ABSTRACT
Background
There is a growing view that ‘Big Ideas of science education’ are useful for teaching science but there is not much knowledge of how teachers work with them.
Purpose
This study explores the conceptualisation and practice of the use of Big Ideas of science education by primary and secondary teachers in Chile.
Sample
A total of 63 science teachers (a purposive sample) from pre-school, primary and secondary education in Valparaíso Region in Chile participated in the study, with 38 of them answering all the questions in the research instrument and 25 answering some of them.
Design and methods
The research instrument was a questionnaire with open-ended questions.
Results
The use of Big Ideas was seen as the ‘natural way’ to teach science, mostly related to the students’ daily lives. Many of the teachers had their own understanding of Big Ideas. They were very positive about Big Ideas, seeing them as a possible way of connecting with the daily lives of students and facilitating progression in students’ learning of science. The teachers also saw Big Ideas as enabling students to work collaboratively and make links between different parts of the curriculum, helping them to understand how science works, and preferable to having to teach an overloaded science curriculum that lacks such an organising framework.
Conclusion
The teachers were more interested in their own creation and development of Big Ideas rather than simply adopting the existing, official published framework and adhering to what is said in the Chilean curriculum regarding the approach of Big Ideas. These results indicate the need to explore in-depth such varied conceptualisations of schoolteachers regarding the approach of Big Ideas. In turn, this can offer empirical insights into the way Big Ideas are treated in policy documents in Chile and elsewhere.
Introduction
It is widely recognised that teaching and learning science is challenging from the perspectives of both teachers and students. From the perspective of schoolteachers, teaching science is a complex task (Loughran, Berry, and Mulhall Citation2012). In terms of curriculum, teachers find it demanding as in almost all countries they are expected to teach very large amounts of knowledge each academic year (e.g. Metz Citation2012). In addition, science curricula have been critiqued for lacking internal connections (the analytical review of Duschl, Maeng, and Sezen Citation2011) and manifesting a lack of progression between primary and secondary education (Harlen Citation2015a). Too often, school science teaching consists of teaching isolated facts and events where the content can be reduced to relative trivia instead of the possibility of developing skills or core ideas (Olson Citation2008). When the science curriculum is presented to classrom teachers in a rigid, predetermined form, teachers lack a sense of ownership, which makes it more difficult for them to teach it in the way it was intended (Ogborn Citation2002).
From the perspective of students, Osborne (Citation2007) argues that concentrating on facts or isolated events distracts students from seeing the beauty of science. In this sense, positive attitudes towards science could decrease because science is shown simply as atomistic events, so that students ‘may miss the big picture’ (Reiss Citation2014, 9). According to Osborne (Citation2007), the needs of the current citizen are related to understanding how and why the knowledge is relevant or is related to other explanations, rather than just facts. In Osborne’s view, there are some fallacies all too often associated with learning science, namely that it can only proceed fact by fact, that the whole body of scientific knowledge needs to be taught (at a level approach for the age range), and that scientific knowledge promotes critical thinking almost by osmosis. In addition, it is a mistake to presume that there exists just one science curriculum, without considering the diversity of students’ abilities, backgrounds or interests. It has been argued that this can result in a lack of student motivation, which can detrimentally affect their attitudes towards science (Osborne, Simon, and Collins Citation2003a; Juan et al. Citation2016).
In trying to address these problems, there has been a growing move among curriculum developers arguing that science education should consider ‘Big Ideas’ as ideas that are able to explain a wide range of scientific facts and phenomena (Metz Citation2012). These ‘ideas enable learners to see connections between different scientific ideas’ and when these are connected, it is easier to use them in new scenarios than other, unconnected ones (Harlen Citation2015a, 97). Despite these possible changes in the intended curriculum, what is perhaps more important is how teachers use such guidelines in their teaching (Reiss Citation2014), indeed, how they can create these changes. According to Ogborn (Citation2002, 143):
One of the strongest conclusions to come out of decades of studies of the success and failure of a wide variety of curriculum innovations is that innovations succeed when teachers feel a sense of ownership of the innovation: that it belongs to them and is not simply imposed on them.
Nevertheless, the notion of teachers generating pedagogically powerful Big Ideas seems to have been lost (Mitchell et al. Citation2016) when the Big Ideas come directly from the discipline of science without considering the actual realities of the classroom. To that extent, there is something missing in the literature: ‘the teacher’s voice’ – which should be heard and theorised by them.
To sum up, there is a growing view that Big Ideas are useful for teaching science (and they have been developed for other subjects too – e.g. Wintersgill et al. Citation2017) but there is limited knowledge of how science teachers work with them in the classroom and how teachers can create/redesign collaboratively their own ‘Big Ideas curriculum’, related to the context – the students, the type of school they work in and their own knowledge and views of science. Addressing this gap, this study explores the intentions and practices in relation to the Big Ideas of science education of science teachers in Chile, thus contributing to knowledge about teaching science through Big Ideas.
Science curriculum and its changes
Many science educators have wondered what is the aim of science education? For some, there is quite a broad consensus that one of the aims is to achieve greater scientific literacy of the population, which means producing citizens to be informed and able to participate actively in a modern, democratic and technological society (Holbrook and Rannikmae Citation2009). Another argument is that the aim of science education, as of education in general, is related to two ends: to ‘lead a life that is personally flourishing and to help others to do so too’ (Reiss Citation2014, 10). A number of other aims of science education have also been proposed (Taber Citation2017; Mansfield and Reiss Citation2020). It is evident that science education has many possible aims. Therefore, it is important that a science curriculum identifies its aims and is coherent with them.
Hollins and Reiss (Citation2016) undertook a review of the science curriculum in eleven different (mostly high-performing) jurisdictions so as to analyse the curriculum in terms of context, coherence and clarity, scope, progression, levels of demand, and assessment. At the start of its documentation, every curriculum stated that it was making changes and there proved to be certain similarities between the curricula in terms of their aims, such as in relation to scientific literacy, inquiry, links between science and technology, and personal and social goals. Author concluded that science curricula are changing across the globe, that there are different ways to develop a curriculum, and that these eleven jurisdictions have both differences and commonalities in the aims they promote.
Some of these changes in the curriculum and in associated pedagogy have been proposed due to the increasingly negative attitudes towards science that most students display as they go through their secondary schooling (Osborne et al. Citation2003b; Barmby, Kind, and Jones Citation2008; Khine Citation2015). Related to the aforementioned changes, in South Korea a new framework for scientific literacy was created with five dimensions: (i) content knowledge (based on Big Ideas); (ii) habits of mind; (iii) character and values; (iv) science as a human endeavour; and (v) metacognition and self-direction (Choi et al. Citation2011). An interesting point related to the first dimension is that the researchers surveyed teachers, asking about the importance of these aspects of scientific literacy and found that teachers declared that there was a lack of understanding of the importance of both Big Ideas and integrated approaches to teaching science (Choi et al. Citation2011). Likewise, in Australia there is a new approach to the science curriculum, focused on Big Ideas and its principles (Mitchell et al. Citation2016). As in the South Korean case, after asking teachers about those changes, the teachers recognised that they had more understanding of the traditional approach to a science curriculum than of a Big Ideas approach. Mitchell et al. (Citation2016) stated that the opportunity was lost to show teachers how they can re-conceptualise their teaching to see content as more connected and so enhance the learning of their students. In Chile too, the new approach of the science curriculum is focused on Big Ideas of science education. For levels 1 to 6, Big Ideas are specified as an overarching aim (Ministerio de Educación de Chile Citation2012), levels 7 to 10 include Big Ideas of science, and levels 11 and 12 include Big Ideas of and about science (the ‘of/about’ distinction being made in the original, 2010 Big Ideas report) (Ministerio de Educación de Chile Citation2015).
Due to the on-going changes in a number of countries that are moving to organise their science curricula around Big Ideas, as exemplified in the preceding paragraph, it is important to know what teachers’ notions of Big Ideas are before teachers are expected to implement them in their teaching. Ogborn (Citation2002) shows us a similar dilemma as a curriculum developer by presenting two questions. The first is about the ownership of a new curriculum, once it has been developed, and the second is about the transformation of this curriculum in terms of the possibility of it being taught in the way that was intended by the curriculum developer. Ogborn concludes that the success of a curriculum development project is related to ‘the sense of ownership of the innovation’ (143). According to Ogborn, there is a need for a ‘guiding vision’ which can give a global view, understanding such a vision to be as flexible as possible for teachers. Ogborn concludes that ‘teachers, not the developers, are the true owners of a curriculum development’ and, in terms of the transformation, he says ‘there is in a way no such thing as material being taught in the way intended’ (146). It is important to acknowledge the teamwork between curriculum developers and the people who can put a curriculum into action, namely teachers with their students.
Big Ideas of science education: definition from academia
In a number of countries, current changes in the science curriculum are related to the Big Ideas of science education. However, there are different definitions and experiences trying to address what these Big Ideas are. The origin of the term is that a group of science educators, from different countries, under the leadership of Wynne Harlen, developed a set of key ideas with the intention to promote in students the understanding of the natural world. This team produced two reports: Principles and Big Ideas of Science Education (Harlen Citation2010) and Working with Big Ideas of Science Education (Harlen Citation2015b). The purpose of their work was to address the problem of the negative perception by students towards science by enabling teachers to teach students these key ideas (Harlen Citation2010, Citation2015a, Citation2015b).
In Sweden, there is a new curriculum focused on demonstrating achieved skills. According to Mutvei and Mattsson (Citation2015), every item of content of that curriculum can be related to a Big Idea, understanding that term as a core idea regarding content. According to Mitchell et al. (Citation2016), in Australia, Big Ideas are ‘a unifying principle that connects and organises a number of smaller ideas or concepts and multiple experiences’ (3); furthermore, these kinds of ideas are powerful (cf. Young Citation2008) because they give a direction to teaching and learning. Mitchell et al. (Citation2016) also offer other definitions of Big Ideas, such as the one from Mitchell et al. (Citation2016, 3) where ‘key ideas are full standalone statements, which give a sense of enduring understandings that students need to develop’ (Mitchell et al. Citation2016, 3) or in terms of Mitchell et al. (Citation2016, 3) who states that Big Ideas ‘can be thought of as the meaningful patterns that enable one to connect the dots of otherwise fragmented knowledge’ (Mitchell et al. Citation2016, 3). For others, a Big Idea is not simply just a part of the field; it needs to make connections across the subject and be generative for students, allowing them to make new connections across the content they are studying (Olson Citation2008; Smith and Girod Citation2003). Big Ideas are common to more than one phenomenon and have the potential to become more sophisticated over time (Plummer and Krajcik Citation2010). In the USA, the definition of Big Ideas came from the American Association for the Advancement of Science’s (AAAS) Project 2061 which established ‘topics of importance for literacy in science, mathematics, and technology’ (Lelliott and Rollnick Citation2010, 1173) and more recently from the NGSS (Next Generation Science Standards) released in 2013 and related to the third part of the Framework for K-12 Science education: practices, crosscutting concepts and core ideas (Bybee Citation2014). The A Framework for K–12 Science Education tries to address the problem of coverage and depth with transversal core ideas in the curriculum (Chalmers et al. Citation2017; Metz Citation2012).
Recent research is attempting to integrate the concept of Big Ideas within and across STEM (Science, Technology, Engineering and Mathematics) disciplines. The proposal is to use Big Ideas of STEM in terms of ideas that can be applied in other disciplines, Big Ideas that are crosscutting, and Big Ideas that are integrated. The understanding here is that ‘Big Ideas refer to key ideas that link numerous discipline understandings into coherent wholes’ (Chalmers et al. Citation2017, 3). Big Ideas of STEM can be Big Ideas of content or Big Ideas of the processes of STEM subjects. Additionally, Chalmers et al. (Citation2017) state that Big Ideas can be Big Ideas about STEM – related to how knowledge is created, evaluated and advanced.
It is evident that there are an increasing number of ways in which Big Ideas are used in the literature. In addition to the usage referred to above, there are Big Ideas about the content, learning and domains of science (Mitchell et al. Citation2016), Big Ideas about homeostasis, evolution, information, interactions, and emergent properties (Cooper Citation2015), Big Ideas about astronomy in terms of the Earth-Sun-Moon system (Lelliott and Rollnick Citation2010; Plummer and Krajcik Citation2010), Big Ideas about all of science (Devick-Fry and LeSage Citation2010), Big Ideas about mathematical thinking, representation, generalisation, pattern and proportionality (Lord and Kiddle Citation2016) and Big Ideas about the Nature of Science (Osborne et al. Citation2003b). Each of these approaches tries to contribute to improving students’ learning of science.
A core advantage of using Big Ideas is that this should allow students to make connections between scientific concepts (Metz Citation2012). When these concepts are connected, it should be easier for students to use them in new scenarios than other, unconnected concepts (Harlen Citation2015a). Also, students should see the relation between different scientific ideas and daily life, motivating them for this new understanding (Harlen Citation2015a). Students should be able to build connections and recognise patterns in different phenomena (Harlen Citation2015a; Chalmers et al. Citation2017) and develop a more sophisticated level of scientific understanding (Plummer and Krajcik Citation2010). For teachers, organising teaching topics according to Big Ideas should enable a deeper understanding and help them to make links between different activities (Mitchell et al. Citation2016; Cartier and Pellathy Citation2009).
Given the increasing diversity of ways in which the term ‘Big Ideas in science education’ is being understood and used, one possibility would be for us to propose a single way (a definition) in which the term can be conceptualised and employed. While such an approach might add clarity, it is not our focus here. For the reasons adduced by Ogborn (Citation2002), which resulted from his experience of co-leading a large school physics curriculum development project, our interest is rather in how teachers work with Big Ideas in their classrooms, looking at their understandings and practices. Such a focus seems to have been missing in the literature to date yet is likely to be vital if the potential of Big Ideas is to be realised for students who are learning science.
Science teachers and the science curriculum
Teachers’ practice is the most powerful influence on students’ learning (Hattie Citation2008; Windschitl et al. Citation2012). This practice is influenced by the curriculum which gives teachers guidelines for teaching, providing the aims, content and sometimes even resources. Usually, teachers do not restructure the curriculum – they simply reproduce the content contained within it; at the most, they make additions or deletions or re-sequence this content (Smith and Girod Citation2003). However, teachers are called to ‘reinterpret the fundamental concepts and methods of the respective disciplines in accessible, engaging, and powerful ways for students’ (Smith and Girod Citation2003, 295). In our experience, science teachers in many countries typically do not have the training (whether pre-service or later) or sense of ownership in their day-to-day work for them to make such changes to the curriculum.
In Chile, the new science curriculum, structured around Big Ideas, and a new law that has created a system of teachers’ professional development, the declarative aim of which is the autonomy of teachers, provides an opportunity for deep curriculum engagement by teachers. The theme of teacher autonomy is an important one in Chile, where memories of the right-wing, military dictatorship that ruled the country from 1973 to 1990 are still fresh. Indeed, the OECD 2018 Teaching and Learning International Survey (TALIS) found that in Chile, 91% of teachers report having control over determining course content in their class, compared to 84% on average across the OECD countries and economies participating in TALIS (OECD Citation2020). During the dictatorship period, and particularly from 1980, the dictatorship implemented different reforms to the educational system in terms of funding and management of the school system, becoming an extreme system regulated by market mechanisms (Ahumada et al. Citation2009; Cox Citation2012). During the dictatorship, in the neoliberal environment the role of the teachers was impoverished due to low salaries and changes in the curriculum. The decisions made in this period did not consider the teacher experience and/or just used their voice as a consultant but without allowing time for reflection and informed opinion (Donoso Citation2005). According to Cox (Citation2012), the four governments that followed the dictatorship tried to implement educational policies focused on quality and equity. Despite this period being called ‘return to the democracy’, these governments did not change the law created in the dictatorship (LOCE) under the same idea of funding and institutional organisation (Donoso Citation2005). In 2006, the discomfort of secondary students was indicated by an extensive strike all over the country, with massive support from the general public; those striking were seeking the derogation of the LOCE and crucial changes in the funding system of public schools. As a result, President Michelle Bachelet created the Presidential Advisory Council on Education with the principal actors of the educational system and representatives of the student movement. After many conflicts, mostly related to the left-wing party trying to remove the element of profit in education and the right-wing party trying to keep it, the Council reached agreement in derogating the LOCE. Thus, in 2009 the new law called the General Law of Education (LGE) was enacted; in 2011, the Law of Quality Assurance of Education was enacted (Cox Citation2012) and in 2016, the Law for Strengthening Public Education was enacted. The implementation of these laws seeks to redefine the role of the public education in Chile, bringing public schools back into state hands. Under this new law a system of teacher professional development was created with the aim of boosting the autonomy of teachers. Potentially, such teacher autonomy could create the space for science teachers to engage in that reinterpretation and reorganisation of the curriculum. However, the question is, do science teachers in Chile have the power and conditions to change the curriculum, based on their context, their students, their knowledge and understanding of science and their schools?
The current curriculum in Chile and the place of Big Ideas
In Chile, the National Curriculum for primary and secondary education states that the focus of science education is the scientific literacy of students so that they can participate in an informed way in the decisions and actions that affect their own wellbeing and the wellbeing of society (Ministerio de Educación de Chile Citation2012, Citation2015, Citation2019). In those same documents, the Ministry declared that in order to cover the huge amount of scientific knowledge, students should be shown science in an integrated way and that, to take advantage of the limited time for learning, science education should consider the ‘Big Ideas’ approach proposed by Harlen (Citation2010) and her group of science educators and scientists from different parts of the world, including Chile.
There is a new curricular document for levels 11 and 12 jointly launched in 2017 by the Curriculum and Assessment Sections in the Ministry of Education and approved in September 2019 which presents the current version of the school science curriculum in Chile (Ministerio de Educación de Chile Citation2019). The document declares that:
To contribute to scientific literacy, it is key to understand the concepts and core ideas in science which allow new knowledge to be built. Big Ideas as a construct allows events and important phenomena of students’ lives to be explained during and after their time in school. Big Ideas are relationships and patterns observed in a broad range of phenomena. These relationships allow an integrated approach to science, which makes possible deeper learning about objects, materials, phenomena and the natural world. (30–31)
The intention is to try to have a curriculum that is appropriate to the context. More specifically, the science curriculum states that science teachers should select specific topics related to the subject and the context in which they are working. In that sense, the curriculum suggests to teachers that from level 1 to 10 the work should be around the Big Ideas of science, considering the Learning Objectives which integrate Biology, Physics and Chemistry. Then, at levels 11 and 12, the Big Ideas about science, which are related to aspects of the nature of science, are also incorporated. For some unexplained reason, two Big Ideas of science (as described by Harlen and her colleagues) are omitted: (2) Objects can affect other objects at a distance; and (6) The solar system is a very small part of one of millions of galaxies in the Universe (Ministerio de Educación de Chile Citation2019).
In Chile’s science national curriculum, the way the approach of Big Ideas is incorporated is not consistently articulated between the different levels of schooling in terms of its meaning, the learning objectives and the aims of science education, nor are these inconsistencies defended. The conceptualisation of Big Ideas for levels 1 to 6 (primary education) is understood as giving meaning ‘to the natural world through identifying the relationships between phenomena’ (Ministerio de Educación de Chile Citation2012, 71). The document for levels 7 to 10 (part of secondary education) treated them as ‘relevant ideas or concepts, which are generative and applied to a diversity of phenomena within an area of knowledge’ (Ministerio de Educación de Chile Citation2015, 18). For levels 11 and 12 (upper end of secondary education), Big Ideas are ‘core concepts and ideas of science that can build other knowledge’ (Ministerio de Educación de Chile Citation2019, 30). The phrases ‘identifying the relationships between phenomena’, ‘relevant ideas’ and ‘core concepts’ may suggest a hierarchy of the notion of Big Ideas as learners age, but such a hierarchy is neither obvious from the choice of words nor explicitly articulated in the curriculum. It seems that the conceptualisation of 2019 considers that Big Ideas embrace the whole content of science, being central to its existence in the science curriculum. In 2012 and 2015, though, Big Ideas are seen as a useful way to organise human understanding of the natural world, but not vital.
How inclusive Big Ideas are seen to be in terms of learning objectives is another difference between the Ministry’s 2012, 2015 and 2019 documents. Learning objectives in the science national curriculum documents include scientific content, scientific skills and overall attitudes. In some curricular documents, the learning objectives are presented as ‘developing’ the understanding of Big Ideas, along with skills and attitudes; in other documents, the learning objectives are the Big Ideas. From levels 1 to 10 (primary and part of secondary education), the Ministry states that ‘These Learning Objectives promote the understanding of the selected eight big ideas of science’ (Ministerio de Educación de Chile Citation2015, 133). In this sense, the learning objectives are overarching and ‘develop’ the Big Ideas and the skills, meaning that Big Ideas are only disciplinary content. In an Appendix of the 2015 document, there is a double-entry table showing the learning objectives separated for biology, physics and chemistry as columns concerning the eight Big Ideas from Harlen’s original set of Big Ideas as rows. Conversely, at levels 11 and 12 the big ideas are the learning objectives which are stated as ‘The understanding of the big ideas of science are expressed as Learning Objectives focused on learning outcomes and competencies’ (Ministerio de Educación de Chile Citation2019, 11). In this 2019 document, the double-entry table has disappeared; instead, there is a proposition of how the same selected eight Big Ideas could be developed in a new subject called Sciences for citizenship that is not biology, physics nor chemistry.
Because of the way the Chilean educational system is currently organised, the same teacher would teach from levels 7 to 12, i.e. throughout all of secondary education. Considering the discrepancy with respect to the official meaning of Big Ideas, this teacher should, in theory, shift her/his understanding of Big Ideas from that of disciplinary content when teaching levels 7 to 10, separated into biology, physics and chemistry, to one in which the term includes skills and attitudes, without the separation into biology, physics and chemistry, when teaching levels 11 and 12. Another difference is how Big Ideas are posed in relation to the aims of science education. At levels 1 to 6, the aim is stated to be ‘for the students to have a successful process of functioning in today’s society’ (Ministerio de Educación de Chile Citation2012, 70). This suggests an instrumental understanding of what science could mean to students’ lives, because science is seen as useful for functioning in contemporary society. However, for levels 7 to 12, the aim is about the scientific literacy of the students, since ‘Big Ideas as a construct allows events and important phenomena of students’ lives to be explained during and after their time in school’ (Ministerio de Educación de Chile Citation2019, 30). This indicates that science education is intended to help students, both while still at school and in their subsequent lives, to understand significant events through the eyes of science.
It bears noting that the initial training of teachers is differentiated into pre-primary, primary and secondary education (each between four and five years depending on the universities’ programmes). Only teachers trained to teach in secondary schools have specialist training to teach either biology, physics or chemistry; primary teachers have the opportunity to undertake a course in science but that is optional; otherwise, they are trained as general educators. At the Ministry level, pre-primary, primary and secondary education are dealt with by different groups, separating the responsibility and the product of making the guidelines for the whole period of schooling (Donoso Citation2005; Gysling Citation2004). Since 2015 there has been an unprecedented national initiative of continuous professional development courses that brings together teachers from pre-primary, primary and secondary education at the same time, aiming to enable them to reflect on the teaching of science in a way that is across these different levels, focusing mostly on scientific inquiry and more recently on Big Ideas of science education (https://basica.mineduc.cl/programa-icec-2/).
Despite the fact that Big Ideas of science education is the current approach to teaching science in Chile and in an increasing number of other countries, there is a lack of knowledge of how science teachers both work with Big Ideas in their classrooms and conceptualise the approach, which is the question that this study addresses. This study therefore aims to explore the intentions and practices in relation to the Big Ideas of science education by science teachers in Chile.
Methods
Participants
A total of 200 science teachers (a purposive sample) from pre-school, primary and secondary education in the Valparaíso Region in Chile were invited to participate in the study and 63 agreed to and did so, with 38 answering all the questions in the research instrument and 25 answering some of them. The list of invited science teachers was built from two sources: i) teachers who had shown interest in activities organised for the master’s in science education degree of the Pontificia Universidad Católica de Valparaíso in Chile; and ii) teachers who were part of a professional development programme from the Ministry of Education.
The research instrument
The research instrument was a questionnaire with open-ended questions, that was organised in three parts (see Appendix for a translation into English). In the first part, there were demographic questions; in the second part, two definitions from the literature on Big Ideas were provided and there were questions about teachers’ practices associated with the concept; in the final part, there were questions related to possible follow-up work focused on Big Ideas. The draft questionnaire was checked and commented on by three experts in the field, which led to some relatively minor changes.
Normally, a questionnaire might be thought quite a ‘thin’ instrument to use when seeking to obtain teachers’ views about curriculum change. However, we anticipated that we would obtain rich data, partly because of our previous experience of using questionnaires with teachers in Chile, partly because of our inclusion of a number of open-ended questions, and partly because the purposive sample targeted teachers whom we knew had considerable interest in school science education. In the event, the returns showed that we were indeed able to obtain rich data.
The questionnaire was available via SurveyMonkey for one month (March 2017). The participants received an information sheet with a consent form via email, before having the opportunity to fill in the questionnaire if they returned the completed consent form. The questionnaire was sent in Spanish.
Data analysis
Item responses were analysed thematically. Thematic analysis (Clarke and Braun Citation2014) is appropriate for a range of theoretical positions, in our case a critical one. It can address research issues such as ‘questions about people’s practices and views and opinions’ (Clarke and Braun Citation2014, 1948) and is appropriate for data-driven research (Joffe Citation2012). This research uses both deductive-inductive and latent themes (Joffe Citation2012), acknowledging both the Big Ideas and the learning progressions that already exist in the literature, whilst seek for new themes from the dataset. Thematic analysis is suitable for questions asking about the ‘specific nature of a given group’s conceptualization of the phenomenon under study’ (Joffe Citation2012, 212), teaching Big Ideas of science education in this case. We therefore followed the steps listed by Braun and Clarke (Citation2006): ‘i) Familiarizing yourself with your data; ii) Generating initial codes; iii) Searching for themes; iv) Reviewing themes; v) Defining and naming themes and; (vi) Producing the report’ (87).
Results
The online questionnaire was answered by 63 teachers. Thirty-eight of these completed the whole questionnaire, including all open-ended questions (parts 2 and 3), while 25 only answered part 1 of the questionnaire. The results are organised in two parts. The first (short) part provides a demographic account of who these teachers are in terms of gender, initial training, level of school in which they are currently teaching and teachers’ type of school/institution. All these demographic variables were analysed in relation to the experience they had when teaching Big Ideas of science education. The second part provides a thematic analysis of the responses to the open-ended questions in the questionnaire illustrating the conceptualisations and issues that arise from the views from these teachers’ experience regarding the creation and teaching of Big Ideas of science education.
First part: who are these teachers?
) show the diverse group of schoolteachers who answered the questionnaire. The way in which responses to the question ‘Given these definitions, do you have experience of teaching with any Big Ideas’ (total n = 63: ‘Yes’ (n = 35); ‘No’ (n = 3); ‘Did not answer’ (n = 25)) vary by teacher gender ()), initial training ()), level of school in which they were currently teaching ()) and type of school ()).
Figure 1. (a) Teachers’ gender. (b) Teachers’ initial training. (c) Teachers’ level of school in which they were currently teaching. (d) Teachers’ type of school/institution.
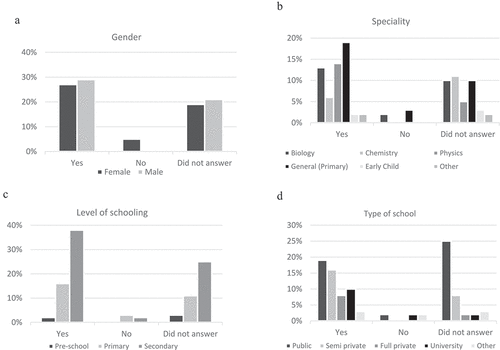
Even though there is no relationship between any of these demographic variables and whether or not respondents said they had experience of teaching with any Big Ideas, the sample is a diverse one with regards to gender, initial training, level of school and school type. Of the 35 teachers who answered that they had experience of teaching Big Ideas, 15 are female and 20 are male. The average age of these females is 40 years old while the average age of the males is 42 years old. Four of the female teachers were trained as primary or pre-school teachers while 11 of them were trained as secondary teachers (six in biology, one in physics and four in chemistry). Three of the male teachers were trained as primary teachers and 17 as secondary teachers (five in biology, eight in physics and four in chemistry). Of the teachers who declared their experience of teaching Big Ideas, one teaches at pre-school level, 14 at primary level, 17 at secondary level of schooling and three in tertiary education.
Despite the absence of any correlations between these demographic aspects and the teachers’ experience of teaching Big Ideas, there are some elements that can be highlighted in relation to the way that Big Ideas are presented in policy documents, such as the Chilean science curriculum.
The distribution of this group regarding the level of schooling at which they teach is interesting because in the national curriculum the approach of Big Ideas was incorporated differently for different levels of schooling. When, in 2012, the Big Ideas approach was introduced, it was initially incorporated only within levels 1 to 6 of schooling. In 2015 it was incorporated within levels 7 to 10, and then in 2019 it was incorporated within levels 11 and 12. So, by 2019, the approach had been incorporated within every level of schooling except for pre-school education. This means that when this questionnaire was distributed and completed in 2017, Big Ideas were explicitly incorporated throughout the primary phase of education, affecting 14 teachers of this group, and when incorporated in part of the secondary phase of education, affecting 17 teachers of this group (i.e. those whose teaching included any of levels 7 to 10), while four of them who teach in pre-primary or tertiary education and the secondary teachers who teach at level 11 and 12 (17 teachers of the group considering the Chilean system) were not affected by the incorporation of Big Ideas into the curriculum but declared that they teach with the approach anyway. Given this, the dependency on the official intended curriculum by teachers is put into question – a theme we develop later in this article – while the way that teachers teach Big Ideas in their classrooms becomes particularly relevant.
Second part: teachers’ views of creating and teaching Big Ideas of science education experience of teaching science with Big Ideas
When the participants were asked ‘Given these definitions, do you have experience of teaching with any Big Ideas?’ (question 2.a), 35 of 38 who responded gave a positive answer (13 of them just answered ‘yes’). Of these teachers who declared that they had experience of teaching with Big Ideas, 22 explained their answer in more detail. Some of the teachers (n = 6) wrote that this (i.e. the questionnaire) was the first time they had come across the concept of ‘Big Ideas’ ‘yes, but not consciously, this questionnaire is my first approach to the topic’ (Lucas – all names are pseudonyms that indicate gender). A number of teachers (n = 9) wrote that the use of this kind of integrated idea was the ‘natural way’ to teach in science, as explained by one teacher:
I had taught various topics related to the idea given [in the questionnaire], but I hadn’t considered it as a big idea of science. At that time, I wasn’t aware that what I was teaching was the result of a previous framework. It was just because this way of teaching adds meaning and context to what was taught. (Camila)
For other teachers (n = 5), the use of Big Ideas was explicitly related to Harlen’s (Citation2010) framework, often with such teachers also acknowledging that Big Ideas are incorporated within the new Chilean curriculum, as explained by one teacher:
Yes, these ideas are the aim in the biology courses I made in the training of teachers of biology where I participated as tutor-mentor. We take them into account (particularly in the training of science teachers) since the proposal of Harlen, which are explicit in the curricula from 2012 for secondary education, constituting one of the referents in the science education proposal to be developed by the teacher in practice. (Patricia)
This also happens in physics:
I think so and it was before knowing the concepts raised by Harlen in 2010. In fact, while teaching at the high school I think that little by little I was asking a lot of questions about my practice, for example what is essential to be taught, what will remain in the performance of my students after dealing with the subject matter? Physics as I learned it was related to making equations and solving algorithms and my first years of teaching were also characterised by this teaching. (Gabriel)
Some of the teachers who said they have experienced teaching with Big Ideas felt either insecure about teaching with them or preferred not to use them as an intended framework:
The truth is that now I have tried to teach with big ideas, but I do not know if I’m doing it well. I am working with the curriculum changes and I try to have the ideas connected to each other, but I have no evidence that this integrating learning is really being achieved by my students. (Javiera)
Yes, but I prefer to create my own big ideas considering the interests of my students regarding scientific issues. (Jaime)
The Big Ideas that these teachers said they had taught (see ; 21 Big Ideas were declared) were sometimes related to the sorts of Big Ideas proposed by Harlen but were sometimes very different. Mostly, they were not ideas but topics or scientific concepts.
Table 1. The variety of experiences of teaching with Big Ideas in terms of level of schooling and the Big Ideas teachers reported teaching.
Learning progression for Big Ideas
When asked ‘If so, which idea? At which level? How do you decide this is a big idea?’ (question 2.b), twelve of the teachers said they had experience teaching a Big Idea across different levels of schooling.
These teachers maintained that Big Ideas should be developed across different levels of schooling which acknowledged the idea of progression of these Big Ideas. One of the teachers explained:
From grade 9 without incorporating the concept of force, I develop the idea that all change is due to an ‘action’. Then in grade 10 I incorporate it as part of its language. In the 11th and 12th levels I continue with this idea of force, such as circular motion (to understand that the curved path implies a change of motion, therefore, there is a force), or to incorporate electric and magnetic force. This idea transcends all physics, whether classical or modern. (Pablo)
In that vein, there is a sense of continuity in the conceptualisation of a Big Idea. One of the teachers defined the concept thus: ‘a Big Idea is generated from other small ideas. The small ideas are connected between them and if we have that clear we can understand what is really fundamental in the curriculum as a whole’ (Dora). The curriculum is seen as a unity, where there is a relationship between the different content at any one level of schooling, and also progression between levels of schooling, as the small ideas that shape any one Big Idea are identified.
Big Ideas and daily life
The motivation for using Big Ideas for teaching science also varies among these teachers. Some of them think that teaching Big Ideas can relate to other concepts and create links with other scientific fields. For others, Big Ideas are used to teach socio-scientific issues and seek relationships between particular Big Ideas and the daily lives of the students. Some of the teachers claim that Big Ideas can be created with students during lessons and become a Big Idea of the group.
When teachers were asked to give their own definitions of a ‘Big Idea’ in question 2.c ‘Do you have your own definition of big idea? Could you please share it with me?’, 26 of them defined it as a concept linked with daily life. A Big Idea was held to allow scaffolding, connecting prior knowledge and articulating new knowledge; it has a relationship with science, technology and society and with socio-scientific issues, and it should be known and understood by the whole population. Moreover, Big Ideas should be close to the students’ lives and contextualised to reality. One of the teachers defined Big Ideas by saying:
I would define them as fundamental ideas of science and technology that the general population should know. In order to understand how our body works, for example, how we interrelate with the environment and how scientific and technological progress impacts society. (Bernarda)
Thus, Big Ideas are seen to have a close relationship with the context of the students and society.
In terms of the benefits for the students, the teachers recognise a motivation to use these kinds of ideas because they are related to the students’ daily lives. The teachers also portrayed the following advantages of using Big Ideas in science lessons in their answers to the question ‘Do you think teaching with big ideas in science could be helpful to the students? Why/Why not?’ (question 2.f): to allow students to work collaboratively; to apply the idea in another, linked piece of content; to understand the idea in a deeper way across different areas of content; to think about new explanations or perspectives; to make it easier to understand the aim of the lesson; to gain a global perspective; to be more critical regarding social issues; to have a systemic view in science; and to understand the key elements of the field and its relation with other fields. In that sense, Big Ideas influence both students and teachers. As explained by one teacher:
In both cases, what was learned was used in other lessons, which was very useful because the students connected physics with other subject content as well as concepts in physics. In addition, the students became a ‘bridge’ between me and another teacher because they mentioned us in the other class, which led us to communicate what we were working on to make the link more evident. (Berta)
Big Ideas and nature of science
To the same extent, Big Ideas allow students to understand how science works and how it has been undertaken in the past. Even though the majority of the teachers referred to the benefits of teaching Big Ideas because they can connect with the current daily life of the students (see previous section on Big Ideas and daily life), ten of these teachers thought that is important to understand how Big Ideas are developed during the history of science and, for that reason, teaching Big Ideas helps students to understand the nature of science and its history. As one teacher put it:
A Big Idea of science could be abstract knowledge that represents a part of the reality in a particular context (historical and social) which allows you to explain the natural world. In addition, these Big Ideas of science are accompanied by the research that has managed to define them as such. That research is in a particular time, it represents questions of real people with real interests that were born from close experiences within the world, with the people, with history, society and culture. (Gabriela)
For such teachers, as they discussed with the students the nature of scientific knowledge, the students became aware of how such knowledge is built and how they are learning about this knowledge. According to one of these teachers, the students can create a Big Idea based on what they know or what their peers know:
Students are reflecting, working in teams and using their knowledge and the knowledge of their peers to get an idea about some content. The importance of working based on big ideas is that the students are the ones who decide what interests them to learn and the teacher can focus and that. (Javiera)
In so doing, Big Ideas can be considered as fundamental content, metacognitive strategy for the students and also a pedagogical strategy for the teachers.
Continuous professional development focused on Big Ideas
In terms of the interest in understanding more about Big Ideas, the majority (n = 31) of the teachers claimed that it is important for them to know more about this framework. The teachers mentioned that it is important for them to gain more experience of teaching with Big Ideas so as to try to improve their teaching and understanding of the concept, as one teacher stated:
Yes, because the discussion and reflection of them allow us to redefine which would be these Big Ideas and how to organise our teaching around them. (Amelia)
Moreover, the teachers stated that enhancing their understanding of Big Ideas could help them to discuss the new Chilean curriculum, to make better decisions of what to teach and to implement new strategies and theory related to teaching Big Ideas. However, these teachers are more interested in their own creation and their own development of Big Ideas rather than adopting the existing framework:
I would be interested in created them, since perhaps those incorporated by the Ministry of Education do not fully coincide with the particular needs of each reality. For example, I would like to share big ideas with teachers who work better than I do for that topic, and this would allow me to learn and to grow in pursuit of my students. Also, the contact with other teachers is always good, knowing other realities, other experiences, and so on. (Roberto)
The teachers identified the following requirements to develop Big Ideas: sharing experiences and practice; working collaboratively among peers; learning from other experiences; reflecting on their own and others’ practice; and acknowledging another point of view. One of the teachers illustrated the whole idea of community of practice as,
Creating Big Ideas and having a certain ‘bank of Big Ideas’ and somehow influencing the school culture with this ‘convention’ that we would be building. (Berta).
Finally, the participants were asked ‘Would you like to participate in a continuing professional development programme focused on Big Ideas?’ (question 3.d). All 38 teachers gave an affirmative answer. They explained that it is not only important to know about Big Ideas but also to adopt a stance of ‘Big Ideas mentality’. In that sense, they claimed that teachers should be permanent learners and researchers of their practice. Furthermore, even though there is a belief without hesitation in their own practice, some of them also believe that the merging of theory and practice will give them a better understanding of how to teach Big Ideas.
Discussion and conclusion
The Big Ideas of science education movement is an attempt to provide a coherent account of precisely what science educators want students to learn as a result of their science lessons from age 5 through to age 17. Despite receiving almost no funding and being the brainchild of just one person, Wynne Harlen (someone who officially ‘retired’ years before starting her work on Big Ideas), Big Ideas of science education can indeed be described as a ‘movement’. Wynne Harlen’s writings about Big Ideas are heavily cited and have had considerable policy impact, resulting in major changes to the school science curricula in a number of countries.
However, to date no research seems to have been undertaken on how school science teachers conceptualise and practise Big Ideas. This is despite the fact that it is well established that for any science curriculum initiative to be successful, it is essential that classroom teachers take ownership of it (Ogborn Citation2002; Levinson Citation2018). This article uses data from 38 teachers in Chile to examine how they understand and experience the Big Ideas of science education.
Perhaps our central finding is that many of the teachers had their own understanding of Big Ideas. Indeed, a number of the teachers said that they were teaching Big Ideas even though they had not come across the term before completing our questionnaire – rather like Mr. Jourdain in Molière’s Le Bourgeois gentilhomme who discovers that he has been speaking prose all his life without realising it. The teachers had a range of ways of conceptualising Big Ideas. A number of them saw a Big Idea as enabling links to be made with other scientific ideas; others used Big Ideas to teach socio-scientific issues and seek relationships between particular Big Ideas and the daily lives of the students; some teachers felt that a Big Idea could be specific to a particular group of students.
These understandings do not contradict the intentions of the team that put together the Big Ideas documents (Harlen Citation2010, Citation2015a, Citation2015b) but are significantly different from them. This distinctiveness is in line with Ogborn’s (Citation2002) experience of leading a large physics curriculum development project. In particular – and we do not know whether this is a particular feature of Chilean science teachers or would be found elsewhere – the teachers were more interested in their own creation and development of Big Ideas rather than simply adopting the existing, published framework which can perhaps be explained by the way that the approach is expressed in the national curriculum.
Despite differences as to how the approach of Big Ideas is expressed in the Chilean curriculum for different age groups of students, the teachers were very positive about Big Ideas, seeing them as a possible way of connecting with the daily lives of students, facilitating progression in students’ learning of science, enabling students to work collaboratively and make links between different parts of the curriculum, helping students to understand how science works and how it has been undertaken in the past, and as preferable to having to teach an overloaded science curriculum that lacks such an organising framework.
The teachers welcomed the possibility of Continuing Professional Development about Big Ideas. It was felt that such Continuing Professional Development would facilitate sharing experiences and practice, working collaboratively with their peers and learning from the experiences of others. It would enable them to reflect on their own practices and those of others, and to acknowledge and learn from other points of view. It is possible that some of these benefits might result from introducing the notion of Big Ideas into Initial Teacher Education. Nevertheless, given that the introduction of Big Ideas into the Chilean science national curriculum is a relatively recent phenomenon, it seems very possible on these grounds alone that Continuing Professional Development courses are likely to be important in embedding a rich understanding and praxis of Big Ideas. In addition, it is possible that experienced teachers are more likely to benefit from sessions on Big Ideas than are student teachers. Indeed, there are now some Continuing Professional Development courses about Big Ideas in operation in Chile.
Finally, there are limitations related to our use of a questionnaire, including the presence of unanswered questions and problems teachers may have had with understanding the questions. Even though the instrument offered an exploration of the way that schoolteachers conceptualise the Big Ideas approach recently incorporated within the Chilean science curriculum, it is not of course possible to generalise regarding the way teachers in other countries implement the approach in their classrooms; furthermore, our data are teachers reporting their views and practices. For these reasons, and given the increasing uptake of Big Ideas in science education, we would advocate more in-depth exploration in Chile and elsewhere of how teachers teach Big Ideas to their students, using classroom observations and interviews to develop a richer picture of the feasibility and realities of the approach. We, the authors, consider that what is presented here is a first step to explore what the Big Ideas approach means to school science teachers, policy documents, such as those that govern science curricula, and continuous professional development programmes. Such research could help the science education community develop a better understanding of the significance and impact of the Big Ideas approach, including the ways it is interpreted, implemented and even questioned.
Disclosure statement
No potential conflict of interest was reported by the author(s).
References
- Ahumada, L., S. Galdames, A. Gonzalez, and P. Herrera. 2009. “El funcionamiento del equipo directivo durante un proceso de autoevaluación institucional en el marco de políticas de aseguramiento de la calidad de la gestión escolar en Chile.” Universitas Psychologica 8 (2): 353–370.
- Barmby, P., P. Kind, and K. Jones. 2008. “Examining Changing Attitudes in Secondary School Science.” International Journal of Science Education 30 (8): 1075–1093. doi:10.1080/09500690701344966.
- Braun, V., and V. Clarke. 2006. “Using Thematic Analysis in Psychology.” Qualitative Research in Psychology 3 (2): 77–101. doi:10.1191/1478088706qp063oa.
- Bybee, R. W. 2014. “NGSS and the next generation of science teachers.” Journal of science teacher education 25 (2): 211–221.
- Cartier, J. L., and S. L. Pellathy. 2009. “Integration with Big Ideas in Mind.” Science and Children 46 (8): 44–47.
- Chalmers, C., M. L. Carter, T. Cooper, and R. Nason. 2017. “Implementing “Big Ideas” to Advance the Teaching and Learning of Science, Technology, Engineering, and Mathematics (STEM).” International Journal of Science and Mathematics Education 15 (1): 25–43. doi:10.1007/s10763-017-9799-1.
- Choi, K., H. Lee, N. Shin, S.-W. Kim, and J. Krajcik. 2011. “Re-conceptualization of Scientific Literacy in South Korea for the 21st Century.” Journal of Research in Science Teaching 48 (6): 670–697. doi:10.1002/tea.20424.
- Clarke, V., and V. Braun. 2014. “Thematic analysis.” Encyclopedia of critical psychology 1947–1952. New York, NY: Springer.
- Cooper, R. 2015. “Teaching the Big Ideas of Biology with Operon Models.” The American Biology Teacher 77 (1): 30–39. doi:10.1525/abt.2015.77.1.5.
- Cox, C. 2012. “Política y políticas educacionales en Chile 1990–2010.” Revista Uruguaya de Ciencia Política 21: 13–43.
- Devick-Fry, J., and T. LeSage. 2010. “Science Literacy Circles: Big Ideas about Science.” Science Activities: Classroom Projects and Curriculum Ideas 47 (2): 35–40. doi:10.1080/00368120903383133.
- Donoso, S. 2005. “Reforma y politica educacional en chile 1990–2004: El neoliberalismo en crisis.” Estudios Pedagógicos 31 (1): 113–135.
- Duschl, R., S. Maeng, and A. Sezen. 2011. “Learning Progressions and Teaching Sequences: A Review and Analysis.” Studies in Science Education 47 (2): 123–182. doi:10.1080/03057267.2011.604476.
- Gysling, J. 2004. “Reforma Curricular: Itinerario de una transformación cultural.” In Políticas educacionales en el cambio de siglo. La reforma del sistema escolar de Chile, edited by C. Cox, 213–252. Santiago, Chile: Editorial Universitaria.
- Harlen, W. 2010. “Principles and Big Ideas of Science Education.” Duns. https://www.ase.org.uk/bigideas
- Harlen, W. 2015a. “Towards Big Ideas of Science Education.” School Science Review 97 (359): 97–107.
- Harlen, W. 2015b. “Working with Big Ideas of Science Education.” Trieste: InterAcademy Partnership. https://www.ase.org.uk/bigideas
- Hattie, J. 2008. Visible Learning: A Synthesis of over 800 Meta-analyses Relating to Achievement. London: Routledge.
- Holbrook, J., and M. Rannikmae. 2009. “The Meaning of Scientific Literacy.” International Journal of Environmental and Science Education 4 (3): 275–288.
- Hollins, M., and M. J. Reiss. 2016. “A review of the school science curricula in eleven high achieving jurisdictions„. The Curriculum Journal 27 (1): 80–94.
- Joffe, H. 2012. “Thematic Analysis.” In Qualitative Research Methods in Mental Health and Psychotherapy: A Guide for Students and Practitioners, edited by D. Harper and A. R. Thompson, Vol. 1, 209–223. Oxford: JohnWiley & Sons.
- Juan, A., V. Reddy, T. Zuze, C. Namome, and S. Hannan. 2016. “Does It Matter whether Students Enjoy Learning Science? Exploring Student Attitudes Towards Science in South Africa.” Policy Brief 08. Cape Town: HSRC.
- Khine, M. S., ed. 2015. Attitude Measurements in Science Education: Classic and Contemporary Approaches. Charlotte, NC: Information Age Publishing.
- Lelliott, A., and M. Rollnick. 2010. “Big Ideas: A Review of Astronomy Education Research 1974–2008.” International Journal of Science Education 32 (13): 1771–1799. doi:10.1080/09500690903214546.
- Levinson, R. 2018. “Realising the School Science Curriculum.” The Curriculum Journal 29 (4): 522–537. doi:10.1080/09585176.2018.1504314.
- Lord, E., and A. Kiddle. 2016. “What Do We Really Mean When We Talk about Big Ideas in Mathematics?” Mathematics Teaching 252: 30–31.
- Loughran, J., A. Berry, and P. Mulhall. 2012. Understanding and Developing Science Teachers’ Pedagogical Content Knowledge. 2nd ed. Rotterdam: Sense.
- Mansfield, J. and M. J. Reiss. 2020.The place of values in the aims of school science education. In: Values in Science Education, edited by Corrigan D., Buntting C., Fitzgerald A. & Jones A, 191–209. Cham: Springer.
- Metz, S. 2012. “Big Ideas.” The Science Teacher 79 (5): 6.
- Ministerio de Educación de Chile. 2012. Bases curriculares primero a sexto básico. Santiago: Unidad Curriculum y Evaluación.
- Ministerio de Educación de Chile. 2015. Nuevas Bases Curriculares y Programas de Estudio 7° y 8° año de Educación Básica/1° y 2° año de Educación Media. Santiago: Unidad Curriculum y Evaluación.
- Ministerio de Educación de Chile. 2019. Bases curriculares 3° y 4° medio. Santiago: Unidad Curriculum y Evaluación.
- Mitchell, I., S. Keast, D. Panizzon, and J. Mitchell. 2016. “Using ‘Big Ideas’ to Enhance Teaching and Student Learning.” Teachers and Teaching 23 (5): 596–610. doi:10.1080/13540602.2016.1218328.
- Mutvei, A., and J.-E. Mattsson. 2015. “Big Ideas in Science Education in Teacher Training Program.” Procedia, Social and Behavioral Sciences 167: 190–197. doi:10.1016/j.sbspro.2014.12.661.
- OECD. 2020. “Results from TALIS 2018, Volume II, Country Note: Chile.” http://www.oecd.org/education/talis/TALIS2018_CN_CHL_Vol_II.pdf
- Ogborn, J. 2002. “Ownership and Transformation: Teachers Using Curriculum Innovations.” Physics Education 37 (2): 142–146. doi:10.1088/0031-9120/37/2/307.
- Olson, J. K. 2008. “Concept-focused Teaching: Using Big Ideas to Guide Instruction in Science.” Science and Children 46 (4): 45–49.
- Osborne, J. 2007. “Science Education for the Twenty First Century.” Eurasia Journal of Mathematics, Science & Technology Education 3 (3): 173–184. doi:10.12973/ejmste/75396.
- Osborne, J., S. Collins, M. Ratcliffe, R. Millar, and R. Duschl. 2003b. “What ‘Ideas-about-science’ Should Be Taught in School Science? – A Delphi Study of the Expert Community.” Journal of Research in Science Teaching 40 (7): 692–720. doi:10.1002/tea.10105.
- Osborne, J., S. Simon, and S. Collins. 2003a. “Attitudes Towards Science: A Review of the Literature and Its Implications.” International Journal of Science Education 25 (9): 1049–1079. doi:10.1080/0950069032000032199.
- Plummer, J. D., and J. Krajcik. 2010. “Building a Learning Progression for Celestial Motion: Elementary Levels from an Earth-based Perspective.” Journal of Research in Science Teaching 47 (7): 768–787. doi:10.1002/tea.20355.
- Reiss, M. 2014. “What place does science have in an aims-based curriculum?„ School Science Review 95 (352): 9–14.
- Smith, J. P., III, and M. Girod. 2003. “John Dewey and Psychologizing the Subject-matter: Big Ideas, Ambitious Teaching, and Teacher Education.” Teaching and Teacher Education 19 (3): 295–307. doi:10.1016/S0742-051X(03)00016-7.
- Taber, K. S. 2017. “Knowledge, Beliefs and Pedagogy: How the Nature of Science Should Inform the Aims of Science Education (And Not Just When Teaching Evolution).” Cultural Studies of Science Education 12 (1): 81–91. doi:10.1007/s11422-016-9750-8.
- Windschitl, M., J. Thompson, M. Braaten, and D. Stroupe. 2012. “Proposing a Core Set of Instructional Practices and Tools for Teachers of Science.” Science Education 96 (5): 878–903. doi:10.1002/sce.21027.
- Wintersgill, B., A. Brine, D. Cush, D. Francis, R. Freathy, F. Henchley, J. Holt, et al. 2017. “Big Ideas for Religious Education.” Exeter: University of Exeter. http://socialsciences.exeter.ac.uk/media/universityofexeter/collegeofsocialsciencesandinternationalstudies/education/research/groupsandnetworks/reandspiritualitynetwork/Big_Ideas_for_RE_E-Book.pdf
- Young, M. F. D. 2008. Bringing Knowledge Back In: From Social Constructivism to Social Realism in the Sociology of Knowledge. London: Routledge.
Appendix
Questionnaire
Big ideas in mind
Dear teacher,
The aim of the following questionnaire is to explore the intentions and practices you have related to teaching with the big ideas of science education. In this sense, there are no such things as ‘right’ or ‘wrong’ answers; the intention of the questionnaire is to obtain your opinions and experience.
Professional and personal information
Date of birth (dd/mm/yy)
Which is your highest degree?
How many years of teaching experience do you have?
At which level do you teach?
If you currently teach in a school, in which type of school do you teach? You can identify more than one school.
How many hours do you work per week?
How many students do you teach per week?
Teaching big ideasBefore the next part of the questionnaire, here are two definitions of a big idea and an example:
‘A progression towards key ideas which together enable understanding of events and phenomena of relevance to students’ lives during and beyond their school years’ (Harlen Citation2010, 2).
‘A big idea is a unifying principle that connects and organises a number of smaller ideas or concepts and multiple experiences. In other words, integrating is one role that makes big ideas pedagogically powerful in that they offer direction for teachers to make learning for students more connected’ (Mitchell et al. Citation2016, 3).
To illustrate, a big idea could be: ‘Organisms are organised on a cellular basis’.
Given these definitions, do you have experience of teaching with any big ideas?
If so, which idea? At which level? How do you decide this is a big idea?
Do you have your own definition of big idea? Could you please share it with me?
Please give me one example of what happen when you taught with this/those big ideas.
If you do not have experience teaching with big ideas, can you think of one idea which you would like to teach in this sense? If possible, describe which idea and level.
Do you think teaching with big ideas in science could be helpful to the students? Why/Why not?
What possibilities do you think big ideas have for teaching science? Why?
3. Working with big ideas
If you have experience teaching with big ideas, can I come and watch you teach one or more of your classes in the future? Please give me your contact details.
Are you interested in understanding more about this topic? Please explain your reasons.
Are you interested in creating and planning your own big ideas with other science teachers? Please explain your reasons.
Would you like to participate in a continuing professional development programme focused on big ideas? Please explain your reasons.