ABSTRACT
Srinivasan et al. provide an interesting overview of the challenges for long-term socio-hydrological predictions. Although agreeing with most of the statements made, we argue for the need to take socio-hydrological analysis a step further and add some fundamental considerations, especially concerning the crucial importance of many (conscious and unconscious) assumptions made upfront of the modelling exercise. Eventual assumptions of technological determinism need correction: Models are not “value-free”, but uncertain, subjective and a product of the society in which they were shaped. It is important to acknowledge this uncertainty and bias when making decisions based on socio-hydrological models, considering also that these models are “social and political actors” in and by themselves. Furthermore, socio-hydrological models require a transdisciplinary approach, since physical water availability is only one of the boundary conditions for society. Last but not least, interaction with stakeholders remains important to enable understanding of what the variable of interest is.
Editor A. Castellarin Associate editor not assigned
1 Introduction
The Panta Rhei Research Initiative (Montanari et al. Citation2013, McMillan et al. Citation2016) of the International Association of Hydrological Sciences (IAHS) triggered the debate on socio-hydrological modelling. As part of the Panta-Rhei Opinion paper series, Srinivasan et al. (Citation2017) provide an interesting overview of the challenges for long-term socio-hydrological predictions. The authors define three challenges: (i) current hydrological models do not (sufficiently) account for the interaction between society and the hydrological system, (ii) there is too little interaction between the model expert and the decision maker and stakeholders, and (iii) many long-term hydrological predictions do not account for global forces that influence local sources. The authors also provide suggestions to improve long-term predictions while accounting for socio-hydrological feedbacks. Although we agree with most of the statements made by Srinivasan et al. (Citation2017), especially the need for stakeholder involvement and looking at possibly differentiated distributive social-economic effects of socio-hydrological modelling (p. 340), we argue for the need to take socio-hydrological analysis a step further and add some fundamental considerations. This particularly relates to the many choices and (conscious and unconscious) assumptions that are made, for example by stakeholders, commissioners and modellers, before and during the modelling exercise: the naturalized discourses that are “materialized” in the model; the effect of biased selection of the stakeholders who can participate in the modelling process; and the social effects that sprout from the model’s results in terms of how these influence the “agenda setting” during policy discussions. The (socio-)hydrological model and modeller form part of, and contribute to, shaping a socio-natural network whereby humans (modellers, societal water interest groups) and non-humans (e.g. nature, water flows and hydrological models) mutually influence and depend on each other. Neither one of them can have “agency” and “act” without the other. Therefore, the socio-hydrological model itself becomes a political “actor” that is socially constructed and therefore not neutral in its effect on the socio-hydrological reality. These considerations ask for actions that foster comprehension of uncertainty, complexity and diversity; critical scrutiny of naturalizing conceptualizations; and transdisciplinary collaboration.
2 The role of models and modellers
Srinivasan et al. (Citation2017) state that the interaction between society and hydrology is too important to be ignored or assumed stable in a hydrological model, not only because society is part of the hydrological system but also because, in turn, the hydrological system is importantly influenced by the societal uses of the model. Hydrological model results can provide policy makers and other stakeholders with information to support decision making, while these decisions will again impact the hydrological system.
Because a “loading-dock” approach has been shown to be ineffective (Feldman and Ingram Citation2009), Srinivasan et al. (Citation2017) argue for an approach where stakeholders are involved in the modelling process (see Fig. 1). We agree with Srinivasan et al. that the model influences society, and that modellers should be aware of that: “If water resources modellers can shape social futures they must accept the responsibility that comes with it” (Srinivasan et al. Citation2017, p. 342). However, beyond this commonly accepted notion that “modellers implicitly model and mould society”, the relative influence of the different stakeholders and the presumed neutrality of the model itself and the role the model plays in policy discussions also need conscious attention and critical scrutiny. Embedded in the model are assumptions on what is important, and what not (e.g. water quantity and not water quality; water flow and not sediment transport; water productivity and not fairer distribution, etc.). Also modelling decisions on (temporal and spatial) scale, numerical methods, dealing with data scarcity and uncertainties are not neutral. They influence not only the model results, but also what the model can do, who can use the model, and for what purposes. Moreover, on a more abstract level, the generally held ideas and worldviews (“discourses”) of how the modellers (and policy makers, and other stakeholders) perceive certain hydrological phenomena, are not neutral. Problems and solutions, and hydro-social phenomena are defined in a particular way, with a particular language and based on particular scientific conventions. This fundamentally influences the model and the modelling results. For example, generally held notions such as “water use efficiency”, “water scarcity”, “groundwater recharge”, “rational water governance”, etc. include a particular perception of the world and direction for finding solutions (Boelens and Vos Citation2012).
Models are the result of assumptions and decisions by the modeller, which are inherently subjective. This subjectivity is more explicit and manifest (and, arguably, better “recognized”) when stakeholders are included in the modelling procedure, as suggested by Srinivasan et al., since stakeholders have an interest in the model results (hence their name “stakeholders”). provides a schematic overview of three ways to see the relationship between (socio-)hydrological models and society. The first approach is the “loading-dock” model, which presumes that the models are neutral instruments that help decision makers to make better decisions for society. The second part of the figure represents the idea of the socio-hydrological model, which is shaped by society through stakeholder participation in the development of the model, and informs decision making by policy makers. In this case the model itself is a neutral (or at least consensually established) tool that enhances decision making by policy makers. It provides explorations of future scenarios based on preferences of stakeholders.
Figure 1. Overview of three different approaches to the analysis of the relationship between hydrological models and society.
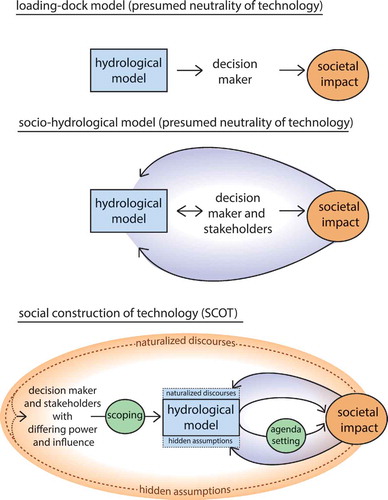
The third part of the figure represents the Social Construction of Technology (SCOT) approach (e.g. Pfaffenberger Citation1988, Bijker and Law Citation1992, Winner Citation1993, Bijker Citation2007). In this approach the (socio-)hydrological model is not a neutral tool: it has particular socio-economic effects because particular choices, knowledge, preferences, and “naturalized discourses” have “materialized” in the model. The specific characteristics and functioning of the model (even if it is explorative) make the model enabled and constrained to produce certain results (and not others). The (mostly unconsciously) biased model will steer the agenda for policy discussions, will subtly and invisibly legitimize certain policies and – often unintentionally or impalpably – will have specific environmental and distributive socio-economic effects. The model is shaped by the model makers themselves, the commissioners of the model and specific stakeholders with specific preferences, interests and values, who are represented in the participatory modelling process (excluding other, less visible and less “obvious” stakeholders, with other ideas and less dominant or less prevailing and common interests). Thus, models are “cultural, social and political constructions” and, in turn, have social consequences and impacts.
3 The social construction of (socio-)hydrological models
In this section we will further explore the relationship between hydrological models and society using the SCOT approach.
3.1 Scoping by model commissioners and stakeholder participation
When modellers take a certain assignment or define a certain objective of the model, their scoping encompasses a great deal of conscious and unconscious choices. Modellers might be inclined to make a model that satisfies the commissioner, and look for ways in which the outcomes of the models cater to the needs of the commissioner. An example is the groundwater model of the Mancha Oriental Aquifer in Spain that defined the area where farmers would get compensation for not using groundwater for one agricultural growing season. The objective of the Water Basin Authority (CHJ for its Spanish acronym) was to reduce groundwater use to the extent that water would still flow in the Jucar River (fed by the Mancha Oriental Aquifer) in a year with low water availability. CHJ calculated a maximum number of irrigated hectares that could be compensated (based on the funds available). CHJ then wanted the modellers to use the Mancha Oriental Aquifer model to determine the zone in which reduction of groundwater abstraction would be most beneficial for increasing river flows in the Jucar River. In other words, they wanted to maximize the effects of the compensation programme with the funds available. The modellers encountered high uncertainties and data scarcity, but in the end determined an area that contained a number of groundwater users that would fit the available budget of the basin organization (see Sanz et al. Citationforthcoming). The river basin authority and the groundwater users were pleased with the model, and in the dry years 2007 and 2008, with the groundwater users refraining from groundwater use, water still flowed in the Jucar River. The zone in which farmers were allowed to request compensation for not pumping was based on the model (in which the extension of the zone was informed by the available budget and not by hydrology per se); in fact, the model could not predict the actual flow of the river in relation to the use of groundwater. Actual “hydrological evidence” was considered less important to make the model work in this particular socio-political context. This illustration shows that uncertainties in the model become ignored in water policy practice and that modellers may commonly adjust to the needs of their commissioners. Many model makers agree that stakeholders should participate in model making (see e.g. Voinov et al. Citation2016, Castilla‐Rho 2017); however, not all stakeholders will have the same degree of participation or the same degree of acceptance of their ideas. Thus, the commissioners and a select number of stakeholders will influence the model (and consequently the model outcomes) more than others.
3.2 Constructing models
To make models, model makers have to take many decisions. These choices and (un)conscious assumptions influence the model and the model results. Due to the lack of a closed system (Oreskes et al. Citation1994), hydrological models are undeniably subject to model uncertainty. A major source of uncertainty is the model structure: uncertainty that stems from an incorrect or incomplete representation of the processes in the system, as demonstrated by many model intercomparison studies (e.g. Best et al. Citation2015). Different model structures, or slightly different parameter values, can lead to completely different model results (e.g. Beven and Binley Citation1992, Uhlenbrook et al. Citation1999, Liu and Gupta Citation2007, Addor et al. Citation2014, Chaney et al. Citation2015). Furthermore, uncertainty in hydrological observations propagates into the model (e.g. Westerberg et al. Citation2011). Coupling the hydrological system to the societal system perhaps increases the realism of the model, but also introduces new sources of uncertainty (Di Baldassarre et al. Citation2017, Westerberg et al. Citation2017). In the first place because society is under the influence of more factors than hydrology alone, but also because socio-hydrological observations are difficult to quantify and therefore prone to uncertainty. Accounting for structural, parameter and data uncertainty, which goes beyond the proposed sensitivity analysis through a future possibility space as described by Srinivasan et al. (Citation2017), implies that an almost infinite number of future possibility spaces exist, dependent on which model, parameterization or calibration dataset is employed. This is another societal space where subjectivity from the modeller is introduced: all these future possibility spaces can have contrasting shapes, and which future possibility space comes out of the model exercise is a concatenation of modelling decisions. Mendoza et al. (Citation2015), for example, showed that different modelling decisions in the model structure and calibration strategy lead to completely different results concerning the portrayal of climate change impact. A hydrological model is a hypothesis of how the system works: the term “hypothesis” already implies the subjectivity (Chamberlin Citation1980, Clark et al. Citation2011) that comes with constructing a hydrological model.
3.3 Naturalized discourses “materializing” in the models
A next level of scrutinizing models is to look at the values, knowledge and interests embedded in the model design. A model reflects the worldview (or discourses, or hypotheses) of the commissioners and makers of a model. Many notions that are taken as “natural” or “of general interest”, are actually particular views on the nature, relevance and importance of certain phenomena. Modellers, consciously, but mostly unconsciously, incorporate certain definitions, perceptions, values and interests into the models they make. An example is the Chicken Creek experiment (Holländer et al. Citation2009): 10 conceptually different models were compared for an artificially constructed basin (Chicken Creek) for which no discharge data were provided. None of the models were able to reproduce the water balance of the basin, because none of them accounted for the substantial erosion processes that occurred in the artificial basin. Personal judgement of the modeller on which processes to include in the model turned out to be key.
Here, we argue that it is crucial to examine critically the “naturalizing force” of (socio-)hydrological modelling, as if these human-invented models would represent “Nature”. Whatever the socio-hydrological model’s components, such models often become truths in themselves, as if they truly represent water’s socio-natural reality. Though being abstractions from reality, which conceptualize complexity by using (collectively accepted or paradigm-bound) conventions, they often conceal the model’s social and political construction. When models explaining socio-natural water behaviour are seen as “real” rather than attempts to “imagine the real”, their assumptions and conventions are hidden, unquestioned. This also holds true for exploratory models that help explore certain future scenarios (Bankes Citation1993, Castilla‐Rho 2017), as these models also necessarily select certain parameters that are explored and not others. Also in those models many choices and assumptions on the functioning of socio-natural systems are materialized, producing biased outcomes and steering the policy discussions in certain directions. An example is the model on the ecological flow of the Ebro in the north of Spain. This model was constructed to legitimize the transfer of about 800 million cubic metres of water per year to the agro-export region in the south of Spain. This model was developed in 2000 as part of the Spanish National Hydrological Plan. The model showed that more than sufficient water was available in average years to allow for the water transfer. However, Ibáñez and Prat (Citation2003) showed that the model overlooked a very important process: the sediment load of the Ebro. The sediment is essential for the unique ecosystem and rice production in the Ebro Delta. Large-scale storing and transfer of Ebro water would have severe effects on the sediment transport to the Ebro Delta. The hydrological model represented the values of the agro-export region, but not the ecological, cultural and economic values of the Ebro Delta.
3.4 Social effects of the use of (socio-)hydrological models
Hydrological and socio-hydrological models have social, economic and political effects, in the sense that they influence policy making and behaviour of actors. King and Kraemer (Citation1993) show that (economic) models can create “self-fulfilling prophesies” when actors react to predictions that make the predictions come true. An example from hydrological modelling could be the depletion of an aquifer that is accelerated because a model predicts the aquifer to be depleted in a certain time.
In general, the apparent scientific need to keep one’s distance, to be objective, and to avoid emotional contact with human subjects keeps most conventional scientific modelling research and policy formulation from sensing their actual hydro-social effects or imagining what might happen in hydro-social reality. By creating distanced views, hydrological models may neglect actual water users’ diversity and on-the-ground complexity of water flow, distribution and control regimes: they unwittingly tend to perceive and construct “equals” that fit into their models (Boelens Citation2009). This “commensuration” (that is, the expression and/or measurement according to one common metric, of elements, entities or features that in fact represent different values, meanings or units), is inherent to modelling (see also Espeland and Stevens Citation1998). Whenever the models are to be used, not just for socio-hydrological analysis and predictions, but also for policy decisions regarding water regime planning and future water distribution, management and governance patterning, their commensurate, non-personal properties and abstract reality easily lead to far-reaching decisions about people’s lives. As Anders (1980) observed, the larger the distance, the proportionally smaller our capacity to imagine, and the less our actions are restrained. Indeed, depersonalized water-planning and (socio-)hydrological models enable desensitized water development and disable approaches and strategies addressing the roots of water conflicts and scarcity problems (i.e. unequal distribution of water access and decision making; e.g. Boelens and Vos Citation2012, Rodríguez de Francisco and Boelens Citation2015). Still, hydrological models fundamentally are socio-natural constructs and power relations, especially when this is neglected or misrecognized.
Socio-hydrological modelling (and water policy making) are inherently social and scientifically and politically contested, and this concealment gives great power to model makers (intentionally or not). Naturalized, depoliticized models and approaches are closely equated with reality and truth, becoming “truth makers” (Boelens Citation2015, p. 93). In the example of the Mancha Oriental Aquifer in Spain, introduced above, the map produced by the model had clear economic effects: a particular group of farmers received subsidies (for not using water), while another group could not access this compensation programme. However, the effects were also political in that the CHJ and the modellers gained prestige and therewith authority. Also in the case of the transfer of the Ebro River in Spain it is clear that the ecological flow model has “truth claims” that could have had severe ecological and social consequences. By leaving out sediment transport processes from the model, the discussion on the controversial water transfer from north to south concentrated on the water quantities, which were apparently sufficient to allow for the water transfer: a clear example of “agenda setting” for a political discussion.
4 Actions
The discussed examples show that models are not objective tools. The model results are uncertain and subjective, and the uncertainty and subjectivity will likely increase when socio-hydrological feedbacks are included in the model. We propose three actions in addition to the suggestions described by Srinivasan et al. (Citation2017).
4.1 Comprehend uncertainty, inequality, diversity and complexity
To obtain valuable socio-hydrological models to support decision making and/or societal action and mobilization, we need to extensively describe and acknowledge the uncertainty in (socio-)hydrological models (Di Baldassarre et al. Citation2017, McMillan et al. Citation2017, Westerberg et al. Citation2017). McMillan et al. (Citation2017), for instance, provide an illustration demonstrating how uncertainty in streamflow observations had a strong economic impact for a hydropower company in Norway. Another example elaborated by the same authors shows that, when modellers were transparent about the uncertainty estimates of groundwater flow, this improved public acceptance of controversial policy. But uncertainty should be analysed not only from a technical point of view (data uncertainty, model structural uncertainty), but also from a sociological perspective. Due to the large uncertainty in (socio-)hydrological models, it is, in principle, almost always possible to create model results that correspond with the interests of the stakeholders or policy makers. Both consciously and unconsciously, this introduces subjectivity from the modeller and the stakeholders involved in the model construction. This will lead to a bias in the model results, and based on these biased results particular decisions will be made. Therefore, though simplifications are unavoidable, the choices made during the modelling process should be as explicit as possible to allow for critical public or open debate; they should remain open as much as possible for diversity of meanings and values of different stakeholders; and they need to take into account the complexities of socio-natural systems. A successful illustration to account for the diverse values of stakeholders is participatory modelling, as conducted by and discussed in, for instance, Rambaldi (Citation2010). Participation, however, should always be conducted with caution and a critical eye, as it can also lead to (and even strengthen) the illegitimate use of power – as scrutinized by Foucault’s studies (e.g. Foucault Citation1975, Citation2007, Citation2008) and discussed in Cooke and Kothari (Citation2001): the so-called “tyranny of participation”.
The concept of “hydrosocial territories” (Boelens et al. Citation2016) starts from the fundamental notion that stakeholders have diverse imagination, knowledge and power to materialize their wished-for socio-natural systems.Footnote1Hydrosocial territories are spatial configurations of people, institutions, water flows, hydraulic technology and the biophysical environment that revolve around the control of water. While diverse actors have divergent political-geographical interests, their territory-building projections and strategies compete, superimpose and align. In this process, the hydro-territorial imaginaries and assumptions of vulnerable stakeholders have less power to materialize. This implies that, in contexts of unequal power relationships, comprehending uncertainty, diversity and complexity in socio-hydrological modelling exercises asks for a particular “situated perspective” (Haraway Citation1991) so as to give due consideration and voice to subordinated interests and marginalized perspectives.
Therefore, although it is impossible to be transparent on all assumptions and aware of all naturalized discourses, we should at least strive to make important biases explicit and to include knowledge, visions, interests and values of marginalized groups. Overall, the social context in which (socio-)hydrological models are constructed is a field that needs far more exploration.
4.2 “De-naturalize”, review and discuss the “hidden” assumptions of models
Naturalizing socio-hydrological models are powerful when people put faith in them; models explaining physical and social water behaviour, beyond entreaties to “imagine the real”, become “real” like their built-in hidden assumptions and unquestioned conventions – a powerful resource for those benefiting from the imaginary model (e.g. water user elites, policy makers, or ourselves, the scientifically accredited socio-hydrologists). Transformed into water policies, power-laden choices and contents are concealed and naturalized, and models become instruments of “water truth production” (Boelens Citation2015).
Models draw attention to certain features while ignoring others, as partial constructions that supposedly represent totality. Whereas variability in the natural system is often conceptualized (Melsen et al. Citation2016a, Citation2016b), conventional modelling and water-policy making also ignore, precisely, human diversity, local water identities, governance forms, and the complexity of rights systems (Vos and Boelens Citation2014). Essentialization, uniformization and universalization equalize human actors and relationships, taking the dominant (mostly white, male, occidental, privileged class, and/or non-indigenous) as the referent (Boelens and Vos Citation2012). Therefore, modellers should be reflective. They should analyse their own conceptualizations, values and assumptions regarding the parameters and construction of the model. Rather than organizing themselves in epistemic communities of like-minded scholars, they and their models benefit from (also) arranging networks that allow for mutual and constructive doubts, critique, objections and antagonism (e.g. Arendt Citation1958, Mouffe Citation2005). This need to de-naturalize and discuss model assumptions through and within critical peer networks asks for complementation by broader reflection and action networks, leading to the following consideration.
4.3 Transdisciplinary dialogues and inclusive stakeholder representations
Constructive dialogues between stakeholders (modellers from different disciplines, policy makers, interest groups) should form part of model making and use. We agree with Srinivasan et al. (Citation2017) that hydrology impacts on society and vice versa, which is a rationale to include this interaction in socio-hydrological models. However, the way water and related benefits and risks are distributed among people depends not only on physical availability, but also on power structures, culture, economy and legislation. Water availability is different from having access to this water. Although a “model of everything” (Srinivasan et al. Citation2017) is indeed not feasible, co-operation among scientists from different disciplines is needed to understand these specific patterns and interactions. Even more important, to reach sustainable and equitable results, interactive socio-hydrological modelling requires the combination of grassroots, academic, activist and political action: engagement across differences (Zwarteveen and Boelens Citation2014).
Srinivasan et al. (Citation2017) also mention this interaction between stakeholders as a need to understand, negotiate and mediate the variable of interest. They provide an example where the stakeholders might be more interested in the fish in the river than the discharge of the river. Although this is clearly an example that needs an interdisciplinary approach (i.e. collaboration with aquatic ecologists), one should also take into account the related social relations: e.g. who values (what type) of fish, who are the fishers, and why do different stakeholders value the fish differently? This requires continuous transdisciplinary dialogue among all the relevant stakeholders. The case of the transfer of the Ebro River also shows the importance of including the less well off and less powerful stakeholders: in that case, the people living in the Ebro Delta, who have much less economic and political power than the agro-export sector in the south of Spain.
5 Conclusions
Models are not “value-free”, but subjective and a product of the society in which they are shaped, which leads to a bias in the model results. It is important to acknowledge this uncertainty and bias when making decisions based on socio-hydrological models, considering also that these models are “social and political actors” in and by themselves, steering hydrological and societal behaviour. Furthermore, socio-hydrological models require a transdisciplinary approach, since physical water availability is only one of the boundary conditions for society: understanding the (mostly uneven) forms of access to this water is equally important. Last but not least, interaction with stakeholders (ranging from water user groups, policy makers and water-development-affected communities to the model makers themselves) remains important to understand what the variable of interest is. Understanding the underlying reasoning of stakeholders requires a deep understanding of the diversity of local water cultures, forms of organizing water rights and governance, and how these are embedded in hydrosocial territories and influenced by societal power structures.
Disclosure statement
No potential conflict of interest was reported by the authors.
Notes
1 Boelens and colleagues conceptualize a “hydrosocial territory” as “the contested imaginary and socio-environmental materialization of a spatially bound multi-scalar network in which humans, water flows, ecological relations, hydraulic infrastructure, financial means, legal-administrative arrangements and cultural institutions and practices are interactively defined, aligned and mobilized through epistemological belief systems, political hierarchies and naturalizing discourses” (Boelens et al. Citation2016, p.2).
References
- Addor, N., et al., 2014. Robust changes and sources of uncertainty in the projected hydrological regimes of Swiss catchments. Water Resources Research, 50, 7541–7562. doi:10.1002/2014WR015549
- Anders, G., 1980 (1956). Die Antiquiertheit des Menschen. Part 1 and 2. Munich: Beck.
- Arendt, H., 1958. The human condition. Chicago: University of Chicago Press.
- Bankes, S., 1993. Exploratory modeling for policy analysis. Operations Research, 41 (3), 435–449. doi:10.1287/opre.41.3.435
- Best, M., et al., 2015. The plumbing of land surface models: benchmarking model performance. Journal of Hydrometeorology, 16, 1425–1442. doi:10.1175/JHM-D-14-0158.1
- Beven, K. and Binley, A., 1992. The future of distributed models: model calibration and uncertainty prediction. Hydrological Processes, 6, 279–298. doi:10.1002/(ISSN)1099-1085
- Bijker, W.E., 2007. Dams and dikes. Thick with politics. Focus-Isis, 98 (1), 109–123. doi:10.1086/512835
- Bijker, W.E. and Law, J., eds, 1992. Shaping technology/building society. Studies in sociotechnical change. Cambridge MA and London, UK: MIT Press.
- Boelens, R., 2009. The politics of disciplining water rights. Development and Change, 40 (2), 307–331. doi:10.1111/dech.2009.40.issue-2
- Boelens, R., 2015. Water, power and identity. The cultural politics of water in the Andes. London, UK: Earthscan, Routledge.
- Boelens, R., et al., 2016. Hydrosocial territories: a political ecology perspective. Water International, 41 (1), 1–14. doi:10.1080/02508060.2016.1134898
- Boelens, R. and Vos, J., 2012. The danger of naturalizing water policy concepts: water productivity and efficiency discourses from field irrigation to virtual water trade. Agricultural Water Management, 108, 16–26. doi:10.1016/j.agwat.2011.06.013
- Castilla-Rho, J.C., 2017. Groundwater modeling with stakeholders: finding the complexity that matters. Groundwater, 55 (5), 620–625. doi:10.1111/gwat.2017.55.issue-5
- Chamberlin, T., 1980. The method of multiple working hypotheses, Science (old series), 15. [accessed 2016 Nov 8]. Available from: http://www.jstor.org/stable/1716334?origin=JSTOR-pdf.
- Chaney, N., et al., 2015. Flood and drought hydrologic monitoring: the role of model parameter uncertainty. Hydrology and Earth System Sciences, 19, 3239–3251. doi:10.5194/hess-19-3239-2015
- Clark, M., Kavetski, D., and Fenicia, F., 2011. Pursuing the method of multiple working hypotheses for hydrological modeling. Water Resources Research, 47. doi:10.1029/2010WR009827
- Cooke, B. and Kothari, U., eds, 2001. Participation: the new tyranny? Chicago: University of Chicago Press.
- Di Baldassarre, G., Brandimarte, L., and Beven, K., 2017. The seventh facet of uncertainty: wrong assumptions, unknowns and surprises in the dynamics of human–water systems. Hydrological Sciences Journal, 61 (9), 1748–1758. doi:10.1080/02626667.2015.1091460
- Espeland, W. and Stevens, M.L., 1998. Commensuration as a social process. Annual Review of Sociology, 24, 313–343. doi:10.1146/annurev.soc.24.1.313
- Feldman, D. and Ingram, H., 2009. Making science useful to decision makers: climate forecasts,water management, and knowledge networks. Weather Climate and Society, 1, 9–21. doi:10.1175/2009WCAS1007.1
- Foucault, M., 1975. Discipline and punish. The birth of the prison. New York: Vintage Books.
- Foucault, M., 2007. Security, territory, population. New York: Picador.
- Foucault, M., 2008. The birth of biopolitics. New York: Palgrave Macmillan.
- Haraway, D., 1991. Simians, cyborgs and women: the reinvention of nature. London: Free Association Books.
- Holländer, H.M., et al., 2009. Comparative predictions of discharge from an artificial catchment (Chicken Creek) using sparse data. Hydrology and Earth System Sciences, 13, 2069–2094. doi:10.5194/hess-13-2069-2009
- Ibáñez, C. and Prat, N.S., 2003. The environmental impact of the Spanish national hydrological plan on the lower Ebro river and delta. International Journal of Water Resources Development, 19 (3), 485–500. doi:10.1080/0790062032000122934
- King, J.L. and Kraemer, K.L., 1993. Models, facts, and the policy process: the political ecology of estimated truth. Irvine: Center for Research on Information Technology and Organizations.
- Liu, Y. and Gupta, H.V., 2007. Uncertainty in hydrologic modeling: towards an integrated data assimilation framework. Water Resources Research, 43, W07401. doi:10.1029/2006WR005756
- McMillan, H., et al., 2017. How uncertainty analysis of streamflow data can reduce costs and promote robust decisions in water management applications. Water Resources Research, 53, 5220–5228. doi:10.1002/2016WR020328
- McMillan, H.K., et al., 2016. Panta Rhei 2013–2015: global perspectives on hydrology, society and change. Hydrological Sciences Journal, 61 (7), 1174–1191.
- Melsen, L., et al., 2016a. Representation of spatial and temporal variability in large-domain hydrological models: case study for a mesoscale pre-Alpine basin. Hydrology and Earth System Sciences, 20, 2207–2226. doi:10.5194/hess-20-2207-2016
- Melsen, L.A., et al., 2016b. HESS Opinions: the need for process-based evaluation of large-domain hyper-resolution models. Hydrology and Earth System Sciences, 20, 1069–1079. doi:10.5194/hess-20-1069-2016
- Mendoza, P., et al., 2015. How do hydrologic modeling decisions affect the portrayal of climate change impacts? Hydrological Processes, 30, 1071–1095. doi:10.1002/hyp.10684
- Montanari, A., et al. 2013. “Panta Rhei - everything flows”: change in hydrology and society - the IAHS scientific decade 2013-2022. Hydrological Sciences Journal, 58 (6), 1256–1275. doi:10.1080/02626667.2013.809088
- Mouffe, C., 2005. On the political. London, UK: Routledge.
- Oreskes, N., Shrader-Frechette, K., and Belitz, K., 1994. Verification, validation, and confirmation of numerical models in the Earth Sciences. Science, 263, 641–646. doi:10.1126/science.263.5147.641
- Pfaffenberger, B., 1988. Fetishised objects and humanised nature: towards an anthropology of technology. Manual (N.S.), 23, 236–252. doi:10.2307/2802804
- Rambaldi, G., 2010. Participatory three-dimensional modelling: guiding principles and applications, 2010 edition. Wageningen, the Netherlands: CTA.
- Rodríguez de Francisco, J.C. and Boelens, R., 2015. Payment for environmental services: mobilising an epistemic community to construct dominant policy. Environmental Politics, 24 (3), 481–500. doi:10.1080/09644016.2015.1014658
- Sanz, D., et al., forthcoming. The social construction and consequences of groundwater modelling: insights from the Mancha oriental aquifer, Spain. submitted to. International Journal of Water Resources Development.
- Srinivasan, V., et al., 2017. Prediction in a socio-hydrological world. Hydrological Sciences Journal, 62 (3), 338–345.
- Uhlenbrook, S., et al., 1999. Prediction uncertainty of conceptual rainfall-runoff models caused by problems in identifying model parameters and structure. Hydrological Sciences Journal, 44, 779–797. doi:10.1080/02626669909492273
- Voinov, A., et al., 2016. Modelling with stakeholders–next generation. Environmental Modelling And. Software, 77, 196–220. doi:10.1016/j.envsoft.2015.11.016
- Vos, J. and Boelens, R., 2014. Sustainability standards and the water question. Development and Change, 45 (2), 205–230. doi:10.1111/dech.12083
- Westerberg, I., et al., 2017. Perceptual models of uncertainty for socio-hydrological systems: a flood risk change example. Hydrological Sciences Journal, 62 (11), 1705–1713. doi:10.1080/02626667.2017.1356926
- Westerberg, I., et al., 2011. Stage-discharge uncertainty derived with a non-stationary rating curve in the Choluteca River, Honduras. Hydrological Processes, 25, 603–613. doi:10.1002/hyp.7848
- Winner, L., 1993. Upon opening the black box and finding it empty: social constructivism and the philosophy of technology. Science, Technology and Human Values, 18 (3), 362–378. doi:10.1177/016224399301800306
- Zwarteveen, M. and Boelens, R., 2014. Defining, researching and struggling for water justice: some conceptual building blocks for research and action. Water International, 39 (2), 143–158. doi:10.1080/02508060.2014.891168