ABSTRACT
One-decade-long observations of post-cut-off channel development and oxbow lake formation are presented to offer insight into the morphological response of the Morava River to neck cut-off. The main objectives were: calculation of the amount of sediment released to the channel by collapse of the meander neck; comparison of floodplain erosion and deposition rates before and after cut-off; and description of the rate and pattern of oxbow lake sedimentation. The amount of sediment released due to cut-off (~35 000 m3) equalled 2–5 years of sediment input by lateral erosion averaged for the past six decades. Bank erosion rates substantially exceeded long-term averages (0.63–6.21 m year−1, post cut-off). Despite a low entrance angle, alluvial plugs in a newly formed oxbow lake were established within a few months. Between 2006 and 2016, sedimentation of the alluvial plugs proceeded at an average rate of 0.55 m year−1, reflecting the time since cut-off and the frequency of overbank flows.
Editor A. Castellarin Associate editor G. Di Baldassarre
Introduction
Formation of cut-offs and oxbow lakes is a common process modifying floodplains of freely meandering alluvial rivers (Hooke Citation2007a). Two mechanisms of channel shortening by cut-off have been described – neck cut-off and chute cut-off (Gagliano and Howard Citation1984). The mechanism of neck cut-off comprises a convergent shift of two meander bends via a process of lateral erosion, progressive narrowing and collapse of the meander neck and sudden channel shortening (Erskine et al. Citation1992, Constantine et al. Citation2010b). Chute cut-off develops owing to incision of a shorter channel, or chute, into a point bar or floodplain during overbank flows (Gay et al. Citation1998, Thompson Citation2003). Generally, cut-offs form during a flood or succession of floods (Erskine and Melville Citation1983), nevertheless occurrence of cut-off is supposed to be a consequence of continuous evolution and inherent instability associated with nonlinear dynamics of single-thread sinuous rivers, as proposed by Stølum (Citation1996) or Hooke (Citation2003, Citation2004). Apart from natural causes, actively meandering rivers in developed regions are subject to engineering works (straightening) and numerous artificial cut-offs (Abizaid Citation2005, Winkley Citation1977).
Formation of cut-off essentially influences the morphology and dynamics of meandering rivers in two ways. First, the active channel itself is affected by release of substantial amounts of sediment when the meander neck is breached, the channel gradient is increased by channel shortening, and upstream knickpoint migration and an increase in lateral erosion rates may occur (Hooke Citation1995, Zinger et al. Citation2011, Dieras et al. Citation2013). Second, an abandoned channel reach is produced, which gradually develops into an oxbow lake, when at least in part the ends of the abandoned channel become clogged with sediment (Erskine et al. Citation1982, Cooper and McHenry Citation1989, Constantine and Dunne Citation2008). Besides their geomorphic effect, oxbow lakes have been acknowledged for their ecological importance, as they represent unique aquatic and semi-terrestrial habitats (Bornette et al. Citation1996, Koc et al. Citation2009, Glińska-Lewczuk and Burandt Citation2011).
The role of cut-offs in the morphodynamics of meandering rivers has been investigated by both empirical and theoretical approaches and on various spatial and temporal scales (e.g. Parker and Ikeda Citation1989, Hooke Citation2007b, Camporeale et al. Citation2008, Braudrick et al. Citation2009, Zinger et al. Citation2011). Empirical approaches include essentially three research directions, including (1) reconstructions of planimetric development of meandering rivers from archival documents such as maps and aerial images; (2) repeated field surveys of meandering reaches illustrating rates of lateral channel migration, changes in channel geometry, and formation of oxbow lakes; and (3) physical modelling of the cut-off process in laboratory experiments (flume studies). Most empirical studies aim at the large spatial scale of whole river reaches, whilst small-scale studies of active channel response to individual cut-offs or oxbow lake development are much less frequent. Theoretical approaches based on mathematical models and computer simulations focus on planimetric evolution of meandering channels, or formation and evolution of floodplain architecture by (meandering) rivers.
Traditional approaches to elucidating the temporal dynamics of meandering rivers are based either on analysis of historical maps and aerial images, or on field surveys (Petts et al. Citation1989). Empirical historical reconstructions flourished using combinations with theoretical approaches and later on from adoption of GIS analysis and modelling techniques (Merwade et al. Citation2008). Early works focused on the relation between bend curvature and channel migration rates (Hickin Citation1974, Hickin and Nanson Citation1975, Nanson and Hickin Citation1983). Later, the role of various environmental variables governing planimetric changes of meandering rivers was acknowledged. Channel migration rates and cut-off production proved to be affected by channel geomorphology (Brice Citation1977, Gurnell et al. Citation1994, Hooke and Yorke Citation2010), floodplain land use and vegetation (Hickin Citation1984, Beeson and Doyle Citation1995, Micheli and Kirchner Citation2002), frequency and magnitude of floods (Hooke Citation1979, Larsen et al. Citation2006, Gautier et al. Citation2007), and human interventions (Rinaldi Citation2003, Surian and Rinaldi Citation2003, Michalková et al. Citation2011).
Theoretical contributions (mathematical modelling) to the process of meandering essentially followed a fluid mechanics approach at first (Camporeale et al. Citation2007). Models of various detail and complexity were elaborated, either linear (Ikeda et al. Citation1981, Johannesson and Parker Citation1989, Zolezzi and Seminara Citation2001) or nonlinear (Smith and McLean Citation1984, Shimizu et al. Citation1992, Darby et al. Citation2002). Later on, modelling approaches diversified and other physical mechanisms influencing the meandering dynamics were involved, such as properties of channel bed sediment (Parker and Andrews Citation1985), occurrence of meander cut-offs (Stølum Citation1996, Camporeale et al. Citation2005), tectonic tilting of the Earth’s surface (Sun et al. Citation2001), or riparian biomass dynamics (Perruca et al. Citation2007). Mathematical models concerning morphodynamics of meandering rivers also vary in suitability for different time and spatial scales (Zolezzi et al. Citation2012). Whilst analytical models bring solutions for reach and wavelength scales (e.g. Repetto et al. Citation2002, Chen and Duan Citation2006, Parker et al. Citation2011), numerical models deal with individual events, multiple events or reach scale, and may potentially be applied for long-term evolution of an entire floodplain (Mosselman Citation1998, Darby et al. Citation2002, Jagers Citation2003, Coulthard and Van de Wiel Citation2006, Rüther and Olsen Citation2007). The literature on mathematical modelling of planimetric properties of meandering river systems is vast to date, however, little effort has yet been invested in validation of models with real-world examples (Beck et al. Citation1984, Howard Citation1984, Camporeale et al. Citation2007).
Our study provides information on geomorphic responses to neck cut-off that occurred during the spring flood in 2006 in the quasi-natural reach of the freely meandering lowland Morava River, Czech Republic, affected previously by channel engineering (straightening, bank stabilization) in the 1930s. It is a kind of empirical study focusing on small spatial and short temporal scales, depicting in a complex way active channel development and oxbow lake formation after the disturbance by a single cut-off event.
Observations of cut-off events have been described in several papers (e.g. Johnson and Paynter Citation1967, Gay et al. Citation1998, Stølum Citation1998). However, descriptive field-based studies providing detailed quantitative insight into the process of subsequent active channel development and oxbow lake formation are still surprisingly sparse. Active channel development after cut-off was addressed in only a few studies such as Hooke (Citation1995), Kiss et al. (Citation2008) or Ondruch and Máčka (Citation2015). Oxbow lakes have been studied more intensively, but rather from the viewpoints of water quality (Cullum et al. Citation2006, Glińska-Lewczuk Citation2009), sediment contamination (Bábek et al. Citation2008), assemblages of aquatic organisms (Winemiller et al. Citation2000, Penczak et al. Citation2004, Miranda Citation2005) or hydrological connectivity (Heiler et al. Citation1995, Amoros and Bornette Citation2002, Hudson et al. Citation2012). Geomorphic studies dealing with factors governing morphological and sedimentological evolution of oxbow lakes are less frequent, with exceptions represented by the work of Citterio and Piégay (Citation2009), Constantine et al. (Citation2010a), Le Coz et al. (Citation2010), Toonen et al. (Citation2012), or Miřijovský et al. (Citation2015). Up to date empirical as well as theoretical studies have paid attention rather to larger spatial and temporal scales, targeting the frequency and spatial distribution of cut-off events, and their role in planimetric evolution of meandering channels. This means that more small-scale empirical studies are needed to depict form, rate, spatial extent and duration of changes caused in meandering rivers by cut-offs, either of natural origin or artificial ones.
Our study complements empirical studies dealing with the behaviour of meandering rivers on larger spatial and temporal scales and may be of value for validation of theoretical studies (mathematical models) modelling the role of cut-offs in planimetric evolution of meandering rivers. The main objectives of the present study were (1) to compare floodplain erosion and accretion rates preceding and following a neck cut-off event, (2) to quantify the volume of sediment released into the channel by one discrete cut-off event, and (3) to estimate the rate and pattern of sediment deposition within an abandoned channel reach (oxbow lake). We employed GIS analysis of channel shifts derived from aerial images, geodetic surveys of channel and oxbow lake morphology, geophysical surveying of oxbow lake sediments and granulometric analysis to achieve these goals.
Study area
The Morava River drainage basin is the largest fluvial system in the eastern part of the Czech Republic. Above the confluence with the Danube, the Morava is 353 km long with a catchment area of 26 578 km2. The Morava rises in the Králický Sněžník Mountains close to the Czech–Polish border at an altitude of 1371 m. When the river leaves the Czech Republic in the lowlands of south Moravia, after traversing 269 km of its course, it has a catchment area of 25 273 km2 at an altitude of 148 m.
The study area is in a re-naturalized freely meandering reach of the floodplain stretching along the 13-km reach of the Morava River between the Strážnice, Bzenec–Přívoz and Rohatec settlements in the southeastern part of the Czech Republic. It is located in the Dolnomoravský úval Basin (northern tip of the Vienna Basin, altitude 150–200 m), where the Morava River floodplain is, on average 3.5 km wide (). The sedimentary infill of the Dolnomoravský úval Basin consists of claystones, siltstones and sandstones of the Miocene. Overlying quaternary sediments are represented by the Upper Pleistocene sands of the so-called Moravian Sahara on the western side of the floodplain. These are late glacial Aeolian deposits moulded into sand dunes (Kadlec et al. Citation2015). These sands are eroded by the lateral erosion of the Morava River, and they form unstable concave banks, reaching a height of up to 15 m. Alluvial sediments are formed by a layer up to 10 m thick of sandy gravel formed during the Upper Pleistocene to the Holocene, and are covered by Holocene flood loams. The uplands adjacent to the alluvial valley are composed of flysch sediments of the Magura Nappe unit of the Western Carpathians, whose thrust fronts are oriented from southeast to northwest (Havlíček et al. Citation2008).
Figure 1. Study reach of the Morava River within (a) the Czech Republic, (b) the entire drainage, and (c) the Strážnické Pomoraví region.
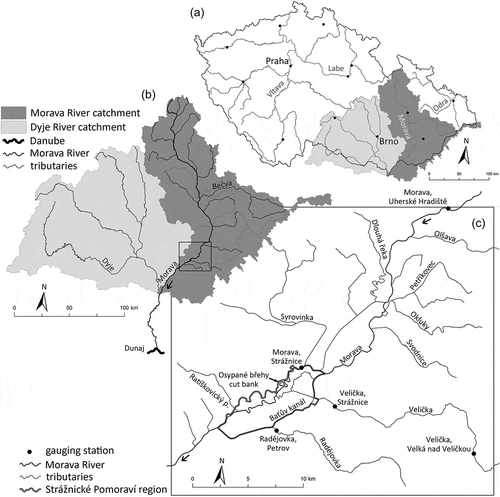
The Strážnice gauging station, which the Czech Hydrometeorological Institute has operated since the 1940s, is located at the upstream end of the study reach. Discharges with N-year recurrence intervals are summarized in . The decades 1961–1970, with 11 events, and 2001–2010, with seven events (Brázdil et al. Citation2011a) are among the time periods with the highest frequencies of floods. To date, the highest peak discharge at the Strážnice station, a flow of 901 m3 s−1, was recorded on 10 July 1997, during what is known as the “flood of the 20th century”, which exceeded the value of Q100. Since cut-off, four events with the magnitude of Q1 or higher have taken place in the studied reach of the Morava River ().
Table 1. List of the N-year discharges of the Morava River at the Strážnice gauging station (river kilometre 133.5, drainage area: 9146 km2) for 1940–2014 (Brázdil et al. Citation2011a).
Figure 2. Daily discharge (Q) of the Morava River at Strážnice gauging station since March 2006. Floods of respective magnitudes are highlighted by horizontal lines.
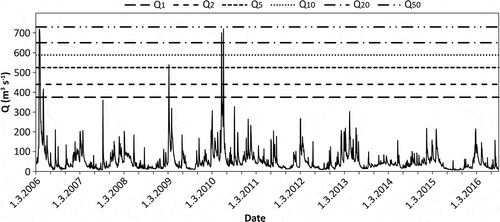
Today, the Morava River in the Strážnické Pomoraví region has a meandering pattern with a sinuosity of approx. 1.6; the width of the meander belt reaches 700 m, the channel bed has a very gentle slope of 0.36‰ and the mean channel width is 70 m. The riverbed is formed mainly by sand and gravel, whereas the river banks are mostly formed by cohesive material of flood loams with a thickness of up to 6 m. Unconsolidated Aeolian sand material is found in some of the bank profiles (Kadlec et al. Citation2009).
Until the 1920s, an anabranching pattern with straight as well as meandering river channels was characteristic of the study river reach. Due to the river regulation works that began in the 1920s, the functionality of smaller anabranching channels has weakened and the flow has progressively been concentrated into a single dominant channel. Tributaries and side channels have been channelized, and a network of drainage ditches was built in order to increase the area of arable land. The length of the dominant channel was shortened by the artificial cut-off of two sequences of meanders. A significant reduction in the connectivity between the channels and the floodplain emerged with the construction of flood-defence dykes, which completely obstructed the overbank flows of the Baťa channel (the former Morávka side channel), the Radějovka River and, partly, of the Morava River itself, and the inundation area dropped to 20% of its original extent (Grygar et al. Citation2010). In the 1920s and 1930s, river regulations triggered the incision of a dominant channel. The most prominent human-related acceleration of fluvial processes took place up until the 1960s (Ondruch and Máčka Citation2015). Currently, the riverbed is approximately as low as 6 m beneath the floodplain. Thus, the frequency of overbank flows has significantly decreased and the floodplain has gradually become hydrologically disconnected; while, at the end of the 19th century, the overbank flows occurred as often as several times a year, nowadays, the bankfull stage is reached only once every 5 years on average (Brázdil et al. Citation2011b).
Methods
Dataset
In order to obtain information on the morphological response of the studied freely meandering reach, it was necessary to gather a substantial dataset. The types of data were twofold: (1) aerial photographs and orthophoto maps for GIS analyses; and (2) field data comprising topographical, electrical resistivity tomography (ERT) and sedimentological data. The whole workflow is depicted in .
Figure 3. Workflow of presented study on the geomorphic effect of a cut-off event on the Morava River.
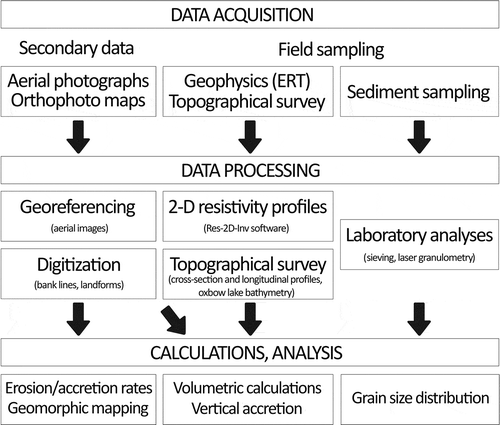
Aerial photographs were taken in April 2003 by the Military Geographical and Hydrometeorological Institute and in September 2006 by J. Wenzel. The orthophoto maps from May 2006, May 2009, October 2012 and May 2014 were all provided by the Czech Office for Surveying, Mapping and Cadastre. Aerial photographs were georeferenced in accordance to the orthophoto map from October 2012. At least 18 reference points were set at the scale of 1:1000. Georeferencing with the employed second-order transformation was accepted if the mean square error was lower than 5 m. The river banks were digitized at a scale of 1:1000. Where the river banks were covered by mature vegetation, they were delineated by merging visible segments of the banks and the central point of the treetops nearest to the channel. Vegetation cover helped distinguish the floodplain surface from the point bars. Due to the deep incision of the riverbed, delineation of the banklines was not affected by fluctuations in the water stage.
A repetitive topographical survey was conducted using a Topcon GPT 9003M total station (resolution of ±2 mm). Longitudinal profiles were constructed in October 2010 and December 2012, applying a natural breaks approach (i.e. irregular steps capturing all the significant changes in the terrain). In total, 82 points were measured for the first date and 106 points were measured for the later date. Cross-sectional profiles at both alluvial plugs were constructed during the field campaigns in November 2014 and May 2016.
The ERT profiling was conducted on the three cross-sections positioned in the vicinity of the breached neck (profiles P3, P4, and PII in ). The profiles were measured during the low water stage, when the batture channel (i.e. the channel connecting the active channel with the oxbow lake via the alluvial plugs) was dry. Resistivity data were acquired using an ARES II/10 multichannel device and a Wenner-Schlumberger array was used to obtain high-resolution (1 m spacing of electrodes) data () for the shallow subsurface area. The root mean square error is 1.33% for the P3 profile, 2.00% for the P4 profile and 1.74% for the PII profile.
Figure 4. Cross-sectional and longitudinal profiles set for topographical, ERT and granulometry measurements. ERT was conducted in profiles P3, P4 and PII.
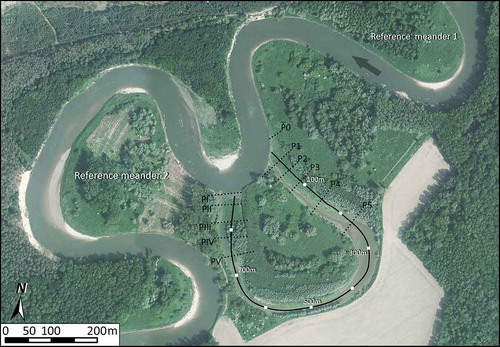
Figure 5. Topographically adjusted ERT profiles for cross-sections in the area of the alluvial plugs.
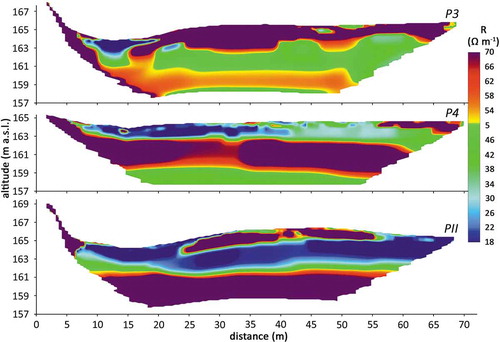
Sediment samples were taken from the surface layer (uppermost 10 cm) in the upstream and downstream alluvial plugs. They were sampled at the lowermost and uppermost points of the cross-sectional profiles taken in November 2014. Samples were taken for grain-size analyses, which were done within two months in the laboratory, where they were manually cleared of organic macroremnants and then left in an oven for 15 min at 550°C in order to exclude the remaining organic matter. The prepared samples were analysed using a CILAS 1190 LD laser granulometer with a measurement range of 0.04–2500 μm. Three repetitive measurements were taken for each sample. The final values underwent further analyses representing an average of all three results.
Data processing
Based on the prepared dataset, geomorphological mapping of landforms was done and main variables were calculated including: (1) erosion and accretion rates, (2) volume of sediment released due to lateral erosion and cut-off event, (3) areal changes of in-channel landforms, and (4) vertical accretion rates.
Lateral erosion and accretion were quantified by constructing migration polygons (Lauer and Parker Citation2008, Micheli and Larsen Citation2010), i.e. the polygons delineated by the channel banklines (centrelines, respectively) at the beginning and end of the studied period. Polygons were constructed for each bank individually instead of for a single centreline, allowing for a separate comparison of the lateral erosion of the outer bank and the floodplain accretion of the inner bank. The floodplain erosion rate and floodplain accretion rate were calculated for the entire meander as the sum of the total area of the migration polygons divided by the length of the studied periods. For more detailed description, we refer to a parallel study by Ondruch and Máčka (Citation2015).
Volumetric calculations of the sediment released by a single cut-off event and by long-term lateral erosion were based on a combination of the planimetric analyses of the aerial photographs or the orthophoto maps, the topographical survey in cross-section profiles and ERT. All parameters used are depicted in . The volume of the material in the meander neck (Vneck) was first calculated simply as:
Figure 6. Sketch of the meander neck geometry with an explanation of the variables entered in the volumetric calculations.
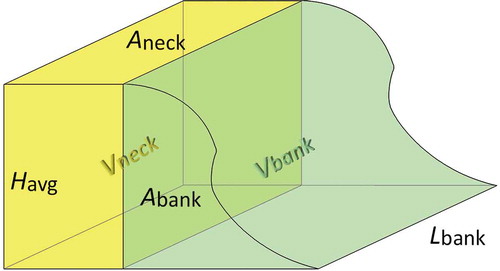
where Havg represents average bank height before cut-off and Aneck is the area of the meander neck that was removed by cut-off. Aneck was calculated as a migration polygon derived from the channel banklines in April 2003 and April 2006. The value of Havg was determined based on analyses of the topographically adjusted ERT profiles. In all three cross-sectional profiles, the apparent interface corresponding to the position of the former channel bed () was observed at an altitude of approximately 161 m a.s.l. This interface is indicated in the ERT profiles by abrupt change in resistivity values (15–20 Ω m−1). The abrupt change was interpreted as a grain-size change from gravels, which formed the former riverbed, to sandy-to-muddy deposits, which represent the infill of the channel after abandonment. The average channel depth was then determined to be 7.5 m within a precision of 0.5 m, which represents an error of 6% for the final volumetric calculation.
However, the aforementioned approach is expected to substantially underestimate the total volume because the bank geometry is not incorporated into the calculations. Therefore, an attempt was made to reconstruct the bank geomorphology in order to reduce the total volumetric calculation error. As no data capturing the channel topography before cut-off were available, reconstruction was based on a comparison of the two reference meanders, situated immediately upstream and downstream from the cut-off (see ). Detailed description of the 10 profiles used in this calculation can be found in Máčka et al. (Citation2011). The volume of the eroded river-bank material (Vbank) was then calculated as:
where Abank is the average cross-sectional area of the river bank derived from 10 cross-sectional profiles from reference meanders, and Lbank is the length of eroded meander neck. Total volume of material (Vcut-off) released by the cut-off was finally calculated (with an estimated total error ~32%) as:
The volume of material entrained into the channel due to lateral erosion (VEr) in the studied meander was calculated as an area of migration (erosion) polygon multiplied by the average channel depth (7.5 m). The erosional areas were calculated by Ondruch and Máčka (Citation2015) from aerial photographs and orthophoto maps. The volumes were calculated for the following periods: 1963–1973, 1973–1982, 1982–1993, 1993–2003 and 2003–2012. Earlier periods (1938–1953 and 1953–1963) were omitted because it was estimated that the active channel had undergone substantial incision until the 1960s (Ondruch and Máčka Citation2015); thus, the value of 7.5 m would be an overestimation beyond an acceptable error for earlier periods. River-bank geometry was excluded in the calculations because it was presumed that lateral erosion progressed in a linear manner across the entire cut bank profile.
Sedimentation rates within the cut-off meander were described by spatial growth of landforms and vertical filling. The planimetric evolution of the landforms in the cut-off meander was quantified by GIS analyses based on the aerial photographs and the orthophoto maps taken on the above-mentioned dates. Areas of landforms were calculated for all studied pre- and post-cut-off periods. All the dates represented low discharge periods with the water stage varying subtly; thus the error in the calculation of the landform areas was less than or equal to 15%. Vertical accretion was calculated simply by altitude differences of longitudinal and cross-sectional profiles.
Results
Active channel
The spring flood of Q50, which occurred in April 2006, breached the 16-m-wide meander neck resulting in an abandonment of 826 m of the active channel. The ratio between the lengths of the active and abandoned channels was 0.22. The sinuosity of the entire 13 km length of the re-naturalized reach dropped from 1.77 to 1.62. The studied meander is situated in the middle of the most dynamic segment (1.5 km long). This segment consists of four meanders and borders with stable channels in both upstream and downstream ends. Because of the cut-off, the segment’s sinuosity dropped from 2.86 to 2.09. Locally, sinuosity of the studied meander decreased from its initial 10.94 to 2.41.
Channel shortening by cut-off resulted in the formation of a new meander bend with increased channel curvature, which, in turn, accelerated the erosion and accumulation processes in the previously stable locations (). During the first month after cut-off, the studied meander experienced removal of approximately 2140 m2 (excluding 3100 m2 due to the breaching of the meander neck) of mature floodplain, and the newly formed cut bank retreated by 11.68 m, on average. Another 5190 m2 of floodplain was eroded between May 2006 and May 2009. The rate of river-bank retreat was 6.21 m year–1. The following three years (May 2009–October 2012) documented the removal of 4940 m2 of floodplain, which corresponds to a river-bank retreat rate of 3.93 m year–1. Floodplain erosion reached 1185 m2 (bankline retreat rate ~0.63 m year–1) during the last period (October 2012–May 2014).
Figure 7. (a) Active channel positions in the studied years. (b) Erosion and accumulation rates for dynamic segment of the Morava River. Whole segment is depicted in . Shadow bars show the position of studied meander.
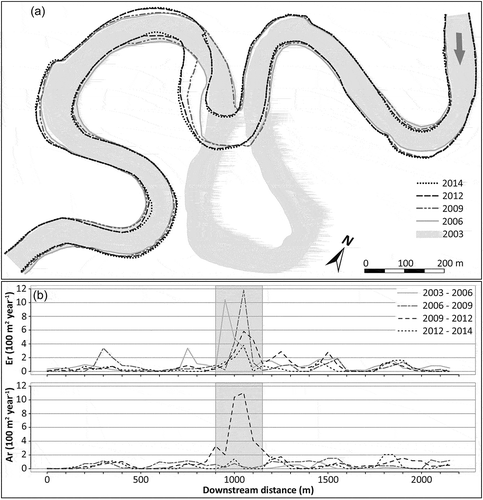
A new point bar started to develop in the active channel immediately after the cut-off; however, a period of 3–6 years after the cut-off was needed for part of the point bar to be attached to the lower floodplain. In total, 8700 m2 of the lower floodplain was formed in 2009–2012. This happened, most probably, because of the flood (Q50) that occurred in November 2010. Another 300 m2 of the lower floodplain was formed in 2012–2014. The total floodplain erosion since the cut-off exceeded the total floodplain development by 3500 m2 and 4385 m2 for 2012 and 2014, respectively.
The volume of sediment released into the channel during the single neck cut-off event was calculated to reach 34 266 m3 (). Yearly input due to lateral erosion varied between 5157 and 15 640 m3. Furthermore, accelerated erosion in 2003–2006, 2006–2009 and 2009–2012 entrained a similarly high amount of material, being due to the cut-off itself (16028, 38918 and 37080 m3, for the respective periods).
Table 2. Comparison of the estimated volume of floodplain removal by long-term pre-cut-off and accelerated post-cut-off lateral erosions of the floodplain (EF) and the discrete cut-off event. AER: total eroded area; VER: total volume of the entrained material; and Δt: length of a period.
Cut-off meander
The establishment of alluvial plugs at the upstream sides of both ends of the cut-off meander occurred within the first few months after cut-off. As the studied meander was cut off under a relatively low (entrance) angle (~24°), flow entering the upstream end of the abandoned channel preserved higher transporting capacity, which resulted in the development of a longer and lower alluvial plug. This is in contrast with the higher and shorter downstream plug, where the angle between active channel and abandoned channel is 105°.
For both the plugs and the point bar, the most prominent growth occurred during the first few months after the cut-off (). In September 2006, these landforms were already covered by dense herbal vegetation, which points to the stability of the landforms and their surface reaching the height above water level for common discharges. After the initial dynamic evolution, periods with limited (2006–2009), re-accelerated (2009–2012) and negligible (2012–2014) spatial growth followed. highlights the differences in the evolution of the upstream and downstream alluvial plugs. Initially, the upstream plug was formed faster than the downstream plug; however, the spatial extent of both plugs gradually became nearly equal. The ratios of the area of the upstream alluvial plug to the area of the downstream alluvial plug were 2.4, 1.9, 1.6, and 1.4 for September 2006, May 2009, October 2012 and May 2014, respectively.
Figure 8. Development of depositional forms within the abandoned meander of the Morava River. Situation was captured in April 2003, September 2006, May 2009, October 2012 and May 2014.
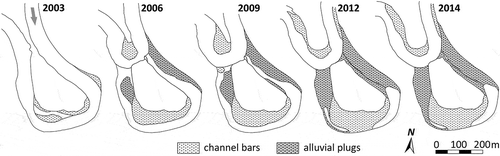
Vertical accretion reached approximately 5 m at the upstream plug (measured for profile P3) and 5.5 m at the downstream plug (PII) between the cut-off event and July 2014 when the site was visited for ERT and topographical survey. The repeated cross-sectional survey in May 2016 documented the slow vertical evolution of all the landforms during the prior 2 years as the accretion reached 40 cm on average for the P3 profile at the upstream plug, and the accretion in the PII profile at the downstream plug was negligible and noticeable only in the lowermost part of the plug.
Two longitudinal profiles constructed in the cut-off meander during the period of accelerated sedimentation (in October 2010 and December 2012) documented the spatial variability in the sedimentation rates and highlighted the fact that both the plugs and the former point bar were the most important sedimentary environments. During these 26 months, on average a 1.5-m-thick layer of sediment accumulated at the upstream plug. Higher accretion occurred at the downstream plug (1.8 m) and at the point bar (1.9 m). The lowest increments in sediment thickness were found in the upstream bend, with accretion only reaching 0.3 m.
No statistically significant difference was proven between the grain size of the samples acquired at the upstream and downstream alluvial plugs. However, taking only the lowermost samples in each cross-section into account, the D50 and D90 values are somewhat higher for the downstream plug. For the topmost samples, the relationship is reversed. Furthermore, the lowermost samples are coarser than the topmost samples in all cases except for the two that are the most distant from the current active channel profiles at the upstream plug. These profiles are often affected by fluctuations of an oxbow lake level, which consequently suppresses the grain size variability.
The upstream plug exhibits a logarithmic relationship between grain size (D50 and D90) and distance from the active channel (L) for all the samples, the batture channel (lowermost samples) as well as the topmost samples. For the downstream plug, this relationship is clearly apparent only for the lowermost samples. A logarithmic relationship was documented between the height (H) and D50 and D90 only for all the samples and the lowermost samples at the downstream plug. No statistically significant relationship was proven for the topmost samples at the downstream plug and all the samples at the upstream plug.
Discussion
Cut-offs are abrupt changes in the morphology of meandering river systems representing crossings of an intrinsic threshold causing fluctuations in channel sinuosity and bed gradient (Knighton Citation2014). Two models of the meander cut-off process have been advocated in the literature – neck cut-off and chute cut-off (see Hooke Citation2004). Chute cut-offs occur when water flowing over the downstream bank of the neck forms a headcut, which propagates across the entire neck, cutting off the meander (Gay et al. Citation1998). Chute cut-off studies have focused on the cut-off process itself (Gay et al. Citation1998, Constantine et al. Citation2010b), the dynamics of the active channel after the cut-off event (Fuller et al. Citation2003, Constantine and Dunne Citation2008) or post-cut-off oxbow lake development (Constantine et al. Citation2010a, Dieras et al. Citation2013). Neck cut-offs occur after the period of convergent shift of two meander bends resulting in breaching of the narrow intermediate neck (Gagliano and Howard Citation1984). Unlike their chute cut-off counterparts, neck cut-offs and consequent development of the active channel and oxbow lake were less studied. As a result, it is still little known how the trajectories of post-cut-off morphological change differ between chute and neck cut-offs. We bring results of decade-long observations on active channel development and oxbow lake sedimentation following neck cut-off of an actively meandering lowland river. Although the active channel and oxbow lake development followed known general trends (development of alluvial plugs, acceleration of active channel lateral migration), the specific local conditions are shown to be influential for the consequent trajectory of morphological development. The morphological changes recorded in both the active channel and oxbow lake are discussed, and comparison with other neck cut-offs of the Morava River documenting the significance of local conditions is presented.
Active channel
The cut-off process is known to play a substantial role in the long-term evolution of meandering rivers by maintaining channel geometry around a steady (critical) state – the principle of the self-organization process as introduced on meandering rivers by Stølum (Citation1996) – and presenting a short noise, which could influence the fluid dynamics even along the entire reach of a river (Camporeale et al. Citation2008). River geometry is commonly described in terms of sinuosity. Within our study reach, the overall sinuosity was too low (1.77) so as to presume that it reached the critical value when the clusters of cut-offs were likely to occur (Hooke Citation2004). Instead, this event could be regarded as an isolated cut-off, occurring over the sub-critical period in the evolution of the reach (Stølum Citation1996). However, taking the most dynamic meandering segment into account, the sinuosity before the cut-off attained a value of 2.86, around which the critical state is more probable.
The function of the studied neck cut-off as a short noise influencing fluid dynamics was observed in the studied reach; however, despite the reduction of a substantial part of the actively meandering segment, the consequences were very local, and, noticeably, they only affected the meander, which underwent cut-off, and the upstream part of the immediately following bend. This suggests that the accelerated bank erosion and new point bar development were driven by the local increase in channel curvature. The channel was able to mitigate the imbalance in the riverbed slope, thus the knickpoint formed did not proceed upstream and facilitate the accelerated erosion on the upstream meander bends. This behaviour differed from the response to an artificial cut-off of six meander bends, which took place within the reach in the 1930s and which caused the accelerated development of an entire dynamic segment. Furthermore, our finding is in contrast to the results reported by Weatherly and Jakob (Citation2014) in their empirical study on the Lillooet River, which exhibited an increase in the erosion rate in both the upstream and downstream directions within 1 year after the cut-off event. A study on the Bollin River and the Dane River by Hooke (Citation1995) revealed a more complex response of erosion to cut-off, reflecting local settings of the particular meander. Our studied meander resembles behaviour described on the Dane River, where one of the studied meanders exhibited erosion immediately downstream the neck cut-off due to changes in flow direction; however, the straight reach that followed further along the course of the river remained stable.
Consistent with the findings of Hooke (Citation1995), in our study, the accelerated floodplain erosion started immediately after cut-off and lasted for approximately 6 years, after which the meander returned to the pre-cut-off erosion rates. In contrast, although the new point bar started to develop simultaneously with erosion, the new floodplain was established as late as the 2009–2012 period. This 3–6-year lag in the formation of a new floodplain reflects the preserved anthropogenic influence from the 1930s, which caused intensive riverbed incision; thus, today, higher discharges with lower frequency are required to cause vertical growth of the point bar and, in turn, floodplain development.
The importance of the cut-off process as a source of material has been acknowledged by studies on chute cut-offs (Fuller et al. Citation2003, Zinger et al. Citation2011, Dieras et al. Citation2013). Zinger et al. (Citation2011) quantified material input due to two successive cut-offs on the Wabash River, and they found that it nearly equalled input due to lateral erosion for the preceding approx. 70 years. However, no study has related long-term lateral erosion with the material input due to neck cut-off. Within our study meander, one discrete event released an amount of material equal to the amount due to lateral erosion of the same meander in 2–5 years, as calculated for the decades since the 1960s. Moreover, accelerated erosion in the newly formed cut bank released material into the active channel with rates substantially higher than most other studied periods (approximately 1.3 times greater than the long-term average). This highlights the secondary effect of cut-off on material input into the channel. Overall, material input due to a single neck cut-off seems to be much less important than material input due to chute cut-off, and it has a more local effect.
Cut-off meander
At the beginning of the abandoned channel development, the most apparent process was the formation of the alluvial plugs. Both alluvial plugs were established within the first few months after cut-off; thus, they were very soon able to divert most of the flow bringing suspended and bedload material into the abandoned channel. However, blockage, i.e. the state with complete flow diversion due to the alluvial plugs preventing water from entering an abandoned channel during normal discharges (Gagliano and Howard Citation1984), was reached 5–6 years after the cut-off. This time frame is consistent with the 2–10 years documented for the Sacramento River (Gagliano and Howard Citation1984) and the 1–7 years documented for the Bollin River and the Dane River (Hooke Citation1995), and it suggests that river size does not substantially control the timing of the complete formation of alluvial plugs.
It has been noted that the diversion angle plays a key role in determining the sedimentation pattern (Constantine et al. Citation2010a). The studied meander cut-off under a low diversion angle (24°) presumes a slower development of the plugs and a faster infill of the abandoned channel. The low entrance angle in the inlet of the studied meander caused the elongation of the upstream plug; however, the observed rapid development of the plugs contradicts the common conception, and points to the importance of the local conditions. Inspection of the aerial images before cut-off suggests that the fast evolution of the upstream plug was conditioned by the pre-cut-off channel topography. Accelerated erosion during the 1930s–1960s resulted in a downstream translation of the meander immediately upstream, leaving the studied meander without a downstream shift. This translation also caused the formation of a channel bar attached to the left bank near the point of inflection. This bar was developing progressively until the cut-off, and, in 1993 (the aerial image in 2003 was taken during a higher flow event; thus, the bars were submerged), it was the most prominent feature opposite the meander neck, which was eventually breached in 2006. This suggests that, during the spring flood in 2006, the bar could drive the flow towards the meander neck, thereby playing a role in positioning the cut-off itself. Furthermore, when the neck was breached, this bar was probably able to divert the flow more readily into the new channel and form favourable conditions for fast development of the alluvial plug.
Within the studied meander, the lowest part of the downstream plug was located at the downstream side of the channel, where the diverted flow was still able to transport the carried material further into the channel. The secondary depression was situated at the upstream side, suggesting the presence of a relatively strong outflow due to centrifugal acceleration and, thus, the presence of a gyre (see cross-sections PII, PIII and PIV in ). This hydraulic pattern with a distinguished elongated gyre is consistent with the one described by Le Coz et al. (Citation2010) in their combined empirical and laboratory study.
Figure 9. (a) Longitudinal and (b) cross-sectional profiles within the abandoned meander of the Morava River.
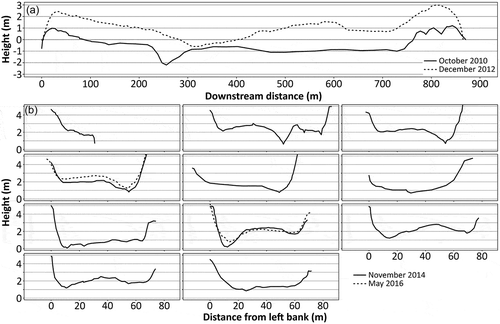
Another characteristic feature of the sedimentation pattern within the studied meander was substantial vertical and spatial growth of the point bar, which proceeded in the downstream direction and which became attached to the downstream plug. Consequently, the downstream bend was nearly filled. In contrast, the upstream bend underwent slow sedimentation with a preserved oxbow lake. This pattern was possibly determined by the low entrance angle that enabled a relatively fast inflow during higher flows, which were able to transport the material further downstream. Then, the material was effectively trapped by the point bar. Additionally, slower development of the downstream plug could facilitate the entrance of water enriched by suspended sediment from the downstream end; thus, it could support sedimentation in the downstream bend.
Sedimentation at both plugs between the cut-off and May 2016 occurred at an average rate of 0.55 m year–1. This rate was substantially higher than the rates observed in the River Rhone basin, France (Citterio and Piégay Citation2009); however, it did not reach values calculated for cut-offs on the Lower Hunter River, Australia, by Erskine et al. (Citation1992). Consistent with Hooke (Citation1995), sedimentation within the studied abandoned meander exhibited high nonlinearity, and this pattern corresponded well with a number of floods with a magnitude of Q1 or higher, which supports the finding of Aalto et al. (Citation2008) from the Strickland River, Papua New Guinea. Moreover, faster rates are expected during the initial stages of the development of abandoned channels; thus, more detailed data would be desirable in order to further compare the sedimentation rates found in this study with the rates reported in other empirical studies.
Channel bottoms of rivers display textural variation across their surfaces (Wolcott and Church Citation1991, Seal and Paola Citation1995, Verdú et al. Citation2005). Patterns of sediment sorting in rivers have commonly been interpreted as a textural response to local differences in flow competence (boundary shear stress) (Powell Citation1998). The size segregation of bed material within cut-off meanders is an imperfectly known phenomenon with virtually no published work, to date. Instead, previous studies have paid attention to the absolute dating of oxbow sediments (e.g. Rowland et al. Citation2005), their contamination histories (e.g. Bábek et al. Citation2008) or the role of oxbow sediments as palaeohydrological archives (e.g. Wolfe et al. Citation2006). The interpretation of recent spatial pattern of grain sizes within the Strážnice meander needs to take into account several factors:
the flow through the cut-off meander is intermittent, depending on the occurrence of increased water levels in the active channel;
the flow through the cut-off meander need not to be unidirectional, only in the downstream direction; however, water may penetrate into the meander via both the upstream and downstream ends;
depending on the relative water elevations of the river and the oxbow lake, the flow through the batture channels (i.e. the channels connecting the active channel with the oxbow lake via the alluvial plugs) may be into or away from the river; and
the flow in the cut-off meander is affected by herbaceous and woody vegetation colonizing the channel bottom.
The interplay of the above-mentioned factors governs the spatiotemporal variability in the sedimentation of different grain sizes within the cut-off meander. Generally, the distribution of grain sizes across the surfaces of the alluvial plugs shows fining in the direction of the flow (from the active channel into the oxbow lake) and bottom-up fining (from the batture channel up to the top of the plug). This situation somewhat resembles the spatial pattern of the grain sizes on channel bars as described by Ashworth and Ferguson (Citation1986), Rice and Church (Citation2010) and Babej et al. (Citation2016).
Comparison with the Litovel meander cut-off
Non-artificial cut-offs are a rather rare phenomenon along the Morava River. There is only one documented example from the last decade to compare with the Strážnice reach (Miřijovský et al. Citation2015); the described neck cut-off occurred in Litovelské Pomoraví (river km 250.3) in 2012 through the collapse of an extremely narrow neck (2.7–3 m, 6 years before cut-off). Breaching of the meander neck was brought about by a much lower discharge than in the case of the Strážnice reach; at the turn of February and March, two successive floods, both with a 1-year recurrence interval, caused the channel shortening. While the first flood (115 m3 s–1) weakened the meander neck, the second flood (118 m3 s–1) caused its collapse.
In the Litovel reach, the mean channel width (19.3 m), the length of the meander bend (approx. 500 m) and the mean discharge (17.1 m3 s–1) were substantially lower than in the Strážnice reach; however, the planform with the flat apex section was fairly similar. Subsequent development after the cut-off was quite different in the two cases. While in the Strážnice reach, high rates of siltation were accompanied by the development of fine-grained alluvial plugs soon after the cut-off, the Litovel reach witnessed lower rates of siltation with a missing alluvial plug at the outflow.
The evolution of the plug at the entrance of the Litovel meander was conditioned by a large wooden structure (wood jam), which was blocking the entire width of the channel and trapping the gravelly sediment. What is now an entrance alluvial plug was, in fact, already formed before the cut-off as a large gravelly deposit trapped by the wood jam. Little of the new sediment was added in the 3 years since the cut-off, as documented by repeated surveying of the cross-section profiles (Knot Citation2015, Miřijovský et al. Citation2015).
Similar to the Strážnice cut-off, the pattern of sedimentation in the Litovel cut-off meander is incompatible with the ideas of Constantine et al. (Citation2010a) about the role of the diversion angle in the development of alluvial plugs. An extensive plug began to develop at the entrance of the Strážnice meander in the first year after the cut-off despite the rather low diversion angle (24°), while in the Litovel meander, in which the diversion angle reached 53°, the entrance plug was an inherited feature, and the aggradation rates were low. The sedimentation pattern, as revealed by repeated cross-section profiles surveying along two respective oxbow lakes, is substantially different. While the Strážnice meander experienced significant changes in the form and dimensions during the 5 years after the cut-off, the post-cut-off development of the Litovel meander was much less dynamic in the same time interval.
Surveying along the Litovel meander from 2011 to 2015 showed that a variable thickness of the sediment was deposited along the oxbow (0.25–1.2 m), with maximum bottom aggradation in a pool approx. 130 m downstream from the cut-off. Today, the overall morphology and dimensions of the oxbow lake approximate the situation of the active channel before the cut-off. The highest rates of siltation were identified first in the deepest sections (pools) located in the meander corners, and then on the lateral bars in the inflow and outflow sections of the meander.
Unlike sedimentation in the oxbow lakes, the development of an active channel in reaction to the cut-off was found to be quite similar in both the Strážnice reach and the Litovel reach. In both cases, the most pronounced morphological change was recorded downstream from the cut-off channel where the development of a new meander loop was initiated. This was accompanied by rapid erosion of the floodplain immediately after the cut-off. In the Litovel reach, the zone of accelerated bank erosion was 60 m long with a mean bank retreat of 3.1 m in the first year, declining progressively to a mean bank retreat of 2.2 m in the third year (Petyniak Citation2014). Similarly, a zone of accelerated bank erosion with a length of 175 m developed in the Strážnice reach, and much higher rates of bank retreat resulted from higher discharge and stream power.
Conceptual models of post-cut-off development need to be elaborated further to incorporate other influencing variables following morphological development. This study shows that a legacy of former engineering modifications, configuration of active channels (as channel bar distribution and size) and large wood occurrence play an important role in release of sediment during a cut-off event and subsequent oxbow lake and active channel development. This study also brings quantitative monitoring data on active channel and oxbow lake development, which are still rather rare.
Summary and conclusions
This study adopted an interdisciplinary approach to gain a more in-depth understanding of the consequences of a cut-off event on a lowland freely meandering river with an artificial training history. A summary of our findings is presented below:
Accelerated bank erosion rates in the meander bend newly formed after the cut-off were documented due to an increase in the channel curvature that substantially exceeded the reach averages for the same period and the long-term averages for the meander itself. However, accelerated erosion remained very local, affecting only the studied meander and the upstream bend of the immediately following meander. This was in contrast with the artificial cut-off of six meanders in the 1930s, which triggered accelerated erosion in both the upstream and downstream directions.
The establishment of a new floodplain was generally slower and lagged by 3–6 years behind erosion, which points to the lasting consequences of the artificial trainings of the 1930s, which resulted in a channel incision reaching 6 m below the current floodplain. Today, larger magnitude floods are needed to cause a point bar accretion sufficient for its attachment to the floodplain.
A vast amount of material was released into the active channel during the cut-off event. This amount equalled the amount released due to the pre-cut-off lateral erosion of the same meander a period of 2–5 years, as calculated for the decades since the 1960s. Moreover, over the course of 6 years, the accelerated erosion released 2.6 times more sediment than during the cut-off event, which highlights the secondary effect of cut-off on material input.
Despite the low entrance angle, both alluvial plugs were formed within a few months after the cut-off. This pattern was pre-determined by channel topography prior to the cut-off that could drive the flow toward the point of breaching. After the event, it was capable of diverting flow into a new active channel, thus forming favourable conditions for the development of the plugs.
Between the cut-off and May 2016, sedimentation at both plugs took place at an average rate of 0.55 m year−1. However, the sedimentation process was documented to be highly nonlinear in time and space, and it corresponded well with the time elapsed since the cut-off and with the occurrence of floods. The highest sediment thickness was found in the downstream bend, whereas the slowest sedimentation occurred in the upstream bend, where a residual lake remained.
The spatial distribution of grain sizes at the alluvial plugs exhibits fining in the flow direction and from the bottom up. This pattern is in agreement with the expected flow hydraulics, and it is apparent despite the dense herbal vegetation cover. Furthermore, it resembles a pattern that has been documented for other types of channel bars.
Overall, this study provides insights into the behaviour of a river that is currently exhibiting spontaneous evolution; however, the river’s artificial history remains apparent. The need to understand these systems has been increasing in importance because it provides useful background information for the restoration strategies that have arisen with the growing social demand to improve the current state of river systems. Moreover, we aim to emphasise the importance of empirical studies, conducted in various settings, in order to assess the interrelationship between generally applicable processes and local specifics. In the studied meander, general hydrological and geomorphic processes associated with meandering rivers and cut-offs were observed; however, their magnitude and pattern were substantially modified by human alterations and local settings. For instance, artificially conditioned riverbed incision increased the amount of sediment that was released into the channel during the neck cut-off event; channel topography, developed prior to cut-off, subdued the effect of diversion angle on post-cut-off sedimentation in the abandoned channel; or change of land cover of the eroded floodplain from arable land to forested surface further modified the flow pattern due to the increased presence of woody debris in the active channel.
In conclusion, the response of meandering river systems to cut-off events is still little known. In our study, we bring an empirical example of river response to single neck cut-off documented on small spatial and short temporal scales. Since only a few studies of this kind have been published to date, more research is needed to elucidate general patterns of channel and oxbow lake post-cut-off development. Limitations of our study may be seen in focusing only on a single cut-off event and applying only a hydro-geomorphological approach (even though a number of field research methods and techniques were used). Based on such limitations, we propose using a multi-disciplinary approach with a combination of field investigations, laboratory experiments and mathematical modelling in future studies. Results of empirical studies on real rivers may be compared advantageously with laboratory experiments. However, such studies are still published only exceptionally, e.g. Le Coz et al. (Citation2010) who used large-scale particle-image velocimetry in their study of the development of an abandoned channel exit. Field studies coupled with laboratory experiments may be of value for simulating flow and sedimentation patterns in oxbow lakes; however, development of mathematical model(s) formalizing the process of neck cut-off, and subsequent responses and interactions of channel and oxbow lake development would provide more a complex picture of the morphodynamics of meandering rivers.
Acknowledgements
The authors would like to thank Lenka Ondráčková and Martin Knot for their help during the field campaigns. Two anonymous reviewers are highly acknowledged for their valuable comments and recommendations, which helped to improve the previous version of the manuscript.
Disclosure statement
No potential conflict of interest was reported by the authors.
Additional information
Funding
References
- Aalto, R., Lauer, J.W., and Dietrich, W.E., 2008. Spatial and temporal dynamics of sediment accumulation and exchange along Strickland River floodplains (Papua New Guinea) over decadal-to-centennial timescales. Journal of Geophysical Research: Earth Surface, 113 (1), 1–22. doi:10.1029/2006JF000627
- Abizaid, C., 2005. An anthropogenic meander cutoff along the Ucayali River, Peruvian Amazon. Geographical Review, 95, 122–135.
- Amoros, C. and Bornette, G., 2002. Connectivity and biocomplexity in waterbodies of riverine floodplains. Freshwater Biology, 47, 761–776. doi:10.1046/j.1365-2427.2002.00905.x
- Ashworth, P.J. and Ferguson, R.I., 1986. Interrelationships of channel processes, changes and sediments in a proglacial braided river. Geografiska Annaler: Series A, Physical Geography, 68 (4), 361–371. doi:10.1080/04353676.1986.11880186
- Babej, J., et al., 2016. Surface grain size variation within gravel bars: a case study of the River Opava, Czech Republic. Geografia Fisica e Dinamica Quaternaria, 39, 3–12.
- Bábek, O., et al., 2008. Contamination history of suspended river sediments accumulated in oxbow lakes over the last 25 years: Morava River (Danube catchment area), Czech Republic. Journal of Soils and Sediments, 8 (3), 165–176. doi:10.1007/s11368-008-0002-8
- Beck, S., Melfi, D.A., and Yalamachili, K., 1984. Lateral migration of the Genesses River, New York. In: C.M. Elliott, ed. River meandering. Reston: American Society of Civil Engineers, 510–517.
- Beeson, C.E. and Doyle, P.F., 1995. Comparison of bank erosion at vegetated and non-vegetated channel bends. Water Resources Bulletin, 31, 983–990. doi:10.1111/j.1752-1688.1995.tb03414.x
- Bornette, G., Amoros, C., and Rostan, J.-C., 1996. River incision and vegetation dynamics in cut-off channels. Aquatic Sciences, 58 (1), 31–51. doi:10.1007/BF00877639
- Braudrick, C.A., et al., 2009. Experimental evidence for the conditions necessary to sustain meandering in coarse-bedded rivers. Proceedings of the National Academy of Sciences, 106, 16936–16941. doi:10.1073/pnas.0909417106
- Brázdil, R., et al., 2011a. Floods and floodplain changes of the River Morava, the Strážnické Pomoraví region (Czech Republic) over the past 130 years. Hydrological Sciences Journal, 56 (7), 1166–1185. doi:10.1080/02626667.2011.608359
- Brázdil, R., et al., 2011b. Fluctuations of floods of the River Morava (Czech Republic) in the 1691–2009 period: interactions of natural and anthropogenic factors. Hydrological Sciences Journal, 56 (3), 468–485. doi:10.1080/02626667.2011.564175
- Brice, J.C., 1977. Lateral migration of the middle Sacramento River, California. US Geological Survey, Water Resources Investigations, 77-43, 1–51.
- Camporeale, C., et al., 2005. On the long-term behaviour of meandering rivers. Water Resources Research, 41, W12403. doi:10.1029/2005WR004109
- Camporeale, C., et al., 2007. Hierarchy of models for meandering rivers and related morphodynamic processes. Reviews of Geophysics, 45, RG1001. doi:10.1029/2005RG000185
- Camporeale, C., et al., 2008. Significance of cut-off in meandering river dynamics. Journal of Geophysical Research, 113 (1), F01001. doi:10.1029/2006JF000694
- Chen, D. and Duan, J.G., 2006. Modeling width adjustment in meandering channels. Journal of Hydrology, 321, 59–76. doi:10.1016/j.jhydrol.2005.07.034
- Citterio, A. and Piégay, H., 2009. Overbank sedimentation rates in former channel lakes: characterization and control factors. Sedimentology, 56, 461–482. doi:10.1111/sed.2009.56.issue-2
- Constantine, J.A., et al., 2010a. Controls on the alluviation of oxbow lakes by bed-material load along the Sacramento river, California. Sedimentology, 57 (2), 389–407. doi:10.1111/j.1365-3091.2009.01084.x
- Constantine, J.A. and Dunne, T., 2008. Meander cut-off and the controls on the production of oxbow lakes. Geology, 36, 23–26. doi:10.1130/G24130A.1
- Constantine, J.A., McLean, S.R., and Dunne, T., 2010b. A mechanism of chute cut-off along large meandering rivers with uniform floodplain topography. Geological Society of America Bulletin, 122, 855–869. doi:10.1130/B26560.1
- Cooper, C.M. and McHenry, J.R., 1989. Sediment accumulation and its effects on a Mississippi River oxbow lake. Environmental Geology, 13, 33–37.
- Coulthard, T.J. and Van de Wiel, M.J., 2006. A cellular model of river meandering. Earth Surface Processes and Landforms, 31, 123–132. doi:10.1002/esp.1315
- Cullum, R.F., et al., 2006. Combined effects of best management practices on water quality in oxbow lakes from agricultural watersheds. Soil and Tillage Research, 90, 212–221. doi:10.1016/j.still.2005.09.004
- Darby, S.E., Alabyan, A.M., and Van de Wiel, M.J., 2002. Numerical simulation of bank erosion and channel migration in meandering rivers. Water Resources Research, 38, 2–21. doi:10.1029/2001WR000602
- Dieras, P.L., et al., 2013. The role of oxbow lakes in the off-channel storage of bed material along the Ain River, France. Geomorphology, 188, 110–119. doi:10.1016/j.geomorph.2012.12.024
- Erskine, W., et al., 1982. Cutoff and oxbow lake. The Australian Geographer, 15, 174–180. doi:10.1080/00049188208702813
- Erskine, W., McFadden, C., and Bishop, P., 1992. Alluvial cut-offs as indicators of former channel conditions. Earth Surface Processes and Landforms, 17, 23–37. doi:10.1002/(ISSN)1096-9837
- Erskine, W. and Melville, M.D., 1983. Impact of the 1978 floods on the channel and floodplain of the lower Macdonald River, NSW. The Australian Geographer, 15, 284–292. doi:10.1080/00049188308702828
- Fuller, I.C., Large, A.R.G., and Milan, D.J., 2003. Quantifying channel development and sediment transfer following chute cut-off in a wandering gravel-bed river. Geomorphology, 54 (3–4), 307–323. doi:10.1016/S0169-555X(02)00374-4
- Gagliano, S.M. and Howard, P.C., 1984. The neck cut-off oxbow lake cycle along the lower Mississippi River. In: C.M. Elliot, ed. River meandering: proceedings of the conference rivers ’83, New Orleans. New York: American Society of Civil Engineers, 147–158.
- Gautier, E., et al., 2007. Temporal relations between meander deformation, water discharge and sediment fluxes in the floodplain of the Rio Beni (Bolivian Amazonia). Earth Surface Processes and Landforms, 32, 230–248. doi:10.1002/(ISSN)1096-9837
- Gay, G.R., et al., 1998. Evolution of cutoffs across meander necks in Powder River, Montana, USA. Earth Surface Processes and Landforms, 23, 651–662. doi:10.1002/(ISSN)1096-9837
- Glińska-Lewczuk, K., 2009. Water quality dynamics of oxbow lakes in young glacial landscape of NE Poland in relation to their hydrological connectivity. Ecological Engineering, 35 (1), 25–37. doi:10.1016/j.ecoleng.2008.08.012
- Glińska-Lewczuk, K. and Burandt, P., 2011. Effect of river straightening on the hydrochemical properties of floodplain lakes: observations from the Łyna and Drwęca Rivers, N Poland. Ecological Engineering, 37 (5), 786–795. doi:10.1016/j.ecoleng.2010.07.028
- Grygar, T.M., et al., 2010. Geochemical tools for the stratigraphic correlation of floodplain deposits of the Morava River in Strážnické Pomoraví, Czech Republic from the last millennium. Catena, 80, 106–121. doi:10.1016/j.catena.2009.09.005
- Gurnell, A.M., Downward, S.R., and Jones, R., 1994. Channel planform change on the river Dee meanders, 1876–1992. Regulated Rivers: Research & Management, 9, 187–204. doi:10.1002/(ISSN)1099-1646
- Havlíček, P., Kučera, Z., and Vachek, M., 2008. Přírodní park Strážnické Pomoraví–Osypané břehy: zkrácení toku Moravy. Zprávy o geologických výzkumech v roce, 2007, 91–92.
- Heiler, G., et al., 1995. Hydrological connectivity and flood pulses as the central aspects for the integrity of a river-floodplain system. Regulated Rivers: Research & Management, 11 (3–4), 351–361. doi:10.1002/rrr.3450110309
- Hickin, E.J., 1974. The development of meanders in natural river channels. American Journal of Science, 274, 414–442. doi:10.2475/ajs.274.4.414
- Hickin, E.J., 1984. Vegetation and river channel dynamics. Canadian Geographer, 28, 111–126. doi:10.1111/cag.1984.28.issue-2
- Hickin, E.J. and Nanson, G.C., 1975. The character of channel migration on the Beatton River, northeast British Columbia, Canada. Geological Society of America Bulletin, 86, 487–494. doi:10.1130/0016-7606(1975)86<487:TCOCMO>2.0.CO;2
- Hooke, J.M., 1979. An analysis of the processes of river bank erosion. Journal of Hydrology, 42, 39–62. doi:10.1016/0022-1694(79)90005-2
- Hooke, J.M., 1995. River channel adjustment to meander cut-offs on the River Bollin and River Dane, northwest England. Geomorphology, 14, 235–253. doi:10.1016/0169-555X(95)00110-Q
- Hooke, J.M., 2003. River meander behaviour and instability: a framework for analysis. Transactions of the Institute of British Geographers, 28, 238–253. doi:10.1111/tran.2003.28.issue-2
- Hooke, J.M., 2004. Cut-offs galore!: occurrence and causes of multiple cut-offs on a meandering river. Geomorphology, 61 (3–4), 225–238. doi:10.1016/j.geomorph.2003.12.006
- Hooke, J.M., 2007a. Spatial variability, mechanisms and propagation of change in an active meandering river. Geomorphology, 84, 277–296. doi:10.1016/j.geomorph.2006.06.005
- Hooke, J.M., 2007b. Complexity, self-organisation and variation in behaviour in meandering rivers. Geomorphology, 91, 236–258. doi:10.1016/j.geomorph.2007.04.021
- Hooke, J.M. and Yorke, L., 2010. Rates, distributions and mechanisms of change in meander morphology over decadal timescales, River Dane, UK. Earth Surface Processes and Landforms, 35, 1601–1614. doi:10.1002/esp.v35:13
- Howard, A.D., 1984. Simulation model of meandering. In: C.M. Elliot, ed. River meandering: proceedings of the conference rivers ’83, New Orleans. New York: American Society of Civil Engineers, 952–963.
- Hudson, P.F., Heitmuller, F.T., and Leitch, M.B., 2012. Hydrologic connectivity of oxbow lakes along the lower Guadalupe River, Texas: the influence of geomorphic and climatic controls on the “flood pulse concept”. Journal of Hydrology, 414–415, 174–183. doi:10.1016/j.jhydrol.2011.10.029
- Ikeda, S.G., Parker, G., and Sawai, K., 1981. Bend theory of river meanders, 1, linear development. Journal of Fluid Mechanics, 112, 363–377. doi:10.1017/S0022112081000451
- Jagers, H.K.A., 2003. Modeling planform changes of braided rivers. PhD thesis. University of Twente, Enschede.
- Johannesson, H. and Parker, G., 1989. Secondary flow in mildly sinuous channel. Journal of Hydraulic Engineering, 115, 289–308. doi:10.1061/(ASCE)0733-9429(1989)115:3(289)
- Johnson, R.H. and Paynter, J., 1967. The development of a cutoff on the River Irk at Chadderton, Lancashire. Geography, 52, 41–49.
- Kadlec, J., et al., 2009. Morava River floodplain development during the last millennium, Strážnické Pomoraví, Czech Republic. The Holocene, 19, 499–509. doi:10.1177/0959683608101398
- Kadlec, J., et al., 2015. Response of fluvial, aeolian and lacustrine systems to Late Pleistocene to Holocene climate change, Lower Moravian Basin, Czech Republic. Geomorphology, 232, 193–208. doi:10.1016/j.geomorph.2014.12.030
- Kiss, T., Fiala, K., and Sipos, G., 2008. Alterations of channel parameters in response to river regulation works since 1840 on the Lower Tisza River (Hungary). Geomorphology, 98, 96–110. doi:10.1016/j.geomorph.2007.02.027
- Knighton, D., 2014. Fluvial forms and processes: a new perspective. London: Routledge.
- Knot, M., 2015. Hydromorfologický monitoring aktuálního vývoje řeky Moravy v Litovelském Pomoraví. Unpublished thesis. Masaryk University.
- Koc, J., Kobus, S., and Glińska-Lewczuk, K., 2009. The significance of oxbow lakes for the ecosystem of afforested river valleys. Journal of Water and Land Development, 13, 115–131.
- Larsen, E.W., Fremier, A.K., and Girvetz, E.H., 2006. Modelling the effects of variable annual flow on river channel meander migration patterns, Sacramento River, California, USA. JAWRA Journal of the American Water Resources Association, 42, 1063–1075. doi:10.1111/j.1752-1688.2006.tb04514.x
- Lauer, J.W. and Parker, G., 2008. Net local removal of floodplain sediment by river meander migration. Geomorphology, 96, 123–149. doi:10.1016/j.geomorph.2007.08.003
- Le Coz, J., et al., 2010. Morphodynamics of the exit of a cut-off meander: experimental findings from field and laboratory studies. Earth Surface Processes and Landforms, 35, 249–261. doi:10.1002/esp.1896
- Máčka, Z., Ondruch, J., and Michalková, M., 2011. Geomorfologické a vegetační změny opuštěného meandru Moravy v oblasti Osypaných břehů pět let po odškrcení. Geologické výzkumy na Moravě a ve Slezsku, 18, 37–42.
- Merwade, V., Cook, A., and Coonrod, J., 2008. GIS techniques for creating river terrain models for hydrodynamic modeling and flood inundation mapping. Environmental Modelling & Software, 23, 1300–1311. doi:10.1016/j.envsoft.2008.03.005
- Michalková, M., et al., 2011. Lateral erosion of the Sacramento River, California (1942–1999), and responses of channel and floodplain lake to human influences. Earth Surface Processes and Landforms, 36, 257–272. doi:10.1002/esp.v36.2
- Micheli, E.R. and Kirchner, J.W., 2002. Effects of wet meadow riparian vegetation on streambank erosion. 1. Remote sensing measurements of streambank migration and erodibility. Earth Surface Processes and Landforms, 27, 627–639. doi:10.1002/esp.v27:6
- Micheli, E.R. and Larsen, E.W., 2010. River channel cut-off dynamics, Sacramento river, California, USA. River Research and Applications, 27, 328–344. doi:10.1002/rra.1360
- Miranda, L.E., 2005. Fish assemblages in oxbow lakes relative to connectivity with the Mississippi River. Transactions of the American Fisheries Society, 134 (6), 1480–1489. doi:10.1577/T05-057.1
- Miřijovský, J., et al., 2015. Spatiotemporal evolution of a unique preserved meandering system in Central Europe – the Morava River near Litovel. Catena, 127, 300–311. doi:10.1016/j.catena.2014.12.006
- Mosselman, E., 1998. Morphological modeling of rivers with erodible banks. Hydrological Processes, 12, 1357–1370. doi:10.1002/(SICI)1099-1085(19980630)12:8<1357::AID-HYP619>3.0.CO;2-7
- Nanson, G.C. and Hickin, E.J., 1983. Channel migration and incision on the Beatton River. Journal of Hydraulic Engineering, 109, 327–337. doi:10.1061/(ASCE)0733-9429(1983)109:3(327)
- Ondruch, J. and Máčka, Z., 2015. Response of lateral channel dynamics of a lowland meandering river to engineering-derived adjustments-an example of the Morava River (Czech Republic). Central European Journal of Geosciences, 7, 588–605.
- Parker, G., et al., 2011. A new framework for modeling the migration of meandering rivers. Earth Surface Processes and Landforms, 36, 70–86. doi:10.1002/esp.v36.1
- Parker, G. and Andrews, E.D., 1985. Sorting of bed load sediment by flow in meander bends. Water Resources Research, 21, 1361–1373. doi:10.1029/WR021i009p01361
- Parker, G. and Ikeda, S., eds., 1989. River meandering. Washington: American Geophysical Union.
- Penczak, T., et al., 2004. Fish assemblage changes relative to environmental factors and time in the Warta River, Poland, and its oxbow lakes. Journal of Fish Biology, 64 (2), 483–501. doi:10.1111/j.0022-1112.2004.00316.x
- Perruca, E., Camporeale, C., and Ridolfi, L., 2007. Significance of riparian vegetation dynamics on meandering river morphodynamics. Water Resources Research, 43, W03430.
- Petts, G.E., Möler, H., and Roux, A.L., eds., 1989. Historical change of large alluvial rivers, Western Europe. Chichester: Wiley.
- Petyniak, O., 2014. Present day condition and future development of the cut-off meander in the Litovelské Pomoraví region. Master thesis. Palacký University in Olomouc.
- Powell, D.M., 1998. Patterns and processes of sediment sorting in gravel-bed rivers. Progress in Physical Geography, 22 (1), 1–32. doi:10.1177/030913339802200101
- Repetto, R., Tubino, M., and Paola, C., 2002. Planimetric instability of channels with variable width. Journal of Fluid Mechanics, 457, 79–109. doi:10.1017/S0022112001007595
- Rice, S.P. and Church, M., 2010. Grain-size sorting within river bars in relation to downstream fining along a wandering channel. Sedimentology, 57 (1), 232–251. doi:10.1111/(ISSN)1365-3091
- Rinaldi, M., 2003. Recent channel adjustments in alluvial rivers of Tuscany, Central Italy. Earth Surface Processes and Landforms, 28, 587–608. doi:10.1002/(ISSN)1096-9837
- Rowland, J.C., et al., 2005. Tie channel sedimentation rates, oxbow formation age and channel migration rate from optically stimulated luminescence (OSL) analysis of floodplain deposits. Earth Surface Processes and Landforms, 30 (9), 1161–1179. doi:10.1002/(ISSN)1096-9837
- Rüther, N. and Olsen, N.R.B., 2007. Modeling free-forming meander evolution in a laboratory channel using three-dimensional computational fluid dynamics. Geomorphology, 89, 308–319. doi:10.1016/j.geomorph.2006.12.009
- Seal, R. and Paola, C., 1995. Observations of downstream fining on the North Fork Toutle River near Mount St. Helens, Washington. Water Resources Research, 31 (5), 1409–1419. doi:10.1029/94WR02976
- Shimizu, Y., Tubino, M., and Watanabe, Y., 1992. Numerical calculations of bed deformation in a range of resonant wavenumbers in meandering channels. Proceedings of Hydraulic Engineering ASCE, 36, 15–22. doi:10.2208/prohe.36.15
- Smith, D. and McLean, S.R., 1984. A model for flow in meandering streams. Water Resources Research, 20, 1301–1315. doi:10.1029/WR020i009p01301
- Stølum, H.H., 1996. River meandering as a self-organized process. Science, 271, 1710–1713. doi:10.1126/science.271.5256.1710
- Stølum, H.-H., 1998. Planform geometry and dynamics of meandering rivers. Geological Society of America Bulletin, 110, 1485–1498. doi:10.1130/0016-7606(1998)110<1485:PGADOM>2.3.CO;2
- Sun, T.P., Meakin, T., and Jøssang, T., 2001. Meander migration and the lateral tilting of floodplains. Water Resources Research, 37, 1485–1502. doi:10.1029/2000WR900343
- Surian, N. and Rinaldi, M., 2003. Morphological response to river engineering and management in alluvial channels in Italy. Geomorphology, 50, 307–326. doi:10.1016/S0169-555X(02)00219-2
- Thompson, D.M., 2003. A geomorphic explanation for a meander cutoff following channel relocation of a coarse-bedded river. Environmental Management, 31, 385–400. doi:10.1007/s00267-002-2842-0
- Toonen, W.H.J., Kleinhans, M.G., and Cohen, K.M., 2012. Sedimentary architecture of abandoned channel fills. Earth Surface Processes and Landforms, 37 (4), 459–472. doi:10.1002/esp.3189
- Verdú, J.M., Batalla, R.J., and Martínez-Casasnovas, J.A., 2005. High-resolution grain-size characterisation of gravel bars using imagery analysis and geo-statistics. Geomorphology, 72 (1–4), 73–93. doi:10.1016/j.geomorph.2005.04.015
- Weatherly, H. and Jakob, M., 2014. Geomorphic response of Lillooet River, British Columbia, to meander cut-offs and base level lowering. Geomorphology, 217, 48–60. doi:10.1016/j.geomorph.2014.04.002
- Winemiller, K.O., et al., 2000. Fish assemblage structure in relation to environmental variation among Brazos River oxbow lakes. Transactions of the American Fisheries Society, 129 (2), 451–468. doi:10.1577/1548-8659(2000)129<0451:FASIRT>2.0.CO;2
- Winkley, B.R., 1977. Man-made cutoffs on the lower Mississippi river, conception, construction, and river response. Vicksburg: Army Engineer District, Potamology Investigations, Report 300-2, 1–209.
- Wolcott, J. and Church, M., 1991. Strategies for sampling spatially heterogeneous phenomena; the example of river gravels. Journal of Sedimentary Petrology, 61 (4), 534–543.
- Wolfe, B.B., et al., 2006. Reconstruction of multi-century flood histories from oxbow lake sediments, Peace-Athabasca Delta, Canada. Hydrological Processes, 20 (19), 4131–4153. doi:10.1002/hyp.6423
- Zinger, J.A., Rhoads, B.L., and Best, J.L., 2011. Extreme sediment pulses generated by bend cut-offs along a large meandering river. Nature Geoscience, 4, 675–678. doi:10.1038/ngeo1260
- Zolezzi, G., Luchi, R., and Tubino, M., 2012. Modeling morphodynamic processes in meandering rivers with spatial width variations. Reviews of Geophysics, 50, RG4005. doi:10.1029/2012RG000392
- Zolezzi, G. and Seminara, G., 2001. Downstream and upstream influence in river meandering, part 1, general theory and application to overdeepening. Journal of Fluid Mechanics, 438, 183–211. doi:10.1017/S002211200100427X