ABSTRACT
The objective of this study was to examine the streamflow variability of Argentinean Andean basins (22°–52°S). Trends and step changes of seven hydrological variables were analysed. In addition, relationships between the hydrological variables and Pacific Decadal Oscillation (PDO), Niño 3.4, and Southern Annular Mode (SAM) indices were analysed. Most streamflow variables showed upward trends in the northwest and central-western basins, while downward trends were identified in the Patagonia (southwestern) region. Streamflow of the central-western and Patagonian basins was positively correlated with the Niño 3.4 index. Moreover, an inverse relationship with the SAM was found in watersheds south of 37°S. Positive step changes associated with the PDO phases in the north and central-western basins in the mid-1970s were detected, while negative step changes resulted in Patagonia between 1970 and 2000. This research provides new evidence of the influence of major climate modes on streamflow variability in the western rivers of Argentina.
Editor R. Woods Associate editor T. Kjeldsen
Introduction
The economic development of cities located on the eastern side of the Argentinean Andes is highly dependent on the availability of water resources. The increase in water demand for agricultural and industrial activities, hydropower production and drinking water supply involves better management. However, knowledge of the main hydrological patterns and factors affecting mountain hydrology conditions is still limited in many regions (Masiokas et al. 2013).
Mountain basins are the most vulnerable environments from the climate change point of view (Birsan et al. Citation2005). Thus, it is important to study streamflow variability and its links to regional climatic conditions and patterns of atmospheric circulation. In particular, on the western side of the Andes, in Chile, streamflow variability of rivers located between 29° and 40°S is associated with climate forcing, such as El Niño Southern Oscillation (ENSO) and Pacific Decadal Oscillation (PDO; Rubio-Álvarez and McPhee Citation2010, Cortés et al. Citation2011, Núñez et al. Citation2013). ENSO is the most important coupled ocean–atmosphere phenomenon to cause global climate variability on inter-annual time scales (Trenberth and Stepaniak Citation2001) and PDO was the main source of climate variability in the North Pacific during the 20th century (Mantua and Hare Citation2002).
In a similar way, streamflow variability and its association with the ocean–atmosphere circulation have been analysed in some western Andean rivers of Argentina. The South American Monsoon System is the main source of precipitation and the consequent discharge of subtropical rivers in northern Argentina (Ferrero et al. Citation2015). Streamflow variability in these basins is strongly linked to sea-surface temperatures (SST) recorded in the subtropical Atlantic Ocean (Flamenco and Villalba Citation2006). The annual, winter and spring flows of the Bermejo River showed an increasing trend (Pasquini and Depetris Citation2007) for the 1940–2003 period. In the context of increasing recurrence of extreme wet events (Ferrero et al. Citation2015), knowledge of the modes of streamflow variability is valuable in terms of reducing water-related environmental hazards.
The headwaters of the central-western Argentinean basins are located at high elevations in the Andes Mountains, where the streamflow is strongly related to winter snowfall (Masiokas et al. Citation2006). Over the 20th century, annual flows of the major rivers (San Juan, Los Patos and Atuel) showed decreasing streamflow trends (Boninsegna and Villalba Citation2006). However, between the mid-20th century and 2010, despite the negative trends in snow cover in the period 1979–2014 (Mernild et al. Citation2017), increasing trends and positive step changes have been noticed in the 1970s (Vich et al. Citation2007, Citation2014, Lauro et al. Citation2016).
Streamflow variability of the central Andes is strongly influenced by large-scale climate forcing, such as ENSO and PDO. The inter-annual variability of streamflow between 30°S and 40°S shows a relationship with the ENSO phases (Aceituno Citation1990, Compagnucci and Vargas Citation1998, Masiokas et al. Citation2006). The warm (cold) phase is associated with precipitation above (below) average and, consequently, the streamflow increase (decrease) in the summer period. This relationship is stronger north of 34°S (Gonzalez-Reyes et al. Citation2017). Furthermore, an extreme hydrological drought in the central Andes during the period 2010–2015 was driven by a cooling in the tropical Pacific Ocean that resembles La Niña conditions (Rivera et al. Citation2017a). During the warm (cold) phase of the PDO, winter snowpack and summer runoff are above (below) average (Masiokas et al. Citation2010, Gonzalez-Reyes et al. Citation2017, Rivera et al. Citation2017b). The multidecadal teleconnection mechanisms of the Pacific Ocean also influence streamflow variability in this region (Gonzalez-Reyes et al. Citation2017). Despite these studies, a gap in the knowledge about the current role of climate forcing and variability on seasonal streamflow exits for the central Andes region. In addition, a particular study for each Andean basin is lacking.
Most of the Patagonian rivers show negative streamflow trends during summer, as was the case for the Negro River for the 1927–2002 period (Pasquini and Depetris Citation2007). Annual and low flows of the Limay River had decreasing trends for the 1903–2004 period (Seoane and Lopez Citation2008). However, the Santa Cruz River, located in the extreme south of Patagonia, showed an increasing trend in the 1956–2009 period, contrasting with other rivers of the region (Pasquini and Depetris Citation2011).
The Southern Annular Mode (SAM; Marshall Citation2003) is the leading pattern of circulation variability in the Southern Hemisphere. In some cases, reductions in precipitation, associated with the SAM, have led to reductions in streamflow (Lara et al. Citation2007, Aravena and Luckman Citation2009). In fact, streamflow drought characteristics (duration and deficits) in Patagonia are consistent with decadal variations of the SAM to dry conditions (Rivera et al. Citation2017c).
However, the SAM is not the only climatic forcing affecting streamflow variability in Patagonia. The SST of the Tropical Pacific Ocean induces changes in the streamflow variability over the Neuquén, Negro, Limay, Chubut and Senguer rivers. These rivers play an important role in the region’s development, because the hydroelectric plants installed on them provide electricity to several parts of Argentina. For that, the role of inter-annual and decadal climate variability and their spatial patterns along the Andean rivers is an important issue.
Considering previous assessments as a baseline, a regional overview and an analysis of different flow variables (mean annual flow, annual maximum, annual minimum and seasonal flows) allows us to understand the changing patterns of the streamflow of the western river basins of Argentina. In this sense, the main objective of this paper is to identify and analyse streamflow variability and its relationship with climate indices in mountain basins of western Argentina between latitudes 22°S and 52°S. The results of this assessment are of paramount importance for the improvement of water resources management in the region.
Study area and basins
The study area includes mountainous basins located on the eastern slopes of the Argentinean Andes from the Bermejo River (22°S) to the Santa Cruz River (52°S). These basins can be gathered spatially into three main groups: basins located in the northwest of Argentina (NWA), those located in the central-west (CWA), also known as the Central Andes, and those located in the southwest of Argentina (SWA or Patagonia) ( and ).
Table 1. Main gauge station characteristics and list of analysed variables
The precipitation regime of the NWA basins, integrated by the Bermejo and Salí Dulce rivers, shows characteristics of monsoon behaviour, with wet summers and dry winters. The maximum precipitation records are from the months December–February (Minetti and Vargas Citation1997), while the hydrological regime is unimodal from September to August. The rainy summer season (January–March) concentrates 52–64% of the flow, whereas the dry winter season (July–September) provides only 1–10% of the flow (). The annual minimum flow is in October and the annual maximum flow is in February–March ().
The CWA basins correspond to the complex and extensive drainage system of the Colorado River (28°–37°S). This system is integrated by important rivers of the central-west region of Argentina, such as San Juan, Mendoza, Tunuyán, Diamante, Atuel, Grande and Colorado. The headwaters of this fluvial system are located in the Andes range in glacial and periglacial environments with elevations higher than 5000 m a.s.l. Snowfall is recorded during Southern Hemisphere winter (June–July–August) over the higher elevations of the Andes. Then, snow melting and glacial ablation feed the rivers during the summer season. Consequently, 66–76% of the flow discharge occurs during warmer seasons from October to March. The winter streamflow represents only 9–17% of the flow (), the hydrological regimen being unimodal from July to June. Hence, the annual minimum streamflow is recorded from June to August, and the annual maximum streamflow occurs from November to February ().
The Patagonian basins (SWA) extend approximately from 37° to 52°S. The northern basins (37°–45°S) are integrated by the Neuquén, Limay and Chubut rivers. Basins located between 42° and 44°S, which drain towards the Pacific Ocean, are the Manso, Futaleufú and Carrenleufú. The hydrological year of Patagonian rivers is from April to March, with a bimodal regime. The first peak occurs in June–July due to the winter precipitation, and the second is in October–November due to the snow melting and glacial ablation in the spring. As shown in , 57–86% of the runoff is recorded in the winter and spring seasons (July–December). The annual minimum flow is from March to May and the annual maximum flow occurs from June to September (). South of 50°S, the Santa Cruz River drains to the Atlantic Ocean, having a unimodal regime due to the seasonal melting of the Patagonian ice field. The hydrological year is from September to August. Seventy-one percent of the flow is concentrated in the summer–autumn season (January–June) (). The Santa Cruz River originates at the mouth of Lake Argentino and forms part of the hydrological network of the Perito Moreno Glacier and Lake Argentino (Pasquini and Depetris Citation2011). The glacier periodically dams a branch of Lake Argentino, resulting in an increase in water level. After a period of two or three years, the dam collapses, producing a water outburst that is buffered by Lake Argentino, and sometimes has a detectable signal from the Santa Cruz River (Pasquini and Depetris Citation2011).
Data screening and methods
Daily streamflow data of 79 gauge stations located in western Argentina (22°–52°S) were considered in a first data screening. Only reliable gauges (51) were used for the analysis. The selected stations have 30-year-long records with less than 20% of missing data (). In the particular case of the Santa Cruz River, monthly data were used because daily streamflow records were incomplete. Records were taken from the database of the Argentinean Subsecretaria de Recursos Hidricos.Footnote1
A brief exploratory data analysis demonstrates that streamflow records are characterized by the presence of outliers in the years of severe and moderate ENSO events. Only 25% of sites were normally distributed, random and independent, whereas 11% reject all the assumptions. Methods followed for these analyses are described in Vich et al. (Citation2014) and Lauro et al. (Citation2016).
Streamflow was analysed using seven variables: mean annual flow, annual maximum flow, annual minimum flow, summer flow (average of January, February and March), autumn flow (average of April, May and June), winter flow (average July, August and September) and spring flow (average October, November and December).
Several tests were applied to analyse the streamflow variability trend and step changes. In both cases the significance level of all the tests was 5%. If at least one of the tests rejects the null hypothesis, then the existing evidence is considered sufficient to accept the alternative hypothesis. For trend detection, the non-parametric Mann-Kendall test was used (Hirsch et al. Citation1982, Westmacott and Burn Citation1997), with two modifications for autocorrelated data (Hamed and Rao Citation1998, Yue and Wang Citation2002). The trend coefficient was estimated by an unbiased estimator proposed by Hirsch et al. (Citation1982).
For step change detection the non-parametric Taylor (Citation2000) test was applied. The test indicates only one step change, the most significant.
To determine the relationship between streamflow and climatic indices (ENSO, PDO and SAM), linear correlation analysis was performed. Mean annual, annual maximum, annual minimum and seasonal flows were correlated with annual climatic indices Niño 3.4, PDO and SAM using year-to-year correlations (). The Niño 3.4 index is based on the anomaly of the SST variations over the east-central tropical Pacific at 5°N–5°S, 170°–120°W (Trenberth Citation1997). The PDO is the leading principal component of North Pacific monthly SST variability (poleward of 20°N) (Mantua et al. Citation1997). The SAM index is defined as the zonal pressure difference between the latitudes of 40°S and 65°S (Marshall Citation2003). Data sources and the periods of analysis are shown in .
Table 2. Climatic indices used in correlation analysis
Results
Trend and step change detection
Of the analysed cases, 25% (292) presented significant trends, and among them 70% were positive and 30% were negative. An equal number of cases (292) were tested for step change analysis, resulting in 42% of these cases having significant step changes. Among them, 73% were positive step changes and 27% were negative.
Most of the positive trends were located north of the Colorado River (36°S), while negative trends were found in the SWA region. A similar distribution was found in the step change analysis; north of 37.1°S, mainly positive changes were found for the 1970s, while south of 37.1°S the step changes were most frequently negative. Apparently, one reason for this pattern is the variable length of record period in each gauge station. The trend and step changes detected are listed in .
Table 3. Trend and step changes for the seven variables analysed (Q). Significant trends are in bold and trend coefficient in m3/s year. For significant step changes, the direction and the year of change is shown. ns: non-significant. A: annual; max: maximum; min: minimum; SU: summer; AU: autumn; WI: winter; SP: spring
In the NWA basins, 53% of the cases presented a significant trend, all of them positive. Minimum flows depicted an increasing trend for all examined stations. Further to the rise in minimum flows, all the low-flow periods represented by the autumn and winter flows indicate an increasing pattern. The inter-annual patterns of three representative rivers of each region (NWA, CWA and SWA) are plotted in . Pozo Sarmiento station (PSA) is the representative station of the Bermejo River. The plot shows an increasing (annual, maximum and minimum) flow after the end of the 1970s.
Figure 3. Series of annual, maximum and minimum flows of the Bermejo (PSA), Mendoza (MEG) and Chubut (CEM) rivers. Significant trends and step changes are shown
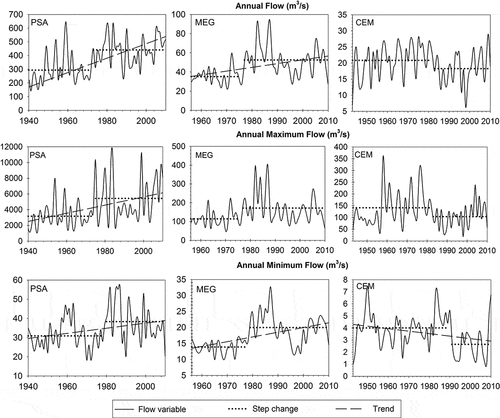
The streamflow of the CWA river basins – integrated by San Juan, Mendoza, Tunuyán, Diamante, Atuel, Grande and Colorado rivers – is highly dependent on the amount of snow accumulated during the winter season and the elevation of air temperature during the spring–summer season. A good number of stations showed an increasing trend in streamflow, most of them related to the low-flow variables.
Among 27 significant cases, 26% showed a positive trend in minimum flow, 22% in winter flow and 15% in autumn flow. These results suggest that the winter temperature is increasing; as a consequence of this warming, melting of snow and degradation of the permafrost could occur. This trend direction pattern is associated with the positive step changes of the 1970s, as shown in for the Mendoza River (MEG station). From a total of 49 significant step changes, 47 cases were positive. Of these, 21% correspond to winter flow, 19% to minimum flow and 17% to autumn flow. The two cases of negative trends were for winter and autumn flow, but in the 1950s. However, a change in the trend direction was not originated.
Despite the positive step changes detected for the 1970s, the Diamante and Pincheira rivers did not show significant trends. The Atuel River had increasing trends in annual, minimum and winter flow for the 1931–2011 period linked to step changes in the 1970s.
Streamflow in the Grande River basin (1971–2011) showed neither significant trends nor step changes. However, downstream at the Barrancas River (1960–2011) increasing trends were found in minimum, autumn and winter flow associated with positive changes in the 1970s. This pattern is mainly attributable to the different record lengths used.
Basins located in the SWA region showed a completely different behaviour, probably related to climate conditions. Significant trends constitute 16% of the cases, most of them being negative and related to low-flow variables. There were trends in minimum and summer flow in 52% of cases, followed by spring flow with 32%. In contrast, for maximum and winter flow, positive step changes were found. These results are coherent because the minimum flow in Patagonian rivers occurs during the summer season. However, the highest flows were observed for the winter season and were related to rainfall.
Furthermore, step changes were mostly negative in this region. Among the 39 significant cases, 42% correspond to minimum and summer flows. For summer flow most cases were in the 1970s, whereas those for minimum flow occurred in different decades. The positive step changes were more frequent for annual maximum flow in the 1990s. Again, these results are conditioned by record series lengths, which are shorter in Patagonian rivers than in the NWA and the CWA regions.
Inter-annual variations, significant trends and step changes in annual, maximum and minimum flows are shown in for the Chubut River (CEM station). Here, the inter-annual pattern of flow shows a decrease after the 1980s supported by the significant step changes. Although the methodology recognizes only one step change, it is possible to identify a positive break in 1957 in maximum flow, whereas a negative break in 1955 is observed in minimum flow.
The Santa Cruz River (1955–2011), the southernmost analysed river, showed an increasing trend in winter flow, which represents the lowest flow season. A positive step change was identified in the summer flow of 1975.
In spite of the different record lengths, a regional pattern of streamflow trends can be detected in the analysed series. Most of the increasing trends are located north of 40°S, while the decreasing trends are located towards the south. The trend direction of a common period (1961–2011) was evaluated to demonstrate this behaviour (). All the variables showed this pattern, except for the autumn and winter flows, which did not show significant trends in the Patagonian basins.
Figure 4. Significant trends for the period 1961–2010. + indicates positive trends and − negative trends. The trend coefficient in m3/year is shown
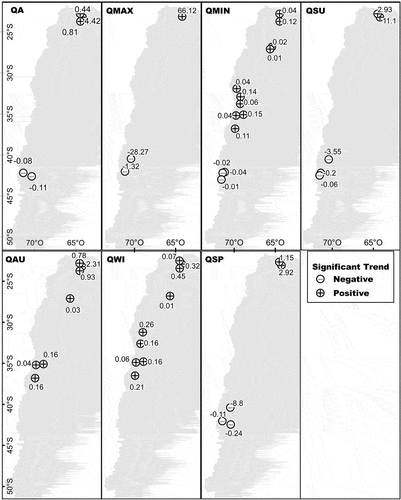
In the NWA and CWA basins, most of the step changes were detected during the 1970s, while for SWA basins those changes occurred during the 1980s and 1990s. This difference could be attributed to the shorter record length for Patagonia. The step change found in the 1970s has an important role in changing the direction of trend in the basins located north of 37°S.
Streamflow correlation with climate indices
The examination of changes in streamflow in relation to changes in global circulation patterns provides useful insights for the study region. The influence of climatic modes on the streamflow has a heterogeneous pattern in terms of the spatial distribution and strength of the correlations ( and ).
Figure 6. Correlations of summer (QSU), autumn (QAU), winter (QWI) and spring (QSP) flow with climate indices
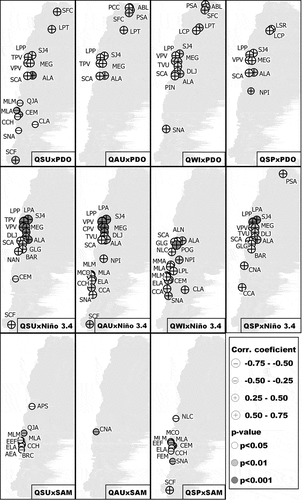
Correlation with PDO
The positive correlation between flow and the PDO indicates that the positive phase of the index is related to the increase of flows in the NWA and CWA regions. In the NWA rivers, annual and seasonal flows were positively correlated with the PDO. The correlation coefficients range from 0.27 (p < 0.05) to 0.41 (p < 0.01). In the CWA rivers, all the flow variables were positively correlated with the PDO, with correlation coefficients in the range 0.25 (p < 0.05) to 0.42 (p < 0.001; and ). Although the NWA and CWA rivers have different streamflow systems, both showed a relationship with the decadal variation of the Pacific Ocean (PDO). Moreover, the step changes during the 1970s in the streamflow are coincident with the cold-to-warm phase change of the PDO index in 1976/77.
Conversely, some Patagonian rivers showed negative correlations (p < 0.05 and p < 0.01), suggesting that the positive (negative) phase of the PDO is related to a decrease (increase) in summer flow (). In those rivers, a negative step change was also found in the 1970s. However, the Neuquén River had a positive correlation with both annual flow (0.22, p < 0.05) and spring flow (0.24, p < 0.05). The Senguer River showed a positive correlation with winter flow (0.28, p < 0.05; ), while the Santa Cruz River had a positive correlation with annual flow (0.31, p < 0.05) and summer flow (0.37, p < 0.01; and ).
Correlation with Niño 3.4 index
Only the spring flow of the Bermejo River was correlated with the Niño 3.4 index (0.29, p < 0.01; ). This finding indicates that, in this region, El Niño is not the most important climate mode related to streamflow variability.
All the streamflow variables of the CWA were positively correlated with the Niño 3.4 index, with correlation coefficients ranging from 0.29 (p < 0.05) to 0.49 (p < 0.001; and ). This suggests that, during the warm phase (El Niño) of the ENSO, the streamflow of this region increases, whereas the opposite occurs during the cold phase (La Niña). However, the winter and minimum flows did not show any significant relationship with the Niño 3.4 index for the San Juan and Mendoza river basins ( and ). This can be explained, because winter/minimum flows depend more on a thermal component than on rain and snowfall.
A small number of sites of the SWA showed correlations between the Niño 3.4 index and minimum, summer and spring flow. Instead, maximum, autumn and winter flows, which represent the highest amount of water in the basins, had stronger links with the Tropical Pacific Ocean SSTs, with correlation coefficients in the range 0.27 (p < 0.05) to 0.59 (p < 0.001). These findings suggest that increases in the equatorial SST cause an increase in streamflow in the SWA region.
Correlation with SAM
The significant correlations found between streamflow and the SAM index were negative, showing that during the positive (negative) phase of the index, streamflow is low (high) ( and ).
The CWA basins negatively correlated with the SAM index were those located south of 34°S. Maximum flow of the Grande River was negatively correlated with the SAM index (−0.34, p < 0.05) and summer flow of the Atuel River was also negatively correlated (−0.33, p < 0.05; and ).
In Patagonia, inverse relationships were found with spring flow (−0.36, p < 0.05) for the Neuquén River basin. In the Limay River basin, correlations were with minimum (−0.38, p < 0.05), summer (−0.44, p < 0.05) and autumn flows (−0.41, p < 0.05), whereas in the Chubut and Senguer river basins, correlations occurred in the annual, minimum and spring flows, with correlation coefficients in the range −0.29 to −0.39 (p < 0.05). Furthermore, the streamflow of the basins located in the Pacific drainage system were also negatively correlated with the SAM index. These correlations were with the annual, minimum, summer and spring flows (p < 0.05 and p < 0.01). In contrast, in the Santa Cruz River basin, a positive correlation with spring flow was detected (0.32, p < 0.05; and ). No river showed significant correlations between winter flows and the SAM index.
Discussion
Streamflow variability in terms of trend and step changes, and their relationships with oceanic and atmospheric indices, was analysed for the Argentinean Western rivers. A regional pattern can be distinguished from this trend analysis. Positive trends were found in basins located north of 35°S, while south of this latitude most of the trends were negative. A similar behaviour was found for the distribution of positive and negative step changes. This could be related to the regional influence of climate forcing. Consequently, sites located in the same climatic region will fluctuate in a similar way, albeit differing due to scale factors and sample randomness. The same regional pattern was revealed in precipitation trends, while temperature tended to increase in all the western Argentina basins (Núñez et al. Citation2008, SAyDS 2015).
We should note that most of the analysed basins have low human impact. Thus, non-stationarity can be due to fluctuations in temperature and precipitation, which are originated by variations in ocean–atmospheric circulation patterns.
NWA basins
The streamflow of the NWA basins showed positive trends generally associated with positive step changes in the 1970s. Therefore, the hydrological cycle is assumed to have intensified during the 1970s, as was reported by Castino et al. (Citation2016) for the period 1971–1977. The increase in flow could be associated with the increase in precipitation in the period 1960–2010 (Garreaud and Aceituno Citation2001, Celis et al. Citation2009, SAyDS 2015). It has been reported that the warm (cold) phase of ENSO results in a decline (increase) in autumn rainfall, and the opposite effect occurs with spring rainfall (Gonzalez et al. Citation2012). However, no relationship was found between the ENSO and the flow of the NWA basins, with the exception of the spring flow of the Bermejo River, which increases during the warm phase of ENSO, as reported for spring precipitation.
The SAM index is based on the zonal pressure difference between latitudes 40°S and 65°S (Marshall Citation2003). As such, it is the main mode of climatic variability in the mid- and high latitudes of the Southern Hemisphere. Therefore, it is difficult to explain its influence on the flow of the NWA region. In fact, no relationship between precipitation and temperature has been found in this region either (Gillett et al. Citation2006, Gonzalez et al. Citation2012).
The most important teleconnection found in this region was regarding the inter-decadal variability. The warm/cold phase of the PDO index is associated with annual and seasonal flow above/below the mean.
CWA basins
In the San Juan River basin, some river flows have increased over the last 60 years (1951–2011 period), matching previous research done on a shorter period of analysis (Vich et al. Citation2007). In addition, Celis et al. (Citation2009) and Poblete and Escudero (Citation2013) pointed out a decreasing trend in the Los Patos and San Juan rivers over the last 100 years.
The Mendoza River showed increasing trends in all the variables for the period 1956–2011. In contrast, Poblete and Escudero (Citation2013) found a non-significant decreasing trend in annual flow for the period 1909–2010. These findings highlight the importance of the dry period of 1956 in the trend analysis. From the beginning of the 20th century to 2010, the streamflow of the San Juan and Mendoza rivers has been decreasing. However, analysis of the second half of the 20th century revealed an increasing trend; this is probably related to the step change detected in the 1970s and the positive phase of PDO for the period 1977–1999.
The mean annual flow of the Atuel River basin increased in the period 1931–2011. In contrast, Boninsegna (Citation2009) did not find any kind of trend in the analysis of the last 100 years, despite the major retreat of glaciers reported in this basin (Le Quesne et al. Citation2009). Several researchers have reported an intense glacial retreat in the Central Andes in recent decades (e.g. Espizua Citation1986, Ferri Hidalgo et al. Citation2006, Leiva et al. Citation2007, Espizua and Pitte Citation2009). Similar to the streamflow increase found in our study, the annual temperature and precipitation in the period 1950–2010 were higher in the summer season (SAyDS 2015). In particular, the positive trend in minimum flows of the CWA region could be associated with the increase in the 0°C isotherm during the winter season (SAyDS 2015).
Changes detected in streamflows during the 1970s have also been noted in temperature and precipitation records (e.g. Compagnucci et al. Citation2002, Agosta and Compagnucci Citation2012, Agosta Citation2013). In agreement with the increase found previously in the river flow variables, growing trends in temperature and annual rainfall of the 1950–2010 period have been detected, being greater in both cases in the summer period (SAyDS 2015). Likewise, an increase in the snowpack was reported, but it was not statistically significant (Masiokas et al. Citation2006).
El Niño Southern Oscillation (ENSO) events seem to be an important source of runoff variability in the Cuyo region. The highest correlation coefficients were found between the Niño 3.4 index and summer streamflow, hence the increasing (decreasing) of Pacific Ocean SST will affect streamflow during the summer season. In fact, the highest correlation values are with the Niño 3.4 index of the previous winter (Carril et al. Citation1997, Compagnucci and Araneo Citation2007). This means that the warm phase of ENSO increases the precipitation of the previous winter, which increases the summer flow. This has been reported for the Central Andes of Argentina and Chile (Aceituno and Garreaud Citation1995, Compagnucci and Vargas Citation1998, Rubio-Álvarez and McPhee Citation2010). Another result to highlight is that the winter flow of the San Juan and Mendoza rivers does not correlate with the Niño 3.4 index, while this relationship is significant in the Atuel and Grande river basins. This suggests that the ENSO events in these basins, besides being related to the snowpack (Gonzalez-Reyes et al. Citation2017), have an effect on temperature.
Our study established significant correlations between the PDO and annual/seasonal flows, meaning that cold (warm) phases of the PDO coincide with decreasing (increasing) streamflow. In agreement with this finding, the precipitation and temperature, which have a direct relationship with the runoff in the central region of the Andes, also showed an increase associated with the climatic jump of the year 1976/77 (Jacques-Coper and Garreaud Citation2014).
The SAM is another source of variability in the summer flow of the Atuel River and the maximum flow of the Grande River. During the positive phase of the index, streamflow is below average, because of the shift in the Westerlies towards Antarctica; consequently, precipitation is below the mean (Romero et al. Citation2014).
SWA basins
Most of trends detected in northern and central Patagonia (37°–50°S) were negative, in spite of the different record lengths. Likewise, for the 1912–2002 period, negative trends in annual flow were reported in the rivers lying between 40° and 43°S, related to the increase in temperature and decreasing precipitation (Masiokas et al. Citation2008).
In addition, the decreasing trend in precipitation in northern and central Patagonia is associated with the positive phase of the SAM (Aravena and Luckman Citation2009), which generates a decrease in the low-pressure system at high latitudes. This feature intensifies westerly winds, and limits the propagation of low-pressure systems towards the north, reducing the amount of rainfall over the region. Moreover, the existence of a positive trend in the SAM index in the last three decades has been extensively documented, with strong westerly winds and warmer air temperatures in Patagonia (Garreaud Citation2009), features that have led to a decrease in rainfall across this region. As expected, our results show that streamflow has an inverse relationship with SAM in this region, supporting the role of SAM trends in the decrease in streamflow in the last 50 years.
In the Limay and Chubut rivers, step changes were negative in the 1970s and 1980s, while, at the end of the 1990s and at the beginning of 2000, positive step changes were detected. The same patterns are found for basins of the Pacific drainage system. The negative step changes found in the Limay River are coincident with a reduction in flow reported by Flamenco and Villalba (Citation2006), despite their using a different record length. Also, a reduction in precipitation in the 1970s was reported for the northwest of Patagonia (Castañeda and Gonzalez Citation2008). In the aforementioned basins, the reduction in summer flow is linked to the PDO; during the positive phase of the index, flows are below the mean.
In contrast to the results found for the northern and central Patagonian rivers, winter flow of the Santa Cruz River (1955–2011) showed a positive trend, which can be related to the increase in southern precipitation at 50°S (Garreaud et al. Citation2013), the temperature increase in western Argentina (SAyDS 2015), and the important glacial retreat in the Patagonian ice field (Masiokas et al. Citation2015, Falaschi et al. Citation2017). The Santa Cruz River annual and summer streamflows are directly correlated with the PDO index, which is also related to the positive step changes in the 1970s. Also, a positive relationship was found between the annual, summer and autumn flows and the Niño 3.4 index. Thus, during the positive phase of the PDO and warm ENSO events, greater flows can be expected.
Conclusions
The aim of the present study was to analyse the streamflow variability in the western Andean basins of Argentina between 22° and 52°S and its relationship with atmospheric circulation patterns. Most of the basins studied have a low anthropogenic impact, suggesting that the lack of stationarity may be caused by fluctuations in temperature and precipitation, which have their origin in variations in ocean–atmosphere circulation patterns. The availability fluctuations in the timing and spatial distribution of water resources due to changes in climatic conditions have been identified.
Step changes (39%) predominate over gradual changes (23%), and the variables that showed the greatest changes are those related to low flows, such as minimum flow, winter flow (in NWA and CWA) and summer flow (in SWA). It is possible to identify a spatial pattern of trends and step changes. Positive flow trends are identified in watersheds north of 35°S, whereas south of this latitude they are mostly negative. Positive step changes were found in the NWA and CWA regions in the 1970s, while in Patagonia, negative changes were detected between 1970 and 2000. These findings suggest that the influence of atmospheric circulation patterns is different throughout the western Argentine basins. The variability of the fluvial regime in the western basins of Argentina is associated with the inter-annual and inter-decadal fluctuations in SST. In the CWA and SWA regions, there is a direct relationship between streamflow and the Niño 3.4 index. Step changes are associated with PDO phase changes in the northern and central-west regions of Argentina. The positive phase of the PDO is related to an increase in the streamflow. The opposite outcome occurs in the summer flow of Patagonia, which decreases during the positive phase of the PDO. The SAM modulates streamflow variability south of 37°S due to its influence on precipitation. During the positive phase of the index, runoff results become lower. Knowledge of the influence of climate forcing on the spatial and temporal variability of streamflow is useful for water resources planning, with implications for policies on water consumption, energy and the agro-industry.
Since most of the observed changes in streamflow were not evenly distributed in the river basins of western Argentina, the adaptation response should be different for each region. In this regard, in the NWA region, policies should focus on flood mitigation due to the increase in streamflow and changes in land use. In the CWA region, attention should be paid to changes in the low-flow periods. Finally, in the SWA region, if the observed trend in flow persists, the management of water resources should focus on dry conditions.
Considering the current extreme drought conditions in western Argentinean river basins, future research should include efforts to understand the physical controls of low streamflow generation.
Acknowledgments
The authors would like to thank the anonymous reviewers for their thoughtful consideration of this manuscript, especially those who agreed to review a second version as well. We are thankful to the Subsecretaría de Recursos Hídricos de Argentina, for providing discharge time series. These results are part of the PhD thesis of Carolina Lauro.
Disclosure statement
No potential conflict of interest was reported by the authors.
Additional information
Funding
Notes
References
- Aceituno, P., 1990. Variabilidad interanual en el caudal de ríos andinos en Chile Central en relación con la temperatura de la superficie del mar en el Pacífico central. Revista de la Sociedad Chilena de Ingeniería Hidráulica, 5 (1), 7–19.
- Aceituno, P. and Garreaud, R., 1995. Impactos de los fenómenos El Niño y La Niña sobre regímenes fluviométricos andinos. Revista de la Sociedad Chilena de Ingeniería Hidráulica, 10 (2), 33–43.
- Agosta, E.A., 2013. The 18.6-year nodal tidal cycle and the bi-decadal precipitation oscillation over the plains to the east of subtropical Andes. South America. International Journal of Climatology, 34 (5), 1606–1614. doi:10.1002/joc.3787
- Agosta, E.A. and Compagnucci, R.H., 2012. Central-West Argentina summer precipitation variability and atmospheric teleconnections. American Meteorological Society, 25, 1657–1677.
- Aravena, J.C. and Luckman, B.H., 2009. Spatio-temporal rainfall patterns in southern South America. International Journal of Climatology, 29, 2106–2120.
- Birsan, M.V., et al., 2005. Streamflow trends in Switzerland. Journal of Hydrology, 314 (2005), 312–329.
- Boninsegna, J., 2009. Impacto del Cambio Climático en la región Centro Oeste de Argentina y posibles medidas de adaptación [online]. Foro: Escenarios climáticos y medidas de adaptación. Available from: https://www.inti.gob.ar/cirsoc/pdf/acciones_nieve_hielo/charlaimpactoshidrico.pdf [Accessed 15 July 2016].
- Boninsegna, J. and Villalba, R., 2006. Los condicionantes geográficos y climáticos. Documento marco sobre la oferta hídrica en los oasis de riego de Mendoza y San Juan [online]. Primer informe a la Secretaría de Ambiente y Desarrollo Sustentable de la Nación. Available from: http://inta.gob.ar/sites/default/files/script-tmp-inta_documento_marco_sobre_la_oferta_hdrica_en_los_oa_1.pdf [Accessed 15 July 2016].
- Carril, A., et al., 1997. Impacts of climate change on the oases of the Argentinean cordillera. Climate Research, 9, 121–129.
- Castañeda, M. and Gonzalez, M., 2008. Statistical analysis of the precipitation trends in the Patagonia region in southern South America. Atmósfera, 21 (3), 303–317.
- Castino, F., Bookhagen, B., and Strecker, M.R., 2016. River-discharge dynamics in the Southern Central Andes and the 1976–77 global climate shift. Geophysical Research Letters, 43 (11), 679–687. doi:10.1002/2016GL070868
- Celis, A., et al., 2009. CAPITULO 5: Cambio climático: variabilidad pasada y una prospectiva de las amenazas de acuerdo a los escenarios futuros. In: Centro Estudios Sociales, ed. Documento País: Riesgos de desastres en Argentina. Buenos Aires, 146–189.
- Compagnucci, R.H., Agosta, E.A., and Vargas, W.M., 2002. Climatic change and quasi-oscillations in central-west Argentina summer precipitation: main features and coherent behavior with southern African region. Climate Dynamics, 18, 421–435.
- Compagnucci, R.H. and Araneo, D., 2007. Alcances de El Niño como predictor del caudal de los ríos andinos argentinos. Ingeniería Hidráulica en México, 22 (3), 23–35.
- Compagnucci, R.H. and Vargas, W.M., 1998. Interannual variability of Cuyo rivers streamflow in Argentinean Andean mountains and ENSO events. International Journal of Climatology, 18, 1593–1609.
- Cortés, G., Vargas, X., and McPhee, J., 2011. Climatic sensivity of streamflow timing in the extratropical western Andes Cordillera. Journal of Hydrology, 405 (1–2), 93–109.
- Espizua, L.E., 1986. Fluctuations of the Rio del Plomo glaciers. Geografiska Annaler, 68 (4), 317–327.
- Espizua, L.E. and Pitte, P., 2009. The Little Ice Age glacier advance in the Central Andes (35°S), Argentina. Palaeogeography, Palaeoclimatology, Palaeoecology, 281 (3–4), 345–350.
- Falaschi, D., et al., 2017. Mass changes of alpine glaciers at the eastern margin of the northen and southern Patagonian icefields between 2000 and 2012. Journal of Glaciology, 63 (238), 258–272. doi:10.1017/jog.2016.136
- Ferrero, M.E., et al., 2015. Tree-ring based reconstruction of Río Bermejo streamflow in subtropical South America. Journal of Hydrology, 525 (2015), 572–584.
- Ferri Hidalgo, L., Espizua, L.E., and Pitte, P., 2006. Glacier fluctuations at Cerro Tupungato. In: PAGES, ed. International symposium: Reconstructing past regional climate variations in South America over the late Holocene: A new PAGES initiative, 4–7 October 2006. Malargüe, Argentina, 73.
- Flamenco, E.A. and Villalba, R., 2006. Relationships between sea surface temperatures and streamflows across the Cordillera de los Andes. In: Monferrer Ltda., ed. Proceedings of 8 ICSHMO, Foz do Iguaçu, 24–28 April 2006. Brazil: INPE, 1259–1263.
- Garreaud, R., et al., 2013. Large-scale control on the patagonian climate. Journal of Climate, 26 (1), 215–230.
- Garreaud, R.D., 2009. The Andes and weather. Advances in Geoscience, 22, 3–11.
- Garreaud, R.D. and Aceituno, P., 2001. Interannual rainfall variability over the South American Altiplano. American Meteorological Society. Journal of Climate, 14 (12), 2779–2789.
- Gillett, N.P., Kell, T.D., and Jones, P.D., 2006. Regional climate impacts of the Southern Annular Mode. Geophysical Research Letters, 33. doi:10.1029/2006gl027721
- Gonzalez, M.H., Cariaga, M.L., and Skansi, M., 2012. Some factors that influence seasonal precipitation in Argentinean Chaco. Advances in Meteorology, 2012 (359164), 13. doi:10.1155/2012/359164
- Gonzalez-Reyes, A., et al., 2017. Spatio-temporal variations in hydroclimate across the Mediterranean Andes (30°–37°S) since the early 20th century. Journal of Hydrometeorology, 18 (2017), 1929–1942.
- Hamed, K.H. and Rao, A.R., 1998. A modified Mann-Kendall trend test for autocorrelated data. Journal of Hydrology, 204 (1–4), 182–196.
- Hirsch, R., Snack, J., and Smith, R., 1982. Techniques of trend analysis for monthly water quality data. Water Resources Research, 18 (1), 107–121.
- Jacques-Coper, M. and Garreaud, R., 2014. Characterization of the 1970s climate shift in South America. International Journal of Climatology. doi:10.1002/joc.4120
- Lara, A., Villalba, R., and Urrutia, R., 2007. A 400-year tree-ring record of the Puelo River summer–fall streamflow in the Valdivian Rainforest eco-region, Chile. Climatic Change, 86, 331–356. doi:10.1007/s10584-007-9287-7
- Lauro, C., Vich, A.I.J., and Moreiras, S.M., 2016. Variabilidad del régimen fluvial en cuencas de la región de Cuyo. GeoActa, 40 (2), 28–51.
- Le Quesne, C., et al., 2009. Long-term glacier variations in the Central Andes of Argentina and Chile, inferred from historical records and tree-ring reconstructed precipitation. Palaeogeography, Palaeoclimatology, Palaeoecology, 281, 334–344.
- Leiva, J.C., Cabrera, G., and Lenzano, L., 2007. 20 years of mass balances on the Pilotoglacier, Las Cuevas River basin, Mendoza, Argentina. Global and Planetary Change, 59, 10–16.
- Mantua, N.J. and Hare, S.R., 2002. The Pacific decadal oscillation. Journal of Oceanography, 58, 35–44.
- Mantua, N.J., et al., 1997. A Pacific interdecadal climate oscillation with impacts on salmon production. Bulletin of the American Meteorological Society, 78, 1069–1079.
- Marshall, G.J., 2003. Trends in the southern annular mode from observations and reanalyses. Journal of Climate, 16, 4134–4143. doi:10.1175/1520-0442(2003)016<4134:Titsam>2.0.Co;2
- Masiokas, M.H., et al., 2015. Inventory and recent changes of small glaciers on the northeast margin of the southern Patagonia icefield, Argentina. Journal of Glaciology, 61 (227), 511–523. doi:10.3189/2015JoG14J094
- Masiokas, M.H., et al., 2008. 20th-century glacier recession and regional hydroclimatic changes in northwestern Patagonia. Global and Planetary Change, 60, 85–100. doi:10.1016/j.gloplacha.2006.07.031
- Masiokas, M.H., et al., 2006. Snowpack variations in the Central Andes of Argentina and Chile, 1951–2005: large-scale atmospheric influences and implications for water resources in the region. Journal of Climate, 19, 6334–6352.
- Masiokas, M.H., et al., 2010. Intra-to multidecadal variations of snowpack and streamflow records in the Andes of Chile and Argentina between 30 and 37°S. Journal of Hydrometeorology, 11, 822–831.
- Masiokas, M.H., et al., 2013. Recent and historic Andean snowpack and streamflow variations and vulnerability to water shortages in central-western Argentina. In: R.A. Pielke, ed. Climate vulnerability: understanding and addressing threats to essential resources. China: Elsevier, Academic Press, 213–227.
- Mernild, S.H., et al., 2017. The Andes Cordillera. Part I: snow distribution, properties, and trends (1979–2014). International Journal of Climatology, 37, 1680–1698. doi:10.1002/joc.4804
- Minetti, J. and Vargas, W., 1997. Trends and jumps in the annual precipitation in South America, south of the 15°S. Atmósfera, 11, 205–222.
- Núñez, J., et al., 2013. Influence of Pacific Ocean multidecadal variability on the distributional properties of hydrological variables in north-central Chile. Journal of Hydrology, 501, 227–240.
- Núñez, M.N., et al., 2008. Impact of land use and precipitation changes on surface temperature trends in Argentina. Journal of Geophysical Research, 113, 1–11. doi:10.1029/2007JD008638
- Pasquini, A.I. and Depetris, P.J., 2007. Discharge trends and flow dynamics of South American rivers draining the southern Atlantic seaboard: an overview. Journal of Hydrology, 333, 385–399.
- Pasquini, A.I. and Depetris, P.J., 2011. Southern Patagonia’s Perito Moreno Glacier, Lake Argentino, and Santa Cruz River hydrological system: an overview. Journal of Hydrology, 405, 48–56.
- Poblete, A.G. and Escudero, S.A., 2013. La sequia en los Andes Centrales y su repercusión en los ríos San Juan y Mendoza. In: E. Valpreda, E. Gabay, and Allessandro, eds. IV congreso nacional de geografía. XI Jornadas cuyanas de geografía, 23–26 Octubre 2013. Mendoza. 1–9.
- Rivera, J.A., Araneo, D.C., and Penalba, O.C., 2017b. Threshold level approach for streamflow droughts analysis in the Central Andes of Argentina: a climatological assessment. Hydrological Sciences Journal. doi:10.1080/02626667.2017.1367095
- Rivera, J.A., et al., 2017c. Regional aspects of streamflow droughts in the Andean rivers of Patagonia, Argentina. Links with large-scale climatic oscillations. Hydrology Research. doi:10.2166/nh.2017.207
- Rivera, J.A., et al., 2017a. Spatio-temporal patterns of the 2010–2015 extreme hydrological drought across the Central Andes, Argentina. Water, 9 (9), 652. doi:10.3390/w9090652
- Romero, P.E., Garbarini, E.M., and González, M.H., 2014. Características hídircas y climáticas del norte Patagónico. In: Tagliavini et al., eds. II Encuentro de investigadores en formación en Recursos Hídricos, 9–10 Octubre 2014. Ezeiza: INA, 95.
- Rubio-Álvarez, E. and McPhee, J., 2010. Patterns of spatial and temporal variability in streamflow records in south central Chile in the period 1952–2003. Water Resources Research, 46, W05514. doi:10.1029/2009WR007982
- Secretaría de Ambiente y Desarrollo Sustentable de la Nación (SAyDS), 2015. Tercera Comunicación Nacional de la República Argentina a la Convención Marco de las Naciones Unidas sobre Cambio Climático. Cambio climático en Argentina; tendencias y proyecciones. Buenos Aires: Secretaría de Ambiente y Desarrollo Sustentable de la Nación.
- Seoane, R. and Lopez, P., 2008. Assessing the effects of climate change on the hydrological regime of the Limay River basin. GeoJournal, 70, 251–256. doi:10.1007/s10708-008-9138-8
- Taylor, W., 2000. Change-point analysis: a powerful new tool for detecting changes. Avaiable from: http://www.variation.com/cpa/tech/changepoint.html [Accessed 15 March 2013].
- Trenberth, K.E., 1997. The definition of El Niño. Bulletin of the American Meteorological Society, 78, 2771–2777.
- Trenberth, K.E. and Stepaniak, D.P., 2001. Indices of El Niño evolution. Journal of Climate, 14, 1697–1701.
- Vich, A.I.J., López, P., and Schumacher, M., 2007. Trend detection in the water regime of the main rivers of the province of Mendoza, Argentina. Geojournal, 70, 233–243. doi:10.1007/s10708-0089136-x
- Vich, A.I.J., Norte, F., and Lauro, C., 2014. Análisis regional de frecuencias de caudales de ríos pertenecientes a cuencas con nacientes en la Cordillera de los Andes. Meteorológica, 39 (1), 3–26.
- Westmacott, J. and Burn, D., 1997. Climate change effects on the hydrologic regime within the Curchill Nelson river basin. Journal of Hydrology, 202, 263–279.
- Yue, S. and Wang, C.Y., 2002. The influence of serial correlation on the Mann-Whitney test for detecting a shift in median. Advances in Water Resources, 25, 325–333.