ABSTRACT
The 2012–2015 drought in north-central coastal California ranks among the three most prolonged periods of below-median annual rainfall in the past 65 years. In three critical coho salmon streams, summer baseflow was less each additional dry year; streams with summer flow early in the drought had no flow for more than two months in latter years. By the third dry year, summer discharge was 1–5% of recent wet-type years, and 10–20% of the first dry year. Multiannual drought also caused increased dry channel conditions: the percentage of flowing channel reduced from 28 to 55% from the first to the third dry years among three study streams. In the first year following drought, dry-season streamflow resembled early to-mid-drought conditions, while in the second, it approached pre-drought discharge. This multiannual drought foreshadows how multi-annual drought predicted under future climate scenarios may affect critical salmonid streams later this century.
Editor A. Castellarin Associate editor not assigned
Introduction
Drought is among the most fundamental features that define a hydrological regime. Drought describes water scarcity, categorized through meteorological, hydrological, agricultural, and socio-economic indices (Wilhite Citation2000, Mishra and Singh Citation2010) . Unlike aridity, which also describes water scarcity, drought represents an irregular period of low water abundance (Bruins and Berliner Citation1998), characterized by statistics such as indices of long-term records (e.g. the Standardized Precipitation Index) or statistics integrating surface soil water supply and demand (e.g. the Palmer Drought Severity Index; Mishra and Singh Citation2010, Ficklin et al. Citation2015). Though considered irregular, drought is often predictable and expected over the long term (Gasith and Resh Citation1999), and planning for drought has become an important consideration among water resource managers worldwide (Wilhite et al. Citation2005, Wilhite Citation2012).
Drought can have profound ecological effects. Periods of water scarcity define the lower limits of ecological processes and boundaries of stream ecosystem resilience (Boulton Citation2003). Irregular low rainfall influences the catchment processes that turn precipitation to streamflow, which controls the capacity for an ecosystem to re-organize and continue to provide its various processes and functions (Gasith and Resh Citation1999). In stream ecosystems, drought influences the most basic dynamics of the flow regime necessary for sustaining aquatic ecosystems: it can cause a reduction or elimination of flowing water, leading to loss of physical habitat and factors that maintain water quality (Lake Citation2003). During droughts, macro-invertebrate and fish communities often shift toward populations more capable of withstanding harsh conditions associated with drought, including decreased water quality and higher water temperatures (Covich et al. Citation2003, Beche et al. Citation2006, Magalhaes et al. Citation2007). Drought influences the growth and survival of organisms in terrestrial ecosystems as well (Wagner et al. Citation2012, Guttery et al. Citation2013, Ludewig et al. Citation2015). The 2012–2015 drought in California drew much attention from researchers and the media for its impacts to human development and to aquatic ecosystems alike. Following two years that were wetter than average in 2010 and 2011, the four years that followed each had below-average precipitation across the state; data from the US Drought Monitor indicate that almost half of the state was in “Exceptional Drought,” and almost all (92%) was in at least in Extreme or Severe Drought, through water year 2015 (NDMC et al. Citation2015). While the four consecutive years of drought in California are less than the recent droughts endured elsewhere in the world, e.g. Australia (Van Dijk et al. Citation2013), or the Middle East (Inbar and Bruins Citation2004), the recent California drought has forced cutbacks on water use for its 35 million people and much of its US$9 billion agricultural industry (Weiser Citation2014, Morin Citation2015); and state resource managers speculate that it may lead to the extirpation of some of the state’s most well-known aquatic organisms, such as the delta smelt and coho salmon (Flimrite Citation2014, Opar Citation2015, Reese and Kasler Citation2015). Throughout the fourth year of drought (2015) streamflow conditions were below normal among the majority of gauged streams in the state (TNC Citation2015), likely threatening stream habitat and connectivity that are important for salmonid survival (Carnie et al. Citation2015).
Much of the scientific research on the 2012–2015 California drought has focused on its severity and causes. In the southern part of the state (California Climate Divisions 4 through 6), the Palmer Drought Severity Index (PDSI) was the lowest in the full 120-year record (Vose et al. Citation2014). An expanded record of PDSI to a duration of 1200 years based on blue oak tree-ring data found that the 2012–2015 drought was the most severe over the 1200-year period (Griffin and Anchukaitis Citation2014, Robeson Citation2015). Though extreme in southern parts of California, PDSI-based drought was not the most severe statewide over the 120-year period of record (Williams et al. Citation2015) (PDSI does not consider impacts of reduced snowpack, which has implications for agricultural and hydrological drought; Mishra and Singh Citation2010). Each PDSI study implicates temperature as a cause of the particularly extreme drought conditions over 2012–2015. Also statewide, reconstruction of rainfall records indicates that the 2012–2014 drought was severe but not unprecedented, occurring three times in the past 440 years (Diaz and Wahl Citation2015). At a site level, studies in Walker Lake, east of the Sierra Nevada range, found the 2012–2015 drought to be more severe than Medieval Climate Anomaly droughts, though not as long-lasting (Hatchett et al. Citation2015).
Research has also investigated the relationship between climatic variability and human drivers of climate change on influencing the 2012–2015 California drought. The absence of precipitation and increased warm temperatures that defined the 2012–2015 drought are more likely attributed to natural variability than human-induced climate change (Seager et al. Citation2015). However, human-caused warming has increased the likelihood of extreme droughts like the 2012–2015 event (Richman and Leslie Citation2015, Williams et al. Citation2015). The factors that produced the atmospheric conditions leading to the drought are more likely to occur under present conditions than in the absence of human emissions (Swain et al. Citation2014). Further, drier periods over recent decades have tended to occur coincident with warmer conditions, and climate model experiments have indicated that human activities have increased the probability that dry-type years are also warm (Diffenbaugh et al. Citation2015).
Despite the abundant body of literature evaluating the recent California drought, few studies have examined its hydrological or ecological consequences, or the recovery of streamflow during subsequent years with normal-type or wet-type rainfall. An evaluation of California discharge based on a constructed historical record indicated that the drought effects were mainly a result of precipitation variability, rather than general warming trends (Mao et al. Citation2015). The drought has caused stress to Central Coast ecosystems, including forests (Asner et al. Citation2016) and grasslands (Potter Citation2015), and led to a rare algae bloom in the San Francisco Bay Delta (Gilbert et al. Citation2014). Such efforts to characterize the range and impacts of drought on flow regime, coupled with additional stressors such as increased extreme event frequency and higher temperatures, will be critical for predicting the effects of climate change on stream ecosystems and their implications for human development and human–ecosystem interactions in the 21st century (Diaz-Nieto and Wilby Citation2005, Palmer et al. Citation2008, Flint and Flint Citation2012). Recent droughts and future climate projections have raised new concerns among water resource managers and aquatic ecologists that the expected conditions in coming decades will cause changes great enough to alter ecosystem resilience and lead to alternate steady ecosystem states (Bond et al. Citation2008, Rahel and Olden Citation2008, Moritz and Agudo Citation2013) – and loss of ecosystem services that benefit human well-being (Breshears et al. Citation2011, Wu Citation2013, Matthews et al. Citation2014). These issues of climate change, flow regime, and ecosystem resilience are especially pertinent in places such as coastal California, where precipitation variability and drought are expected to become more common in the coming decades (Micheli et al. Citation2012) and stream ecosystems are prone to reach alternate steady states (Moyle Citation2014).
Drought also influences the quality of habitat that streams can provide for fish and other aquatic organisms. Declining water levels lead to restricted movement, lower food availability, and increased density (and thus increased competition), as well as increased physiological stress from lower water quality (Hakala and Hartman Citation2004, May and Lee Citation2004, Harvey et al. Citation2006, Stradmeyer et al. Citation2008, Irvine et al. Citation2009). In Mediterranean-climate coastal California, the prolonged summer dry season causes steadily declining flow; survival of organisms such as steelhead trout (Oncorhynchus mykiss) and coho salmon (O. kisutch) declines as pool habitat and dissolved oxygen recede through the dry season (Woelfle-Erskine et al. Citation2017). Over multiple years, years with lower dry-season baseflow by late in the dry season (September) have lower survival of juvenile salmonids than years with higher dry-season baseflow (Grantham et al. Citation2012). In coastal California, where humans and aquatic ecosystems already coexist precariously through the summer dry season (Deitch and Kondolf Citation2015), the relationship between drought conditions – especially multi-annual drought – and streamflow is poorly understood. This is especially the case for small, first- through third-order streams. Though such streams comprise the majority of the drainage network, most flow gauges in the region are on large streams regulated by dams. Concerns over the impacts of drought on streamflow are so great that the State Water Resources Control Board adopted an Emergency Drought Regulation for all water users along four streams in this region that have been identified as critical for endangered coho salmon: Green Valley, Dutch Bill, Mark West, and Mill Creeks (SWRCB Citation2015a); these regulations were not renewed in 2016 and 2017, when rainfall more closely resembled average conditions. The purpose of this research is to place the 2012–2015 drought conditions in coastal California into a broader context of multi-decadal rainfall records, and then illustrate the effects of this drought on streamflow and habitat in these four coastal catchments sequentially through each of the last six dry seasons, using streamflow records beginning in wetter-than-average years and extending through four years of persistent drought and then through two years of average or above-average rainfall following the 2012–2015 drought.
Study area and methods
Study area
Northern coastal California is a region of transition in North America, separating the characteristically wet northwestern part of the continent from the arid southwest. As a result, it shares the characteristics of both regions. It has wet winters and dry summers, with more than 90% of the annual precipitation falling in the wet half of the year. While much of the region receives annual rainfall similar to the Midwestern United States, the range of annual rainfall in coastal California over the past 50 years is approximately double (Deitch and Kondolf Citation2015). Because all of the precipitation in this region occurs as rainfall, streamflow follows similar patterns: more than 90% of the annual discharge occurs during the wet half of the year, and streams recede toward (and often reach) intermittence through summer and early fall until the rainy season begins again (Deitch et al. Citation2009a).
Coastal California is also a transitional region for stream ecosystems. The freshwater streams of California represent the southern extent of range for steelhead trout and coho salmon. Coho native to the region are listed as threatened or endangered from the Oregon border to approximately the Monterey Bay (south of which they are not present), and steelhead are listed as endangered in the southern part of the state and threatened elsewhere (NMFS Citation2012) (). Freshwater streams are a key part of the coho and steelhead life cycle. Adults migrate from the ocean to spawn in freshwater streams during the winter rainy season, and juveniles remain in streams through the summer dry season before migrating to the ocean as smolts (steelhead often remain in freshwater streams as juveniles for more than one summer dry season). Population numbers for both species (especially coho) have declined precipitously over the past three decades (Steiner Environmental Consulting Citation1996, NMFS Citation2012), resulting in aggressive efforts to enhance the population through broodstock programs and conservation hatcheries (Conrad et al. Citation2006, Obedzinski et al. Citation2009), and through habitat restoration (Christian-Smith and Merenlender Citation2010).
Figure 1. California climate regions and distribution of federally endangered salmonids (Central Coast coho to the north, Southern Coast steelhead to the south) and threatened salmonids (Southern Oregon/Northern California coho, Northern Coast steelhead, Central Coast steelhead, South-Central Coast steelhead) in Coastal California.
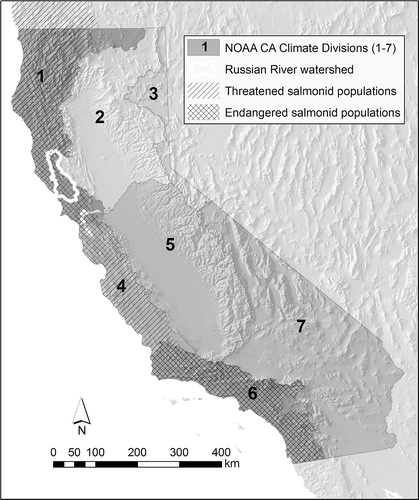
The Russian River of Sonoma and Mendocino Counties, located at the southern end of the North Coast California Climate Division (), is the largest catchment in the Central California Coast Evolutionarily Significant Unit for coho salmon (NMFS Citation2012). It represents typical habitat for coho and steelhead in this region; the Russian River is a sixth-order stream, but more than 70% of its USGS blue-line drainage network is first, second, or third order (Deitch and Kondolf Citation2015). These tributary streams represent the majority of the drainage network identified by NOAA Fisheries Division as critical habitat for federally protected salmonids (). Russian River tributaries such as Mill, Dutch Bill, Green Valley, and Mark West Creeks – rural streams that drain mountainous catchments ranging in area from 25 to 100 km2 – are identified as either Core or Tier 1 streams for the recovery of coho salmon listed in the NOAA Coho Salmon Recovery Plan (NMFS Citation2012). These streams are also often subject to surface water diversion by nearby landowners who use water for agricultural, recreational, and domestic purposes (Deitch et al. Citation2013, Citation2016); they have become focal watersheds for emergency regulatory actions to reduce the impacts of small diversions on oversummering juvenile salmonids (SWRCB Citation2015b). On an annual scale, human water needs in such watersheds comprise a small fraction of overall rainfall and discharge; but water demand through summer may equal or exceed the entirety of expected discharge from such streams during the summer dry season (Deitch et al. Citation2009b).
Methods
To characterize local rainfall patterns each year, we collected daily rainfall records from Global Historical Climatology Network (GHCN) weather stations near project streams in Sonoma County, California (available through the National Climatic Data Center at http://www.ncdc.noaa.gov/cdo-web/): Graton (station ID GHCND:USC00043578), located centrally in the Green Valley Creek catchment; and Healdsburg (station ID GHCND:USC00043875 through August 2012 and GHCND:US1CASN0051 through October 2017), located near the Mill Creek and Mark West Creek catchments. We calculated annual rainfall for water years 1951–2017 (October 1 to September 30), and graphed daily rainfall for both stations from 2011 to 2017 (one wet year followed by four dry years followed by two wet years) to identify trends in the occurrence of rainfall each year (e.g. whether rainfall occurred early, late, or evenly distributed through each rainy season). For an additional measure of drought intensity, we collected PDSI data for the NOAA California North Coast Drainage Climate Division over the available period of record.Footnote1
We installed water-level gauges (mostly in spring 2010, with a few later in 2011) in each drainage network and measured streamflow at regular intervals to develop flow records for each dry season from 2010 to 2017. Each gauge consists of a vented pressure transducer (in-situ LevelTROLL 500) encased in flexible PVC, affixed to the channel bank beside a staff plate gauge and set to record water level at 15-minute intervals. These pressure transducers were chosen for their accuracy (0.1% over the 0–11.5 ft [3.5 m] range, to an accuracy of 0.01 ft corresponds to 0.3 cm); staff plate records were used to detect and calibrate any drift by pressure transducers over the summer study period. Between May and October, streamflow was measured approximately monthly using Price Mini current meters following methods outlined by the US Geological Survey (Rantz Citation1982) and the California Department of Fish and Wildlife (CDFW Citation2013). Using the measured streamflow values, we created rating curves to correlate streamflow with stage and developed 15-minute streamflow records for each site. Mean daily flow data were plotted to examine trends in streamflow recession through the 2012–2015 drought, and then recovery through the following two summer seasons.
Gauges were installed to develop flow records at ecologically important locations in the drainage network (namely, at places that provide important salmon habitat). Streamflow records from these gauges are especially unique because most streamflow records from California are either from large rivers (mostly with reservoirs upstream), or associated with regulatory actions such as water quality-related Total Maximum Daily Load (TMDL) listings (especially in urban streams). Few data are available from these lightly developed, moderately inhabited streams which provide most of the critical habitat for salmonids, and long-term records characterizing drought events in past decades are nonexistent.
In addition to measuring streamflow at each flow gauge through the dry season, we walked much of each drainage network in late September 2012, 2013, 2014, 2015, and 2016 (reflecting four drought years and one normal-type year following) and recorded conditions in reaches through each drainage network as flowing, intermittent (with disconnected pools), or dry. Late summer represents a critical time for survival of salmonids in the region: based on observations in previous years, water levels typically recede (often past intermittency) through the dry season until late September, when they stabilize before autumn rains begin again (possibly a result of reduced evapotranspiration, a combination of shorter days and loss of deciduous tree foliage). Because low water levels also indicate poor habitat quality; we used these observations to indicate reaches in each drainage network where oversummer salmonid habitat is tolerable (wet/flowing), harmful (intermittent), or impossible (dry), and for documenting how the extent of habitat changes through a multi-year drought.
Results
Rainfall patterns and drought
Rainfall records over the past six decades demonstrate the variability of annual precipitation from one year to the next (). Recorded rainfall was below average (average is 1080 mm at Healdsburg and 1050 mm at Graton) frequently over the 64 years of record: at both sites, at least 25% of the years had less than 75% of average rainfall, and at least 15% of the years had less than two-thirds of the average. Two years had less than half the average.
Rainfall records suggest that the drought of 2012–2015 is neither the longest nor most severe drought in recent history (). The period 1987–1992 comprised six consecutive years with annual rainfall ranging between 60 and 80% of average; the 2012–2015 drought comprised four years with annual rainfall ranging from 55 to 90% of average. The drought of 1976/77 was more severe in terms of low annual rainfall (two consecutive years with less than half the average annual rainfall) but comprised only two years of below-average annual rainfall. Summer PDSI values indicate that the year during the 2012–2015 period when drought in the Northern Coastal California Climate Division was most severe represented the third-most severe drought year over the 120-year PDSI record (in contrast to Central and Southern California), with more severe drought occurring in 1924 and 1985 ().
Figure 4. June–July–August Palmer Drought Severity Index over the period 1895–2017 for the Northern Coastal California Climate Division and the average of Southern California Climate Divisions 4, 5, and 6.
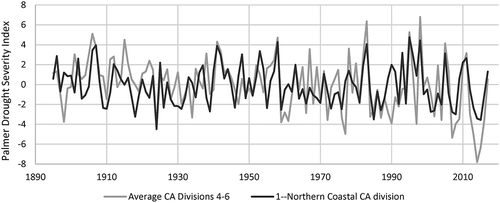
In the following years 2016 and 2017, rainfall approached and then exceeded average conditions. Rainfall in 2016 was slightly below average at Graton and at Healdsburg (92 and 98% of average, respectively); and in 2017 was among the highest 10% of annual rainfall over the 66-year period of record (). PDSI in the North California Coast region (as well as in Central and Southern California) increased in 2016 and again in 2017.
Precipitation records over the past eight rainy seasons show the variability in the volume and timing of rainfall within each year (). Rainfall in water years 2010 and 2011 (110 and 120% of average, respectively) occurred early in winter (November and December) as well as later in winter (February and March), with many days throughout the winter having rainfall greater than 25 mm. In the following drought years, most of the rainfall occurred in either early winter (2013, 2015) or late winter (2012, 2014). Rainfall in water years 2016 and 2017 resumed a pattern of early winter and late winter rainfall, though with greater magnitude and persisting later into the dry season in 2017. Measurable rainfall occurred during the month of June in 2011, 2013, 2016, and 2017, making up approximately 3–6% of the annual total; as well as mid–late September 2013, 2014, and 2015.
Dry-season streamflow
Streamflow records from each project stream illustrate the gradual recession toward intermittence each year (). The data also show a stark difference in discharge among pre-drought wet years and dry years. In wet years (2010, 2011), streams have greater baseflow and sustain discharge through the year; in dry years, they often do not. Rather, the flow records show a consistent pattern of less baseflow and earlier intermittence in each sequential dry year in three of the four streams. Two of the streams that sustained flow through 2011 and 2012 (the first year of below-average rainfall) became intermittent in late summer 2014 and 2015, resulting in at least one month of zero flow in each year. The outlier among these streams was Mark West Creek, which sustained flow through each of the drought years; its increase in flow in late August 2014 appears to have occurred as a result of the magnitude 6.0 earthquake that occurred on 23 August 2014 (though data before 23 August suggest flow would likely have persisted through 2014 in the absence of the earthquake). Daily discharge in 2015 was similar in each stream as in 2014, though was lower in Dutch Bill and Green Valley Creeks (near Graton, which received 700 mm of rainfall in 2015) and higher in Mill and Mark West Creeks (near Healdsburg, which received 920 mm of rainfall in 2015). Rainfall in mid-September 2015 caused flow to resume in three of the four streams, and flow was sustained through the rest of the year.
Figure 6. Daily discharge in Dutch Bill, Green Valley, Mill, and Mark West Creeks, June–October, 2010–2017. The Green Valley Creek gauge failed in late June 2012, but measured flow through summer 2012, showing an overall similar trend to other streams.
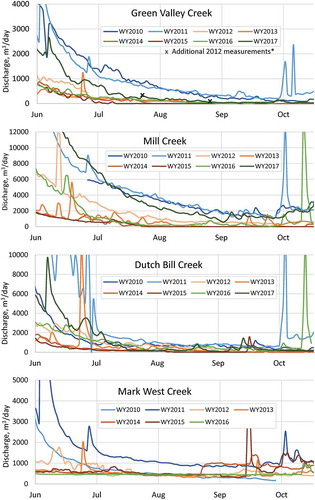
Streamflow records in summer 2016 and 2017 indicate a lagging effect of drought into years with average and above-average rainfall. Three of the four streams sustained discharge through summer 2016 (with Green Valley Creek becoming intermittent in mid-September 2016), though streamflow was at levels similar to those recorded in early- and mid-drought years (2012 and 2013). Streamflow in 2017 also resembled early- and mid-drought conditions in two of the streams (Green Valley and Dutch Bill Creeks): both streams maintained flow through the dry season, though flow in 2017 was much less than in pre-drought years. In Mill Creek, flow in 2017 was close to pre-drought (2011) conditions. Streamflow following drought in the anomalous Mark West Creek resembled conditions recorded in previous normal- and drought-type years (2011–2015; the Mark West Creek gauge failed in early summer 2017).
Monthly discharge data further illustrate the trend of less summer discharge in each subsequent dry year, and lagging recovery in the years following (). In three of the four streams, monthly discharge was progressively less with each subsequent drought year; discharge in August and September of the latter drought years 2013, 2014, and 2015 were commonly an order of magnitude less than discharge in the same months of the wetter years 2010 and 2011. In two of the streams, wet year discharge late in the year (e.g. September 2010 and 2011) was similar in magnitude to dry-year monthly discharge in June, early in the dry season. Averaged among three of the four catchments (not including Mark West Creek), monthly discharge in June 2014 and 2015 was less than 20% of the discharge in June 2010; July 2014 discharge was 15% of July 2010 (July 2015 was 10% of July 2010); August 2014 discharge was 4% of August 2010; September 2014 discharge was 1% of September 2010; and October 2014 discharge was 4% of October 2010. Discharge through the dry season in Mark West Creek was of similar magnitude in 2010 as in subsequent drought years, with more discharge in 2014 as a result of the August 2014 earthquake.
Figure 7. Monthly discharge through the dry season June–October, 2010–2017 in Dutch Bill, Green Valley, Mill, and Mark West Creeks (NA indicates monthly flow records were incomplete: streamflow in Mill Creek in June 2010 and Mark West Creek in October 2010 was not measured in its entirety; Green Valley Creek gauge failed in late June 2012).
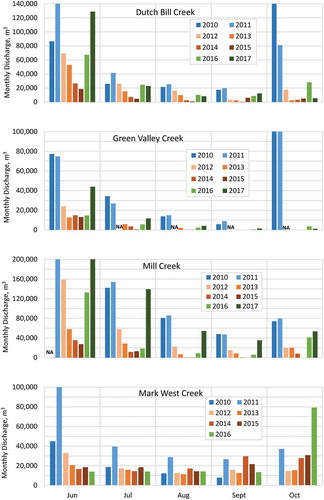
Monthly discharge through the dry seasons 2016 and 2017 illustrated the lagging effect of drought through years with average and above-average rainfall. Monthly discharge in 2016 was similar to early- and mid-drought years 2012 and 2013 in all four streams. In 2017, monthly discharge exceeded monthly discharge during each drought year, but remained less than pre-drought conditions.
Dry-season habitat
Documentation of dry-season habitat indicates a similar trend of progressively less suitable habitat conditions for salmonids with each subsequent dry year. In each of the three creeks, Mill, Green Valley, and Dutch Bill, the extent of documented flowing stream declined with each subsequent year. From 2012 to 2014, the fraction of surveyed stream with continuous surface flow in Mill Creek declined by more than 55% (). Similarly, the fraction of stream documented as flowing in Green Valley Creek and Dutch Bill Creek declined by 28 and 51%, respectively, from 2012 to 2014. In all three of these streams, the amount of channel classified as separated pools and dry channel increased from 2012 to 2014, migrating from alluvial fan reaches at downstream confluences (all of which were dry in September of each drought year) farther upstream into the mountain canyons with each additional drought year. In 2015, streams were not surveyed for surface connectivity until after rainfall on 16 September, which caused widespread re-connection of pools and resumption of flow in Mill Creek and Dutch Bill Creek that was absent prior to 16 September rainfall. The extent of suitable dry-season habitat in 2016 exceeded habitat conditions even in the first drought year, with larger amounts of stream identified as flowing in 2016, compared to 2012.
Discussion
The data presented in this study illustrate the impact of continued drought on streamflow in ecologically important coastal California streams, and recovery in the years following. Four years of drought resulted not only in below-average discharge; rather, each additional dry year resulted in less discharge through the dry season. Monthly discharge in the third dry year 2014 not only had less than 10% of the discharge of wet years; it had less than one-third the discharge of the first dry year 2012. This trend is not directly related to less annual rainfall each subsequent year: water year 2013 had more total rainfall than 2012, but 2013 monthly discharge was approximately half of the same months in 2012. Discharge in the first year following drought (2016) still was similar to early- and mid-drought discharge; but, by 2017, monthly discharge was generally greater than in drought years.
Rainfall and drought context
Long-term records from Healdsburg and Graton, CA, described above illustrate the extent of multi-annual rainfall variability from one year to the next. The drought of 2012–2015 (4 years, averaging 770 mm) represents one of three periods of below-average rainfall for four or more consecutive years: the others were 1959–1962 (4 years, averaging 850 mm) and 1987–1992 (6 years, averaging 740 mm). The difference between 2015 rainfall in Healdsburg and Graton (920 and 700 mm, respectively) underscores the spatial heterogeneity of rainfall in the area; Healdsburg received a large amount of rainfall in one particular event, causing local officials to proclaim it “far more than a 100-year storm” (with 150 mm, or 6 inches, of rain falling over 12 hours; Mason Citation2014). Based on summer PDSI data, drought in Northern Coastal California was more severe in 2014 and 2015 than any year during the 1959–1962 or 1987–1992 droughts. This may be a result of gradually warmer temperature in California over the past several decades (Swain Citation2015), thus increasing evapotranspiration and magnifying the severity of drought caused by precipitation. It may also be due to the spatial resolution of the NOAA PDSI data: the Northern Coastal California Climate Division covers an area approximately 15 times the area of the Russian River watershed, and rainfall can be highly variable over the region (Rantz and Thompson Citation1967). Higher-resolution PDSI data (e.g. Ficklin et al. Citation2015) may help to better understand the ecological consequences of drought.
Trends in discharge
The multi-annual trend of summer discharge in coho salmon streams through four years of drought illustrates the complexities of subsurface baseflow pathways. Water moves through the “cryptic reservoirs” of stream catchments over time scales that are difficult to predict without a thorough mechanistic understanding of underlying processes (McDonnell Citation2003, Sayama et al. Citation2011). If travel time (the time for rainfall that infiltrates into the subsurface to become surface streamflow) in meso-scale watersheds can be on the order of years – as stable isotope studies (e.g. Rodgers et al. Citation2005, Soulsby and Tetzlaff Citation2008) indicate – then the summer discharge in streams such as Mark West, Mill, Dutch Bill, and Green Valley Creeks includes water that fell as rain in previous years. Our results suggest that subsurface sources and pathways that provide summer baseflow over multi-annual scales in three of the four streams (Mill, Green Valley, and Dutch Bill Creeks) are not replenished in dry years as they are in wet years, resulting in persistently lagging baseflow with each subsequent dry year, similar to amplified effects over time documented in other regions with temperate climates (Eltahir and Yeh Citation1999, Peters et al. Citation2006).
This pattern of less baseflow each year occurs regardless of whether rainfall occurs early or late in the winter: for example, most of 2014 rainfall occurred later in the rainy season (February–April) than in 2013 (November–December), yet summer discharge was greater in 2013 than in summer 2014. This pattern also occurs regardless of rainfall magnitude: for example, measured rainfall was greater in 2013 than in 2012, but summer baseflow was much less in 2013. Hydrological studies in Australia during the Millennium drought found baseflow sources continuing to decline six years after the beginning of the drought, with effects persisting after normal precipitation returned (Leblanc et al. Citation2012). If subsurface pathways that provide baseflow to northern California streams have travel times on the order of years, then the lower-than-normal summer baseflow observed in 2016 and 2017 may have occurred because baseflow-producing sources would not yet have been replenished.
The anomalous Mark West Creek maintains comparatively steady baseflow through each drought year, and in the one year following (2016). This discrepancy relative to the other streams may be a result of a different surface geological formation over most of the Mark West watershed above our stream gauge, which can have a strong influence on baseflow variability as streams approach intermittence (Costigan et al. Citation2015). Unlike Mill, Dutch Bill and Green Valley creeks, which have surface geology made up mostly of Franciscan bedrock, the surface geology of Mark West Creek is mostly Sonoma Volcanic ash tuff (placed atop Franciscan bedrock approximately 3 MYA; Graymer et al. Citation2007). The ash tuff has substantially different geohydrological properties from Franciscan bedrock: whereas Franciscan formations are renowned for poor aquifer material (often considered aquicludes; e.g. Su et al. Citation2007), Sonoma Volcanic ash tuff is suitable aquifer material for many local water uses (Kleinfelder Citation2004). Franciscan geological formations are often characterized as having many bedrock fractures, which convey most of the subsurface water to streams (hydraulic conductivity in Franciscan bedrock is otherwise very low); whereas water has been observed percolating out of ash tuff bedrock along Mark West Creek even in July 2013 (CEMAR Citation2014).
Observations of habitat conditions through each drainage network help to complete the picture of drought impacts in each catchment. Though not documented formally, the authors in 2010 and 2011 observed persistent flow in all but the lowermost portion of each stream: though these alluvial fan reaches provide critical corridors for salmonid outmigration in winter and spring, they become dry in the dry season even in years with above-average rainfall, where surface flow infiltrates into coarse gravel. In each of our study streams, the extent of dry channel extends farther upstream with each additional dry year, and the total length of stream that sustains year-round flow in upper reaches steadily declines. Evaluations of habitat in 2016 (following drought) indicated flowing water and intermittent pools throughout each drainage network, as discharge more closely resembled early-drought conditions.
Less flow during the summer dry season leads to reduced survival of salmonids (Grantham et al. Citation2012), likely a result of two factors. First, juvenile salmonid survival tends to be higher in stream reaches with more habitat (in terms of pool size and volume) and lower in stream reaches with less habitat (May and Lee Citation2004). Reduced habitat resulting from declining streamflow can cause increased competition among fish populations for food and restrict movement within a stream reach (Stradmeyer et al. Citation2008, Irvine et al. Citation2009). These factors can become exacerbated once streams become intermittent: flow may reach a steady level (zero), but pool habitat continues to recede (Labbe and Fausch Citation2000). Second, declining flow leads to reduced water quality. Dissolved oxygen recedes as streamflow recedes, and it continues to decline after stream reaches become disconnected (Woelfle-Erskine et al. Citation2017), likely creating conditions that cause dissolved oxygen to fall below levels commonly considered as survival thresholds for salmonids (2–5 mg/L; Herrmann et al. Citation1962).
This desiccation of the drainage network may have profound impacts on survival of salmon and steelhead in coastal California during the period 2012–2015. Previous research in northern California and southern Oregon characterized varying conditions of flowing water and intermittence through a drainage network (May and Lee Citation2004, Hwan and Carlson Citation2016, Woelfle-Erskine et al. Citation2017); reaches where flow continues through the dry season provide refugia for organisms such as salmonids that are sensitive to habitat quality and water quality (Magoulick and Kobza Citation2003). Our habitat surveys indicate that the amount of drainage network identified as intermittent or dry increases with each additional year of drought, although these effects vary by stream: Green Valley Creek and Dutch Bill Creek have substantial reductions in habitat with surface flow after four years of drought, suggesting a major decline in refugia over the course of the four-year drought. In Mill Creek and Green Valley Creek, surface flow continues through the upper portions of the drainage network (where pools are frequently separated by bedrock in addition to gravel riffles); juvenile salmonids that oversummer in these upper reaches likely have a better chance for survival through the dry season than those in reaches downstream. Our assessments also indicate the resilience of stream habitat following drought: after one year of non-drought conditions (summer 2016), pools retained connectivity in late September through most of each drainage.
Ecological implications
Like many biota that live in Mediterranean-climate regions, salmonids such as steelhead and coho salmon have life history adaptations that allow them to survive through periods of drought (Moyle Citation2002). Steelhead trout are well known for these adaptations: they can tolerate higher temperature than other salmonids, they disperse over a greater area, and they have greater flexibility in their age when they return to freshwater streams to spawn (Shapovalov and Taft Citation1954, Pearse et al. Citation2009, Hayes et al. Citation2011). Coho salmon are not as flexible (Shapovalov and Taft Citation1954, p.575, Konecki et al. Citation1995), and their inability to adapt to drought may have played a role in tipping the coastal California population toward extirpation at the end of the 20th century. If the final two years of the 2012–2015 drought resulted in two years with prolonged periods of no flow through summer through large portions of the drainage network (making the likelihood of survival for oversummering juvenile coho salmon very low), the six-year drought from 1987 to 1992 likely had similar effects, but for two additional years. This would have placed severe stress on the overall coho salmon population: if no flow through multiple months equates to high juvenile coho mortality, then four consecutive years of such mortality may have caused a steep decline in the population. Extensive portions of the drainage network identified as having flowing water in 2016 (following drought) indicate better habitat conditions than most (if not all) of the drought; while this may not correlate to conditions that are “good” for fish (without knowing water quality dynamics or factors influencing food supply), it likely indicates conditions that are better than occurred when much of the drainage network was intermittent or dry.
Conclusion
In terms of annual rainfall, the drought of 2012–2015 represents one of the three most severe and persistent drought periods in the past 65 years, with annual rainfall ranging from 55 to 90% of the median for four consecutive years. During this period of below-average rainfall, streams carried less water with each additional summer; by the third drought year, streams that maintained flow in wetter-than-normal years ceased to flow for more than two months. Such persistent drought likely has severe impacts on fish such as steelhead trout and coho salmon, as each progressive drought year creates conditions that are increasingly harsh for survival. Recovery and the resumption of streamflow to resemble pre-drought conditions was not immediate, with streamflow resembling drought conditions the first year following drought and approaching pre-drought conditions in most streams after the second post-drought year.
If droughts such as the one from 2012 to 2015 become more common in the coming decades, maintaining salmonids in this region will be challenging. Adult salmonids typically spend up to three years in the ocean as adults before returning to spawn in natal streams; thus, a drought lasting four years can affect an entire population of salmonids in a region. Changing the practices of water management in the region (shifting away from summer diversions) may reduce some of the most acute human-caused pressures on salmonids and have the added benefit of improving water supply resilience, which will likely be important later this century if a megadrought occurs as predicted.
Acknowledgements
We thank K. Smetak, N. Reseburg, and G. Becker for assistance in field work, and M. A. King for a helpful review of an earlier manuscript. The authors also are thankful to two anonymous reviewers who provided useful reviews of earlier versions of this manuscript.
Disclosure statement
The authors have no interest or relationship, financial or otherwise, that might be perceived as influencing an author’s objectivity or any other conflict of interest.
Additional information
Funding
Notes
1 available through the National Climatic Data Center at http://www.ncdc.noaa.gov/ temp-and-precip/drought/historical-palmers/.
References
- Asner, G.P., et al., 2016. Progressive forest canopy water loss during the 2012–2015 California drought. Proceedings of the National Academy of Sciences, 113 (2), E249–E255. doi:10.1073/pnas.1523397113
- Beche, L.A., McElravy, E.P., and Resh, V.H., 2006. Long-term seasonal variation in the biological traits of benthic-macroinvertebrates in two Mediterranean-climate streams in California, USA. Freshwater Biology, 51 (1), 56–75. doi:10.1111/FWB.2006.51.issue-1
- Bond, N.R., Lake, P.S., and Arthington, A.H., 2008. The impacts of drought on freshwater ecosystems: an Australian perspective. Hydrobiologia, 600 (1), 3–16. doi:10.1007/s10750-008-9326-z
- Boulton, A.J., 2003. Parallels and contrasts in the effects of drought on stream macroinvertebrate assemblages. Freshwater Biology, 48, 1173–1185. doi:10.1046/j.1365-2427.2003.01084.x
- Breshears, D.D., López-Hoffman, L., and Graumlich, L.J., 2011. When ecosystem services crash: preparing for big, fast, patchy climate change. Ambio, 40 (3), 256–263.
- Bruins, H.J. and Berliner, P.R., 1998. Bioclimatic aridity, climatic variability, drought and desertification: definitions and management options. In: H.J. Bruins and H. Lithwick, eds. The arid frontier. The GeoJournal Library, vol 41. Dordrecht: Springer, 97–116.
- Carnie, R., et al., 2015. Habitat connectivity as a metric for aquatic microhabitat quality: application to Chinook salmon spawning habitat. Ecohydrology. doi:10.1002/eco.1696
- CDFW (California Department of Fish and Wildlife). 2013. Standard Operating Procedures for Discahrge Measurements in Wadeable Streams in Calfiornia. CDFW, Sacramento, CA.
- CEMAR (Center for Ecosystem Management and Restoration). 2014. Report on the Hydrologic Characteristics of Mark West Creek. CEMAR, Oakland, CA, 58 pp.
- Christian-Smith, J. and Merenlender, A.M., 2010. The disconnect between restoration goals and practices: A case study of watershed restoration in the Russian river basin, California. Restoration Ecology, 18 (1), 95–102. doi:10.1111/rec.2010.18.issue-1
- Conrad, J.L., et al. 2006. Annual Report for the Russian River Coho Salmon Captive Broodstock Program: Hatchery Operations and Monitoring Activities, July 2004 – June 2005. University of California Cooperative Extension Program. Santa Rosa, California.
- Costigan, K.H., et al., 2015. Understanding controls on flow permanence in intermittent rivers to aid ecological research: integrating meteorology, geology and land cover. Ecohydrology. doi:10.1002/eco.1712
- Covich, A.P., Crowl, T.A., and Scatena, F.N., 2003. Effects of extreme low flows on freshwater shrimps in a perennial tropical stream. Freshwater Biology, 48 (7), 1199–1206. doi:10.1046/j.1365-2427.2003.01093.x
- Deitch, M.J. and Kondolf, G.M., 2015. Salmon in a mediterranean climate: California’s incendiary mix. In: A. Lassiter, ed. Sustainable water: challenges and solutions from California. Berkeley, CA: University of California Press.
- Deitch, M.J., Kondolf, G.M., and Merenlender, A.M., 2009a. Hydrologic impacts of small-scale instream diversions for frost protection and heat protection in the California wine country. River Research and Applications, 25, 118–134. doi:10.1002/rra.1100
- Deitch, M.J., Kondolf, G.M., and Merenlender, A.M., 2009b. Surface water balance to evaluate the hydrological impacts of small instream diversions and application to the Russian river basin, California, USA. Aquatic Conservation: Marine and Freshwater Ecosystems, 19, 274–284. doi:10.1002/aqc.v19:3
- Deitch, M.J., Merenlender, A.M., and Feirer, S.T., 2013. Cumulative effects of small reservoirs on streamflow in northern Coastal California catchments. Water Resources Management, 27, 5101–5118. doi:10.1007/s11269-013-0455-4
- Deitch, M.J., Van Docto, M., and Feirer, S.T., 2016. A spatially explicit framework for assessing the effects of weather and water rights on streamflow. Applied Geography, 67, 14–26. doi:10.1016/j.apgeog.2015.11.018
- Diaz, H.F. and Wahl, E.R., 2015. Recent California water year precipitation deficits: A 440-year perspective. Journal of Climate, 28 (12), 4637–4652. doi:10.1175/JCLI-D-14-00774.1
- Diaz-Nieto, J. and Wilby, R.L., 2005. A comparison of statistical downscaling and climate change factor methods: impacts on low flows in the River Thames, United Kingdom. Climatic Change, 69 (2–3), 245–268. doi:10.1007/s10584-005-1157-6
- Diffenbaugh, N.S., Swain, D.L., and Touma, D., 2015. Anthropogenic warming has increased drought risk in California. Proceedings of the National Academy of Sciences, 112 (13), 3931–3936. doi:10.1073/pnas.142238511
- Eltahir, E.A.B. and Yeh, P.J.F., 1999. On the asymmetric response of aquifer water level to floods and droughts in Illinois. Water Resources Research, 35 (4), 1199–1217. doi:10.1029/1998WR900071
- Ficklin, D.L., et al., 2015. A climatic deconstruction of recent drought trends in the United States. Environmental Research Letters, 10 (4), 044009. doi:10.1088/1748-9326/10/4/044009
- Flimrite, P. 2014. California drought threatens coho salmon with extinction. San Francisco Chronicle, San Francisco, CA. http://www.sfgate.com/science/article/California-drought-threatens-coho-salmon-with-5175736.php
- Flint, L.E. and Flint, A.L. 2012. Simulation of climate change in San Francisco Bay basins, California: case studies in the Russian river valley and Santa Cruz mountains. Santa Rosa, CA: US Geological Survey Report No. 2012-5132.
- Gasith, A. and Resh, V.H., 1999. Streams in mediterranean climate regions: abiotic influences and biotic responses to predictable seasonal events. Annual Review of Ecology and Systematics, 30, 51–81. doi:10.1146/annurev.ecolsys.30.1.51
- Glibert, P.M., et al., 2014. Major–but rare–spring blooms in 2014 in San Francisco Bay Delta, California, a result of the long-term drought, increased residence time, and altered nutrient loads and forms. Journal of Experimental Marine Biology and Ecology, 460, 8–18. doi:10.1016/j.jembe.2014.06.001
- Grantham, T.E., et al., 2012. The role of streamflow and land use in limiting oversummer survival of Juvenile steelhead in California streams. Transactions of the American Fisheries Society, 141 (3), 585–598. doi:10.1080/00028487.2012.683472
- Graymer, R.W., et al. 2007. Geologic map and map database of eastern Sonoma and western Napa Counties, California. US Geological Survey Scientific Investigations Map 2956, 30 p., 1 sheet, 1:100,000 scale.
- Griffin, D. and Anchukaitis, K.J., 2014. How unusual is the 2012–2014 California drought? Geophysical Research Letters, 41 (24), 9017–9023. doi:10.1002/2014GL062433
- Guttery, M.R., et al., 2013. Effects of landscape-scale environmental variation on greater Sage-Grouse Chick Survival. PLoS ONE, 8 (6), e65582. doi:10.1371/journal.pone.0065582
- Hakala, J.P. and Hartman, K.J., 2004. Drought effect on stream morphology and brook trout (Salvelinus fontinalis) populations in forested headwater stream. Hydrobiologia, 515 (1–3), 203–213. doi:10.1023/B:HYDR.0000027330.12182.24
- Harvey, B.C., Nakamoto, R.J., and White, J.L., 2006. Reduced streamflow lowers dry-season growth of rainbow Trout in a small stream. Transactions of the American Fisheries Society, 135 (4), 998–1005. doi:10.1577/T05-233.1
- Hatchett, B.J., et al., 2015. Placing the 2012–2015 California-Nevada drought into a paleoclimatic context: insights from Walker Lake, California-Nevada, USA. Geophysical Research Letters, 42 (20), 8632–8640. doi:10.1002/2015GL065841
- Hayes, S.A., et al. 2011. Down, up, down and “smolting” twice? seasonal movement patterns by juvenile steelhead (Oncorhynchus mykiss) in a coastal watershed with a bar closing estuary. Canadian Journal of Fisheries and Aquatic Sciences, 68 (8), 1341–1350. doi:10.1139/f2011-062
- Herrmann, R.B., Warren, C.E., and Doudoroff, P., 1962. Influence of oxygen concentration on the growth of juvenile coho salmon. Transactions of the American Fisheries Society, 91 (2), 155–167. doi:10.1577/1548-8659(1962)91[155:IOOCOT]2.0.CO;2
- Hwan, J.L. and Carlson, S.M., 2016. Fragmentation of an intermittent stream during seasonal drought: intra-annual and interannual patterns and biological consequences. River Research and Applications, 32 (5), 856–870.
- Inbar, M.O.S.H.E. and Bruins, H.J., 2004. Environmental impact of multi-annual drought in the Jordan Kinneret watershed, Israel. Land Degradation & Development, 15 (3), 243–256. doi:10.1002/ldr.612
- Irvine, R.L., et al., 2009. The effects of flow reduction rates on fish stranding in British Columbia, Canada. River Research and Applications, 25 (4), 405–415. doi:10.1002/rra.v25:4
- Kleinfelder, Inc, 2004. Pilot study of groundwater conditions in the Joy Road, Mark West Springs, and Bennett valley areas of Sonoma County, California. Santa Rosa, CA: Sonoma County Permit Resource Management Department.
- Konecki, J.T., Woody, C.A., and Quinn, T.P., 1995. Temperature preference in two populations of juvenile coho salmon (Oncorhynchus kisutch). Env. Journal of Fish Biology, 44, 417–421. doi:10.1007/BF00008256
- Labbe, T.R. and Fausch, K.D., 2000. Dynamics of intermittent stream habitat regulate persistence of a threatened fish at muliple scales. Ecological Applications, 10 (6), 1774–1791. doi:10.1890/1051-0761(2000)010[1774:DOISHR]2.0.CO;2
- Lake, P.S., 2003. Ecological effects of perturbation by drought in flowing waters. Freshwater Biology, 48, 1161–1172. doi:10.1046/j.1365-2427.2003.01086.x
- Leblanc, M., et al., 2012. A review of historic and future hydrological changes in the Murray-darling basin. Global and Planetary Change, 80–81, 226–246. doi:10.1016/j.gloplacha.2011.10.012
- Ludewig, K., et al., 2015. Effects of reduced summer precipitation on productivity and forage quality of floodplain meadows at the Elbe and the Rhine River. PLoS ONE, 10 (5), e0124140. doi:10.1371/journal.pone.0124140
- Magalhaes, M.F., et al., 2007. Effects of multi-year droughts on fish assemblages of seasonally drying Mediterranean streams. Freshwater Biology, 52, 1494–1510. doi:10.1111/j.1365-2427.2007.01781.x
- Magoulick, D.D. and Kobza, R.M., 2003. The role of refugia for fishes during drought: a review and synthesis. Freshwater Biology, 48 (7), 1186–1198. doi:10.1046/j.1365-2427.2003.01089.x
- Mao, Y., Nijssen, B., and Lettenmaier, D.P., 2015. Is climate change implicated in the 2013–2014 California drought? A hydrologic perspective. Geophysical Research Letters,42, 2805–2813. doi:10.1002/2015GL06345
- Mason, C. 2014. Storm of the Century for Healdsburg. The Press Democrat, Santa Rosa, CA, http://www.pressdemocrat.com/news/3255021-181/storm-of-the-century-for?gallery=3483065&artslide=0
- Matthews, S.N., et al., 2014. Assessing and comparing risk to climate changes among forested locations: implications for ecosystem services. Landscape Ecology, 29 (2), 213–228. doi:10.1007/s10980-013-9965-y
- May, C.L. and Lee, D.C., 2004. The relationships among in-channel sediment storage, pool depth, and summer survival of Juvenile Salmonids in Oregon coast range streams. North American Journal of Fisheries Management, 24 (3), 761–774. doi:10.1577/M03-073.1
- McDonnell, J.J., 2003. Where does water go when it rains? Moving beyond the variable source area concept of rainfall-runoff response. Hydrological Processes, 17 (9), 1869–1875. doi:10.1002/(ISSN)1099-1085
- Micheli, E., et al., 2012. Downscaling future climate projections to the watershed scale: A north San Francisco Bay case study. San Francisco Estuary and Watershed Science, 10, 4. doi:10.15447/sfews
- Mishra, A.K. and Singh, V.P., 2010. A review of drought concepts. Journal of Hydrology, 391 (1), 202–216. doi:10.1016/j.jhydrol.2010.07.012
- Morin, M. 2015. Water agency approves farmers’ voluntary water reduction plan. Los Angeles Times, Los Angeles, CA, http://www.latimes.com/local/lanow/la-me-ln-water-rights-20150522-story.html
- Moritz, C. and Agudo, R., 2013. The future of species under climate change: resilience or decline? Science, 341 (6145), 504–508. doi:10.1126/science.1237190
- Moyle, P.B., 2002. Inland fishes of California. Berkeley: University of California Press.
- Moyle, P.B., 2014. Novel aquatic ecosystems: the new reality for streams in California and other Mediterranean climate regions. River Research and Applications, 30 (10), 1335–1344. doi:10.1002/rra.2709
- NDMC, USDA and NOAA (National Drought Mitigation Center, US Department of Agriculture and the National Oceanic and Atmospheric Administration). 2015. National drought monitor shapefiles, Year 2015. National Drought Mitigation Center. http://droughtmonitor.unl.edu/MapsAndData/GISData.aspx
- NMFS (National Marine Fisheries Service). 2012. Final recovery plan for central California coast coho salmon evolutionarily significant unit. National Marine Fisheries Service, Southwest Region, Santa Rosa, California.
- Obedzinski, M., et al. 2009. Russian River Coho Salmon Captive Broodstock Program Monitoring Activities: Annual Report, July 2007 to June 2008. University of California Cooperative Extension and Sea Grant Program. Santa Rosa, CA.
- Opar, A. 2015. Fish out of water: scary-low snowpack may spell disaster for some California wildlife and ecosystems. OnEarth, https://www.nrdc.org/onearth/fish-out-water
- Palmer, M.A., et al., 2008. Climate change and the world’s river basins: anticipating management options. Frontiers in Ecology and the Environment, 6, 81–89. doi:10.1890/060148
- Pearse, D.E., et al. 2009. Over the falls? Rapid evolution of ecotypic differentiation in steelhead/rainbow trout (Oncorhynchus mykiss). Journal of Heredity, 100 (5), 515–525. doi:10.1093/jhered/esp040
- Peters, E., et al., 2006. Propagation and spatial distribution of drought in a groundwater catchment. Journal of Hydrology, 321 (1), 257–275. doi:10.1016/j.jhydrol.2005.08.004
- Potter, C., 2015. Assessment of the immediate impacts of the 2013-2014 drought on ecosystems of the California central coast. Western North American Naturalist, 75 (2), 129–145. doi:10.3398/064.075.0202
- Rahel, F.J. and Olden, J.D., 2008. Assessing the effects of climate change on aquatic invasive species. Conservation Biology, 22 (3), 521–533. doi:10.1111/j.1523-1739.2008.00950.x
- Rantz, S.E. 1982. Measurement and computation of streamflow. Washington, DC: US Geological Survey, Water Supply Paper 2175.
- Rantz, S.E. and Thompson, T.H. 1967. Surface water hydrology of California coastal basins between San Francisco Bay and Eel River. Washington, DC: US Geological Survey Water-Supply Paper 1851.
- Reese, P. and Kasler, D. 2015. California water regulators approve massive cutback orders. Sacramento Bee, Sacramento, CA, http://www.sacbee.com/news/state/california/water-and-drought/article20277303.html
- Richman, M.B. and Leslie, L.M., 2015. Uniqueness and causes of the California drought. Procedia Computer Science, 61, 428–435. doi:10.1016/j.procs.2015.09.181
- Robeson, S.M., 2015. Revisiting the recent California drought as an extreme value. Geophysical Research Letters, 42 (16), 6771–6779. doi:10.1002/2015GL064593
- Rodgers, P.J., et al., 2005. Using stable isotope tracers to assess hydrological flow paths, residence times and landscape influences in a nested mesoscale catchment. Hydrology and Earth System Sciences, 9, 139–155. doi:10.5194/hess-9-139-2005
- Sayama, T., et al., 2011. How much water can a watershed store? Hydrological Processes, 25 (25), 3899–3908. doi:10.1002/hyp.v25.25
- Seager, R., et al., 2015. Causes of the 2011 to 2014 California drought. Journal of Climatology. doi:10.1175/JCLI-D-14-00860.1
- Shapovalov, L. and Taft, A.C., 1954. The life histories of the steelhead rainbow trout (Salmo gairdneri gairdneri) and silver salmon (Oncorhynchus kisutch): with special reference to Waddell Creek, California, and recommendations regarding their management. Sacramento, CA, USA: California Department of Fish and Game.
- Soulsby, C. and Tetzlaff, D., 2008. Towards simple approaches for mean residence time estimation in ungauged basins using tracers and soil distributions. Journal of Hydrology, 363 (1), 60–74. doi:10.1016/j.jhydrol.2008.10.001
- Steiner Environmental Consulting. 1996. A history of the salmonid decline in the Russian river. Steiner Environmental, Potter Valley, California
- Stradmeyer, L., et al., 2008. Competition between brown trout and Atlantic salmon parr over pool refuges during rapid dewatering. Journal of Fish Biology, 72 (4), 848–860. doi:10.1111/jfb.2008.72.issue-4
- Su, G.W., et al., 2007. Analysis of pumping-induced unsaturated regions beneath a perennial river. Water Resources Research, 43, 8. doi:10.1029/2006WR005389
- Swain, D.L., 2015. A tale of two California droughts: lessons amidst record warmth and dryness in a region of complex physical and human geography. Geophysical Research Letters, 42, 9999–10,003. doi:10.1002/2015GL066628
- Swain, D.L., et al., 2014. The extraordinary California drought of 2013–2014: character, context, and the role of climate change. Bulletin of the American Meteorological Society, 95 (7), S3–S7.
- SWRCB (State Water Resources Control Board), 2015a. Reolution No. 2015-0045 to adopt a drought-related emergency regulation requiring enhanced water conservation and additional water user information for the protection of specific fisheries in tributaries to the Russian River. California State Water Resources Control Board, Sacramento, CA, http://www.swrcb.ca.gov/board_decisions/adopted_orders/resolutions/2015/rs2015_0045.pdf
- SWRCB (State Water Resources Control Board) 2015b. Order WR 2015-0026-DWR for additional information in the matter of diversion of water from Dutch Bill Creek, Green Valley Creek, portions of Mark West Creek, and Mill Creek watersheds. California State Water Resources Control Board, Sacramento, CA, http://www.waterboards.ca.gov/waterrights/water_issues/programs/drought/docs/rrtribs/wr20150026_dwr_links.pdf
- TNC (The Nature Conservancy), 2015. California drought dashboard. The Nature Conservancy, San Francisco, CA, http://www.casalmon.org/disappearing-rivers
- Van Dijk, A.I., et al., 2013. The Millennium Drought in southeast Australia (2001–2009): natural and human causes and implications for water resources, ecosystems, economy, and society. Water Resources Research, 49 (2), 1040–1057. doi:10.1002/wrcr.20123
- Vose, R.S., et al., 2014. Improved historical temperature and precipitation time series for US climate divisions. Journal of Applied Meteorology and Climatology, 53 (5), 1232–1251. doi:10.1175/JAMC-D-13-0248.1
- Wagner, F., et al., 2012. Water availability is the main climate driver of neotropical tree growth. PLoS ONE, 7 (4), e34074. doi:10.1371/journal.pone.0034074
- Weiser, M. 2014. California needs more big storms to beat drought. Sacramento Bee, Sacramento, CA http://www.sacbee.com/news/state/california/water-and-drought/article.20686734.html
- Wilhite, D.A., 2000. Drought as a natural hazard: concepts and definitions. Drought, a Global Assessment, 1, 3–18.
- Wilhite, D.A. 2012. Drought Assessment, Management, and Planning: Theory and Case Studies: Theory and Case Studies. Springer Science & Business Media, Dec 6, 2012 293
- Wilhite, D.A., Hayes, M.J., and Knutson, C.L., 2005. Drought preparedness planning: building institutional capacity. In: D.A. Wilhite, ed. Drought and water crises: science, technology, and management issues. Boca Raton, FL: CRC Press, 93–135.
- Williams, A.P., et al., 2015. Contribution of anthropogenic warming to California drought during 2012–2014. Geophysical Research Letters, 42 (16), 6819–6828. doi:10.1002/2015GL064924
- Woelfle‐Erskine, C., Larsen, L.G., and Carlson, S.M., 2017. Abiotic habitat thresholds for salmonid over-summer survival in intermittent streams. Ecosphere, 8 (2). doi:10.1002/ecs2.1645
- Wu, J., 2013. Landscape sustainability science: ecosystem services and human well-being in changing landscapes. Landscape Ecology, 28 (6), 999–1023. doi:10.1007/s10980-013-9894-9