ABSTRACT
Areas of Western and Central Europe were severely affected by devastating droughts in 2003 and 2015. A sharp decrease in annual atmospheric precipitation led to diminished spring discharges. Analysis of the fluctuations in discharge from three groups of springs in the south of Poland, namely in the Sudetes and Carpathian mountains, and in the German province of Bavaria revealed an average discharge reduction ranging from 52 to 4%. The study proved the existence of a relationship between diminished groundwater recharge from precipitation and the type of rock forming the reservoir as well as the parameters of the rock in the drained catchment. Spring discharge regime was compared for various periods to show that a decline in the regime correlates with a decline in river flow rate; considering the continually changing weather patterns, this correlation is arguably a key factor in sustainable water management.
Editor A. Castellarin Associate editor I. Overton
1 Introduction
The climatic conditions of Western and Central Europe mean that the single most important factor affecting spring discharge regimes is precipitation. A prolonged period without rainfall leads to a drought, first meteorological, then agricultural, hydrological (Maidment Citation1993) and finally hydrogeological (Staśko et al. Citation2008). This in turn causes a gradual reduction in spring discharges or even their temporary disappearance. Studies such as those published by Ma et al. (Citation2004), Gholami et al. (Citation2008) and Korhonen and Kuusisto (Citation2010) document the influence of precipitation levels and hydrogeological parameters of aquifers on spring regimes, emphasizing the importance of this issue in protecting and managing available water resources.
One of the most severe droughts in the last 20 years occurred in June and July 2015 and affected most of the European continent (European Drought Observatory Citation2015). It was particularly severe in France, the Benelux countries, Germany, Hungary, Czech Republic, in northern areas of Italy and Spain, and in Poland. During the drought, the precipitation deficit exceeded 100–130 mm, indicating a reduction by as much as 80% in comparison with the annual average. Continuously high air temperatures combined with a lack of precipitation had adverse effects on agriculture, forestry, water management and even the energy industry. In extreme cases, springs, mountain streams and wells would dry up, depriving local populations of water.
This paper presents the results of measurements and describes the analysis of spring discharge regimes along with their response to low precipitation/recharge.
2 Aim of the study
The paper focuses on the way spring discharges changed in response to decreased precipitation levels during the 2015 drought. In order to be able to investigate this, several datasets were studied. The analysis included precipitation levels recorded between 1996 and 2015, as well as spring discharges measured during the 18-year period 1997–2015 in Germany and in Poland. The standard precipitation index (SPI) classification system served as the basis for determining precipitation conditions for each given year. The change in average spring discharges and the timing of the occurrence of the irregular discharge volume caused by decreased recharge are described, too.
3 Area under study
The area under study includes 17 springs in Western and Central Europe, 10 of which are located in Germany (Bavaria) and seven in Poland (four in the Sudetes Mountains, Lower Silesia Province, one in Opole Province and two in the Carpathian Mountains). The locations are given in . The geographical distribution of the springs enabled the scientists to identify three distinct groups within a 300–350-km distance, the axes of which corresponded with the following meridians: 11.40°, 16.40° and 21.00° ().
4 Method
The data reflecting precipitation levels came from five meteorological observation posts (Hammelburg, Laufen/Oberbayern, Grosser Arber, Halblech-Buching, Neustadt/Oberfranken) monitored by the Bavarian Hydrological Services, as well as seven meteorological stations (Pobiedna, Kowary, Mieroszów, Międzylesie, Słoszów, Głuchołazy, Tylicz) belonging to the observation and data measurement network of the Polish Institute of Meteorology and Water Management. The observation posts in question were located at a variety of altitudes (220–1460 m a.s.l.) and in close proximity to the springs whose discharges were being studied. Precipitation conditions in the area under study were evaluated in order to identify very dry and extremely dry years. This was carried out with the help of the standardized precipitation index (SPI), which is used to determine precipitation conditions on any given time scale and under a wide variety of climactic conditions (McKee et al. Citation1993, Citation1995, Łabędzki Citation2006). In order to calculate the SPI for a given precipitation level (P), the rainfall event was normalized through the use of a transformation function f(P), and then the following formula was employed:
where f(P) = 3√P is the normalized sum of annual precipitation levels, is the average value of the normalized rainfall events, and d is the mean standard deviation of the normalized rainfall events.
The classification system for the precipitation conditions along with the probability of their occurrence is shown in .
Table 1. Classification of precipitation conditions according to the standardized precipitation index (SPI) and the corresponding probabilities.
Data on spring discharges came from the observation posts of the Bavarian Hydrological Services (Germany/Bavaria), the National Hydrogeological Services (Lower Silesia and Opole Province), the environmental monitoring base of the “Multivita” bottling plant in Tylicz (Carpathian Poland Province), and independent research in the Sudetes. Measurements at these posts were usually carried out once every week. In the case of springs where electronic measuring probes had been installed, data recording took place daily. The group of springs under study included resurgence fissures located at different altitudes and water draining from a variety of rocks differing from each other in terms of both lithology and age ().
Table 2. Characteristics of chosen springs.
The analysis of the springs included an evaluation of average discharges during the 1997–2015 period and for individual years, with particular focus on the year 2015. This allowed the springs whose discharges had the greatest decline to be singled out from each of the groups (Bavaria – Lower Silesia and Opole Province – Carpathians). In the case of those springs, variations in discharge were analysed and compared with those recorded during the years when precipitation levels were close to the normal range.
5 Results
5.1 Precipitation levels
Analyses of the precipitation levels in Bavaria point to a significant variation. The highest and lowest levels were recorded during different years, depending on location (). In 2015 in Germany, the lowest precipitation levels were recorded in two of the five monitored stations: Laufen/Oberbayern and Grosser Arber. At other locations, the lowest levels were recorded in 1996, 2003 and 2006. Average annual precipitation levels during the 1996–2015 period ranged from 655 mm (Hammelburg) to 1488 mm (Grosser Arber). In Poland, the precipitation levels recorded at most stations were lowest in the year 2015 (), with the exception of the station in Tylicz, where precipitation levels were lowest in 2003 (628 mm) and in 2015 totalled 755 mm. At individual posts, the difference between the lowest and the average precipitation levels ranged from 164 to 389 mm, while between the year with the highest rainfall levels and the year with the lowest precipitation the difference reached 827 mm (Grosser Arber, ).
Table 3. Precipitation (P) levels (mm) at selected stations.
The data shown in indicate that, in 2015, the Sudetes and parts of Bavaria, experienced the lowest precipitation levels of all the years studied (1996–2015). In Lower Silesia and Opole Province, the SPI method yielded a result of (≤−2.0), indicative of an extremely dry period (). In the Carpathians, 2015 was described as dry, while 2003 was considered extremely dry (Tylicz). In 2015 in Bavaria, extremely low levels of precipitation were recorded at two locations. Dry years included both 2015 and 2003. In other years, annual precipitation deficits were not significant. Precipitation levels in the years preceding 2015 were similar to the annual average in the years 2012–2013 (). A few of the locations recorded precipitation levels that deviated slightly from the average, and allowed the period to be classified as dry or wet.
Table 4. Evaluation of precipitation conditions at selected posts in individual years, based on the SPI classification.
5.2 Spring discharges and regimes
The spring discharge of the four groups in different locations (Sudetes, Bavaria, Carpathian and Bystrzyckie Mountains) was analysed. A comparison was made between the average discharge for the 1997–2015 period and the average discharge established in the year 2015, indicating variations ranging from 54.1 to 36.8% (). Based on the precipitation distribution and levels in 2015, it was noticed that 15 of the 17 springs experienced a decline in discharge.
Table 5. Average discharges (SQ) of the studied springs and percentage change.
Springs located in Lower Silesia (625 and 656) experienced the greatest drop in average discharge, sometimes in excess of 50% of the initial volume. A fairly significant lowering of spring discharge occurred at Różanka (718). In Poland, the springs with the smallest variations in discharge (ranging from −2.3 to −4.8%) were Rudziczka (661) and Zdrój Główny (Z-1). In one case (Bradowiec, Z-7), the discharge actually increased by 36.8%. The discharge of this particular spring has been increasing steadily since 2010, when its catchment system was modernized. In Bavaria, as in Poland, only one spring showed an increase in discharge (22504). In the case of the remaining springs, the discharge decreased by anything from −11.2 to −31.8%, except for the Hohenmühle spring in Bavaria (5503).
During the period of low groundwater recharge in 2015, the five springs monitored by the PSH (Polish Hydrogeological Service) displayed fluctuations in discharge. In the case of the Różanka spring (718), the drop in discharge was particularly significant, reaching 72% in comparison with the annual average. Similarly, the spring in Kowary (625), which originates in granite rocks, showed a 50% drop in discharge. The springs in Szczytna (607) and Rudziczki (661), which originate in sedimentary Cretaceous and Quaternary rocks, appeared to experience much smaller annual reductions in discharge, amounting to just 22 and 14%, respectively. Reliable data from the Carpathians came from Zdrój Główny (Z-1) and pointed to a lowering of the average discharge by 4.8%.
In the case of the springs in Bavaria, which showed the greatest fall in the average discharge (springs 5503, , and 23585, ), no significant increase in discharge was observed in the summer and autumn of 2015, owing to a lack of precipitation. Peak levels were recorded after the thaw and following the spring rains from March to May. Towards the end of November and the beginning of December, spring discharges declined gradually, the meagre rainfall unable to replenish them. A different situation took place in 2013, when precipitation levels were within the normal range. During the summer and autumn rains of that year, between one and several short episodes of significant increase in spring discharge were noted. The discharge of spring 5503 reached maximum levels in response to the rainfall in June, and the amplitude of its variation was close to 10 dm3/s.
In the case of spring 23585, the irregular discharge volume occurred around the same time in both 2013 and 2015. Maximum discharges were observed towards the end of May and the beginning of June, while the lowest levels were measured around the end of the year ().
From January to March or May 2013 and 2015, the discharges of the springs in Lower Silesia and Opole Province were similar (–). In the case of low-discharge springs (652, , and 718, ), no notable discrepancies were found towards the end of the year (October–December) either. The key factor here was the lack of rainfall between May and August 2015, which resulted in the absence of maximum summer levels. In the case of high-discharge springs (), the rainfall deficiency in summer caused a twofold reduction in discharge at the end of the year.
Figure 4. Variations in discharge of spring 625 (Sudetes Mountains) in 2013 and 2015. See for location.
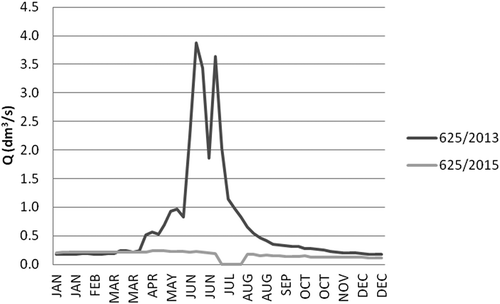
Figure 5. Variations in discharge of spring 718 (Sudetes Mountains) in 2013 and 2015. See for location.
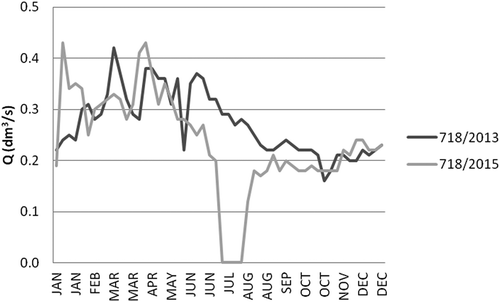
Figure 6. Variations in discharge of springs 607 and 656 (Sudetes Mountains) in 2013 and 2015. See for locations.
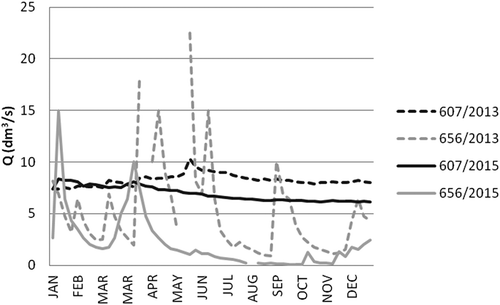
In the Tylicz region, the difference in precipitation levels between 2014 (normal) and 2015 (dry) had a similar effect on the occurrence of irregular discharge volume. Until June, the discharge of spring Z-1 (Zdrój Główny) followed a similar pattern in both years, sometimes with levels in 2015 being higher than those in 2014 (). The rainfall deficiency in July and August 2015 resulted in the absence of recharge and a reduction in spring discharge. As expected, the lowest discharge levels in 2015 were in November, whereas in the normal year, spring discharges did not reach their lowest point until the winter months of December and January.
6 Discussion
Tendencies towards an increase in temperature and the occurrence of extreme events such as long-lasting droughts have been observed in southern Poland. Analyses of meteorological data for the periods 1946–2001 (Bartholy and Pongrácz Citation2007) and 1951–2000 (Łupikasza and Bielec-Bakowska Citation2005) have shown growth in the frequency, time of duration and intensity of heat waves and a drop in the number of days when the volume of rainfall was lower than 5, 1 and 0.1 mm. During these periods a marked increase in and continuous extension of the HWDI (heat wave duration index) could be discerned. The number of days with temperatures exceeding 20°C had also risen. The authors of the IPCC Fourth Assessment Report (Meehl et al. Citation2007) suggest that, in the 21st century, these trends will continue; in milder climates, this may lead to an increased frequency, duration and intensity of heat waves and a greater risk of droughts. This is disturbing, because the research conducted so far (Drużkowski Citation2001, Loáiciga Citation2003, Apaydin Citation2010, Radhakrishnan and Elango Citation2011) seems to indicate that climate changes adversely affect groundwater resources. As a result of drought, over 1000 shallow private wells have dried up in some parts of the Carpathians (Kowalczyk Citation2016). Depending on the region, model simulations for dry scenarios confidently predict a maximum drop in groundwater levels from 3.1 m (Woldeamlak et al. Citation2007) to as much as 40 m b.g.l. (below ground level) (Buczyński and Wcisło Citation2013) in the upper slopes and mountainous watersheds.
A decrease in rainfall leads to considerably reduced infiltration and lowering of the groundwater table, which in turn has a discernible impact on spring discharge. The results obtained in our study bear a close resemblance to the simulations. For the vast majority of the springs, scarce snow cover in the winter of 2014/15 and extremely low rainfall in 2015 contributed directly and substantially to a sharp decline in their discharge.
These results are consistent with the findings of the hydrogeological services under the hydrological system (Sadurski Citation2016). In the years with normal levels of precipitation, such as 2013, the discharges of the these springs are of nival–pluvial origin (–). The initial increase in discharge occurs as a result of the melting of snow cover and rainfall in spring. In June and July, precipitation often causes spring discharges to reach levels even higher than those measured during the vernal peak. The springs’ response to the relatively modest snow cover in the winter of 2014/15 combined with the lack of precipitation during the preceding summer months affected both their average discharge and the features and timing of the occurrence of the irregular discharge volume.
In comparison with the 1996–2015 period, average variations in precipitation levels in 2015 () ranged from −32.9% (Lower Silesia and Opole Province), to −22.2% (Bavaria) and −13.3% (Carpathians). As a result, decreased precipitation levels in 2015 caused the average spring discharge to drop by 36.9% in Lower Silesia and Opole Province and 19.1% in Bavaria.
In Lower Silesia and Opole Province, the abnormally low precipitation in 2015 caused greater variations in spring discharge. In July 2015, spring discharge disappeared altogether at three locations (625, 657, 718, –). However, these incidents were extreme and only temporary, lasting for about three weeks. After that time, the springs resumed their normal flow, but their discharge remained low.
The most dramatic decline in the rainfall and spring discharge was recorded in Lower Silesia and Opole Province, where the rainfall dropped in 2015 by 39.2%, compared with the 1996–2015 period; the same was true for spring discharges, which dropped by 36.9%. Such a fluctuation has an important impact on low river flow, agriculture and water management, including nature conservation (Nyirfa and Harron Citation2001, Tomiałojć Citation2003), in this and other regions.
Conversely, insignificant fluctuations of discharge were noticed in the Tylicz region. These data were corroborated by a separate study of a sharp decline in spring discharge, carried out in the hydrogeological year 2015, i.e. November 2014–January 2015, by Sadurski (Citation2016). He found that the lowest discharge in the Sudetes Mountains was recorded in July 2015. In comparison with the average spanning several years, the figures were lower that month by 2.08 dm3/s. At the beginning of the hydrogeological year, the discharge was usually lower by 0.25–0.7 dm3/s, only to go down subsequently to at least 1 dm3/s. The same pattern for that period was traced in the Carpathians, where the lowest levels were measured in July and August, falling by 1.44 and 1.56 dm3/s, respectively, compared with the region’s overall average. Likewise, Moniewski (Citation2015) observed that, for the majority of springs in Central Europe, winter is an essential period of groundwater recharge, but summer rainfall also plays a key role in the supply to springs. A prevalence of nival–pluvial regime is demonstrated by the time of discharge concentration that falls between early March and mid-July.
Similar results were produced after the analysis of the regime of springs flowing out of the crystalline rocks of Kamieńczyk catchment in the eastern Sudetes, Lower Silesia: 10-year-long systematic as well as periodic observations were consistent in demonstrating a decrease in discharge comparable to other springs monitored within the Lower Silesian network of the PSH. The differences between the overall average for discharge and the 2015 average were within the range 38.1–52.5% (). None of the springs seems to have disappeared during the monitoring, which may be indicative of a large aquifer capacity of groundwater in the drained catchment.
Table 6. Fluctuations in discharge (SQ) of springs monitored periodically in the Kamieńczyk area (Sudetes) in the years 2006–2015 and percentage change.
Research conducted in Germany demonstrated that spring discharges fell steeply following heat waves and lack of rain in the summer, in some cases to a record low in November, according to the Bavarian State Office for the Environment (Bayerisches Landesamt für Umwelt Citation2016). Additional readings made in the autumn of 2015 showed that the spring discharges were much lower than at the beginning of the year. It is noteworthy that some of the springs monitored in 2015 under the research project showed the reverse: they either experienced a rise or only an insignificant drop in the annual discharge, which is attributable to a delayed reaction of the springs to climate changes, notably the rainfall. This reaction depends heavily on the distance between drainage and recharge areas, the depth of the water-bearing layers, and their physical properties, namely hydraulic transmissivity and aquifer capacity. This is largely substantiated by e.g. Fiorillo and Guadagno (Citation2010b), who pointed to a correlation between SPI and karstic spring discharge: they found that the best correlation occurs for time scales of 9 and 12 months. Rice (Citation2007) demonstrated similarly that the springs in central Arizona, USA, had a delayed reaction to climate changes, which would indicate that the spring discharge is in general less vulnerable to rainfall. Buczyński (Citation2017) analysed the connection between geological structure and spring regime, confirming that the springs located in crystalline rocks have a higher coefficient of variation (Cv = 30–42%) than those located in compact sedimentary rocks (Cv = 19–30%). Taking into account retention capabilities and discharge regime in dry seasons, Negi and Joshi (Citation2004) distinguished two types of springs in the Western Himalayas in their study of the reaction to rainfall. Moniewski (Citation2015), in contrast, proved that the outflow from karst springs, which are characterized by higher seasonal discharge, occurs on average about three weeks later than from fissure springs. Altitude was also found to be significant: the discharge of springs located at higher elevations is characterized by greater seasonal variability and a later concentration date.
Recent findings point to a more markedly varied discharge from the springs draining crystalline rocks, which reflects the fact that the immediate environment does not create conditions fostering a retention of massive quantities of groundwater. In Lower Silesia and Opole Province, another pattern emerged: springs located at higher altitudes experienced a more noticeable fall in discharge. Such a pattern cannot be discerned in the case of Bavaria, where the sharpest drop was reported in a spring located at the lowest altitude, flowing out of Triassic sandstones. Furthermore, the springs located in water-bearing rocks whose composition is identical showed enormous variations: e.g. Bavarian springs originating in sandstones showed discharge drops of −11% (spring 17527) down to −46% (spring 5503).
Extremely low rainfall in 2015 had an adverse effect on spring discharge in 2016. Delayed seepage was confirmed in selected springs in August and September of 2016, when the discharge would differ by over 80% of the overall average for extended periods of time. The measurements suggest that low rainfall affects high-discharge springs most severely. Conversely, low-discharge springs would restore their capacity after patchy rains in the autumn and winter months, despite a cessation of outflow in the preceding summer, and their discharge was comparable with the years when rainfall was normal. Fiorillo and Guadagno (Citation2010a) also demonstrated that drought may have much more powerful impact on springs characterized by the highest discharges.
The rate of spring discharge decline may be determined by the Maillet formula (Castany Citation1972), where a key component is the volume of water accumulated at the beginning of the period in which the decline occurs. It is crucial therefore that the assessment of groundwater resources is made before the dry or low-recharge season begins. Another important issue is the features of groundwater reservoirs: the readings and examinations carried out by, for instance, Kowalski (Citation1980) show that recession curves vary extensively, which is suggestive of zones or layers of different transmissivity. This, in turn, incontrovertibly has an effect on the rate at which water is withdrawn from the system. It can be inferred from the analysis of residence time and groundwater flow time that the age of water in porous or fractured rock aquifers ranges from a few to several years (Staśko and Tarka Citation2002). It is understandable, therefore, that a simple recharge impulse moved over from the preceding year has a knock-on effect. The concepts of water-bearing systems and excessive exploitation (Staśko Citation1992, Custodio Citation2002) combine to develop a hypothesis that springs rely on the surrounding reservoirs to be fed in dry seasons. In general, a decrease in discharge of the springs is associated with a lower inflow to the river valleys and with a reduction of streamflow. This depletes the available water resources. Additionally, it causes significant losses in agriculture (Eitzinger et al. Citation2003) and forestry, and adversely affects transport over water.
7 Conclusions
Spring discharge regime is characterized by a plethora of quite strong and varied reactions to diminished precipitation recharge. In the dry years of 1999 and 2003, although preceded by sustained periods of average annual rainfall, no discernible anomalies in spring discharges were detected. This arguably highlights the importance of storage capacity of the water-bearing rocks, as the resources acquired in previous years compensate for temporary water depletion. The extremely dry year 2015, preceded by the dry year 2014, witnessed brief periods, between two and three weeks, of abnormally low discharge. The most noticeable changes were documented for the springs originating in igneous and metamorphic Carboniferous rocks (granites), Lower Palaeozoic rocks (tuffs) and Proterozoic rocks (gneiss and schist). These rocks are typical for their low groundwater volume, which results in sudden and violent reactions to changes in rainfall. Lack of regular recharge leads to a rapid decline in discharge, and, in extreme circumstances, to the disappearance of springs. A decrease by about 32–54% was recorded in the case of Cretaceous sedimentary rocks, whereas high water-bearing rocks showed practically no drop: this is validated by the outflow of spring 661 (Rudziczki) draining Quaternary sedimentary rocks. However, the deep drought in 2015 shows a similar pattern as that illustrated by the rate of the spring reactions recorded in the dry period 1989–1990, and the years 1992 and 2003.
Acknowledgements
We wish to thank associate editor Dr Ian Overton and anonymous reviewers for their detailed comments, which were invaluable in the editing process and in our understanding of methods relevant to groundwater circulation analysis. Suggestions made by the reviewers were very helpful in the process of eliminating errors and imperfections in the first version.
Disclosure statement
No potential conflict of interest was reported by the authors.
Additional information
Funding
References
- Apaydin, A., 2010. Response of groundwater to climate variation: fluctuations of groundwater level and well yields in the Halacli aquifer (Cankiri, Turkey). Environmental Monitoring and Assessment, 165, 653–663. doi:10.1007/s10661-009-0976-8
- Bartholy, J. and Pongrácz, R., 2007. Regional analysis of extreme temperature and precipitation indices for the Carpathian Basin from 1946 to 2001. Global and Planetary Change, 57, 83–95. doi:10.1016/j.gloplacha.2006.11.002
- Bayerisches Landesamt für Umwelt, 2016. Gewässerkundlicher Jahresbericht 2015. Augsburg: LfU.
- Buczyński, S., 2017. Temporal variability of springs in catchment areas located in the Sudeten Mountains. Hydrology Research (in revision). doi:10.2166/nh.2017.229
- Buczyński, S. and Wcisło, M., 2013. Predicting climate-induced changes in groundwater resourcess on the basis of hydrogeological model research: case study of the Carpathian flysch belt. Episodes, 36 (2), 105–114.
- Castany, G., 1972. Poszukiwanie i eksploatacja wód podziemnych [Prospection and exploiting groundwater]. Warszawa: Wyd. Geol.
- Custodio, E., 2002. Aquifer overexploitation: what does it mean? Hydrogeology Journal, 10 (2), 254–277. doi:10.1007/s10040-002-0188-6
- Drużkowski, M., 2001. The influence of natural and human-related factors on the water circulation in the Carpathian foothills (southern Poland). Water Resources Management, 15 (2), 93–108. doi:10.1023/A:1012502702064
- Eitzinger, J., et al., 2003. A simulation study of the effect of soil water balance and water stress on winter wheat production under different climate change scenarios. Agricultural Water Management, 61, 195–217. doi:10.1016/S0378-3774(03)00024-6
- European Drought Observatory, 2015. Drought news August 2015[online]. (Based on data until 31 July 2015). Available from: http://edo.jrc.ec.europa.eu [Accessed 17 Jun 2016].
- Fiorillo, F. and Guadagno, F.M., 2010a. Analysis of spring discharges during droughts: example from karst systems of southern Italy. In: B. Andreo, F. Carrasco, J. Durán, and J. LaMoreaux, eds. Advances in research in Karst Media. Environmental earth sciences. Berlin: Springer.
- Fiorillo, F. and Guadagno, F.M., 2010b. Karst spring discharges analysis in relation to drought periods, using the SPI. Water Resources Management, 24, 1867–1884. doi:10.1007/s11269-009-9528-9
- Gholami, V., Azodi, M., and Taghvaye Salimi, E., 2008. Modeling of karst and alluvial springs discharge in the central Alborz highlands and on the Caspian southern coast. Caspian Journal of Environmental Science, 6 (1), 41–45.
- Korhonen, J. and Kuusisto, E., 2010. Long-term changes in the discharge regime in Finland. Hydrology Research, 41 (3–4), 253–268. doi:10.2166/nh.2010.112
- Kowalczyk, A., 2016. Low groundwater: causes and effects (in Polish with English summary). Technol. Wody, 5, 4–10.
- Kowalski, S., 1980. Charakterystyka hydrogeologiczna źródeł Gór Stołowych [Hydrogeological description of Góry Stołowe springs, in Polish]. Kwartalnik Geologiczny, 24 (4), 885–904.
- Łabędzki, L., 2006. Agricultural drought. Overview problems and methods for monitoring and classification (in Polish with English summary). Water-Environment-Rural Areas, 17, 1–107.
- Loáiciga, H.A., 2003. Climate change and ground water. Annals of the Association of American Geographers, 93 (1), 30–41. doi:10.1111/1467-8306.93103
- Łupikasza, E. and Bielec-Bakowska, Z., 2005. Synoptic conditions of the thermal extreme days in Małopolsca in the second half of the 20th century (in Polish with English summary). Folia Geographica, XXXV–XXXVI, 93–112.
- Ma, T., Wang, Y., and Guo, Q., 2004. Response of carbonate aquifer to climate change in northern China: a case study at the Shentou karst springs. Journal of Hydrology, 297, 274–284. doi:10.1016/j.jhydrol.2004.04.020
- Maidment, D.R., 1993. Handbook of hydrology. New York: McGraw-Hill, Inc.
- McKee, T.B., Doesken, N.J., and Kleist, J., 1993. The relationship of drought frequency and duration to time scales. In: Proceedings of the 8th Conference of Applied Climatology[online], 17−22 January 1993, Anaheim, CA, 179−184. Available from: http://www.droughtmanagement.info/literature/AMS_Relationship_Drought_Frequency_Duration_Time_Scales_1993.pdf [Accessed 24 Feb 2018].
- McKee, T.B., Doesken, N.J., and Kleist, J., 1995. Drought monitoring with multiple time scales. In: Preprints of 9th Conference of Applied Climatology, 15−20 January 1995, Dallas, TX. Boston, MA: American Meteorological Society, 233−236.
- Meehl, G.A., et al., 2007. Global climate projections. In: S. Solomon, ed. Climate change 2007: the physical science basis. Contribution of Working Group I to the Fourth Assessment Report of the Intergovernmental Panel on Climate Change. Cambridge, UK: Cambridge University Press, 747–846.
- Moniewski, P., 2015. Seasonal variability of discharge from selected springs in Central Europe. Episodes, 38 (3), 189–196.
- Negi, G.C.S. and Joshi, V., 2004. Rainfall and spring discharge patterns in two small drainage catchments in the Western Himalayan Mountains, India. The Environmentalist, 24, 19–28. doi:10.1023/B:ENVR.0000046343.45118.78
- Nyirfa, W.N. and Harron, B.H., 2001. Assessment of climate change on the agricultural resources of the Canadian prairies. Regina: Agriculture and Agri-Food Canada, Research report of the Prairie Adaptation Research Collaborative.
- Radhakrishnan, N. and Elango, L., 2011. Study of influence of terrain and climatic factors on groundwater-level fluctuation in a minor river basin using GIS. Geo-Spatial Information Science, 14 (3), 190–197. doi:10.1007/s11806-011-0530-z
- Rice, S.E., 2007. Springs as indicators of drought: physical and geochemical analyses in the Middle Verde river watershed, Arizona. Thesis (MSc). Northern Arizona University, USA.
- Sadurski, A., ed., 2016. Hydrogeological annual report Polish Hydrogeological Survey (in Polish with English summary). Warszawa: Państwowy Instytut Badawczy.
- Staśko, S., 1992. Hydrological drought, overexploitation or inconsistency (in Polish with English summary). Wrocław: Mat. Konf. Problemy Hydrogeol. Pd.-Zach. Polski, 97–102.
- Staśko, S., Olichwer, T., and Tarka, R., 2008. Hydrogeologial drought in the Sudetes. Water resources management in the River Odra catchment (in Polish with English summary). Wrocław: Wyd. RZGW, 37–46.
- Staśko, S. and Tarka, R., 2002. Recharge and groundwater discharge in mountainous areas, based on research in the Śnieżnik Massif (in Polish with English summary). Wrocław: Acta Univ. Wratisl. Prace Geol.-Mineral., 2528, 1–86.
- Tomiałojć, L., 2003. Implications of climate change for nature conservation. In: J.L. Pyka, et al., eds. Man and climate in the 20th century. Wrocław: Acta Universitatis Wratislaviensis, 2542, Stu. Geo., 75, 31–50.
- Woldeamlak, S.T., Batelaan, O., and De Smedt, F., 2007. Effects of climate change on the groundwater system in the Grote-Nete catchment, Belgium. Hydrogeology Journal, 15, 891–901. doi:10.1007/s10040-006-0145-x