope hydrograph separations and rapid delivery of pre-event water from drainage basins. Progress in Physical Geography, 18, 16–41. doi:10.1177/030913339401800102
- Cable, J., Ogle, K., and Williams, D., 2011. Contribution of glacier meltwater to streamflow in the Wind River Range, Wyoming, inferred via a Bayesian mixing model applied to isotopic measurements. Hydrological Processes, 25, 2228–2236. doi:10.1002/hyp.v25.14
- Carturan, L., et al., 2016. Catchment-scale permafrost mapping using spring water characteristics. Permafrost and Periglacial Processes, 27, 253–270. doi:10.1002/ppp.1875
- Chen, Y., et al., 2016. Changes in Central Asia’s water tower: past, present and future [J]. Scientific Reports, 6. doi:10.1038/srep39364
- Chen, Y.N., et al., 2006. Regional climate change and its effects on river runoff in the Tarim Basin, China. Hydrological Processes, 20 (10), 2207–2216. doi:10.1002/hyp.6200
- Dahlke, H.E., et al., 2014. Isotopic investigation of runoff generation in a glacierized catchment in northern Sweden. Hydrological Processes, 28 (3), 1383–1398. doi:10.1002/hyp.9668
- Dansgaard, W., 1964. Stable isotopes in precipitation. Tellus, 14, 436–468.
- Fan, Y.T., et al., 2015. Characteristics of water isotopes and ice-snowmelt quantification in the Tizinafu River, north Kunlun Mountains, Central Asia. Quaternary International, 380-381, 116–122. doi:10.1016/j.quaint.2014.05.020
- Gregoretti, C., et al., 2016. Runoff of small rocky headwater catchments: field observations and hydrological modeling. Water Resources Research, 52 (10), 8138–8158. doi:10.1002/2016WR018675
- Hooper, R.P., 2003. Diagnostic tools for mixing models of stream water chemistry. Water Resources Research, 39 (3), 1055. doi:10.1029/2002WR001528
- Hooper, R.P., Christophersen, N., and Peters, N.E., 1990. Modelling streamwater chemistry as a mixture of soilwater end-members. Georgia. Journal of Hydrology, 116, 321–343. doi:10.1016/0022-1694(90)90131-G
- Hrachowitz, M., et al., 2011. On the value of combined event runoff and tracer analysis to improve understanding of catchment functioning in a data-scarce semi-arid area. Hydrology and Earth System Sciences, 15, 2007–2024. doi:10.5194/hess-15-2007-2011
- Immerzeel, W.W., Van Beek, L.P.H., and Bierkens, M.F.P., 2010. Climate change will affect the Asian Water Towers. Science, 328, 1382–1385. doi:10.1126/science.1183188
- Joel, E., et al., 2009. Controls on the isotopic composition of surface water and precipitation in the Northern Andes, Colombian Eastern Cordillera. Geochimica Et Cosmochimiac Acta, 73, 6999–7018. doi:10.1016/j.gca.2009.08.030
- Jones, I.C., Banner, J.L., and Humphrey, J.D., 2000. Estimating recharge in a tropical karst aquifer. Water Resources Research, 36, 1289–1299. doi:10.1029/1999WR900358
- Katz, B.G., et al., 1997. Use of chemical and isotopic tracers to characterize the interactions between ground water and surface water in mantled karst. Ground Water, 35, 1014–1028. doi:10.1111/gwat.1997.35.issue-6
- Kong, Y. and Pang, Z., 2012. Evaluating the sensitivity of glacier rivers to climate change based on hydrograph separation of discharge. Journal of Hydrology, 121–129. doi:10.1016/j.jhydrol.2012.02.029
- Li, Z.X., et al., 2015b. The evolution and environmental significance of glaciochemistry during the ablation period in the north of Tibetan Plateau, China. Quaternary International, 374, 93–109. doi:10.1016/j.quaint.2014.06.071
- Li, Z.X., et al., 2014. Study on the contribution from cryosphere to runoff in the cold Alpine Basin: a case study of Hulugou River Basin in the Qilian Mountains. Global Planetary Change, 122 (2014), 345–361. doi:10.1016/j.gloplacha.2014.10.001
- Li, Z.X., et al., 2015a. Can monsoon moisture arrive Qilian Mountains in summer? Quaternary International, 358, 113–125. doi:10.1016/j.quaint.2014.08.046
- McGuire, K.J. and McDonnell, J.J., 2006. A review and evaluation of catchment transit time modeling. Journal of Hydrology, 330, 543–563. doi:10.1016/j.jhydrol.2006.04.020
- Mirus, B.B. and Loague, K., 2013. How runoff begins (and ends): characterizing hydrologic response at the catchment scale. Water Resources Research, 49, 1–20. doi:10.1002/wrcr.20218
- Penna, D., et al., 2011. The influence of soil moisture on threshold runoff generation processes in an alpine headwater catchment. Hydrology and Earth System Sciences, 15, 689–702. doi:10.5194/hess-15-689-2011
- Penna, D., et al., 2016. Hydrological response of an Alpine catchment to rainfall and snowmelt events. Journal of Hydrology, 537, 382–397. doi:10.1016/j.jhydrol.2016.03.040
- Roshan, G.R. and Grab, S.W., 2012. Regional climate change scenarios and their impacts on water requirements for wheat production in Iran. International Journal of Plant Production, 6, 239–265.
- Shanley, J.B., et al., 2002. Controls on old and new water contributions to stream flow at some nested catchments in Vermont, USA. Hydrological Processes, 16, 589–609. doi:10.1002/hyp.312
- Sun, C.J., et al., 2016a. Spatial and temporal characteristics of stable isotopes in the Tarim River Basin. Isotopes in Environmental & Health Studies, 52 (3), 281–297. doi:10.1080/10256016.2016.1125350
- Sun, C.J., et al., 2016b. Comparative study of streamflow components in two inland rivers in the Tianshan Mountains, Northwest China. Environmental Earth Sciences, 75 (9), 727. doi:10.1007/s12665-016-5314-1
- Sun, C.J., et al., 2016c. Isotopic time-series partitioning of streamflow components under regional climate change in the Urumqi River, northwest China. Hydrological Sciences Journal, 61 (8), 1443–1459. doi:10.1080/02626667.2015.1031757
- Sun, C.J., et al., 2015. Analysis on the streamflow components of the Typical inland River, Northwest China. Hydrological Sciences Journal, 61 (5), 970–981. doi:10.1080/02626667.2014.1000914
- Tao, P., et al., 2012. Characteristics of water stable isotopes and hydrograph separation in Baishui catchment during the wet season in Mt. Yulong region, south western China. Hydrological Processes. doi:10.1002/hyp.9479
- Tekleab, S., Wenninger, J., and Uhlenbrook, S., 2013. Identifying residence times and streamflow generation processes using δ18O and δ2H in meso-scale catchments in the Abay/Upper Blue Nile, Ethiopia. Hydrology and Earth System Sciences, 10, 10333–10377. doi:10.5194/hessd-10-10333-2013
- Thayyen, R.J., Gergan, J.T., and Dobhal, D.P., 2007. Role of glaciers and snow cover on headwater river hydrology in monsoon regime – micro-scale study of Din Gad catchment, Garhwal Himalaya, India. Current Science, 92, 376–382.
- Wang, S., 2014. Hydrochemical and isotopic characteristics of groundwater in the Yanqi Basin of Xinjiang province, northwest China. Environmental Earth Sciences, 71 (1), 427–440. doi:10.1007/s12665-013-2450-8
- Wels, C., Cornett, R.J., and Lazerte, B.D., 1991. Hydrograph separation: a comparison of geochemical and isotopic tracers. Journal of Hydrology, 122, 253–274. doi:10.1016/0022-1694(91)90181-G
- Zarei, H., et al., 2014. Runoff generation processes during the wet-up phase in a semi-arid basin in Iran. Hydrology and Earth System Sciences, 11, 3787–3810. doi:10.5194/hessd-11-3787-2014
- Zhao, L.J., et al., 2011. Isotopic evidence for the moisture origin and composition of surface runoff in the headwaters of the Heihe River basin. Chinese Science Bulletin, 5656 (1), 58–67.
- Zhonghe, P., et al., 2011. Processes affecting isotope in precipitaiton of an arid region. Tellus B, 63, 353–359.
- Zhou, J.X., et al., 2015. Hydrograph separation in the headwaters of the Shule river basin: combining water chemistry and stable isotopes. Advances in Meteorology, 2015 (2), 1–10, Article ID 830306.
- Zohrabi, N., et al., 2011. Analyzing the two-dimensional plot of the interannual climate variability for detection of the climate change in the Large Karoun River Basin, Iran. African Journal of Biotechnology, 10 (20), 4138–4146.