ABSTRACT
To guide scientists and society regarding the hydrologic consequences of anthropogenic climate change, earth scientists increasingly develop qualitative predictions and quantitative ensembles of models, some of which have important economic or (geo)political implications. However, with unprecedented human population, environmental degradation, and water scarcity, climatic factors are increasingly invoked falsely to explain failures of environmental governance, a phenomenon termed climatization. We propose a first typology of climatization in hydrology. Scientific climatization occurs when – during the normal course of a scientific investigation – a hydrologic state is falsely attributed to climatic factors, often due to a conceptual model that excludes human impacts or a simplified methodology that fails to quantify uncertainty. In contrast, securitization-aligned climatization occurs when a securitizing state actor requires that scientists attribute observed hydrologic states to climatic factors. Maintaining the credibility of earth science requires that earth scientists vigorously contest both scientific climatization and securitization of global change hydrology.
Editor S. Archfield ; Guest editor M. Sivapalan
1 Growing evidence of Earth’s finiteness
As Earth’s human population approaches eight billion people, signs of its limited carrying capacity proliferate (Warren Citation2015, Citation2016, Gotmark et al. Citation2018, Anderson Citation2019). With respect to water resources, four billion people face severe water scarcity (Mekonnen and Hoekstra Citation2016). This water scarcity in turn threatens human and consequently national security (El-Sayed and Mansour Citation2017) as well as peaceable relations amongst nations (Petersen-Perlman et al. Citation2017). When human or agricultural water requirements clash with those of natural systems, the latter often suffer (Dery and Salomon Citation1997, Vorosmarty et al. Citation2010), as demonstrated by increasingly widespread evidence of Tragedy of the Commons (Hardin Citation1968) in the water resources of Earth’s water-limited regions – manifested by decreasing terrestrial water storage (Müller et al. Citation2017, Rodell et al. Citation2018, Wang et al. Citation2018),Footnote1 river flows (Hillel et al. Citation2015, Citation2019, Tal Citation2017, Best Citation2019), and lake water storage (Micklin Citation1988, Citation2007, Aghakouchak et al. Citation2015, Moore Citation2016, Fazel et al. Citation2017, Wurtsbaugh et al. Citation2017, Rodell et al. Citation2018, Morin et al. Citation2018, Chaudhari et al. Citation2018, Khazaei et al. Citation2019, Wine et al. Citation2019b, Wine Citation2019e). Similar to water, land has also become increasingly scarce (Lambin and Meyfroidt Citation2011), which may in part explain the increasing population living in low-lying hazard-prone coastal areas (Grant et al. Citation2015). Protecting the global environment while providing humanity with needed water and land requirements presents a “monumental challenge” (Goodland Citation1995).
Despite widespread perceptions to the contrary (Abbott et al. Citation2019a, Citation2019b), to meet needs of a growing human population for food and water, Earth systems have been extensively altered (Vörösmarty et al. Citation1997, Citation2004, Citation2013, Vörösmarty and Sahagian Citation2000, Falkenmark et al. Citation2003, Wilcox et al. Citation2011, Rockström et al. Citation2014). Of the water consumed by humanity, evapotranspiration from agriculture accounts for over 90% (Döll Citation2009, Siebert et al. Citation2010, Hoekstra and Mekonnen Citation2012, Scanlon et al. Citation2017). While most of humanity’s global water footprint is satisfied by green water (Hoekstra and Wiedmann Citation2014), increasing rates of blue water consumption by irrigated agriculture have demanded corresponding increases in groundwater pumping and reservoir storage (Graf Citation1999). Transboundary watersheds present a particularly acute challenge if water requirements increase amongst all riparian states, while renewable water supplies remain limiting (Bakker and Duncan Citation2017, Wine Citation2019b, Citation2019c, Citation2019e). In affected domains, failure of governance can also serve as an impetus to increase local water consumption, thereby further stressing water resources within already water-limited regions (Dalby and Moussavi Citation2017).
In coastal regions rising populations are driving urban expansion, even in areas vulnerable to flooding (Hallegatte et al. Citation2013, Hinkel et al. Citation2014, Güneralp et al. Citation2015, Mcgranahan et al. Citation2016). The most important driver of increasing global exposure to coastal flooding is rising population in low-lying coastal areas (Hanson et al. Citation2010, Bouwer Citation2011, Cazenave and Cozannet Citation2014). Furthermore, coastal areas may be managed to fulfill multiple conflicting objectives; for example, saline water for shrimp farming may threaten freshwater or soil quality (Ali Citation2006, Ahmed Citation2011, Chowdhury et al. Citation2011, Grant et al. Citation2015). Moreover, upstream diversions of water may increase coastal salinity, thereby further impacting agriculture (Mirza Citation1998, Gain and Giupponi Citation2014). Hence, even without considering impacts of climate change, rising coastal populations, upstream flow diversions, and competing interests set the stage for vulnerability to flooding and environmental degradation.
2 Enter climatization
In the Anthropocene Epoch (Lewis and Maslin Citation2015), governments contend with convergence of resource scarcity, increasing marginal costs to acquire scarce resources,Footnote2 scientific support of anthropogenic climate change theory, and alignment between climate change projections and securitized issues (). In coastal areas a leading contender to mitigate water scarcity is seawater desalination, though it is expensive compared to exploitation of existing renewable freshwater resources, thereby presenting a disincentive to implement supply-side solutions. At the same time, the scientific community has increasingly supported anthropogenic climate change theory (Manabe and Wetherald Citation1967, Keeling et al. Citation1976, Oreskes Citation2004, Doran and Zimmerman Citation2009, Anderegg et al. Citation2010, Gleick et al. Citation2010, Cook et al. Citation2013, Citation2016, Maibach et al. Citation2014) while investing greatly to predict its hydrologic impacts (Arnell Citation1999, Held and Soden Citation2006, Maxwell and Kollet Citation2008, Kundzewicz et al. Citation2009b, Brown et al. Citation2019). In certain cases, impacts of climate change – relative to some control period – projected to transpire in future decades are already observed. For example, in certain parts of the world where water scarcity is predicted to result from climate change, heightened water scarcity is already observed.Footnote3 This convergence of factors has led to widespread contentious attribution to climatic factors of lake shrinkage (Madani Citation2014, Madani et al. Citation2016, Wurtsbaugh et al. Citation2017, Wine and Davison Citation2019, Zeitoun et al. Citation2019, Wine et al. Citation2019, Wine Citation2019a, Citation2019b, Citation2019c, Citation2019e), environmental degradation in Bangladesh (Grant et al. Citation2015), and the Syrian civil war (De Châtel Citation2014, Hendrix Citation2017, Selby et al. Citation2017a, Citation2017b), though in the Anthropocene Epoch climate change is not necessarily the most important factor (Grimm et al. Citation1997, Ferguson and Maxwell Citation2012, Feitelson and Tubi Citation2017, Wine et al. Citation2018, Wijngaard et al. Citation2018, Momblanch et al. Citation2019b) affecting hydrological processes.
Figure 1. Human domination of Earth systems presents global land and water managers with numerous challenges, which include phenomena that are inconvenient truths vis-a-vis the local politics, social norms, or perceived national security interests. Where pursuit of sustainable environmental governance has deteriorated, responsibility for environmental degradation may be mistakenly assigned to climatic drivers to fulfill expedient objectives by exploiting any number of vehicles. Several exemplars of climatization have become apparent within different domains and contexts. Each exemplar may implicate a particular subset of climatic processes with numerous consequences from both sociopolitical and scientific perspectives. The credibility of Earth sciences demands that scientists vigorously confront climatization.
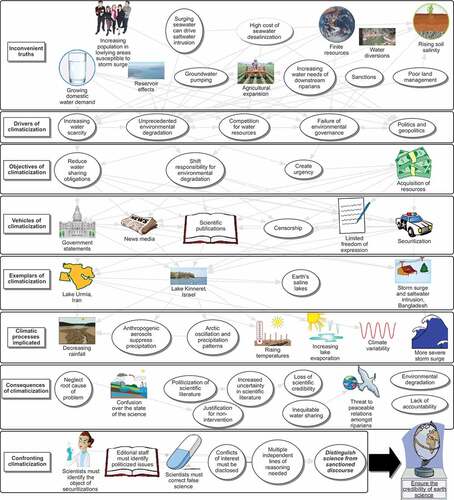
False attribution to climatic factors has been termed climatization (Grant et al. Citation2015). Grant et al. (Citation2015) define climatization as “framing a disastrous event or degraded environmental condition as caused by climate change, in order to reach an intended goal or to distract the discussion from the real problem which might have a different root course than caused by the climate change effects.” According to this definition, climatization is inherently driven by securitization. In the interest of framing a physical hydrology discussion without treating its sociopolitical context, Wine and Davison (Citation2019) proposed an alternative definition of climatization: “overemphasis of climatic factors relative to the demonstrated quantitative impacts of other anthropogenic drivers in eliciting an observed state”. This second definition is broader in that it could encompass climatization that may or may not be driven by securitization. On this basis, we propose a first typology of climatization.
In scientific climatization, climatic factors are overemphasized in certain studies, as part of the normal business of science, in which multiple hypotheses are considered and, in due course, certain hypotheses are falsified, whereas others are supported (Platt Citation1964). In this paradigm scientists’ only objective is to seek the truth and their professional liberties are uninfluenced by any securitizing state actor in terms of funding, carrying out their study, or disseminating results. In contrast, in securitization-aligned climatization, water resources are deemed by a state actor to be a national security issue – securitized (Zeitoun and Warner Citation2006, Stetter et al. Citation2011). As part of this securitization, freedom of expression of scientists domiciled within the stateFootnote4 is limited to a set of ideas perceived by the government to be beneficial (or non-detrimental) to national security, and therefore acceptable – sanctioned discourse (Feitelson Citation1999, Citation2002, Wine Citation2019d) – a central component of which may involve scapegoating climatic factors. The goal of this paper is to synthesize past research into a typology that improves understanding of the causes, characteristics, and consequences of climatization. In contrast to past research that focused solely on physical hydrology (Wine and Davison Citation2019), here we note the role of water resource securitization in climatization. This explicit acknowledgement of securitization is consistent with growing recognition of its relevance to hydrological process understanding (Goulden et al. Citation2010, Brooks and Trottier Citation2010, Yu et al. Citation2015, Schmeier and Shubber Citation2018, Farnum Citation2018, Grech-Madin et al. Citation2018, Wine Citation2019e).
3 Scientific versus securitization-aligned climatization
Scientific climatizationFootnote5 may occur when a conceptual model excludes a key process (Wine et al. Citation2019a, Abbott et al. Citation2019a, Citation2019b), when uncertainty is not fully accounted for, or when an interdisciplinary and complex process is interrogated from the perspective of a single discipline. Evidence of scientific climatization has arisen in cases where researchers domiciled in countries that protect freedom of expression () or in countries where the state has no apparent interest in the outcome of research on a particular topic, implicate climatic factors despite evidence to the contrary. That climatic factors (including climate change) may influence hydrologic states is a valid alternative hypothesis. Even when this hypothesis is subsequently questioned, the end result is often a better understanding of hydrologic science once all perspectives have been presented.
Figure 2. The majority of Earth’s human population resides in states classified by Freedom in the World as either not free or only partially free. Even among certain states categorized as free, water resource securitizations have been reported. Degree of freedom is from Freedom in the World 2018 compiled by Freedom House.
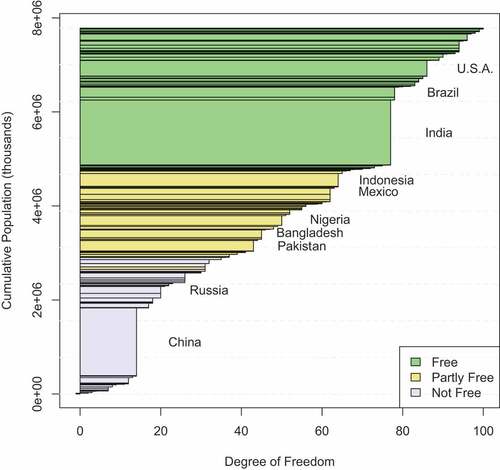
Securitization () aligned climatization has been identified to fulfill a number of expedient (geo)political objectives including absolving government of responsibility for environmental degradation (Dalby and Moussavi Citation2017), influencing water sharing in a transboundary watershed (Wine Citation2019a), or obtaining funding from those countries chiefly responsible for emissions of greenhouse gases (Grant et al. Citation2015). In contrast to scientific climatization, which transpires in an environment in which the scientists’ freedom of expression is protected, securitization-aligned climatization may occur in states that encourage or coerce scientists to limit their publications to topics that either align with a securitization or at a minimum do not contest that securitization. These limits on freedom of expression in securitized domains contrast with the expression of a wide range of views in the international scientific literature. While elements within the scientific community have recommended that certain views be censored (Huard Citation2011), the international scientific community as a whole has not adopted this recommendation.
Table 1. Reported securitizations.
4 Exemplars of climatization
4.1 Shrinkage of the Caspian Sea and Great Salt Lake
In inland waters, apparent instances of climatized processes include the shrinkage of the Caspian Sea and Great Salt Lake (). In the case of the Caspian Sea, Chen et al. (Citation2017) describe the role of rising temperatures in the sea’s shrinkage as “dominant”, whereas Van Dijk et al. (Citation2014) implicate inflow variability. Yet, after considering both climatic factors and human impacts, Rodell et al. (Citation2018) implicate the Aral Sea Syndrome: “diversions and direct withdrawals of water from the rivers” in the Caspian Sea’s basin. This choice by Rodell et al. (Citation2018) of a conceptual model that includes both climatic and direct human impacts may be the most important factor in reliable attribution of present-day hydrologic processes. In contrast, Van Dijk et al. (Citation2014) do not consider the possibility that decreasing Caspian Sea stage could be driven by increased basin water consumption. Similarly, Chen et al. (Citation2017)’s attribution of climate change induced increase in evaporation rates did not quantify the relative roles of increasing evaporation versus contemporary evaporative water consumption by irrigated agriculture.
Figure 3. As Earth has warmed, the mean annual water level in the Great Salt Lake has decreased (1880–2018). However, while warming can increase open water evaporation, this inverse relationship largely reflects a spurious correlation. The primary driver of the Great Salt Lake’s shrinkage is increasing human water consumption, with the role of rising temperatures being of lesser importance by an order of magnitude (Wurtsbaugh et al. Citation2017, Wine et al. Citation2019a).
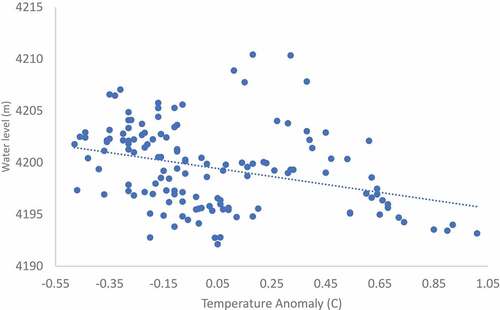
Meng (Citation2019) concludes that “climate changes … drive the dynamics” of the Great Salt Lake on the basis of statistical methods and by assuming that inflows are only climatically controlled and are not impacted by consumptive withdrawals in the lake’s basin. In contrast, when both basin water consumption (Wurtsbaugh et al. Citation2017) and increases in lake evaporation due to rising temperatures (Wine et al. Citation2019a) are quantitatively estimated, the latter is shown to be small. Wine et al. (Citation2019a) notes that most water resource development in the Great Salt Lake basin occurred before 1900, such that to fully capture the Great Salt Lake’s pre-development condition requires a study period that extends back 150–200 years from the present. Thus, in the case of shrinking lakes, a conceptual model that includes all relevant processes along with physically based quantification of the influence of those processes can prevent inadvertent climatization.
4.2 Shrinkage of Lake Urmia
Lake Urmia’s shrinkage () has also been climatized. Shadkam et al. (Citation2016a) suggested that Lake Urmia is shrinking principally due to climate change in contrast to other research (Madani Citation2014, Aghakouchak et al. Citation2015, Tourian et al. Citation2015, Taravat et al. Citation2016, Madani et al. Citation2016, Alizadeh-Choobari et al. Citation2016, Hamzekhani et al. Citation2016, Jalili et al. Citation2016, Fazel et al. Citation2017, Ashraf et al. Citation2017, Citation2018, Alborzi et al. Citation2018, Chaudhari et al. Citation2018, Ghale et al. Citation2018, Khazaei et al. Citation2019) pointing to water resource development as the primary driver. In contrast to other instances of climatization in which human impacts were absent from the conceptual model, Shadkam et al. (Citation2016a) correctly aim to quantify the relative impacts of irrigated agriculture and climatic changes. However, the equifinality thesis (Beven Citation2006, Müller and Levy Citation2019) has led Beven (Citation1993) to conclude that the “application of distributed hydrological models is more an exercise in prophecy than prediction”. Shadkam et al. (Citation2016a) note that a particular set of Variable Infiltration Capacity model parameters supports the conclusion that primarily climate change is shrinking Lake Urmia. However, they provide no evidence of an attempt to determine the uncertainty interval around this attribution or to “define critical experiments that will allow models as hypotheses to be adequately differentiated” (Beven Citation2012). Thus, even sophisticated modeling exercises can result in scientific climatization if the uncertainty inherent in hydrological modeling is not considered.
Figure 4. As agricultural water consumption increased in Lake Urmia’s basin the lake shrank from its historical extent due to a persistent negative water balance. Irrigated agricultural areas are from Meier et al. (Citation2018); historical lake extent, Lehner and Döll (Citation2004); watershed area (Lehner and Grill Citation2013); and current surface water extent from Landsat Normalized Difference Vegetation Index (NDVI) subject to the threshold of NDVI<-0.1.
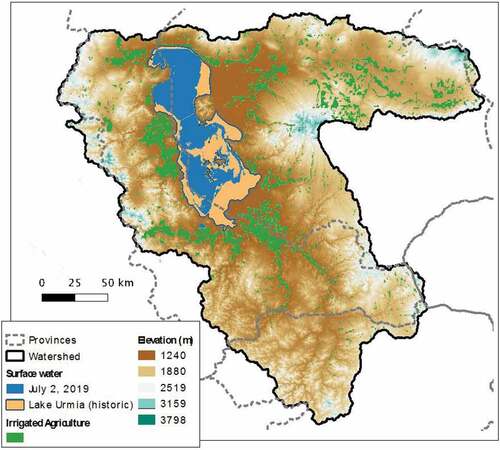
Prior to 1998 Lake Urmia’s stage remained within its historical range (Alborzi et al. Citation2018). However, since the 1980s water consumption by irrigated land in its basin nearly tripled in response to a doubling of irrigated area, leading to desiccation of 86% of Lake Urmia’s former area by 2016 (Chaudhari et al. Citation2018). By the early 2000s, the increase in water consumption in its basin pushed the lake to a tipping point such that it has not since rebounded to its “ecological threshold” (Alborzi et al. Citation2018). This desiccation was associated with a near quadrupling of the Iranian population since 1960, which increased water stress, along with competition amongst provinces to secure scarce water resources for development (Madani Citation2014).
Observing that rising temperatures correlated with the decline of inflows to Lake Urmia, Fathian et al. (Citation2014) and Fathian et al. (Citation2016) suggest that rising temperatures drove an increase in basin evapotranspiration. Though they allow that decreasing streamflows may be caused by both rising temperatures and over-exploitation of water resources, the possibility that the importance of these two processes is essentially co-equal is left open. Similarly, Jalili et al. (Citation2012), Fathian (Citation2019), Arkian et al. (Citation2018), Nazeri Tahroudi et al. (Citation2019), and Nourani et al. (Citation2018) acknowledge human impacts or over-exploitation, but focus on the role of climatic conditions. This literature states clearly that over-exploitation is non-zero but does not suggest that remedying over-exploitation alone would restore Lake Urmia.
Another approach resulting in ambiguity regarding the relative roles of climate and human impacts involves quantifying the impacts of different processes but reporting them together. On the basis of system dynamics modeling Hassanzadeh et al. (Citation2012) report “results show that … changes in inflows due to the climate change and overuse of surface water resources is the main factor for 65% of the effect”. Even when authors, using similar data and methods, achieve similar results – detecting significant long-term trends in streamflow near Lake Urmia while significant trends are not detected in the lake’s headwaters – some resist implicating water management activities. For example, Fazel et al. (Citation2017) conclude that “the results showed that irrigation was by far the main driving force for river flow regime changes in the lake basin.” In contrast, Fathian et al. (Citation2014) conclude (indefinitely) that “it can be primarily attributed to climate change and over-exploitation”.
4.3 The feasibility of restoring Lake Urmia
The aforementioned hydrologic model developed by Shadkam et al. (Citation2016a) was forced by a subset of bias-adjusted general circulation model predictions for mid-century (), resulting in the impactful conclusion that “future water management plans are not robust under climate change” (Shadkam et al. Citation2016b). This pessimistic conclusion exacerbates existing concerns that “there is a serious risk of implementation failure due to contradictory national policy agendas of lake restoration and agricultural development, insufficient budget allocation for realizing the restoration plan, lack of provincial accountability for the spending of resources made available for the implementation of restoration measures, and potential future political instability which may lead to less attention to the restoration process” (Salimi et al. Citation2019). Taken together with research on the present climate suggesting that restoring environmental flows to Lake Urmia is (technically) feasible (Alborzi et al. Citation2018), we would conclude that the primary challenge in restoring Lake Urmia is political, not climate change. Similarly, Beven (Citation2011) states “I believe in climate change (and the potential impacts of other catchment changes) but I would suggest that we need better ways of deciding how precautionary to be in planning for the future.” This sentiment is substantiated by questions raised about the credibility of climate projections given that they are poorly correlated with observations in the historical record (Nohara et al. Citation2006, Douglass et al. Citation2008, Koutsoyiannis et al. Citation2009, Citation2010, Kundzewicz et al. Citation2009a, Citation2009b, Anagnostopoulos et al. Citation2010, Schwartz et al. Citation2010, Christy et al. Citation2010, Stockwell Citation2010, Kundzewicz and Stakhiv Citation2010, Scafetta Citation2012, Citation2013, Legates Citation2014, Frigg et al. Citation2015, Pindyck Citation2017, Connolly et al. Citation2019, Nissan et al. Citation2019). Despite understanding that parameter uncertainty can be as large as global climate model (GCM) uncertainty (Wilby Citation2005), Shadkam et al. (Citation2016b) do not quantify how hydrological model parameter uncertainty propagates with GCM uncertainty. The result is a conclusion that climatizes the nature of the Lake Urmia restoration challenge, while disregarding the admonition of Kundzewicz and Stakhiv (Citation2010) that “climate models are not … ready for ‘prime time’ yet, at least for direct application to water management problems. Much more research needs to be done, and models need to be improved considerably before they can be used effectively for adaptation planning and design.”
Figure 5. Change in precipitation (%) in the future (2010–2099) relative to a baseline period (1971–2000) under RCP8.5 (top left) and RCP2.6 (top right). The red bars represent the projections of the five GCMs selected by Shadkam et al. (Citation2016b) and their ensemble mean, and the blue bars represent the projections of five other GCMs and their ensemble mean. Similar figures are provided for different projection periods (2010–2039, 2040–2069, and 2070–2099) consistent with Shadkam et al. (Citation2016b). The figure highlights that robust projections require consideration of a wide range of possible future climatic conditions.
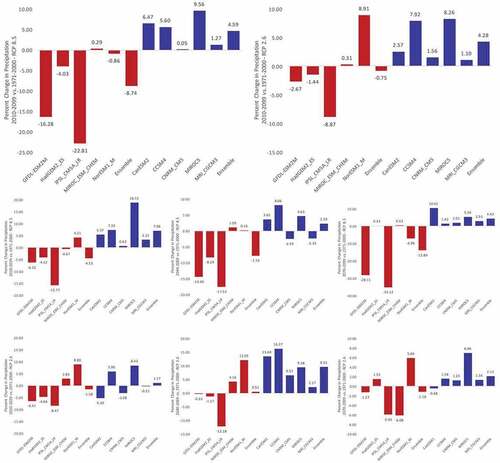
4.4 The Syrian civil war
Climate change has also been implicated in contributing to the initiation of the recent civil war in Syria (Kelley et al. Citation2015, Citation2017, Werrell et al. Citation2015). This determination has been based in part on assessment that “anthropogenic greenhouse gas and aerosol forcing are key attributable factors for this increased drying” that may have brought about elevated Mediterranean drought frequency (Hoerling et al. Citation2012). In contrast to the climatic attribution, Selby et al. (Citation2017b) state “there is thus no good evidence to conclude that global climate change-related drought in Syria was a contributory causal factor in the country’s civil war.” Moreover, while acknowledging the drought in Syria, De Châtel (Citation2014) points also to “a host of political, economic and social grievances.” While accepting evidence of a climate change signal in Syria’s pre-conflict drought, Hendrix (Citation2017) observes: “ … the drought that affected Syria also affected neighboring Jordan, Lebanon and Cyprus, yet widespread violence did not occur there.” More recently, Mach et al. (Citation2019) conclude: “climate has affected organized armed conflict within countries. However, other drivers, such as low socioeconomic development and low capabilities of the state, are judged to be substantially more influential, and the mechanisms of climate–conflict linkages remain akey uncertainty.” The complexity and interdisciplinarity of many of today’s critical challenges recalls the recommendation of Wesselink et al. (Citation2014): “ …research should be done by teams of people with arange of backgrounds.” Adhering to such guidance, including on topics with important policy implications, might prevent perceptions of climatization, in favor of interdisciplinary collaboration.
4.5 Lake Kinneret
In the transboundary context, climatization can be used, together with projections of future increases in aridity, to justify reduced water-sharing obligations. Water-sharing agreements involve proportional sharing of renewable water resources. Thus, a reduction in renewable water would reduce the amount of water subject to water-sharing agreements. However, under non-stationarity, the amount of renewable water in transboundary basins is expected to change (Ward Citation2013, Jafroudi Citation2018), though how to quantify this change credibly and equitably incorporate non-stationarity into water-sharing agreements remains unresolved (Cooley and Gleick Citation2011). In the case of Lake Kinneret’s watershed, part of the transboundary Jordan River basin (), both Israel (Wine Citation2019b, Citation2019c, Citation2019e) and Syria (Haddadin Citation2011) have been implicated in climatizing decreasing flows to downstream riparian states – the Kingdom of Jordan and the Palestinian Authority – potentially allowing the upstream riparians to increase their own water consumption (Shentsis et al. Citation2019, Wine et al. Citation2019b). In the case of Israel, which accesses the Mediterranean Sea, climatization reduces its obligation to bear the expense of desalinizing water to meet needs of the downstream riparian states.
Figure 6. In the transboundary Jordan River basin, hydrologic science determines how water availability and quality will change under climatic non-stationarity. However, securitization of these transboundary water resources has manifested when a securitizing state actor enforces data secrecy (Wine Citation2019e) that obscures the relative roles of endogenous versus exogenous drivers (Wine Citation2019b). By obfuscating hydrologic process understanding in a transboundary basin, an upstream riparian may increase water consumption or impact water quality while falsely assigning to climatic factors responsibility for water scarcity or degraded water quality (Wine Citation2019a).
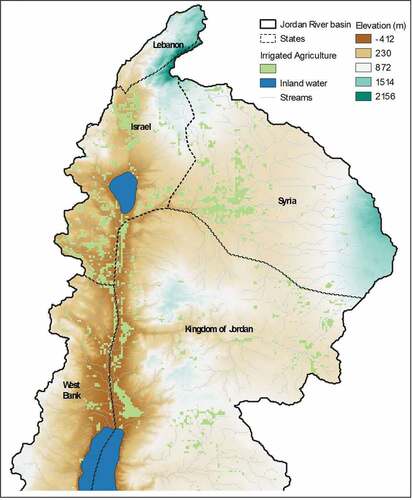
Lake Kinneret has religious significance and is the only regional freshwater lake. Consequently, it has been studied extensively. Historically, it was an exorheic lake fed primarily by the Upper Jordan River and emptying into the Lower Jordan River, which terminates at the Dead Sea. However, as Lake Kinneret’s water balance has become more negative the formerly exorheic lake has effectively become an endorheic lake, with natural outflows to the Lower Jordan River having effectively ceased decades ago. The consequence of this reduced natural flow, as well as decreased pumping rates, has been water quality impairment, such that lake water quality no longer meets US EPA drinking water standards of 250 mg L−1 of chloride. The Israel Jordan Peace Treaty requires that neither country take actions that harm the quality of the water supplied to the other, such that if Israel were substantially responsible for the observed water quality degradation in Lake Kinneret, from whence the Kingdom of Jordan is supplied with water, that would be a violation of the Treaty. Rising populations in the Jordan River basin, a renewed focus on a peace agreement between Israel and the Palestinians, and the deadline for cancelling or renewing a 25-year land access agreement serve as the socio-political backdrop of present heightened tensions over water. Though many studies do not explicitly consider Lake Kinneret’s location in the transboundary Jordan River basin, any hydrological science results from Lake Kinneret are directly relevant to water sharing amongst Israel, the Kingdom of Jordan, and the Palestinian Authority.
Early on, Inbar and Bruins (Citation2004) implicated “human interference, water pumping and flow diversion” in impacting Lake Kinneret. This attribution is similar to conclusions in the neighboring Yarmouk basin (Muller et al. Citation2016, Shentsis et al. Citation2019) and consistent with Morin et al. (Citation2018): “From 1964 [when Israel’s National Water Carrier was completed], substantial human intervention in the lakeFootnote6 watershed caused a steep decline of lake level.Footnote7” However, Givati and Rosenfeld (Citation2007, Citation2009) suggested that decreasing net inflows into Lake Kinneret were driven primarily by suppression of orographic precipitation. This mechanism was disputed by Alpert et al. (Citation2008) and Halfon et al. (Citation2009) for most of central and northern Israel, excluding the Golan Heights – the most important recharge zone of Lake Kinneret’s securitized transboundary watershed. Numerous studies have claimed that available water in Lake Kinneret’s headwaters is decreasing and attributed this decrease to anthropogenic climate change (Tal Citation2017, Citation2018, Citation2019a, Citation2019b, Citation2019c, Givati et al. Citation2019, Gophen Citation2019), though in most cases failing to provide a detailed methodology.Footnote8 Tal (Citation2018) suggests that available water has already decreased by 40% since the mid-1970s, which contrasts with climate change projections suggesting only a possible 17.5% decline by the end of the century (Smiatek and Kunstmann Citation2016). These deterministic attributions and projections, respectively, also contrast with those that consider variability and include the possibility of little or no impact of climate change on precipitation (Rimmer et al. Citation2011). Assertions of a 40% decline in available water also disagree with Samuels et al. (Citation2010) who state “changes in streamflow of the Jordan River and its tributaries [as a consequence of climate change] are not significant in the near future.”
Investigations of Lake Kinneret’s water balance have been limited through access restrictions imposed on key datasets, including water consumption (Klein Citation1998, Avni et al. Citation2015, Wine et al. Citation2019b, Wine Citation2019e). However, recent studies that investigate Lake Kinneret’s shrinkage have found less evidence for the climate change hypothesis and stronger evidence of increasing water consumption associated with rising human population (Wine and Davison Citation2019, Wine Citation2019a, Citation2019b, Citation2019c, Citation2019e, Wine et al. Citation2019b). This conclusion is based on headwater streamflows and long-term precipitation where no significant trends were detected (Wine et al. Citation2019b). Moreover, inability to reconcile the relative magnitudes of observed (satellite derived) increases in water consumption by irrigated agriculture with streamflow decreases is most consistent with increasing diversion of water (Wine et al. Citation2019b). Wine (Citation2019a), Citation(2019c), and Citation(2019b) specifically critique certain aspects of the science that assigns Lake Kinneret’s desiccation to climatic factors.
4.6 Bangladesh
Historically, Bangladesh has been impacted by natural disasters and environmental degradation – including cyclones (Islam and Peterson Citation2009) and saltwater intrusion (Chowdhury Citation2010). The impacts of the cyclones depend not only on their characteristics but also on the number of people and amount of infrastructure affected. These impacts, in turn, are controlled in part by the efficacy of flood control measures such as embankments. Flooding induced by cyclones, diversion of the Ganges (Mirza Citation1998), and farming shrimp with saline water (Chowdhury et al. Citation2011) contribute to saltwater intrusion. As a consequence of climate change these historic phenomena – cyclones and saltwater intrusion – could become more severe, with an increase in storm magnitude and frequency (Mirza Citation2003) and sea-level rise (Chen and Mueller Citation2018). However, despite the reality of existing human impacts on Bangladesh, government officials have focused on projected impacts of anthropogenic climate change, aiming to obtain development assistance funding (Grant et al. Citation2015). Grant et al. (Citation2015) deemed this exclusive focus on climate change projections, even in the presence of known human impacts, climatization.
4.7 Lake Hulun
Studies of regional lake loss explicitly note that agricultural water consumption is a key driver of lake loss in certain regions of China, though without quantifying the extent to which irrigated agriculture contributed to the shrinkage of individual lakes (Liu et al. Citation2013, Tao et al. Citation2015). Nonetheless, shrinkage of Lake Hulun, China, has been attributed to decreasing river discharge due to decreasing precipitation, with no reference to any impacts of irrigated agriculture (Cai et al. Citation2016, Zheng et al. Citation2016), despite the apparent influence of irrigated agriculture on the basin’s water balance ().
4.8 Himalayas
A recent review of global change hydrology literature in the Himalayas by Momblanch et al. (Citation2019a) found that:
[N]one of the reviewed studies include other associated consequences of socio-economic change on water abstraction, reservoir storage capacity, hydropower and irrigation demand, which may be more important determinants of the future water security than climate change. This omission may limit the usefulness of modelling outcomes as it obscures correlations between drivers and impacts. For instance, the impact of human water consumption change triggered by non-climate factors (such as population increase, improvements in standard of living) may be misattributed to temperature increase if models do not account for relevant socio-economic component of global change.
This focus on climate may be driven by the transboundary character of the rivers draining the Himalayas combined with securitization triggered by “fragile political stability between co-riparian countries due to distrust and power asymmetry.” Securitization has led to “data secrecy around water management and consumption that prevents incorporation of these components” in hydrologic modeling. They suggest that “the particular geo-political situation of the region, which can prevent ‘sensitive’ hydro-meteorological data from being shared or even collected due to conflicts related to water resources sharing in the Indus, Ganges, and Brahmaputra transboundary basins, may hinder the identification of the main drivers of hydrological change and, thereby, the design and implementation of adequate adaptation measures.” Advancing knowledge on securitized hydrologic science topics may then require that “transboundary research projects should be promoted by independent international institutions.”
4.9 India
Water has been securitized in India’s transboundary basins (Hanasz Citation2014, Grech-Madin et al. Citation2018). “The Indian government considers hydrological data for the Ganges to be a matter of national security and has not released it publicly since 1973” (Johnston and Smakhtin Citation2014). Similarly, with respect to transboundary basins to which India is a riparian, Thenkabail et al. (Citation2009) note “reluctance on part of the states to furnish irrigated area data in view of their vested interests in sharing of water.” Unavailability of reliable water consumption data “is clearly a problem for calibration and validation of models in a system where the infrastructure is evolving rapidly” (Johnston and Smakhtin Citation2014) in that it increases the uncertainty in hydrologic modeling. This enhanced uncertainty may then set the stage for erroneous understanding regarding the relative role of climatic versus human drivers in bringing about observed hydrologic states.
5 Mitigating climatization
Increasing recognition that human health depends on planetary health or GeoHealth (Almada et al. Citation2017) is apropos, for example, relative to Earth’s besieged inland waters given the dependence of humanity on freshwater and fisheries therein, as well as the negative health impacts of lake desiccation (Micklin Citation1988, Citation2007, Citation2016, Brooks et al. Citation2016). While science has the potential to rationally inform public policy for the betterment of humanity (Kirchner Citation2017), this relies on science remaining an objective search for the truth and scorning vested interests. In contrast, climatization undermines the credibility of environmental science literature.
What then can be done to mitigate climatization? What can editors and reviewers of hydrological sciences journals do to ensure that climatization does not co-opt environmental science literature to strengthen securitization? Publications that climatize environmental degradation may:
Choose a conceptual model of the water cycle that inappropriately neglects instances of human domination (Abbott et al. Citation2019a, Citation2019b).
Rely heavily on empirical correlations, falsely assuming that statistical correlations imply causation. (Müller and Levy (Citation2019) and Ferraro et al. (Citation2019) suggest strategies and challenges for mitigating equifinality in coupled human-natural systems.)
Rely on qualitative lines of reasoning to justify quantitative conclusions.
Fail to quantify volumes or fluxes attributed to different global change drivers using methods of physical hydrology.
Address only a single aspect of a multi-disciplinary question.
Fail to provide the methodology and data required to repeat calculations.
Rely entirely on a single line of reasoning while excluding numerous lines of reasoning or datasets that would readily falsify the climatic thesis.
Fail to validate selected models or otherwise demonstrate that the selected model is objectively optimal.
Fail to quantify model uncertainty and its propagation.
Rely heavily on consequential datasets with important implications, but that have not been verified or replicated by an independent third party.
By increasing awareness of these characteristics of publications, gatekeepers of the scientific literature may screen out dubious science, including on securitized issues, prior to publication.
6 Causes, characteristics, and consequences of climatization
Scientific climatization occurs when the role of climatic drivers in eliciting an observed state is overstated as part of the normal business of science. This overstatement can occur readily due to the often-high correlation between climatic and non-climatic drivers, growing awareness of anthropogenic climate change, and a persistent propensity for disciplinary investigation of inter-disciplinary problems. Instances of scientific climatization may occur when an idealized conceptual model is adopted (Abbott et al. Citation2019b), the effects of model uncertainty are neglected, a disciplinary team of scientists studies an interdisciplinary problem, or when incisive attempts to falsify the climatic thesis are overlooked. As a consequence of climatization, hydrologic process understanding is impaired until the misattribution has been corrected. The consequence of this misattribution then is a weakened scientific basis for water management. However, once scientific climatization is corrected, it often results in a more robust scientific literature.
Securitization-aligned climatization bears many similarities to scientific climatization, with the exception of impairment of freedom of expression of affected scientists through encouragement and coercion (Wine Citation2019d) by a securitizing state actor.Footnote9 This coercion arises from the great expense involved in solving hydrologic problems – often water scarcity or water quality degradation – in comparison to the substantially lower expense of attributing those problems to climatic factors outside of the government’s control. As a result, instances of securitization-aligned climatization may be characterized by secrecy regarding key hydrologic data (Momblanch et al. Citation2019a, Wine Citation2019e), questions of reproducibility (Abbott et al. Citation2019a), or conclusions by scientists domiciled in securitized states that differ from those of scientists whose freedom of expression is protected (Wine Citation2019d), even on the basis of similar results. In contrast to scientific climatization, which following correction tends to lead to improved scientific understanding, this is not the intent of securitization-aligned climatization. By persistently funding, encouraging, and coercing a particular view, this perspective tends to persist in the scientific literature. The result of this persistent securitizing state-sponsored climatization is ambiguity regarding the state of the hydrologic science, with all that that implies for water policy.
7 Implications for socio-hydrology
In traditional hydrology, relevant state variables driving hydrologic dynamics include hydraulic head, temperatures, and solute concentration. In socio-hydrology, these traditional state variables remain relevant, but an additional suite of state variables has been acknowledged (). These variables are considered in the seminal socio-hydrology literature, which has often pointed to case studies in which feedbacks between co-evolving human and hydrologic systems drive the dynamics of both (Sivapalan et al. Citation2012, Kandasamy et al. Citation2014, Sivapalan et al. Citation2014). In such case studies, a typical pattern may emerge in which humans modify the hydrologic system, perceive the modification to be excessively detrimental to the environment, then take action aimed at undoing that component of the environmental exploitation deemed excessive. However, when this paradigm is considered in the context of the present work, a cross-cutting variable critical to socio-hydrology emerges – the degree of water resource securitization. This securitization has the potential to influence the nature of feedbacks between human and water systems, for example, as a consequence of securitization-aligned climatization, which may persistently alter hydrologic process understanding. Securitization is related to suppression of freedom of expression and suppression of freedoms more broadly. This suppression of freedom in turn is known to strongly influence fertility rates (), a factor determining global population, which forces the global hydrologic system through changes in agricultural areas. Hence, in socio-hydrology, consideration of degree of water resource securitization and freedom, where appropriate, may ensure that conceptual and mathematical models attain optimal structure.
Table 2. State variables in socio-hydrology (Sivapalan et al. Citation2012, Citation2014, Sivapalan Citation2015, Di Baldassarre et al. Citation2015, Loucks Citation2015, Gober and Wheater Citation2015, Troy et al. Citation2015a, Citation2015b, Tian et al. Citation2019).
Figure 8. With increasing freedom progress toward a sustainable human population is achieved (Campbell et al. Citation2013, Warren Citation2015, Citation2016). Degree of freedom is from the 2018 Freedom in the World Index compiled by Freedom House. Fertility Rate is from the World Fact Book, estimated for 2018.
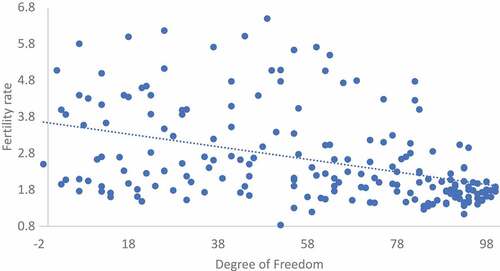
Acknowledgements
Constructive critiques from three named reviewers—Drs. Demetris Koutsoyiannis, Pete Loucks, and Marc Mueller—and one anonymous reviewer are gratefully acknowledged. The support of the journal editors and contributions of several other unnamed colleagues in strengthening this manuscript were very much appreciated.
Disclosure statement
No potential conflict of interest was reported by the author.
Additional information
Funding
Notes
1 The rate of progression toward Tragedy of the Commons may be governed by the degree of mutual coercion, mutually agreed upon. For example, Müller et al. (Citation2017) suggest that an impending Tragedy of the Commons can be postponed substantially by increasing the distance between groundwater pumping centers.
2 This refers to, for example, the relatively large expense of building and operating a large-scale desalination plant as opposed to using local renewable high-quality surface water or groundwater sources.
3 For example, some climate change scenarios predict decreasing renewable water availability in the Jordan River and Lake Urmia basins. It is important to note though that climate change is only one candidate cause of water scarcity with another being increased human water consumption to support growing populations.
4 Evidence of securitization has arisen in both authoritarian states as well as those with a democratic system of governance.
5 The author currently has a paper in review arguing that one of the author’s own previous works climatized the shrinkage of Lake Kinneret by overestimating the role drought.
6 Dead Sea.
7 Lake Kinneret is an exorheic lake upstream of the Dead Sea from which water is drawn to Israel’s National Water Carrier (NWC). Hence, negative water balance in the Dead Sea due to the NWC necessarily implies negative water balance in Lake Kinneret as well.
8 The absence of a detailed methodology challenges falsification or verification of these results.
9 See the Academic Freedom Monitoring Project compiled by Scholars at Risk or the Committee of Concerned Scientists for means by which securitizing state actors seek to influence scholarly and scientific activities.
References
- Abbott, B.W., et al. 2019a. A water cycle for the Anthropocene. Hydrological Processes, In Press, 33, 3046–3052. doi:10.1002/hyp.13544.
- Abbott, B.W., et al. 2019b. Human domination of the global water cycle absent from depictions and perceptions. Nature Geoscience, 12, 533–540. doi:10.1038/s41561-019-0374-y.
- Aghakouchak, A., et al., 2015. Aral Sea syndrome desiccates Lake Urmia: call for action. Journal of Great Lakes Research, 41 (1), 307–311. doi:10.1016/j.jglr.2014.12.007.
- Ahmed, A., 2011. Some of the major environmental problems relating to land use changes in the coastal areas of Bangladesh: a review. Journal of Geography and Regional Planning, 4 (1), 1–8.
- Alborzi, A., et al. 2018. Climate-informed environmental inflows to revive a drying lake facing meteorological and anthropogenic droughts. Environmental Research Letters, 13 (8), 084010. doi:10.1088/1748-9326/aad246
- Ali, A.M.S., 2006. Rice to shrimp: land use/land cover changes and soil degradation in southwestern Bangladesh. Land Use Policy, 23 (4), 421–435. doi:10.1016/j.landusepol.2005.02.001.
- Alizadeh-Choobari, O., et al., 2016. Climate change and anthropogenic impacts on the rapid shrinkage of Lake Urmia. International Journal of Climatology, 36 (13), 4276–4286. doi:10.1002/joc.4630.
- Almada, A.A., et al., 2017. A case for planetary health/geohealth. GeoHealth, 1 (2), 75–78. doi:10.1002/2017gh000084.
- Alpert, P., Halfon, N., and Levin, Z., 2008. Does air pollution really suppress precipitation in Israel? Journal of Applied Meteorology and Climatology, 47 (4), 933–943. doi:10.1175/2007jamc1803.1.
- Amesheva, I., 2017. Environmental degradation and economic development in China: an interrelated governance challenge. Law and Development Review, 10 (2). doi:10.1515/ldr-2017-0020.
- Anagnostopoulos, G.G., et al., 2010. A comparison of local and aggregated climate model outputs with observed data. Hydrological Sciences Journal, 55 (7), 1094–1110. doi:10.1080/02626667.2010.513518.
- Anderegg, W.R.L., et al., 2010. Expert credibility in climate change. Proceedings of the National Academy of Sciences, 107 (27), 12107–12109. doi:10.1073/pnas.1003187107.
- Anderson, D.J., 2019. Population and the environment — time for another contraception revolution. New England Journal of Medicine, 381 (5), 397–399. doi:10.1056/NEJMp1906733.
- Arkian, F., Nicholson, S.E., and Ziaie, B., 2018. Meteorological factors affecting the sudden decline in Lake Urmia’s water level. Theoretical and Applied Climatology, 13 (1), 641–651. doi:10.1007/s00704-016-1992-6.
- Arnell, N.W., 1999. Climate change and global water resources. Global Environmental Change, 9, S31–S49. doi:10.1016/S0959-3780(99)00017-5
- Ashraf, B., et al., 2017. Quantifying anthropogenic stress on groundwater resources. Scientific Reports, 7 (1), 12910. doi:10.1038/s41598-017-12877-4.
- Ashraf, S., et al., 2018. Compounding effects of human activities and climatic changes on surface water availability in Iran. Climatic Change, 152 (3–4), 379–391. doi:10.1007/s10584-018-2336-6.
- Avni, N., Fishbain, B., and Shamir, U., 2015. Water consumption patterns as a basis for water demand modeling. Water Resources Research, 51 (10), 8165–8181. doi:10.1002/2014wr016662.
- Bakker, M.H.N. and Duncan, J.A., 2017. Future bottlenecks in international river basins: where transboundary institutions, population growth and hydrological variability intersect. Water International, 42 (4), 400–424. doi:10.1080/02508060.2017.1331412.
- Best, J., 2019. Anthropogenic stresses on the world’s big rivers. Nature Geoscience, 12 (1), 7–21. doi:10.1038/s41561-018-0262-x.
- Beven, K., 1993. Prophecy, reality and uncertainty in distributed hydrological modelling. Advances in Water Resources, 16 (1), 41–51. doi:10.1016/0309-1708(93)90028-E.
- Beven, K., 2006. A manifesto for the equifinality thesis. Journal of Hydrology, 320 (1–2), 18–36. doi:10.1016/j.jhydrol.2005.07.007.
- Beven, K., 2011. I believe in climate change but how precautionary do we need to be in planning for the future? Hydrological Processes, 25 (9), 1517–1520. doi:10.1002/hyp.7939.
- Beven, K., 2012. Causal models as multiple working hypotheses about environmental processes. Comptes Rendus Geoscience, 344 (2), 77–88. doi:10.1016/j.crte.2012.01.005.
- Bouwer, L.M., 2011. Have disaster losses increased due to anthropogenic climate change? Bulletin of the American Meteorological Society, 92 (1), 39–46. doi:10.1175/2010bams3092.1.
- Brooks, B.W., et al., 2016. Are harmful algal blooms becoming the greatest inland water quality threat to public health and aquatic ecosystems? Environmental Toxicology And Chemistry / SETAC, 35 (1), 6–13. doi:10.1002/etc.3220.
- Brooks, D. and Trottier, J., 2010. Confronting water in an Israeli-Palestinian peace agreement. Journal of Hydrology, 382 (1–4), 103–114. doi:10.1016/j.jhydrol.2009.12.021.
- Brown, T.C., Mahat, V., and Ramirez, J.A., 2019. Adaptation to future water shortages in the United States caused by population growth and climate change. Earth’s Future, 7, 219–234. doi:10.1029/2018ef001091
- Cai, Z.S., et al. 2016. Is China’s fifth-largest inland lake to dry-up? Incorporated hydrological and satellite-based methods for forecasting Hulun lake water levels. Advances in Water Resources, 94, 185–199. doi:10.1016/j.advwatres.2016.05.010.
- Campbell, M.M., Prata, N., and Potts, M., 2013. The impact of freedom on fertility decline. The Journal of Family Planning and Reproductive Health Care, 39 (1), 44–50. doi:10.1136/jfprhc-2012-100405.
- Cazenave, A. and Cozannet, G.L., 2014. Sea level rise and its coastal impacts. Earth’s Future, 2 (2), 15–34. doi:10.1002/2013ef000188.
- Chaudhari, S., et al. 2018. Climate and anthropogenic contributions to the desiccation of the second largest saline lake in the twentieth century. Journal of Hydrology, 560, 342–353. doi:10.1016/j.jhydrol.2018.03.034.
- Chen, J. and Mueller, V., 2018. Coastal climate change, soil salinity and human migration in Bangladesh. Nature Climate Change, 8 (11), 981–985. doi:10.1038/s41558-018-0313-.
- Chen, J.L., et al., 2017. Long-term Caspian Sea level change. Geophysical Research Letters, 44 (13), 6993–7001. doi:10.1002/2017gl073958.
- Chowdhury, M.A., et al., 2011. Effect of combined shrimp and rice farming on water and soil quality in Bangladesh. Aquaculture International, 19 (6), 1193–1206. doi:10.1007/s10499-011-9433-0.
- Chowdhury, N.T., 2010. Water management in Bangladesh: an analytical review. Water Policy, 12 (1), 32–51. doi:10.2166/wp.2009.112.
- Christy, J.R., et al., 2010. What do observational datasets say about modeled tropospheric temperature trends since 1979? Remote Sensing, 2 (9), 2148–2169. doi:10.3390/rs2092148.
- Connolly, R., et al. 2019. Northern hemisphere snow-cover trends (1967–2018): a comparison between climate models and observations. Geosciences, 9 (3). doi:10.3390/geosciences9030135.
- Cook, J., et al. 2013. Quantifying the consensus on anthropogenic global warming in the scientific literature. Environmental Research Letters, 8 (2), 024024. doi:10.1088/1748-9326/8/2/024024
- Cook, J., et al. 2016. Consensus on consensus: a synthesis of consensus estimates on human-caused global warming. Environmental Research Letters, 11 (4), 048002. doi:10.1088/1748-9326/11/4/04800
- Cooley, H. and Gleick, P.H., 2011. Climate-proofing transboundary water agreements. Hydrological Sciences Journal, 56 (4), 711–718. doi:10.1080/02626667.2011.576651.
- Dalby, S. and Moussavi, Z., 2017. Environmental security, geopolitics and the case of lake Urmia’s disappearance. Global Change Peace & Security, 29 (1), 39–55. doi:10.1080/14781158.2016.1228623.
- De Châtel, F., 2014. The role of drought and climate change in the Syrian uprising: untangling the triggers of the revolution. Middle Eastern Studies, 50 (4), 521–535. doi:10.1080/00263206.2013.850076.
- Dery, D. and Salomon, I., 1997. “After me, the deluge”: uncertainty and water policy in Israel. International Journal of Water Resources Development, 13 (1), 93–110. doi:10.1080/07900629749953.
- Di Baldassarre, G., et al., 2015. Debates—perspectives on socio-hydrology: capturing feedbacks between physical and social processes. Water Resources Research, 51 (6), 4770–4781. doi:10.1002/2014WR016416.
- Döll, P., 2009. Vulnerability to the impact of climate change on renewable groundwater resources: a global-scale assessment. Environmental Research Letters, 4 (3), 035006. doi:10.1088/1748-9326/4/3/035006.
- Doran, P.T. and Zimmerman, M.K., 2009. Examining the scientific consensus on climate change. Eos, Transactions American Geophysical Union, 90 (3), 22–23. doi:10.1029/2009EO030002.
- Douglass, D.H., et al., 2008. A comparison of tropical temperature trends with model predictions. International Journal of Climatology, 28 (13), 1693–1701. doi:10.1002/joc.1651.
- El-Sayed, M.K. and Mansour, R.S., 2017. Water scarcity as a non-traditional threat to security in the Middle East. India Quarterly-a Journal of International Affairs, 73 (2), 227–240. doi:10.1177/0974928417699916.
- Falkenmark, M., Folke, C., and Meybeck, M., 2003. Global analysis of river systems: from earth system controls to Anthropocene syndromes. Philosophical Transactions of the Royal Society of London. Series B: Biological Sciences, 358 (1440), 1935–1955. doi:10.1098/rstb.2003.1379.
- Farnum, R.L., 2018. Drops of diplomacy: questioning the scale of hydro-diplomacy through fog-harvesting. Journal of Hydrology, 562, 446–454. doi:10.1016/j.jhydrol.2018.05.012
- Fathian, F., et al. 2016. Trends in hydrological and climatic variables affected by four variations of the Mann-Kendall approach in Urmia Lake Basin, Iran. Hydrological Sciences Journal, 1–13. doi:10.1080/02626667.2014.932911.
- Fathian, F., 2019. Dynamic memory of Urmia Lake water-level fluctuations in hydroclimatic variables. Theoretical and Applied Climatology, 138, 591–603. doi:10.1007/s00704-019-02844-6
- Fathian, F., Morid, S., and Kahya, E., 2014. Identification of trends in hydrological and climatic variables in Urmia Lake Basin, Iran. Theoretical and Applied Climatology, 119 (3–4), 443–464. doi:10.1007/s00704-014-1120-4.
- Fazel, N., Haghighi, A.T., and Klove, B., 2017. Analysis of land use and climate change impacts by comparing river flow records for headwaters and lowland reaches. Global and Planetary Change, 158, 47–56. doi:10.1016/j.gloplacha.2017.09.014
- Feitelson, E., 1999. Social norms, rationales and policies: reframing farmland protection in Israel. Journal of Rural Studies, 15 (4), 431–446. doi:10.1016/s0743-0167(99)00013-3.
- Feitelson, E., 2002. Implications of shifts in the Israeli water discourse for Israeli-Palestinian water negotiations. Political Geography, 21 (3), 293–318. doi:10.1016/S0962-6298(01)00038-5.
- Feitelson, E. and Tubi, A., 2017. A main driver or an intermediate variable? Climate change, water and security in the Middle East. Global Environmental Change, 44, 39–48. doi:10.1016/j.gloenvcha.2017.03.001
- Ferguson, I.M. and Maxwell, R.M., 2012. Human impacts on terrestrial hydrology: climate change versus pumping and irrigation. Environmental Research Letters, 7 (4), 044022. doi:10.1088/1748-9326/7/4/044022.
- Ferraro, P.J., Sanchirico, J.N., and Smith, M.D., 2019. Causal inference in coupled human and natural systems. Proceedings of the National Academy of Sciences of the USA, 116 (12), 5311–5318. doi:10.1073/pnas.1805563115.
- Frigg, R., Smith, L.A., and Stainforth, D.A., 2015. An assessment of the foundational assumptions in high-resolution climate projections: the case of UKCP09. Synthese, 192 (12), 3979–4008. doi:10.1007/s11229-015-0739-8.
- Gain, A. and Giupponi, C., 2014. Impact of the Farakka dam on thresholds of the hydrologic flow regime in the lower Ganges river basin (Bangladesh). Water, 6 (8), 2501–2518. doi:10.3390/w6082501.
- Ghale, Y., Altunkaynak, A., and Unal, A., 2018. Investigation anthropogenic impacts and climate factors on drying up of Urmia Lake using water budget and drought analysis. Water Resources Management, 32 (1), 325–337. doi:10.1007/s11269-017-1812-5.
- Givati, A., et al. 2019. Climate change impacts on streamflow at the upper Jordan river based on an ensemble of regional climate models. Journal of Hydrology: Regional Studies, 21, 92–109. doi:10.1016/j.ejrh.2018.12.004.
- Givati, A. and Rosenfeld, D., 2007. Possible impacts of anthropogenic aerosols on water resources of the Jordan river and the Sea of Galilee. Water Resources Research, 43 (10). doi:10.1029/2006wr005771.
- Givati, A. and Rosenfeld, D., 2009. Comments on “does air pollution really suppress precipitation in Israel?”. Journal of Applied Meteorology and Climatology, 48 (8), 1733–1750. doi:10.1175/2009jamc1902.
- Gleick, P.H., et al., 2010. Climate change and the integrity of science. Science, 328 (5979), 689. doi:10.1126/science.328.5979.689.
- Gober, P. and Wheater, H.S., 2015. Debates—perspectives on socio-hydrology: modeling flood risk as a public policy problem. Water Resources Research, 51 (6), 4782–4788. doi:10.1002/2015WR016945.
- Goodland, R., 1995. The concept of environmental sustainability. Annual Review of Ecology and Systematics, 26 (1), 1–24. doi:10.1146/annurev.es.26.110195.000245.
- Gophen, M., 2019. Climate change and water loss in the Kinneret drainage basin. Land Use Policy, 80, 424–429. doi:10.1016/j.landusepol.2018.03.00
- Gotmark, F., Cafaro, P., and O’sullivan, J., 2018. Aging human populations: good for us, good for the earth. Trends in Ecology and Evolution, 33 (11), 851–862. doi:10.1016/j.tree.2018.08.015.
- Goulden, M., Conway, D., and Persechino, A., 2010. Adaptation to climate change in international river basins in Africa: a review/adaptation au changement climatique dans les bassins fluviaux internationaux en Afrique: Une revue. Hydrological Sciences Journal, 54 (5), 805–828. doi:10.1623/hysj.54.5.805.
- Graf, W.L., 1999. Dam nation: a geographic census of American dams and their large-scale hydrologic impacts. Water Resources Research, 35 (4), 1305–1311. doi:10.1029/1999WR900016.
- Grant, S., Tamason, C.C., and Jensen, P.K.M., 2015. Climatization: a critical perspective of framing disasters as climate change events. Climate Risk Management, 10, 27–34. doi:10.1016/j.crm.2015.09.00
- Grech-Madin, C., et al. 2018. Negotiating water across levels: a peace and conflict “toolbox” for water diplomacy. Journal of Hydrology, 559, 100–109. doi:10.1016/j.jhydrol.2018.02.008.
- Grimm, N.B., et al. 1997. Sensitivity of aquatic ecosystems to climatic and anthropogenic changes: the basin and range, American southwest and Mexico. Hydrological Processes, 11 (8), 1023–1041. doi:10.1002/(ISSN)1099-1085.
- Güneralp, B., Güneralp, İ., and Liu, Y., 2015. Changing global patterns of urban exposure to flood and drought hazards. Global Environmental Change, 31, 217–225. doi:10.1016/j.gloenvcha.2015.01.002
- Haddadin, M.J., 2011. Water: triggering cooperation between former enemies. Water International, 36 (2), 178–185. doi:10.1080/02508060.2011.557996.
- Halfon, N., Levin, Z., and Alpert, P., 2009. Temporal rainfall fluctuations in Israel and their possible link to urban and air pollution effects. Environmental Research Letters, 4 (2), 025001. doi:10.1088/1748-9326/4/2/025001.
- Hallegatte, S., et al. 2013. Future flood losses in major coastal cities. Nature Climate Change, 3, 802. doi:10.1038/nclimate1979.
- Hamzekhani, F.G., Saghafian, B., and Araghinejad, S., 2016. Environmental management in Urmia Lake: thresholds approach. International Journal of Water Resources Development, 32 (1), 77–88. doi:10.1080/07900627.2015.1024829.
- Hanasz, P., 2014. Power flows: hydro-hegemony and water conflicts in South Asia. Security Challenges, 10 (3), 95–112.
- Hanson, S., et al., 2010. A global ranking of port cities with high exposure to climate extremes. Climatic Change, 104 (1), 89–111. doi:10.1007/s10584-010-9977-4.
- Hardin, G., 1968. The tragedy of the commons. Science, 162 (3859), 1243. doi:10.1126/science.162.3859.1243.
- Hassanzadeh, E., Zarghami, M., and Hassanzadeh, Y., 2012. Determining the main factors in declining the Urmia Lake level by using system dynamics modeling. Water Resources Management, 26 (1), 129–145. doi:10.1007/s11269-011-9909-8.
- Held, I.M. and Soden, B.J., 2006. Robust responses of the hydrological cycle to global warming. Journal of Climate, 19 (21), 5686–5699. doi:10.1175/jcli3990.1.
- Hendrix, C.S., 2017. A comment on “climate change and the Syrian civil war revisited”. Political Geography, 60, 251–252. doi:10.1016/j.polgeo.2017.06.010
- Hillel, N., et al. 2015. Water quality and discharge of the Lower Jordan river. Journal of Hydrology, 527, 1096–1105. doi:10.1016/j.jhydrol.2015.06.002.
- Hillel, N., et al. 2019. Identifying spatiotemporal variations in groundwater-surface water interactions using shallow pore water chemistry in the lower Jordan river. Advances in Water Resources, 131. doi:10.1016/j.advwatres.2019.103388.
- Hinkel, J., et al., 2014. Coastal flood damage and adaptation costs under 21st century sea-level rise. Proceedings of the National Academy of Sciences USA, 111 (9), 3292–3297. doi:10.1073/pnas.1222469111.
- Hoekstra, A.Y. and Mekonnen, M.M., 2012. The water footprint of humanity. Proceedings of the National Academy of Sciences USA, 109 (9), 3232–3237. doi:10.1073/pnas.1109936109.
- Hoekstra, A.Y. and Wiedmann, T.O., 2014. Humanity’s unsustainable environmental footprint. Science, 344 (6188), 1114–1117. doi:10.1126/science.1248365.
- Hoerling, M., et al., 2012. On the increased frequency of Mediterranean drought. Journal of Climate, 25 (6), 2146–2161. doi:10.1175/jcli-d-11-00296.1.
- Huard, D., 2011. Discussion of “a comparison of local and aggregated climate model outputs with observed data” a black eye for the hydrological sciences journal. Hydrological Sciences Journal, 56 (7), 1330–1333. doi:10.1080/02626667.2011.610758.
- Inbar, M. and Bruins, H.J., 2004. Environmental impact of multi-annual drought in the Jordan Kinneret watershed, Israel. Land Degradation & Development, 15 (3), 243–256. doi:10.1002/ldr.612.
- Islam, T. and Peterson, R.E., 2009. Climatology of landfalling tropical cyclones in Bangladesh 1877–2003. Natural Hazards, 48 (1), 115–135. doi:10.1007/s11069-008-9252-4.
- Jafroudi, M., 2018. Climate change and accommodation of water availability in transboundary rivers: lessons learned from the Guadiana basin. Water Policy, 20 (2), 203–217. doi:10.2166/wp.2018.178.
- Jalili, S., et al., 2012. The influence of large-scale atmospheric circulation weather types on variations in the water level of Lake Urmia, Iran. International Journal of Climatology, 32 (13), 1990–1996. doi:10.1002/joc.2422.
- Jalili, S., Hamidi, S.A., and Namdar Ghanbari, R., 2016. Climate variability and anthropogenic effects on Lake Urmia water level fluctuations, Northwestern Iran. Hydrological Sciences Journal, 61 (10), 1759–1769. doi:10.1080/02626667.2015.1036757.
- Johnston, R. and Smakhtin, V., 2014. Hydrological modeling of large river basins: how much is enough? Water Resources Management, 28 (10), 2695–2730. doi:10.1007/s11269-014-0637-8.
- Kandasamy, J., et al., 2014. Socio-hydrologic drivers of the pendulum swing between agricultural development and environmental health: a case study from Murrumbidgee river basin, Australia. Hydrol. Earth Syst. Sci., 18 (3), 1027–1041. doi:10.5194/hess-18-1027-2014.
- Keeling, C.D., et al. 1976. Atmospheric carbon-dioxide variations at Mauna-Loa observatory, Hawaii. Tellus, 28 (6), 538–551.
- Kelley, C., et al. 2017. Commentary on the Syria case: climate as a contributing factor. Political Geography, 60, 245–247. doi:10.1016/j.polgeo.2017.06.013.
- Kelley, C.P., et al., 2015. Climate change in the fertile crescent and implications of the recent Syrian drought. Proceedings of the National Academy of Sciences USA, 112 (11), 3241–3246. doi:10.1073/pnas.1421533112.
- Khazaei, B., et al. 2019. Climatic or regionally induced by humans? Tracing hydro-climatic and land-use changes to better understand the Lake Urmia tragedy. Journal of Hydrology, 569, 203–217. doi:10.1016/j.jhydrol.2018.12.004.
- Kirchner, J.W., 2017. Science, politics, and rationality in a partisan era. Water Resources Research, 53 (5), 3545–3549. doi:10.1002/2017wr020882.
- Klein, M., 1998. Water balance of the upper Jordan river basin. Water International, 23 (4), 244–248. doi:10.1080/02508069808686778.
- Koutsoyiannis, D., et al., 2009. Climate, hydrology and freshwater: towards an interactive incorporation of hydrological experience into climate research. Hydrological Sciences Journal, 54 (2), 394–405. doi:10.1623/hysj.54.2.394.
- Koutsoyiannis, D., et al., 2010. On the credibility of climate predictions. Hydrological Sciences Journal, 53 (4), 671–684. doi:10.1623/hysj.53.4.671.
- Kundzewicz, Z.W., et al., 2009a. Water and climate projections. Hydrological Sciences Journal, 54 (2), 406–415. doi:10.1623/hysj.54.2.406.
- Kundzewicz, Z.W., et al., 2009b. The implications of projected climate change for freshwater resources and their management. Hydrological Sciences Journal, 53 (1), 3–10. doi:10.1623/hysj.53.1.3.
- Kundzewicz, Z.W. and Stakhiv, E.Z., 2010. Are climate models “ready for prime time” in water resources management applications, or is more research needed? Hydrological Sciences Journal, 55 (7), 1085–1089. doi:10.1080/02626667.2010.513211.
- Lambin, E.F. and Meyfroidt, P., 2011. Global land use change, economic globalization, and the looming land scarcity. Proceedings of the National Academy of Sciences USA, 108 (9), 3465–3472. doi:10.1073/pnas.1100480108.
- Legates, D.R., 2014. Climate models and their simulation of precipitation. Energy & Environment, 25 (6–7), 1163–1175. doi:10.1260/0958-305X.25.6-7.1163.
- Lehner, B. and Döll, P., 2004. Development and validation of a global database of lakes, reservoirs and wetlands. Journal of Hydrology, 296 (1–4), 1–22. doi:10.1016/j.jhydrol.2004.03.028.
- Lehner, B. and Grill, G., 2013. Global river hydrography and network routing: baseline data and new approaches to study the world’s large river systems. Hydrological Processes, 27 (15), 2171–2186. doi:10.1002/hyp.9740.
- Lewis, S.L. and Maslin, M.A., 2015. Defining the Anthropocene. Nature, 519 (7542), 171–180. doi:10.1038/nature14258.
- Liu, H., et al., 2013. Disappearing lakes in semiarid northern China: drivers and environmental impact. Environmental Science & Technology, 47 (21), 12107–12114. doi:10.1021/es305298q.
- Loucks, D.P., 2015. Debates—perspectives on socio-hydrology: simulating hydrologic-human interactions. Water Resources Research, 51 (6), 4789–4794. doi:10.1002/2015WR017002.
- Mach, K.J., et al., 2019. Climate as a risk factor for armed conflict. Nature, 571 (7764), 193–197. doi:10.1038/s41586-019-1300-6.
- Madani, K., 2014. Water management in Iran: what is causing the looming crisis? Journal of Environmental Studies and Sciences, 4 (4), 315–328. doi:10.1007/s13412-014-0182-z.
- Madani, K., Aghakouchak, A., and Mirchi, A., 2016. Iran’s socio-economic drought: challenges of a water-bankrupt nation. Iranian Studies, 49 (6), 997–1016. doi:10.1080/00210862.2016.1259286.
- Maibach, E., Myers, T., and Leiserowitz, A., 2014. Climate scientists need to set the record straight: there is a scientific consensus that human-caused climate change is happening. Earth’s Future, 2 (5), 295–298. doi:10.1002/2013ef000226.
- Manabe, S. and Wetherald, R.T., 1967. Thermal equilibrium of the atmosphere with a given distribution of relative humidity. Journal of the Atmospheric Sciences, 24 (3), 241–259. doi:10.1175/1520-0469(1967)024<0241:TEOTAW>2.0.CO;2.
- Maxwell, R.M. and Kollet, S.J., 2008. Interdependence of groundwater dynamics and land-energy feedbacks under climate change. Nature Geoscience, 1, 665. doi:10.1038/ngeo315
- Mcgranahan, G., Balk, D., and Anderson, B., 2016. The rising tide: assessing the risks of climate change and human settlements in low elevation coastal zones. Environment and Urbanization, 19 (1), 17–37. doi:10.1177/0956247807076960.
- Meier, J., Zabel, F., and Mauser, W., 2018. A global approach to estimate irrigated areas—a comparison between different data and statistics. Hydrology and Earth System Sciences, 22 (2), 1119–1133. doi:10.5194/hess-22-1119-2018.
- Mekonnen, M.M. and Hoekstra, A.Y., 2016. Four billion people facing severe water scarcity. Science Advances, 2 (2), e1500323. doi:10.1126/sciadv.1500323.
- Meng, Q., 2019. Climate change and extreme weather drive the declines of saline lakes: a showcase of the Great Salt Lake. Climate, 7 (2), 19. doi:10.3390/cli7020019.
- Micklin, P., 2007. The Aral Sea disaster. Annual Review of Earth and Planetary Sciences, 35, 47–72.
- Micklin, P., 2016. The future Aral Sea: hope and despair. Environmental Earth Sciences, 75 (9). doi:10.1007/s12665-016-5614-5.
- Micklin, P.P., 1988. Desiccation of the Aral Sea - a water management disaster in the Soviet-Union. Science, 241 (4870), 1170–1175. doi:10.1126/science.241.4870.1170.
- Mirza, M.M.Q., 1998. Diversion of the Ganges water at Farakka and its effects on salinity in Bangladesh. Environmental Management, 22 (5), 711–722. doi:10.1007/s002679900141.
- Mirza, M.M.Q., 2003. Climate change and extreme weather events: can developing countries adapt? Climate Policy, 3 (3), 233–248. doi:10.3763/cpol.2003.0330.
- Momblanch, A., et al. 2019b. Untangling the water-food-energy-environment nexus for global change adaptation in a complex Himalayan water resource system. The Science of the Total Environment, 655, 35–47. doi:10.1016/j.scitotenv.2018.11.045.
- Momblanch, A., Holman, I.P., and Jain, S.K., 2019a. Current practice and recommendations for modelling global change impacts on water resource in the Himalayas. Water, 11 (6), 1303. doi:10.3390/w11061303.
- Moore, J.N., 2016. Recent desiccation of western Great Basin saline lakes: lessons from Lake Abert, Oregon, U.S.A. Science of the Total Environment, 554–555, 142–154. doi:10.1016/j.scitotenv.2016.02.161
- Morin, E., et al., 2018. Mean, variance, and trends of Levant precipitation over the past 4500 years from reconstructed Dead Sea levels and stochastic modeling. Quaternary Research, 91 (2), 751–767. doi:10.1017/qua.2018.98.
- Muller, M.F., et al., 2016. Impact of the Syrian refugee crisis on land use and transboundary freshwater resources. Proceedings of the National Academy of Sciences USA, 113 (52), 14932–14937. doi:10.1073/pnas.1614342113.
- Müller, M.F. and Levy, M.C., 2019. Complementary vantage points: integrating hydrology and economics for sociohydrologic knowledge generation. Water Resources Research, 55 (4), 2549–2571. doi:10.1029/2019WR024786.
- Müller, M.F., Müller-Itten, M.C., and Gorelick, S.M., 2017. How Jordan and Saudi Arabia are avoiding a tragedy of the commons over shared groundwater. Water Resources Research, 53 (7), 5451–5468. doi:10.1002/2016wr020261.
- Nazeri Tahroudi, M., Ramezani, Y., and Ahmadi, F., 2019. Investigating the trend and time of precipitation and river flow rate changes in Lake Urmia Basin, Iran. Arabian Journal of Geosciences, 12 (6), 219. doi:10.1007/s12517-019-4373-5.
- Nissan, H., et al. 2019. On the use and misuse of climate change projections in international development. Wiley Interdisciplinary Reviews: Climate Change, 10 (3). doi:10.1002/wcc.579.
- Nohara, D., et al., 2006. Impact of climate change on river discharge projected by multimodel ensemble. Journal of Hydrometeorology, 7 (5), 1076–1089. doi:10.1175/JHM531.1.
- Nourani, V., et al., 2018. Investigating the effect of hydroclimatological variables on Urmia Lake water level using wavelet coherence measure. Journal of Water and Climate Change, 10 (1), 13–29. doi:10.2166/wcc.2018.261.
- Oreskes, N., 2004. The scientific consensus on climate change. Science, 306 (5702), 1686. doi:10.1126/science.1103618.
- Petersen-Perlman, J.D., Veilleux, J.C., and Wolf, A.T., 2017. International water conflict and cooperation: challenges and opportunities. Water International, 42 (2), 105–120. doi:10.1080/02508060.2017.1276041.
- Pindyck, R.S., 2017. The use and misuse of models for climate policy. Review of Environmental Economics and Policy, 11 (1), 100–114. doi:10.1093/reep/rew012.
- Platt, J.R., 1964. Strong inference. Science, 146 (3642), 347. doi:10.1126/science.146.3642.347.
- Rimmer, A., et al., 2011. Using ensemble of climate models to evaluate future water and solutes budgets in Lake Kinneret, Israel. Journal of Hydrology, 410 (3–4), 248–259. doi:10.1016/j.jhydrol.2011.09.025.
- Rockström, J., et al. 2014. The unfolding water drama in the Anthropocene: towards a resilience based perspective on water for global sustainability. Ecohydrology. doi:10.1002/eco.1562.
- Rodell, M., et al., 2018. Emerging trends in global freshwater availability. Nature, 557 (7707), 651–659. doi:10.1038/s41586-018-0123-1.
- Salimi, J., Maknoon, R., and Meijerink, S., 2019. Designing institutions for watershed management: a case study of the Urmia Lake restoration national committee. Water Alternatives, 12 (2), 609–635.
- Samuels, R., et al., 2010. Climate change impacts on Jordan river flow: downscaling application from a regional climate model. Journal of Hydrometeorology, 11 (4), 860–879. doi:10.1175/2010jhm1177.1.
- Scafetta, N., 2012. Testing an astronomically based decadal-scale empirical harmonic climate model versus the IPCC (2007) general circulation climate models. Journal of Atmospheric and Solar-Terrestrial Physics, 80, 124–137. doi:10.1016/j.jastp.2011.12.005
- Scafetta, N., 2013. Discussion on climate oscillations: CMIP5 general circulation models versus a semi-empirical harmonic model based on astronomical cycles. Earth-Science Reviews, 126, 321–357. doi:10.1016/j.earscirev.2013.08.008
- Scanlon, B.R., et al., 2017. The food-energy-water nexus: transforming science for society. Water Resources Research, 53 (5), 3550–3556. doi:10.1002/2017wr020889.
- Schmeier, S. and Shubber, Z., 2018. Anchoring water diplomacy – the legal nature of international river basin organizations. Journal of Hydrology, 567, 114–120. doi:10.1016/j.jhydrol.2018.09.054
- Schwartz, S.E., et al., 2010. Why hasn’t earth warmed as much as expected? Journal of Climate, 23 (10), 2453–2464. doi:10.1175/2009jcli3461.1.
- Selby, J., et al. 2017a. Climate change and the Syrian civil war revisited: a rejoinder. Political Geography, 60, 253–255. doi:10.1016/j.polgeo.2017.08.001.
- Selby, J., et al. 2017b. Climate change and the Syrian civil war revisited. Political Geography, 60, 232–244. doi:10.1016/j.polgeo.2017.05.007.
- Shadkam, S., et al., 2016a. Impacts of climate change and water resources development on the declining inflow into Iran’s Urmia Lake. Journal of Great Lakes Research, 42 (5), 942–952. doi:10.1016/j.jglr.2016.07.033.
- Shadkam, S., et al. 2016b. Preserving the world second largest hypersaline lake under future irrigation and climate change. Science of the Total Environment, 559, 317–325. doi:10.1016/j.scitotenv.2016.03.190.
- Shentsis, I., et al. 2019. Assessing water consumption and aquifer discharge through springs based on the joint use of rain and flow data in the Yarmouk river basin. Environmental Earth Sciences, 78 (12). doi:10.1007/s12665-019-8359-0.
- Siebert, S., et al., 2010. Groundwater use for irrigation – a global inventory. Hydrology and Earth System Sciences, 14 (10), 1863–1880. doi:10.5194/hess-14-1863-2010.
- Sivapalan, M., et al., 2014. Socio-hydrology: use-inspired water sustainability science for the Anthropocene. Earth’s Future, 2 (4), 225–230. doi:10.1002/2013ef000164.
- Sivapalan, M., 2015. Debates—perspectives on socio-hydrology: changing water systems and the “tyranny of small problems”—socio-hydrology. Water Resources Research, 51 (6), 4795–4805. doi:10.1002/2015WR017080.
- Sivapalan, M., Savenije, H.H.G., and Blöschl, G., 2012. Socio-hydrology: a new science of people and water. Hydrological Processes, 26 (8), 1270–1276. doi:10.1002/hyp.8426.
- Smiatek, G. and Kunstmann, H., 2016. Expected future runoff of the upper Jordan river simulated with a CORDEX climate data ensemble. Journal of Hydrometeorology, 17 (3), 865–879. doi:10.1175/jhm-d-15-0066.1.
- Stetter, S., et al., 2011. Conflicts about water: securitizations in a global context. Cooperation and Conflict, 46 (4), 441–459. doi:10.1177/0010836711422462.
- Stockwell, D.R.B., 2010. Critique of drought models in the Australian drought exceptional circumstances report (decr). Energy & Environment, 21 (5), 425–436. doi:10.1260/0958-305x.21.5.425.
- Tal, A., 2017. Will demography defeat river rehabilitation efforts? The case of the river Jordan. Water Research, 111, 404–419. doi:10.1016/j.watres.2016.11.047
- Tal, A., 2018. Addressing desalination’s carbon footprint: the Israeli experience. Water, 10 (2), 197. doi:10.3390/w10020197.
- Tal, A., 2019a. Climate change’s impact on Lake Kinneret: letting the data tell the story. Science of the Total Environment, 685, 1272–1275. doi:10.1016/j.scitotenv.2019.05.282
- Tal, A., 2019b. The implications of climate change driven depletion of Lake Kinneret water levels: the compelling case for climate change-triggered precipitation impact on Lake Kinneret’s low water levels. Science of the Total Environment, 664, 1045–1051. doi:10.1016/j.scitotenv.2019.02.106
- Tal, A., 2019c. Letter to the editor regarding Wine et al. (2019): lake Kinneret and climate change. Science of the Total Environment, 664, 175–176. doi:10.1016/j.scitotenv.2019.01.371
- Tao, S., et al., 2015. Rapid loss of lakes on the Mongolian Plateau. Proceedings of the National Academy of Sciences USA, 112 (7), 2281–2286. doi:10.1073/pnas.1411748112.
- Taravat, A., et al. 2016. A spaceborne multisensory, multitemporal approach to monitor water level and storage variations of lakes. Water, 8 (11), 478. doi:10.3390/w8110478
- Thenkabail, P., et al., 2009. Irrigated area maps and statistics of India using remote sensing and national statistics. Remote Sensing, 1 (2), 50–67. doi:10.3390/rs1020050.
- Tian, F., et al., 2019. Dynamics and driving mechanisms of asymmetric human water consumption during alternating wet and dry periods. Hydrological Sciences Journal, 64 (5), 507–524. doi:10.1080/02626667.2019.1588972.
- Tourian, M.J., et al. 2015. A spaceborne multisensor approach to monitor the desiccation of Lake Urmia in Iran. Remote Sensing of Environment, 156, 349–360. doi:10.1016/j.rse.2014.10.006.
- Troy, T.J., et al., 2015a. Moving sociohydrology forward: a synthesis across studies. Hydrology and Earth System Sciences, 19 (8), 3667–3679. doi:10.5194/hess-19-3667-2015.
- Troy, T.J., Pavao-Zuckerman, M., and Evans, T.P., 2015b. Debates—perspectives on socio-hydrology: socio-hydrologic modeling: tradeoffs, hypothesis testing, and validation. Water Resources Research, 51 (6), 4806–4814. doi:10.1002/2015WR017046.
- Van Dijk, A.I.J.M., et al., 2014. A global water cycle reanalysis (2003–2012) merging satellite gravimetry and altimetry observations with a hydrological multi-model ensemble. Hydrology and Earth System Sciences, 18 (8), 2955–2973. doi:10.5194/hess-18-2955-2014.
- Vörösmarty, C., et al., 2004. Humans transforming the global water system. Eos, Transactions American Geophysical Union, 85 (48), 509–514. doi:10.1029/2004eo480001.
- Vorosmarty, C.J., et al., 2010. Global threats to human water security and river biodiversity. Nature, 467 (7315), 555–561. doi:10.1038/nature09440.
- Vörösmarty, C.J., et al. 1997. The storage and aging of continental runoff in large reservoir systems of the world. Ambio, 26 (4), 210–219.
- Vörösmarty, C.J., et al., 2013. Global water, the anthropocene and the transformation of a science. Current Opinion in Environmental Sustainability, 5 (6), 539–550. doi:10.1016/j.cosust.2013.10.005.
- Vörösmarty, C.J. and Sahagian, D., 2000. Anthropogenic disturbance of the terrestrial water cycle. Bioscience, 50 (9), 753–765. doi:10.1641/0006-3568(2000)050[0753:ADOTTW]2.0.CO;2.
- Wang, J., et al. 2018. Recent global decline in endorheic basin water storages. Nature Geoscience, 11, 926–932. doi:10.1038/s41561-018-0265-7.
- Ward, F.A., 2013. Forging sustainable transboundary water-sharing agreements: barriers and opportunities. Water Policy, 15 (3), 386–417. doi:10.2166/wp.2012.003.
- Warren, S.G., 2015. Can human populations be stabilized? Earth’s Future, 3 (2), 82–94. doi:10.1002/2014ef000275.
- Warren, S.G., 2016. Reply to comment by John Colarusso on “can human populations be stabilized?”. Earth’s Future, 4 (2), 18–19. doi:10.1002/2015EF000341.
- Werrell, C.E., Femia, F., and Sternberg, T., 2015. Did we see it coming? State fragility, climate vulnerability, and the uprisings in Syria and Egypt. SAIS Review of International Affairs, 35 (1), 29–46. doi:10.1353/sais.2015.0002.
- Wesselink, A., et al., 2014. Equipped to deal with uncertainty in climate and impacts predictions: lessons from internal peer review. Climatic Change, 132 (1), 1–14. doi:10.1007/s10584-014-1213-1.
- Wijngaard, R.R., et al., 2018. Climate change vs. Socio-economic development: understanding the future south Asian water gap. Hydrology and Earth System Sciences, 22 (12), 6297–6321. doi:10.5194/hess-22-6297-2018.
- Wilby, R.L., 2005. Uncertainty in water resource model parameters used for climate change impact assessment. Hydrological Processes, 19 (16), 3201–3219. doi:10.1002/hyp.5819.
- Wilcox, B.P., Sorice, M.G., and Young, M.H., 2011. Dryland ecohydrology in the Anthropocene: taking stock of human–ecological interactions. Geography Compass, 5 (3), 112–127. doi:10.1111/j.1749-8198.2011.00413.x.
- Wine, M.L., 2019a. Letter to editor re Tal (2019): climaticization of environmental degradation—an Anthropocene Epoch response to failure of governance. Science of the Total Environment, 685, 1269–1271. doi:10.1016/j.scitotenv.2019.04.343
- Wine, M.L., 2019b. Response to comment on “agriculture, diversions, and drought shrinking Galilee Sea”. Science of the Total Environment, 663, 436–437. doi:10.1016/j.scitotenv.2019.01.372
- Wine, M.L., 2019c. There is no black hole swallowing water in the Hula valley. Land Use Policy, 84, 363–364. doi:10.1016/j.landusepol.2019.02.046
- Wine, M.L., 2019d. Toward strong science to support equitable water sharing in securitized transboundary watersheds. Biologia, doi:10.2478/s11756-019-00334-8.
- Wine, M.L., 2019e. Under non-stationarity securitization contributes to uncertainty and tragedy of the commons. Journal of Hydrology, 568, 716–721. doi:10.1016/j.jhydrol.2018.11.044
- Wine, M.L., et al. 2019. Climatization—negligent attribution of Great Salt Lake desiccation: a comment on Meng (2019). Climate, 7 (5), 67. doi:10.3390/cli7050067
- Wine, M.L., Cadol, D., and Makhnin, O., 2018. In ecoregions across western USA streamflow increases during post-wildfire recovery. Environmental Research Letters, 13 (1), 1–14. doi:10.1088/1748-9326/aa9c5a.
- Wine, M.L. and Davison, J.H., 2019. Untangling global change impacts on hydrological processes: resisting climatization. Hydrological Processes, In Press, 33, 2148–2155. doi:10.1002/hyp.13483.
- Wine, M.L., Rimmer, A., and Laronne, J.B., 2019b. Agriculture, diversions, and drought shrinking Galilee Sea. Science of the Total Environment, 651, 70–83. doi:10.1016/j.scitotenv.2018.09.058
- Wurtsbaugh, W.A., et al., 2017. Decline of the world’s saline lakes. Nature Geoscience, 10 (11), 816–821. doi:10.1038/ngeo3052.
- Yu, J., et al., 2015. Power asymmetry in conflict resolution with application to a water pollution dispute in China. Water Resources Research, 51 (10), 8627–8645. doi:10.1002/2014wr016257.
- Zeitoun, M., et al. 2019. Analysis for water conflict transformation. Water International, 1–20. doi:10.1080/02508060.2019.1607479.
- Zeitoun, M. and Warner, J., 2006. Hydro-hegemony – a framework for analysis of trans-boundary water conflicts. Water Policy, 8 (5), 435–460. doi:10.2166/wp.2006.054.
- Zheng, J.J., et al. 2016. Monitoring changes in the water volume of Hulun lake by integrating satellite altimetry data and Landsat images between 1992 and 2010. Journal of Applied Remote Sensing, 10, 14. doi:10.1117/1.Jrs.10.016029.