ABSTRACT
This is an invited essay by the Dooge Medallist of the 2017 International Hydrological Prize. The paper reflects a broad perspective on hydrology, as a result of the author’s long experience. It is suggested that transgressing the traditional hydrological perspective, by increasing the scale of research, as well as interdisciplinarization have been, and are likely to remain, key drivers of the development of hydrology. Gaps in knowledge and research challenges are reviewed, and the interlinked areas of stationarity, extremes and projections for the future are discussed. Finally, after reviewing the achievements of Jim Dooge, examples of others following in his footsteps are presented.
Editor D. Koutsoyiannis; Associate editor A. Castellarin
1 Introduction
The title of this essay draws from Quo vadis? – a novel by the Polish writer and 1905 winner of the Nobel Prize for literature, Henryk Sienkiewicz, It also resembles the title of the Quo vadimus? Symposium, organized by the American Geophysical Union in Vancouver, Canada, during the IUGG General Assembly in 1987, at which I delivered a contribution on hydrology. It is also reminiscent of the paper title “Flood protection and management: Quo vadimus?”, by Kundzewicz and Takeuchi (Citation1999). The form of the question I am asking now in the title, using a Latin verb (seconda persona), reflects the fact that I am gradually dissociating myself from research, increasingly becoming a passive observer rather than a doer. This is a comfortable position, though. A saying originating from the Far East states that “onlookers see more than players do”.
Hydrology – science about the terrestrial component of the water (hydrological) cycle – has a dual nature. It is interesting as a science, but has an important applied aspect in management of precious water resources. Water is the essence of life and a major constituent of living matter. Processes of life are based on the continuous exchange of water between living matter and the environment, so that the German poet Goethe (1749–1832) called water Lebenselixier (elixir of life). Water is a universal carrier and solvent of substances. It is needed in virtually every human endeavour. In the Earth’s system, it plays a crucial role in the global mass and heat exchange processes, acting as a climatic thermostat.
The most important external conditions affecting the development of hydrology relate to fundamental problems whereby water is becoming an increasingly scarce resource in increasingly high demand. Hydrology aids humans in solving water-related problems that are becoming increasingly severe, due to the dynamic population increase (globally, more than sixfold since 1850, i.e. from 1.25 billion in 1850 to over 7.6 billion in March 2018), as well as growing anthropopressure and human aspirations for high living standards. In many regions, problems related to water for food, water for the environment, flood protection and water quality have become widespread and urgent. Three categories of aeternal water problems are related to water scarcity, abundance and poor quality – in plain words: too little, too much and too dirty. In order to facilitate an adequate supply of freshwater of suitable quality, enhanced assessment of water resources and likely future demand has to be carried out, and appropriate measures for ensuring water supply, while considering sustainable development, have to be devised.
As Dooge Medallist of the 2017 International Hydrological Prize, I was invited to contribute an article to Hydrological Sciences Journal – the periodical where I served as Editor or Co-Editor for 18 years. When selecting a topic for this paper, I thought about the likely expectations of the readership and decided to sketch a broad perspective reflecting my experience in international hydrology dating back to the mid-1970s, which has been quite intense since my term as a chairman of the IAHS (International Association of Hydrological Sciences) Hydrology 2000 Working GroupFootnote1 in 1983–1987. In Section 2, I express a view that transgressing the traditional hydrological perspective by increasing the scale of research, as well as interdisciplinarization, have been key drivers in the development of hydrology during the past decades. Next, in Section 3, gaps in knowledge and research challenges are reviewed; in Sections 4–6, selected interlinked issues in hydrology, related to stationarity, extreme events and projections for the future, are discussed. Finally, a few examples of individuals following in the footsteps of Jim Dooge are given.
2 Beyond the classical hydrological perspective
Hydrology is an old, proud, science, with its own tradition, culture and language, even if, at times, the status of this science has been found inadequate by hydrologists themselves (see Klemeš Citation1986). Hydrologists have attempted to identify the earliest use of the term “hydrology”. In their recent presentation at EGUFootnote2 in April 2018, Demetris Koutsoyiannis and Nikos Mamassis reported that the noun “hydrologia” (plural) was used in a book, in Latin, dated 1589, while the noun “hydrologie” was part of the title of a book published in French in 1614. It cannot be excluded, though, that somebody digs up an earlier use of the term.
2.1 The scales
The traditional hydrological scale was restricted to the river basin. However, in his pioneering work, Eagleson (Citation1986) promoted the term “global-scale hydrology”, stating: “He who controls the future of global-scale models controls the direction of hydrology.” I feel that this statement remains basically valid now, although, of course, as many women are now at the top of the hydrological field, perhaps it should start “Those who control …”.
Thus, the scale of hydrological studies increases. Fewer single-catchment experiments are being undertaken, and the results of such studies are not easy to publish in increasingly demanding, high-impact journals with interest in the macro-scale. Large-scale and global-scale hydrology have gained much ground. Results of global-scale analyses based on data and modelling are reported in numerous publications. A roster of global datasets is now available in open access (see review by Archfield et al. Citation2015). However, some of the available datasets are quite poor (see Kauffeldt et al. Citation2013), even at relatively small catchment scales (see Beven and Smith Citation2015). Moreover, people are using small-scale parameterizations at inappropriate scales (e.g. use of pedo-transfer functions in global models when they are not even appropriate at field scales) (see Beven et al. Citation2015).
Furthermore, hydrologists increasingly cooperate at the continental (in particular, European) and global scales, and their results are available worldwide in the scientific literature, including dedicated data journals.
In the global system, everything is connected to everything else. Hence, water systems are intimately interwoven with other systems, so that any change in one of these systems induces a change in another.
2.2 Interdisciplinarization
Hydrologists partake in vibrant developments of integrated modelling at large scales. An inter-sectoral impact–model intercomparison has been undertaken, bringing together impact modellers across sectors and scales, worldwide, to create more consistent and comprehensive projections of the impacts of climate change. This activity included hydrological models at global (see Dankers et al. Citation2014, Prudhomme et al. Citation2014, Schewe et al. Citation2014) and regional scales (see Krysanova et al. Citation2017). However, typically, hydrological global-scale models are not subject to rigorous calibration and verification at the river-basin scale, hence the results of such studies can be “thin” in mesoscale and even worse at the catchment scale. Rendering models coupled and interdisciplinary increases the uncertainties dramatically.
The number of models used in hydrology is growing fast. It seems necessary to order the plethora of available models; hence, model intercomparison activities are much needed. An intercomparison of regional models, calibrated and validated at the basin scale, was reported by Vetter et al. (Citation2017). It would be great to be able to critically evaluate the assumptions upon which the models are based. However, this could be complicated, because models can have very many interlinked components. Models are often computerizations of existing concepts that have been linked together. It is important to be aware of simplifications that have been made and of their influence on the results. The availability of increasing computational power does not necessarily lead to the development of new hydrological theories. The starting point in model intercomparison could be to test the models’ performance against observations as being fit for purpose, and rejecting models that perform poorly (see Beven Citation2018). Only after that could a critical evaluation of some process description in the poor models be done (see Krysanova et al. Citation2018).
There are many unsolved problems in pure hydrology. However, in addition to a need for disciplinary depth, it is also necessary to overcome existing compartmentalization by removing traditional disciplinary divides. Indeed, advances in hydrology are very likely associated with interdisciplinary research. The science of the water cycle embraces the interface with other, interconnected, areas, foremost with the larger domains of physics, chemistry and biology. Hydrology intersects with other geosciences (e.g. meteorology, climatology, oceanology, cryospheric sciences, geology, geomorphology, palaeology and soil sciences), as well as ecology, biogeochemistry, water resources management, civil and environmental engineering, hydraulics, remote sensing, environmental sciences, agriculture and forestry, urban systems and sociology. The above list is not exclusive – it can be extended ad libitum. There are many recognized, and already baptized, areas at the interface of hydrology and other scientific disciplines, such as: hydrometeorology, hydro-ecology and ecohydrology, hydrosociology, hydro-informatics, hydropsychology and hydropolitics.
Extension of the scope of hydrology leads to better integration of the sciences and the generation of results that are reflected in high-impact publications, addressed to a larger audience. Hydrologists increasingly connect and collaborate with other disciplines, also in high-budget, interdisciplinary projects that address important broader, real-world issues related to water resources.
Modern hydrology has progressed largely by harnessing advances in technology: growing computer power, application software, telecommunications, remote sensing and data transmission, the Internet of Things. Yet, there is still a considerable problem with hydrological data, despite masses of available information based on remote sensing, for example. Satellite information must be backed up with ground truth data, but networks of hydrological observation stations in many regions have been largely inadequate, and are also shrinking, for financial reasons.
While the disciplinary modelling community attempts to render their (often physically-based or conceptual) models more advanced and more adequate, there is room for alternative approaches, including those of black-box type. Actually, this has been known in hydrology for nearly 90 years now, since the advent of the Instantaneous Unit Hydrograph. Now, we see the advanced data-driven induction of models that lend themselves well to application in hydrology. The occurrence of a publication based on data-mining (Schmidt and Lipson Citation2009) in Science may be interpreted as a sign of nobilitation of this methodology. Schmidt and Lipson (Citation2009) distilled free-form natural laws from experimental data, without assuming any prior knowledge about the physics of the examined process. They automatically identified useful analytical relations driving the system dynamics. A bio-inspired search algorithm was demonstrated to unveil meaningful analytical links in experimental data from a physical system (see Stanisławska et al. Citation2013). Papers based on data-driven models (often prepared by non-hydrologists), aimed at optimal usage of existing data (and therefore subject to David Hume’s induction problem related to responses under future changed conditions), have already penetrated deeply into the hydrological literature. Their authors have ignored disciplinary model knowledge, introducing tools external to hydrology, whose names often originate from the life sciences – such as artificial intelligence, genetic algorithms, neural networks, etc.
2.3 Non-hydrologists take interest in hydrological problems
There are important consequences of crossing disciplinary borders: the transfer of useful tools developed beyond hydrology can be a valuable contribution to hydrology (see Kundzewicz et al. 1987a). However, typically, intruders from other disciplines are not warmly greeted by the community already populating the discipline, for obvious and well-understood reasons. Roederer (Citation1985) gave examples of such reasons, for a more general case of geophysics: greed (competition for limited resources), territorial dominance (we are the champions!), and fear of the unknown (reluctance to learn new things at a mature age).
“There is no need to re-think hydrology from scratch” wrote Montanari and Koutsoyiannis (Citation2014). However, non-hydrologists, i.e. researchers without a “hydrological licence”, are being increasingly active in addressing important topics, strictly in the realm of hydrology. For instance, non-hydrologists, Kleinen and Petschel-Held (Citation2007) published a controversial, innovative, thought-provoking and visionary, flood-related paper in Climatic Change, even if they may have pursued a “mission impossible” from a hydrological viewpoint. More recently, non-hydrologists Boers et al. (Citation2014) published a paper on “prediction of extreme floods” in a high-impact, non-hydrological journal (Nature Communications). It must have been reviewed by non-hydrologists, because none of the three technical terms mentioned in the title of this paper are consistent with the common hydrological interpretation. The paper is devoted to precipitation rather than floods, but it is fair to state that it is an exciting paper on hindcasting intense precipitation, based on analysis of satellite data in South America, with high temporal resolution. Technically, the authors used the concept of “network divergence on directed networks derived from a non-linear synchronization measure”, which is unlikely to appeal to a broader hydrological community.
Another group of non-hydrologists, Willner et al. (Citation2018), computed, based on application of 10 global-scale hydrological models, the increase in flood protection that would be necessary to keep high-end fluvial flood risk at the present level. They carried out the analysis worldwide for sub-national administrative units and found that, in many regions, strong adaptation efforts are needed soon. Their paper, published in Science Advances, conveys a strong practice-oriented message, even if flood hazard projections for a particular area, reported in various studies, can differ considerably (Kundzewicz et al. Citation2017a). Willner et al. (Citation2018) found that more than half of the United States need to at least double their protection level within the next two decades. However, the actionability of these findings raises doubt. For instance, their projections for Poland would appear quite puzzling to the people responsible, locally or regionally, for flood risk reduction, climate change adaptation, and water management in the country. According to the projections in question, throughout the northern half of Poland, the protection has to increase considerably “by a range between 1 and 3 levels”. In fact, the risk of rain-caused river flooding is high in the south rather than in the north of Poland. Large rivers, the Vistula and the Odra, carry water masses from the mountains and the uplands in the southern part of the country to the north of Poland, towards the Baltic Sea. It seems that Willner et al. (Citation2018) advocate for hard actions based on soft, and highly uncertain, premises.
It can be noted that, whether hydrologists greet them or not, researchers from other disciplines tend to appropriate some topical areas, central to hydrology. Examples can be found at the interface between hydrology and climatology. In reports of the IPCC (Intergovernmental Panel on Climate Change), the science of climate (the domain of Working Group I) includes hydrological variables, such as river runoff and soil moisture. This is because the current climate models (Earth system models, ESM) include such variables, even if usually in a rudimentary way. Hydrological variables are needed in ESMs to represent the full water cycle. The projections of precipitation by climate models at both short and long time scales are poor, and the use of bias correction remains necessary, or else the results are out of tune.
3 Gaps in knowledge and research challenges
Recently, Günter Blöschl, IAHS President, issued a call to arms – to all hydrologists of the world, seeking 23 unsolved problems in hydrology that could revolutionize research in the 21st century.Footnote3 The number refers to the idea of 23 unsolved mathematical problems formulated by David Hilbert at the beginning of the 20th century. Such challenging hydrological problems should relate to observed phenomena, be universal (applicable to many regions) and specific (solvable). In fact, Blöschl revisited the idea of creating a list of challenging research areas in hydrology that had already attracted attention in 1964, when the Committee on Status and Needs of Hydrology of the American Geophysical Union (AGU) determined a list of 63 challenging research areas in hydrology.
In the mid-1980s, the Hydrology 2000 Working Group of IAHS (Kundzewicz et al. Citation1987a, Citation1987b) noted with surprise that, in spite of the significant progress achieved in hydrology, many of the problems listed in 1964 still remained at the centre of hydrological research interest two decades later, and continued to provide challenging research directions. Gaps in knowledge were found to be still relevant, since new pressures on the hydrological system required new levels of accuracy and sophistication in problem solving. A sample of the challenging areas mentioned in 1964 is: evapotranspiration, effects of land use, channel behaviour, basin characteristics, hydroclimatic inferences from natural phenomena, rainfall–runoff relations and areal distribution of storm precipitation. Szöllösi-Nagy et al. (Citation1987) observed that after two decades we had “the very same issues on our shopping list”. This observation remains valid – estimating evaporation (and more generally, estimating fluxes), identified as a challenge in 1964, was still recognized as a major challenge in the Hydrology 2020 report (Oki et al. Citation2006). In the report of the NRC (National Research Council of the National Academies, USA) (NRC Citation2012, p. 157), we read: “At the most fundamental level, critical gaps remain in the knowledge about hydrologic fluxes. Evaporation, transpiration, and groundwater fluxes interconnect the water, energy, and biogeochemical cycles yet adequate measurements and estimates of these fluxes are elusive, even for relatively pristine natural systems.” Among the many research gaps recognized in NRC (Citation2012, p. 157) are “the downstream consequences of urban growth or changes in the severity, duration, and occurrence of floods and droughts as a result of climate change.” Also, application of this understanding to making projections for the future is a challenge.
Among the challenging questions in the seminal paper by Eagleson (Citation1986) are the following two: (i) Where will a drop of water evaporating from here fall? and (ii) Where has the drop of water falling here come from? In general terms, NRC (Citation2012, p. 163) has identified today’s three major areas related to complex water problems that define the challenges: (i) water cycle – an agent of change; (ii) water and life; as well as (iii) clean water for the future.
Among general difficulties recognized in the development of hydrology, one can regard complexity of systems and processes, their interconnections and feedbacks. There are highly nonlinear systems in irregular and heterogeneous environments, with thresholds and tipping points. However, they are also strongly constrained by the boundary conditions of mass inputs and catchment characteristics; hence, Budyko’s approach that does not show nonlinear dynamic divergences in behaviour. An important problem is whether the global climate system will do so – and how soon. There is a scarcity of general theories and the value of the signal-to-noise ratio in hydrology is rather low. Furthermore, the traditional disciplinary compartmentalization can also be recognized as an important barrier (yet much has been already done to remove this barrier).
The characteristic property of hydrological systems is that the opportunity to perform active experiments, i.e. to shape the input signal to the system, hardly exists. Typically, geophysical, including hydrological, experiments are performed by nature, and scientists can merely observe them in a passive way. Nevertheless, after having observed different events, the ones appropriate for further study (e.g. those most suitable for a particular objective, such as model identification, or those resembling future conditions of interest) can be evaluated.
One can expect that hydrological research in the future may be directed to areas where the present gaps in knowledge and barriers to development are identified. However, that has not been the case since 1964 (we just have many more models because of the development of computing) – so there is no guarantee that it will change now. Moreover, there is no community definition of what is required to solve some of these problems in terms of measurement techniques. When trying to project the developments of hydrology as a scientific discipline, one can identify gaps in knowledge, as well as extrapolate existing trends, and anticipate further inputs from outside of hydrology.
As noted by Kundzewicz et al. (1987a, Citation1987b), the sources of progress in hydrology are twofold: responding to essential gaps in knowledge and understanding, and responding to burning utilitarian needs, where new solutions may bring large tangible benefits. Hence, the development of hydrological research must provide a trade-off between the, possibly conflicting, priorities of long-term scientific enquiry and of immediate application to economic, societal and environmental problems. This makes it necessary to find a balance between basic research of broad relevance, but no immediate application, and applied research of immediate relevance to a specific, possibly urgent, problem. Sometimes problems of immediate concern cannot be solved without breaking barriers in fundamental research. Two mechanisms can be distinguished that trigger discoveries and push science forwards. One is curiosity-driven fascination with theory and with methods whose development is likely to trigger a breakthrough. The other relates to problems that should be solved, even if the available methods may not suffice. This situation can be expressed colloquially as “necessity is the mother of invention”. Sometimes, the identification of an interdisciplinary problem related to water can define a need for a hydrological solution.
Sivakumar and Singh (Citation2015) edited a special issue of Journal of Hydrologic Engineering, devoted to grand challenges in hydrology. They defined grand challenges by the depth of their scientific meanings and/or the breadth of their societal implications. Among the topics and associated challenges selected by Sivakumar and Singh (Citation2015) were: parameter estimation in hydrological models and data issues – focusing on information content (Beven and Smith Citation2015); resampling methods (Sikorska et al. Citation2015); predictions in ungauged basins (Seibert and McDonnell Citation2015); climate change impact studies – freshwater resources (Kundzewicz and Gerten Citation2015); and hydrosystem–ecosystem interactions (Zalewski Citation2015). Yet, Sivakumar and Singh (Citation2015) admitted that the roster of grand challenges that they selected does not cover such important areas as: hydroinformatics; land-use change hydrology; surface water–groundwater interactions; global water scarcity issues and solutions; coupled human–water relations; and transboundary water management (including the role of hydropolitics).
Among the present strong growth areas are: exohydrology (studying water on other planets); inferences from the Gravity Recovery and Climate Experiment (GRACE); the water–land–food–energy nexus, as well as the concept of water footprint and virtual water trade. However, not all well-auguring areas prove to meet expectations. Some fashions from the “days of yore”, such as the Geomorphological Unit Hydrograph have not triggered any breakthrough and are not considered to hold promise now. As pointed out by Beven (Citation1986), it is more important to know how much to route than how to route. And that problem remains – the prediction of how much runoff to route in an arbitrary catchment is not solved (without a high degree of uncertainty) at any catchment scale, especially when even the observations are hydrologically inconsistent (Beven and Smith Citation2015).
There are inherent limitations to the development of causal models in hydrology. On the one hand, the available data may not suffice for construction of cause–effect relationships. On the other hand, known causal laws may be insufficient for construction of an advanced, and more detailed, model because of the complexity of feedbacks and interrelations in natural systems. Progress in causal modelling cannot be based on mere manipulation of our limited present hydrological knowledge. We should have better observations that would give some idea of patterns and sources of runoff. We cannot even, for example, assess incremental discharges downstream with any certainty, which would be a basic requirement for better modelling concepts.
The computational barrier has practically disappeared in most problem areas (but not if adequate assessments of uncertainties are needed, before we can try to reduce those uncertainties), yet the identification and verification barriers are apparent in hydrological modelling. This is particularly true of the interface between point and areal hydrology. For example, because of strong spatial variability, effective (lumped) values of groundwater system parameters for a whole catchment are difficult to determine. In general, determination of distributed parameters demands a dense, and therefore costly, spatial resolution of measurements. And it may be entirely irrelevant – we should not, for example, be using Darcy-Richards equations at larger scales, as nonlinear processes do not aggregate linearly. Nevertheless, remote sensing and data transmission, combined with suitable models capable of handling large amounts of input data and sufficient ground-truth backup, may solve problems of the hydrology of ungauged areas (Sivapalan et al. Citation2003).
Complexity at a smaller scale in hydrology may turn into simplicity at a larger scale, although the parameters of a model at one scale need not have direct and straightforward relationships to parameters of models at other scales. Trying to connect the laws of behaviour operative at different scales could play a fundamental role in future research in hydrology, but such efforts could be misguided and superseded by “models of everywhere” concepts and learning about places (Beven and Alcock Citation2012).
A truly important challenge is how to effectively communicate research results of hydrology to policy makers, while being honest about uncertainties and testing whether models are, in fact, fit for purpose. The role of hydrological scientists in the transdisciplinary approach with multiple stakeholders needs to be enhanced. Ismail-Zadeh et al. (Citation2017) plead for forging a paradigm shift in disaster science, providing hydrology for society by a transdisciplinary approach.
4 Stationarity: dead or alive? Mortal or immortal?
Heraclitus’s Panta rhei, the observation that everything keeps flowing and changing, was selected as the Leitmotif for a long-term scientific initiative of IAHS (Montanari et al. Citation2013). Indeed, hydrological systems have been always changing, even before the recognized acceleration of climate and land-use changes affected them.
A short paper announcing the death of stationarity, published by Milly et al. (Citation2008) has attracted considerable attention worldwide. Some writers criticize the intriguing and catchy title of the article, questioning the usage of the term “stationarity” and the proclamation of the death of stationarity, so that Milly et al. (Citation2015) published a response to the critiques.
The notion of the adjective “stationary” in the Merriam-Webster dictionary is interpreted as “fixed in a station, course, or mode (immobile)” or “unchanging in condition (e.g. a stationary population)”, and its first known use is dated as early as in the 15th century. That is, when Kolmogorov and Khintchine introduced the scientific foundations of stationary stochastic processes in the 1930s, they helped themselves to a non-scientific, common language expression with some 500 years of history (as adjective; the noun – stationarity – is probably much younger).
Hydrological systems, in general, behave in nonlinear and nonstationary way, even if their behaviour can be considered as locally (piecewise) near-linear and quasi-stationary, over some time interval. Therefore, convenient, and well-developed methods of analysis of linear and stationary systems have been in broad use.
Stationarity has been a practically useful concept that served its role well in a slowly changing world. Some authors perceive a time series of hydrological signals as a superposition of a deterministic (trend, representing change), a periodic (e.g. seasonal oscillation) and a stochastic component, and the last is assumed stationary (Matalas Citation2012, Montanari and Koutsoyiannis Citation2014). Hence, the unquestioned and omnipresent change is encapsulated in the deterministic component.
In the paper by Milly et al. (Citation2008), stationarity was interpreted as the idea that natural systems fluctuate within an unchanging envelope of variability, i.e. the probability density function of the variable of interest is invariant to shifts in time (or it is one-year-periodic). This is a colloquial sense, appealing to the broad readership of Science, which is not restricted to experts in stationary stochastic processes.
One could distinguish three possibilities – stationarity is dead (as per the title of the essay by Milly et al. Citation2008), stationarity is alive, or stationarity has never been born. The last option must clearly be rejected, because use of the notion of stationarity has been ubiquitous in hydrological science and practice. Indeed, stationarity is of great practical convenience; hence, it may well be “wanted dead or alive” – see Lins and Cohn (Citation2011). They noted that “a stationary process with long-term persistence can appear indistinguishable from a nonstationary process with a deterministic trend.” If stationarity is alive then it can be regarded as either mortal or immortal. Montanari and Koutsoyiannis (Citation2014) subscribe to the latter point of view, even if immortality is tantamount to the notion of an infinite lifetime; hence, it is an abstract, ideal, theoretical construct.
The assumption of temporal invariance of the N-year annual maximum river discharge, QN, with probability of exceedence in any one year being 1/N, is of particular practical importance in hydrological design (e.g. determination of design floods for structural flood defences – dikes, embankments, reservoir spillways, relief channels, etc.). In a nonstationary world, QN determined for the 1980s can be considerably different from QN determined for the 2010s, and even more so for the 2040s or 2070s.
5 Hydrological extremes
Hydrological extremes, floods and droughts, remain at the forefront of hydrological research. On the one hand, the damage caused by them has been high and increasing. For instance, floods continue to kill thousands of people annually and cause material loss of the order of tens of billions of US dollars. On the other hand, various aspects of hydrological extremes raise considerable scientific interest within hydrology and beyond.
As noted in Kundzewicz et al. (Citation2017b), it has not been possible to find ubiquitous flood hazard changes in observation records for Europe, so far. However, an increasing trend has been detected in the number of large floods, even if the year-to-year, as well as the decadal, variabilities are strong.
An increase in the horizon and accuracy of drought and flood forecasting remains among essential challenges. This includes forecasting of propensity to occurrence of droughts and floods in the rhythm of oscillation of the atmosphere–ocean system (e.g. ENSO: El Niño Southern Oscillation; NAO: North Atlantic Oscillation; AMO: Atlantic Multidecadal Oscillation; PDO: Pacific Decadal Oscillation).
The concept of flood control design is of crucial importance in natural hazard risk reduction, water management and climate change adaptation. This necessitates flood frequency studies, possibly including those of “mission-impossible” type, such as determination of a 1000-year flood based on 30 years of data. This is clearly a hopeless task, requiring huge extrapolation, even under the assumption of stationarity!
The Clausius-Clapeyron equation (which has recently been developed via the principle of maximum entropy by Koutsoyiannis Citation2012, Citation2014) suggests theoretical links between climate change and flood risk, since a warming should lead to an increase in saturation vapour pressure, hence an increase in the potential of intense precipitation. However, an increase in a potential state is not equivalent to an increase of the real state.
Floods may be caused by several generation mechanisms: intense and/or long-lasting precipitation, snowmelt, rain on snow or ice, flow obstruction (e.g. ice jam, landslide), dam failure, storm surge, urban inundations due to inadequate storm sewerage, or groundwater saturation. Hence, often flood-related corollaries hold for a subset of flood-generating mechanisms.
The Panta Rhei framework embraces changes in flood risk (see Kreibich et al. Citation2017) that depend on (Kundzewicz and Schellnhuber Citation2004):
(a) changes in socio-economic systems (land-use change, increasing exposure and damage potential – floodplain development, growing wealth in flood-prone areas, changing flood-risk perception);
(b) changes in hydrological/terrestrial systems (land-cover change accompanying land-use change: urbanization, deforestation, elimination of natural inundation areas – wetlands, floodplains; river regulation – riverbed straightening and shortening, embankments; damming of rivers, changing conditions of transformation of precipitation into runoff by way of increase of impermeable areas, watershed management, structural flood defences); and
(c) changes in climate and atmospheric system (holding capacity of the atmosphere, intense precipitation, changing precipitation phase, seasonality, snowmelt pattern, atmospheric circulation patterns).
The mechanisms of changes in flood hazard and flood risk can be really complex, as demonstrated in Wyzga et al. (Citation2018). Hence, the topical area of flood research covers far more than hydrology. But then uncertainties just become greater, due to “wrong assumptions, unknowns and surprises in the dynamics of human–water systems” (Di Baldassarre et al. Citation2016). So the issue is how to be precautionary in such circumstances (see Beven Citation2011).
Flood-related research deals with water resources management (e.g. implementation of the Floods Directive of the European Union), flood risk reduction, adaptation to climate change, sociology, economy, law, decision science, management, sociology, administration, governance, biology and ecology (e.g. the flood pulse concept).
The topical area of drought recognizes the taxonomy of droughts. A drought can be meteorological (precipitation deficit), agricultural (soil moisture deficit), hydrological (related to surface waters: streamflow droughts – low river stage, streamflow; lake level; or groundwater – low aquifer recharge and groundwater level), environmental, or economic – incurring ecosystem or financial losses. The driving forces include: precipitation deficiency, atmospheric circulation patterns, temperature, catchment storage, as well as factors in the domain of anthropogenics: water extraction and use – overcultivation, mis-management. The impacts of drought embrace: problems and losses in water supply for agriculture, industry, municipal systems, energy – hydropower loss, lack of cooling water; malnutrition; famine; ecological consequences (e.g. rivers running dry); wildfires; and water quality (less water to dilute pollutants).
A grand challenge related to hydrological extremes appeals to both the climate and the hydrology communities: “to understand the nature of ongoing changes in climate and hydrology and the apparent anomalies that exist in reconciling their extreme manifestations” (NRC Citation2011).
The field of hydrological extremes – floods and droughts – is an area where it is absolutely crucial to bridge hydrology with other disciplines. To paraphrase Gilbert White – hydrological extremes are acts of God, while people are responsible for the damage. One cannot influence the variability of precipitation, yet one can do a lot to reduce flood and drought risk. In the STARFLOOD project, a roster of strategies useful in flood risk reduction was examined (Driessen et al. Citation2016, Hegger et al. Citation2016). The two principal strategies assume flood-risk prevention (keeping people away from destructive water) and flood defence (keeping destructive water away from people) (Dieperink et al. Citation2016). The former aims to decrease the consequences of flooding by decreasing the exposure of people and property via prohibiting or discouraging development in areas at risk of flooding (e.g. spatial planning, re-allotment policy, expropriation policy). The latter aims to decrease the probability of areas being flooded through infrastructural works (e.g. dikes, dams, embankments) that increase the capacity of existing channels for water conveyance, or the creation of new water storage spaces. In addition, at times – despite all the efforts towards flood-risk prevention and flood defence – destructive water reaches humans and their wealth; therefore, strategies of flood risk mitigation, flood preparation (including flood forecasting and warning) and flood recovery are necessary.
6 Hydrological projections for the future
There is no doubt that hydrological projections for the future are badly needed to inform water management. Montanari and Koutsoyiannis (Citation2014) criticized assumptions of perfect knowledge, perfect information and exact predictions that clearly cannot stand (but, actually, are not made). They stated that many “deem future scenarios obtained with deterministic models as credible predictions of the distant future”. Well, this cannot be true for authors using deterministic models in impact studies, as the uncertainties of projections (rather than predictions, not to give a false feeling of fine accuracy and credibility) are broadly recognized and often quantified, e.g. Kundzewicz et al. (Citation2017a). Uncertainty assessment related to the projections of impacts has become a widely applied state-of-the-art approach (Kundzewicz et al. Citation2018). “Are climate models ready for prime time in water resources management applications or is more research needed?” asked Kundzewicz and Stakhiv (Citation2010).
Projections for the future can only be based on models whose core concepts are physically-based, to the greatest extent possible, because it is a plausible assumption that the laws of physics will not change in the unknown, and largely unknowable, albeit definitely warmer, future. However, this is not strictly true for hydrological models in which the changes are dominated by changes to the input signals. A data-based model might suffice if a sufficient range of conditions is available. The exceptions are if the catchment characteristics are changing significantly, or if the future is expected to be totally out of the range of the past. But neither is taken into account in most impact studies, where models are calibrated on past data and no account is taken of changes in catchment characteristics. The assumption then is that the concepts hold outside the range of calibration. This might be just as untrue as for an extrapolated data-based model, since the concept might be quite wrong.
It is recommended to use multiple hydrological models in impact studies, but then an important dilemma related to the model selection arises, as discussed in Krysanova et al. (Citation2018). Is it better to use all the available hydrological models (multi-model ensemble) disregarding their performance in the historical period, or to use a subset of the available models consisting of only those that were able to mimic past observations sufficiently well? The authors argue that the second approach is more reliable for getting credible projections with lower uncertainty, and suggest guidelines for evaluation of the global and catchment-scale models intended for impact studies.
However, some experts (e.g. Koutsoyiannis et al. Citation2008, Citation2009, Beven Citation2011, Citation2018) take the stance that these studies seem to be a waste of time and money, especially since the climate scenarios are unreliable. We do not need climate projections to be precautionary about future change – see for example the sensitivity analyses of Prudhomme et al. (Citation2013). The only reason for doing so would be to be able to estimate the probabilities in a risk-based decision analysis, but the projections and model ensemble do not give probabilities anyway (in particular they are incomplete in important ways) so decisions need to be made in quite a different way based on costs of “future-proofing”. If we convert projections to probabilities (as well as formally determine uncertainties) using e.g. Bayesian methods, the result is not satisfactory (see Tyralis and Koutsoyiannis Citation2017).
The main problem related to GCMs (general circulation models) in the hydrological context is the large discrepancy between GCM projections over some regions (e.g. Africa), responsible for a major share in total uncertainty. Another great problem is whether or not to use bias correction of GCMs which leads to a nonlinear transformation. Further, there is still low spatial resolution of GCMs, and the need for downscaling. At the global hydrological models end, existing models should be evaluated on their performance in the historical period if they are intended for impact studies (see Krysanova et al. Citation2018).
The present state of the Earth system is unique and unprecedented, having “exited the envelope of natural variability” (Milly et al. Citation2008). This could be for several reasons related to climate change and – more broadly – anthropopressure, e.g. changes in land management. The term “anthropocene” was coined per analogiam to geological parlance as the totality of human activities, whose effects can be regarded as comparable to those caused by geological factors (Crutzen Citation2002). Steffen et al. (Citation2015) hypothetise that human activity has already led to crossing of four planetary boundaries (related to climate change, loss of biosphere integrity, land-system change, and altered biogeochemical cycles, but not water resources). However, some experts contest the last element of this list. Transgressing a planetary boundary may drive the Earth system into a less hospitable state, since processes regulating the stability and resilience of the system, and providing conditions upon which our societies depend, are adversely affected.
No doubt it is necessary to prepare for the existing variability of water availability (for which mankind is not adequately prepared today), but this is not likely to be sufficient for future changes (see Kundzewicz et al. Citation2014). Under strong and irreducible uncertainty, two alternative courses of action can be envisaged: the precautionary principle (a variation of the min-max concept – to choose the approach minimizing the worst outcome), and adaptive management, based on ensembles and multi-model probabilistic approaches (Kundzewicz et al. Citation2018). Yet, Beven (Citation2011), having confessed that he believed in climate change, soberly raised the question: “how precautionary do we need to be in planning for the future?”
Noting that climate change has rendered water management more difficult due to uncertainty in future changes of hydrological conditions, Döll et al. (Citation2015) advocated for extending the approach of adaptive Integrated Water Resources Management (IWRM) by considering the risk of climate change. They called for embracing the uncertainty of future climate and its impacts in decision making, by probabilistic assessment of the future water conditions for different scenarios, and “developing a portfolio of low-regret solutions that reduce vulnerability and can be implemented and modified progressively as future conditions evolve.” However, that does not require “probabilistic” impact studies. In fact, climate modellers do not put probabilities on scenarios. Nor do they require climate projections to look at sensitivity to greater extremes.
Now, for a range of reasons (climate change, anthropopressure, land-use and land-cover change, and urbanization in particular), a 100-year flood for a particular location can be dramatically different from a design flood determined for some time in the distant past. Hence, despite the huge uncertainty of projections, practitioners and water managers in some countries and regions (e.g. in Europe) are already considering explicitly how to incorporate the potential effects of global change into policies and specific design guidelines via reasonable adjustments (Kundzewicz et al. Citation2017a, Citation2008). Developments in this regard that clearly illustrate the science–policy interface have taken place in the USA and are likely to be of considerable interest to the HSJ readership.
On 30 January 2015, former US President Barack Obama issued Executive Order (EO) 13690 on Establishing a Federal Flood Risk Management Standard and a Process for Further Soliciting and Considering Stakeholder Input,Footnote4 replacing the old EO11988 (after 38 years of validity). The document states that it is the policy of the USA to improve the resilience of communities and Federal assets against the impacts of flooding and to recognize the risks and losses due to climate change and other threats. As per EO13690, establishing a floodplain results from using one of the following approaches (bold font added for emphasis):
the elevation and flood hazard area that result from using a climate-informed science approach that uses the best-available, actionable hydrologic and hydraulic data and methods that integrate current and future changes in flooding based on climate science. This approach will also include an emphasis on whether the action is a critical action as one of the factors to be considered when conducting the analysis;
the elevation and flood hazard area that result from using the freeboard value, reached by adding an additional 2 feet to the base flood elevation for non-critical actions and by adding an additional 3 feet to the base flood elevation for critical actions;
the area subject to flooding by the 0.2 percent annual chance flood; or
the elevation and flood hazard area that result from using any other method identified in an update to the FFRMS.Footnote5
Examples of critical buildings in (i) and (ii) above are hospitals and evacuation centres. So, indeed, institutionally, we do not need climate projections to be precautionary (cf. the idea of an additional 2 or 3 feet [~0.6 or 0.9 m], as a safety margin).
It turned out that the life of EO13690 was rather short. On 15 August 2017, President Donald Trump issued EO13807: Establishing Discipline and Accountability in the Environmental Review and Permitting Process for Infrastructure,Footnote6 in which EO13690 was revoked. Section 1 of EO13807, which chimes with Trump’s motto “make America great again”, explains the rationale (bold font added for emphasis):
America needs increased infrastructure investment to strengthen our economy, enhance our competitiveness in world trade, create jobs and increase wages for our workers, and reduce the costs of goods and services for our families. The poor condition of America’s infrastructure has been estimated to cost a typical American household thousands of dollars each year. Inefficiencies in current infrastructure project decisions, including management of environmental reviews and permit decisions or authorizations, have delayed infrastructure investments, increased project costs, and blocked the American people from enjoying improved infrastructure that would benefit our economy, society, and environment. More efficient and effective Federal infrastructure decisions can transform our economy, so the Federal Government, as a whole, must change the way it processes environmental reviews and authorization decisions.
Improved flood mitigation measures (and maintenance) would seem to be a good example of worthwhile investment to protect assets in the USA or elsewhere, especially given the catastrophic losses caused by many recent floods, including three mega-floods in North America (USA and Puerto Rico) since the introduction of EO13807. The cost–benefit ratio is often small, even without worrying about future changes in conditions. So there is some politics about the statement in EO13690 to “integrate current and future changes in flooding based on climate science” rather than just being precautionary. To say that climate change might be dominating the flood-risk change factor in the future (as evidenced by climate and sociological projections) is still a political statement and should be recognized as such.
In fact, virtually all scientists gathered around the IAHS Panta Rhei banner would agree that things are changing. Floods in Europe do not really show that yet, but we would expect them to do so before too long, and some of us worry that things might change much more rapidly than the constrained future climate scenarios suggest. So, with or without climate scenarios, we expect the frequencies of occurrence of both extremes to change – with the expectation of an increase in economic and social consequences. Since we do not invest enough in being precautionary now (except perhaps in the Netherlands, where flood safety standards are very high), the message should be that we need to invest more – without knowing the future frequencies of occurrence. So, a major challenge is – how can this be done?
7 On the shoulders of giants
Professor James (Jim) C. I. Dooge (1922–2010), the patron of the science medal of the International Hydrological Prize of IAHS, UNESCO and WMO, was a man of great talent and extraordinary achievements, laying the foundations of the linear theory of hydrological systems (Dooge Citation1973). He was one of the first hydrologists to recognize the potential of climate change science and climate change impact science, long before publishing in the area (Dooge Citation1990, Citation1992a, Citation1992b). Jim led professional learned societies, being President of IAHS in 1975–1979, Secretary-General of the International Council for Science (ICSU) in 1980–1982 and President of ICSU in 1993–1996. He built a brilliant hydrological career in both practice (Electricity Supply Board in Ireland and Irish Office of Public Works) and academia – after two years at the University of Iowa, USA, Jim was appointed Professor at University College Cork, Ireland, in 1958 and at University College Dublin in 1980. Jim was an expert consultant to specialized United Nations agencies, such as UNESCO, WMO, UNEP, FAO, as well as to the Directorate General (DG) XII (research) of the European Commission.
Jim was also very successful in politics. His political career started with being elected to the Dublin (Baile Átha Cliath) County Council for the Fine Gael party in 1948. He was first elected to the Irish Senate (Seanad Éireann) in 1961 and continued to serve until 1987, being Chairman (Cathaoirleach) from 1973 to 1977. He was a close policy adviser to Dr FitzGerald, leader of Fine Gael and Prime Minister. Jim was appointed Minister for Foreign Affairs of the Republic of Ireland and played a role in the development of the European Union during the Irish presidency in 1984.
Being the recipient of the Dooge Medal of the 2017 International Hydrology Prize of IAHS, UNESCO and WMO has indeed a very special meaning to me. I had the privilege to know Jim and to collaborate with him, and have fine memories of my visit to Dublin on exchange with the Royal Irish Academy in 1982/83, at Jim’s invitation. In the 1980s, together with a few colleagues from Poland, I collaborated with Jim, who paid numerous visits to our country (). Together, we worked on what we called “Doogology”, but there were only a handful of people, worldwide, interested in this rather theoretical area. Hence, even though papers prepared in cooperation with Jim were published in fine, disciplinary, peer-reviewed journals, rated in ISI Web of Science (e.g. Dooge et al. Citation1983, Kundzewicz and Dooge Citation1985, Citation1989, Romanowicz et al. Citation1988), they have not been among the bibliometric leaders, in terms of citations.
Figure 1. James C. I. Dooge (right) and the author, Zbigniew W. Kundzewicz (left) in Poland in 1985. At the time, Jim was five years younger than I am now. Tempora mutantur et nos mutamur in illis.
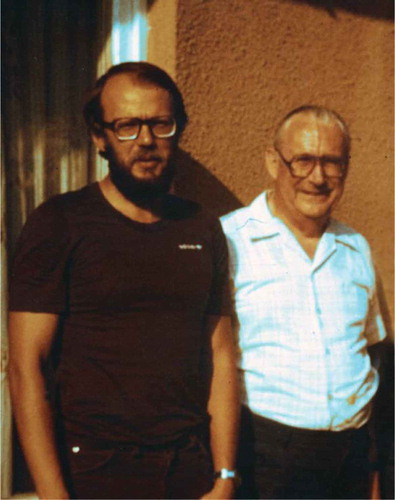
Jim Dooge provided a fine example of an ambitious, self-assured, talented and hard-working hydrologist reaching to the stars. There have been other hydrologists who followed in Jim’s footsteps in one way or another. Several post-Dooge hydrologists have reached high places, being interested in interfaces with other areas and open to interdisciplinary cooperation.
Hydrologists have held leading positions in international learned societies, extending beyond hydrology. For instance, Uri Shamir was President of the International Union of Geodesy and Geophysics (IUGG), Günter Blöschl was President (2013–2015) and Alberto Montanari is President-elect (2019–2021)Footnote7 of the European Geosciences Union (EGU). Oscar E. Meinzer and Peter S. Eagleson were presidents of the American Geophysical Union (1947–1948 and 1986–1988, respectively). Hydrologists have become members of many national academies, as well as international academies such as the Pontifical Academy of Sciences (Ignacio Rodriguez-Iturbe), Academia Europaea (Ghislain de Marsily, Zbigniew W. Kundzewicz), and the Royal Society of London (Keith Beven). Taikan Oki is Senior Vice-Rector of the United Nations University and Assistant Secretary-General of the United Nations.
There are dozens of articles, authored or co-authored by hydrologists, that have been published in prestigious, high-impact and high-circulation scientific periodicals, such as Science and Nature and their journal families, as well as the Proceedings of the National Academy of Sciences (USA).
Hydrologists compete for large research programmes and projects with representatives of other disciplines and sometimes succeed. At the University of Saskatchewan, Canada, Howard WheaterFootnote8 has won a gigantic, Ca$143m grant for implementation of the programme “Global Water Futures – Solutions to Water Threats in an Era of Global Change”, which has likely been the world’s largest, university-led, single-funding envelope dedicated to water research. Günter Blöschl has won a very prestigious advanced grant “Deciphering River Flood Change” from the European Research Council.
Success in fundraising for research projects often results from expectations as to the importance of the project for society, even if the idea may be curiosity driven. However, in general, the competition is becoming increasingly tough, worldwide. It was noted in NRC (Citation2012, Fig. 5.5) that, in the USA, the number of grant proposals in hydrology increases, but the success rate decreases in time.
Some hydrologists have made brilliant careers in administration and politics. One path is for hydrologists, trained in leading universities, to make spectacular careers after returning to their home countries. Thus, Guo Shenglian, who made his PhD in Galway, Ireland, under Jim Nash, became Vice Governor in the People’s Government of Hubei Province in China (population in excess of 57 million) and Deputy to the National People’s Congress (several times). Chen Jining, a former associate of Howard Wheater, became President of Tsinghua University, Beijing, and Environment Minister in China.
I have been associated with the international hydrological society, IAHS, for a long time – actually, throughout my entire scientific career. The beginning dates back to a divided world when Poland was on the “wrong” side of the impermeable “iron curtain”. In 1975, a year after graduating from the Technical University of Warsaw, I attended my first scientific conference abroad – the IAHS conference in Bratislava, Czechoslovakia, to which I could travel without having to leave the former Soviet bloc. In 1983, at the IUGG General Assembly in Hamburg, I became a member of the IAHS Hydrology 2000 Working Group, and soon I was elected a chairman of this group. At the IUGG General Assembly in Vancouver, in August 1987, I delivered the hydrology contribution to the Quo Vadimus Symposium and was the first recipient of the Tison Award of the IAHS. Then, I served as Secretary of ICWRS (1991–1999) and IAHS Editor (1997–2015).
In addition to IAHS, I have been seriously involved in the activities of the Intergovernmental Panel on Climate Change (IPCC), where I played many roles over a period of more than two decades, since 1994. I have been Co-ordinating Lead Author four times – probably more than anyone else. The IPCC Technical Paper on Climate Change and Water (Bates et al. Citation2008), which I co-edited and co-authored, and which was published in all six official languages of the United Nations, is quite well known and cited in thousands of publications (3710 citations, according to Google Scholar, 2 June 2018). In the most recent IPCC initiative, the Sixth Assessment Report, which is planned to bear fruit in 2021, I have been invited to act as a Review Editor of the water chapter (Working Group II).
My activities in IAHS and IPCC gave me the opportunity to interact with, and take lessons from, intellectual giants of the hydrology and climate communities. Jim Dooge was one of them.
Acknowledgements
I have been witness to a considerable part of the recent history of hydrology and have had the privilege to know people who made this history, and to collaborate with them. The international scientists, extending beyond hydrology, who have influenced me most and to whom I owe very much, are/were (in chronological order): Erich Plate, Jim Dooge, Vit Klemeš, John Rodda, John Schellnhuber and Demetris Koutsoyiannis, as well as two eminent Polish scientists: Witold Strupczewski and Zdzisław Kaczmarek.
I also wish to acknowledge Keith Beven, Attilio Castellarin, Demetris Koutsoyiannis, Valentina Krysanova, and Kuniyoshi Takeuchi, who generously offered many useful comments on a draft of this essay. In several instances I truly benefited from their wise advice. I am grateful to Frances Watkins for her very useful comments and for editing this contribution.
I wish to thank Demetris Koutsoyiannis for his long-term collaboration and friendship and for, together with Alberto Montanari, proposing my nomination for the 2017 Dooge Medal of the International Hydrology Prize. Furthermore, I wish to express my gratitude to 11 IAHS national committees for supporting my nomination and to the inter-agency award committee who made the decision. Special thanks go to my wife and family for love, patience, and every support along my scientific path.
Disclosure statement
No potential conflict of interest was reported by the author.
Notes
1 Composition of the IAHS Hydrology 2000 Working Group: Zbigniew W. Kundzewicz (chair), Abel Afouda, Edmund D. Andrews, David N. Collins, Jose Cordova-Rodriguez, Lars Gottschalk, Syed I. Hasnain, Jan Szolgay, Andras Szöllösi-Nagy, Bruce W. Webb.
2 Presentation “From mythology to science: the development of scientific hydrological concepts in the Greek antiquity” by Demetris Koutsoyiannis and Nikos Mamassis at the EGU 2018 – Session HS1.8: History of Hydrology, http://www.itia.ntua.gr/en/docinfo/1801/.
5 Federal Flood Risk Management Standard.
8 Incidentally, Howard is winner of the 2018 Dooge Medal of the International Hydrological Prize.
References
- Archfield, S.A., et al., 2015. Accelerating advances in continental domain hydrologic modeling. Water Resources Research, 51 (12), 10078–10091. doi:10.1002/2015WR017498
- Bates, B.C., et al., eds., 2008. Climate change and water. Technical paper of the Intergovernmental Panel on Climate Change. Geneva: IPCC Secretariat, 210.
- Beven, K., et al., 2015. Hyperresolution information and hyperresolution ignorance in modelling the hydrology of the land surface. Science China, Earth Sciences, Special Topic: Watershed Science, 58 (1), 25–35. doi:10.1007/s11430-014-5003-4
- Beven, K. and Smith, P., 2015. Concepts of information content and likelihood in parameter calibration for hydrological simulation models. Journal of Hydrologic Engineering, 20 (1), SI, A4014010. doi:10.1061/(ASCE)HE.1943-5584.0000991
- Beven, K.J., 1986. Runoff production and flood frequency in catchments of order n: an alternative approach. In: V.K. Gupta, I. Rodriguez-Iturbe, and E.F. Wood, eds. Scale problems in hydrology. Dordrecht: Reidel, 107–131.
- Beven, K.J., 2011. I believe in climate change but how precautionary do we need to be in planning for the future? Hydrological Processes (Hptoday), 25, 1517–1520. doi:10.1002/hyp.7939
- Beven, K.J., 2018. On hypothesis testing in hydrology: why falsification of models is still a really good idea. WIREs Water, 5, e1278. doi:10.1002/wat2.1278
- Beven, K.J. and Alcock, R.E., 2012. Modelling everything everywhere: a new approach to decision-making for water management under uncertainty. Freshwater Biology, 57 (SI Supplement: 1), 124–132. doi:10.1111/j.1365-2427.2011.02592.x
- Boers, N., et al., 2014. Prediction of extreme floods in the eastern Central Andes based on a complex networks approach. Nature Communications, 5 (5199). doi:10.1038/ncomms6199
- Crutzen, P.J., 2002. Geology of mankind. Nature, 415 (6867), 23. doi:10.1038/415023a
- Dankers, R., et al., 2014. First look at changes in flood hazard in the inter-sectoral impact model intercomparison project ensemble. Proceedings of the National Academy of Sciences, 111, 3257–3261. doi:10.1073/pnas.1302078110
- Di Baldassarre, G., Brandimarte, L., and Beven, K., 2016. The seventh facet of uncertainty: wrong assumptions, unknowns and surprises in the dynamics of human–water systems. Hydrological Sciences Journal, 61 (9), 1748–1758. doi:10.1080/02626667.2015.1091460
- Dieperink, C., et al., 2016. Recurrent governance challenges in the implementation and alignment of flood risk management strategies: a review. Water Resources Management, 30 (13), 4467–4481. doi:10.1007/s11269-016-1491-7
- Döll, P., et al., 2015. Integrating risks of climate change into water management. Hydrological Sciences Journal, 60 (1), 4–13. doi:10.1080/02626667.2014.967250
- Dooge, J.C.I., 1973. Linear theory of hydrologic systems. Washington, DC: Agricultural Research Service, US Department of Agriculture, 327.
- Dooge, J.C.I., 1990. Research on the global earth system. Annals of the New York Academy of Sciences, 610, 45–46. doi:10.1111/j.1749-6632.1990.tb16932.x
- Dooge, J.C.I., 1992a. Sensitivity of runoff to climate change – a Hortonian approach. Bulletin of the American Meteorological Society, 73 (12), 2013–2024. doi:10.1175/1520-0477(1992)073<2013:SORTCC>2.0.CO;2
- Dooge, J.C.I., 1992b. Hydrologic models and climate change. Journal of Geophysical Research –Atmospheres, 97 (D3), 2677–2686. doi:10.1029/91JD02156
- Dooge, J.C.I., Kundzewicz, Z.W., and Napiorkowski, J.J., 1983. On backwater effects in linear diffusion flood routing. Hydrological Sciences Journal, 28 (3), 391–402. doi:10.1080/02626668309491978
- Driessen, P.P.J., et al., 2016. Toward more resilient flood risk governance. Ecology and Society, 21 (4), 53. doi:10.5751/ES-08921-210453
- Eagleson, P.S., 1986. The emergence of global-scale hydrology. Water Resources Research, 22 (9S), S6–S14. doi:10.1029/WR022i09Sp0006S
- Hegger, D.L.T., et al., 2016. Toward more flood resilience: is a diversification of flood risk management strategies the way forward? Ecology and Society, 21 (4), 52. doi:10.5751/ES-08854-210452
- Ismail-Zadeh, A.T., et al., 2017. Forging a paradigm shift in disaster science. Natural Hazards, 86, 969–988. doi:10.1007/s11069-016-2726-x
- Kauffeldt, A., et al., 2013. Disinformative data in large-scale hydrological modelling. Hydrology and Earth System Sciences, 17 (7), 2845–2857. doi:10.5194/hess-17-2845-2013
- Kleinen, T. and Petschel-Held, G., 2007. Integrated assessment of changes in flooding probabilities due to climate change. Climatic Change, 81 (3–4), 283–312. doi:10.1007/s10584-006-9159-6
- Klemeš, V., 1986. Dilettantism in hydrology: transition or destiny? Water Resources Research, 22 (9S), S177–S188. doi:10.1029/WR022i09Sp0177S
- Koutsoyiannis, D., et al., 2008. On the credibility of climate predictions. Hydrological Sciences Journal, 53 (4), 671–684. doi:10.1623/hysj.53.4.671
- Koutsoyiannis, D., et al., 2009. Climate, hydrology and freshwater: towards an interactive incorporation of hydrological experience into climate research. Discussion of “The implications of projected climate change for freshwater resources and their management” by Kundzewicz et al. (2008). Hydrological Sciences Journal, 54 (2), 394–405. doi:10.1623/hysj.54.2.394
- Koutsoyiannis, D., 2012. Clausius-Clapeyron equation and saturation vapour pressure: simple theory reconciled with practice. European Journal of Physics, 33 (2), 295–305. doi:10.1088/0143-0807/33/2/295
- Koutsoyiannis, D., 2014. Entropy: from thermodynamics to hydrology. Entropy, 16 (3), 1287–1314. doi:10.3390/e16031287
- Kreibich, H., et al., 2017. Adaptation to flood risk: results of international paired flood event studies. Earth’s Future, 5 (10), 953–965. doi:10.1002/2017EF000606
- Krysanova, V., et al., 2017. Intercomparison of regional-scale hydrological models and climate change impacts projected for 12 large river basins worldwide – a synthesis. Environmental Research Letters, 12, 105002. doi:10.1088/1748-9326/aa8359
- Krysanova, V., et al., 2018. How the performance of hydrological models relates to credibility of projections under climate change. Hydrological Sciences Journal, 63 (5), 696–720. doi:10.1080/02626667.2018.1446214
- Kundzewicz, Z.W., et al., 1987a. Mathematical modelling. In: Z.W. Kundzewicz, eds. Hydrology 2000. Wallingford: International Association of Hydrological Sciences, IAHS Publ. no. 171, 71–78.
- Kundzewicz, Z.W., et al., 1987b. Hydrological sciences in perspective. In: Z.W. Kundzewicz, eds. Hydrology 2000. Wallingford: International Association of Hydrological Sciences, IAHS Publ. no. 171, 1–7.
- Kundzewicz, Z.W., et al., 2008. The implications of projected climate change for freshwater resources and their management. Hydrological Sciences Journal, 53 (1), 3–10. doi:10.1623/hysj.53.1.3
- Kundzewicz, Z.W., et al., 2014. Flood risk and climate change: global and regional perspectives. Hydrological Sciences Journal, 59 (1), 1–28. doi:10.1080/02626667.2013.857411
- Kundzewicz, Z.W., et al., 2017a. Differences in flood hazard projections in Europe – their causes and consequences for decision making. Hydrological Sciences Journal, 62 (1), 1–14. doi:10.1080/02626667.2016.1241398
- Kundzewicz, Z.W., et al., 2018. Uncertainty in climate change impacts on water resources. Environmental Science & Policy, 79, 1–8. doi:10.1016/j.envsci.2017.10.008
- Kundzewicz, Z.W. and Dooge, J.C.I., 1985. Unified structural approach to linear flood routing. Advances in Water Resources, 8 (1), 37–43. doi:10.1016/0309-1708(85)90079-X
- Kundzewicz, Z.W. and Dooge, J.C.I., 1989. Attenuation and phase-shift in linear flood routing. Hydrological Sciences Journal, 34 (1), 21–40. doi:10.1080/02626668909491306
- Kundzewicz, Z.W. and Gerten, D., 2015. Grand challenges related to the assessment of climate change. Impacts on freshwater resources. Journal of Hydrologic Engineering, 20 (1), SI, A4014011. doi:10.1061/(ASCE)HE.1943-5584.0001012
- Kundzewicz, Z.W., Pińskwar, I., and Brakenridge, G.R., 2017b. Changes in river flood hazard in Europe: a review. Hydrology Research. doi:10.2166/nh.2017.016280
- Kundzewicz, Z.W. and Schellnhuber, H.-J., 2004. Floods in the IPCC TAR perspective. Natural Hazards, 31, 111–128. doi:10.1023/B:NHAZ.0000020257.09228.7b
- Kundzewicz, Z.W. and Stakhiv, E., 2010. Are climate models ‘ready for prime time’ in water resources management applications or is more research needed? Hydrological Sciences Journal, 55 (7), 1085–1089. doi:10.1080/02626667.2010.513211
- Kundzewicz, Z.W. and Takeuchi, K., 1999. Flood protection and management: quo vadimus? Hydrological Sciences Journal, 44 (3), 417–432. doi:10.1080/02626669909492237
- Lins, H.F. and Cohn, T.A., 2011. Stationarity: wanted dead or alive? Journal of the American Water Resources Association, 47, 475–480. doi:10.1111/j.1752-1688.2011.00542.x
- Matalas, N.C., 2012. Comment on the announced death of stationarity. Journal of Water Resources Planning and Management, 138, 311–312. doi:10.1061/(ASCE)WR.1943-5452.0000215
- Milly, P.C.D., et al., 2008. Stationarity is dead: whither water management? Science, 319, 573–574. doi:10.1126/science.1151915
- Milly, P.C.D., et al., 2015. On critiques of “Stationarity is dead: whither water management?”. Water Resources Research, 51, 7785–7789. doi:10.1002/2015WR017408
- Montanari, A., et al., 2013. “Panta Rhei – everything flows”: change in hydrology and society—the IAHS scientific decade 2013–2022. Hydrological Sciences Journal, 58, 1256–1275. doi:10.1080/02626667.2013.809088
- Montanari, A. and Koutsoyiannis, D., 2014. Modeling and mitigating natural hazards: stationarity is immortal! Water Resources Research, 50, 9748–9756. doi:10.1002/2014WR016092
- NRC, 2011. Global change and extreme hydrology: testing conventional wisdom. Washington, DC: The National Academies Press.
- NRC (National Research Council of the National Academies, USA), Committee on Challenges and Opportunities in the Hydrologic Sciences; Water Science and Technology Board; Division on Earth and Life Studies, 2012. Challenges and opportunities in the hydrologic sciences. Washington, DC: The National Academies Press, 200. ISBN 978-0-309-38701-9. doi:10.17226/13293
- Oki, T., Valeo, C., and Heal, K., eds., 2006. Hydrology 2020. An integrating science to meet world water challenges. Wallingford: International Association of Hydrological Sciences, IAHS Publication no. 300.
- Prudhomme, C., et al., 2013. Climate change and river flooding: part 2 sensitivity characterisation for British catchments and example vulnerability assessments. Climatic Change, 119 (3–4), 949–964. doi:10.1007/s10584-013-0726-3
- Prudhomme, C., et al., 2014. Hydrological droughts in the 21st century, hotspots and uncertainties from a global multimodel ensemble experiment. Proceedings of the National Academy of Sciences, 111 (9), 3262–3267. doi:10.1073/pnas.1222473110
- Roederer, J.G., 1985. Tearing down disciplinary barriers. Eos, Transactions American Geophysical Union, 66 (40), 681–685. doi:10.1029/EO066i040p00681
- Romanowicz, R.J., Dooge, J.C.I., and Kundzewicz, Z.W., 1988. Moments and cumulants of linearized St Venant equation. Advances in Water Resources, 11 (2), 92–100. doi:10.1016/0309-1708(88)90042-5
- Schewe, J., et al., 2014. Multimodel assessment of water scarcity under climate change. Proceedings of the National Academy of Sciences, 111 (9), 3245–3250. doi:10.1073/pnas.1222460110
- Schmidt, M. and Lipson, H., 2009. Distilling free-form natural laws from experimental data. Science, 324 (5923), 81–85. doi:10.1126/science.1165893
- Seibert, J. and McDonnell, J.J., 2015. Gauging the ungauged basin: relative value of soft and hard data. Journal of Hydrologic Engineering, 20 (1), SI, A4014004. doi:10.1061/(ASCE)HE.1943-5584.0000861
- Sikorska, A.E., Montanari, A., and Koutsoyiannis, D., 2015. Estimating the uncertainty of hydrological predictions through data-driven resampling techniques. Journal of Hydrologic Engineering, 20 (1), SI, A4014009. doi:10.1061/(ASCE)HE.1943-5584.0000926
- Sivakumar, B. and Singh, V.P., 2015. Special issue on grand challenges in hydrology. Introduction. Journal of Hydrologic Engineering, 20 (1), SI, A2014001. doi:10.1061/(ASCE)HE.1943-5584.0000983
- Sivapalan, M., et al., 2003. IAHS decade on predictions in ungauged basins, PUB. 2003–2012: shaping an exciting future for the hydrological sciences. Hydrological Sciences Journal, 48 (6), 857–880. doi:10.1623/hysj.48.6.857.51421
- Stanisławska, K., Kundzewicz, Z.W., and Krawiec, K., 2013. Hindcasting global temperature by evolutionary computation. Acta Geophysica, 61 (3), 732–751. doi:10.2478/s11600-012-0091-z
- Steffen, W., et al., 2015. Planetary boundaries: guiding human development on a changing planet. Science (Express, Online). doi:10.1126/science.1259855
- Szöllösi-Nagy, A., et al., 1987. Surface water hydrology. In: Z.W. Kundzewicz, eds. Hydrology 2000. Wallingford: International Association of Hydrological Sciences, IAHS Publ. no. 171, 9–15.
- Tyralis, H. and Koutsoyiannis, D., 2017. On the prediction of persistent processes using the output of deterministic models. Hydrological Sciences Journal, 62 (13), 2083–2102. doi:10.1080/02626667.2017.1361535
- Vetter, T., et al., 2017. Evaluation of sources of uncertainty in projected hydrological changes under climate change in 12 large-scale river basins. Climatic Change, 141, 419–433. doi:10.1007/s10584-016-1794-y
- Willner, S.N., et al., 2018. Adaptation required to preserve future high-end river flood risk at present levels. Science Advances, 4 (1), eaao1914. doi:10.1126/sciadv.aao1914
- Wyzga, B., et al., 2018. Comprehensive approach to the reduction of river flood risk: case study of the Upper Vistula Basin. Science of the Total Environment, 631–632, 1251–1267. doi:10.1016/j.scitotenv.2018.03.015
- Zalewski, M., 2015. Ecohydrology and hydrologic engineering: regulation of hydrology-biota interactions for sustainability. Journal of Hydrologic Engineering, 20 (1), SI, A4014012. doi:10.1061/(ASCE)HE.1943-5584.0000999