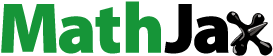
ABSTRACT
This case study reports the results of a 12-year (2005–2016) follow-up study of two Olympic champion rowers. The rowers were prospective athletes at the junior level when the study began, and we monitored their relevant physiological and performance data annually. Our findings indicated that their V̇O2max gradually increased up to about 22 years of age and leveled off at a value of approximately 7 l·min−1 with minimal fluctuations thereafter. However, the variables that directly influence the V̇O2max changed. There was an age-related decline in maximal heart rate of about 0.5 beats·year−1, while oxygen pulse, which serves as an indirect measure of stroke volume, correspondingly increased by about 1 ml O2·beat−1 per year, allowing the athletes to maintain exceptional V̇O2max values. Maximal minute power of the studied rowers, derived each year from their ramp-wise tests, closely resembled the mean power output sustained during the 2000-m all-out tests on a rowing ergometer. A 12-year improvement of 28% and 33% occurred for the mean power output sustained over 2000 and 6000-m on a rowing ergometer, respectively. The findings contribute to the body of knowledge on athletes representing the true elites of their respective sports.
Introduction
Multi-year studies that describe the development of physiological and performance characteristics of endurance athletes who represent the true elites of their respective sports (i.e., Olympic and world champions) are rare. This is probably because of the limited access to such athletes and the finite nature of the population. Specifically, in the sport of rowing, to the best of our knowledge, only three case studies addressing the physiological and performance characteristics of Olympic and world champions over a multi-year period have been published (Lacour, Messonnier, & Bourdin, Citation2009; Mikulic, Citation2011; Nybo, Schmidt, Fritzdorf, & Nordsborg, Citation2014). Two studies (Lacour et al., Citation2009; Nybo et al., Citation2014) examined periods of peak career performance of rowers which, in a study by Nybo et al. (Citation2014), lasted more than 20 years. In addition, we reported on a 6-year physiological and performance development of four rowers, from competing at a junior level to acquiring elite status by winning a world championship title (Mikulic, Citation2011). We aim to (a) expand on our previously reported findings (Mikulic, Citation2011) by providing additional data from the monitored 6-year period, and (b) report the results for the additional six-year monitoring (12 years in total) of two of the athletes that were followed in our previous study (Mikulic, Citation2011).
Rowing is a strength-endurance, whole-body activity that requires the activation of almost all muscles in the body (Secher, Citation2000). Aerobic endurance is particularly important for successful rowing, and it comes as no surprise that maximal oxygen uptake, expressed in l·min−1, often emerges as a strong correlate of rowing performance. For example, in a recent study (Ingham, Pringle, Hardman, Fudge, & Richmond, Citation2013), V̇O2max correlated strongly (r = 0.88 – 0.90) with 2000-m time trial on a rowing ergometer in a group of trained rowers. Given the importance of V̇O2max for success in competitive rowing, this physiological variable has traditionally been of great interest to rowing athletes, their coaches, and sports physiologists. The V̇O2max relies on arterial oxygen delivery or, more precisely, on maximal cardiac output (max) and arterial oxygen content (Bassett & Howley, Citation2000). Maximal cardiac output is classically defined as a product of stroke volume (SV) and heart rate (HR). Heart rate can be measured directly using a heart rate monitor, while the oxygen pulse (i.e., the volume of oxygen consumed by the body per heartbeat) serves as an indirect indicator of stroke volume.
Maximal minute power (MMW) is a measure that has been used extensively for monitoring the performance capability of athletes competing in other endurance sports. It is defined as the highest mean power output sustained over any 60-second period during a ramp-wise test (Bird & Davidson, Citation1997). Although referred to in different ways in previous studies involving distance runners and cyclists (Ingham et al., Citation2013), it is one the strongest correlates of performance in these athletes. In rowing, MMW had, until recently, not been investigated. Ingham et al. (Citation2013) derived it from a ramp-wise test performed by a group of trained rowers, and they reported it as the strongest single correlate of 2000-m time trial on a rowing ergometer (r = 0.98). Importantly, the authors reported a minimal difference (~6 W; amounting to <2%) between MMW and mean power output sustained over a 2000-m time trial on a rowing ergometer, thus supporting the earlier findings of Bourdin, Messonnier, Hager, and Lacour (Citation2004) who derived a similar measure and also reported a strong association (r = 0.92) and a minimal difference (~13 W) between mean power output sustained over the 2000-m distance on a rowing ergometer. Indeed, MMW is a valid and useful measure in understanding, predicting, and monitoring rowing performance.
In addition to the use of rowing ergometers in laboratories for physiological and performance assessment, coaches routinely use these devices to evaluate rower’s exercise capacity. All-out tests over 2000-m and 6000-m are among the most frequently used tests on a rowing ergometer. The duration of a 2000-m rowing ergometer test closely corresponds to the duration of 2000-m on-water races, thereby simulating the metabolic demands of actual on-water performance. A 6000-m ergometer test, on the other hand, is intended to assess the prolonged exercise performance of rowers. It should be noted that these tests are also commonly used as important criteria for selecting rowing athletes for national team crews.
The primary purpose of the current case study was to describe the development of the above-described physiological and performance characteristics of two world-class men heavyweight rowers over a 12-year period of their respective competitive careers. The multi-year development of V̇O2max in these athletes was investigated, as well as changes in physiological variables that directly affect V̇O2max: HRmax and SV. We also charted the development of MMW because of its strong association with performance over 2000 m on a rowing ergometer.
The secondary purpose of the study was to investigate adaptations to long-term rowing training using the physiological and performance measurements and the relationships between these measures. For this study, the 12-year period during which the athletes were followed was divided into three four-year developmental phases: phase 1 – competing at the junior – and early-senior levels; phase 2 – achieving world-class status by winning a world championship title and an Olympic medal; and phase 3 – maintaining world-class status by winning three consecutive world championship titles and, subsequently, an Olympic gold medal.
Methods
This case study reports the results of a 12-year study of a world-class rowing crew, the men’s heavyweight double-sculls, the 2016 Olympic champions. In addition to the Olympic gold medal won in 2016 in Rio de Janeiro, the two studied rowers have, either together as a double-scull crew or as members of a quadruple-scull crew, won a total of 4 world championship titles (2010, 2013 – 2015) and a silver medal at the 2012 Olympic Games in London.
Annual testing
Physiological and performance assessments completed at regular intervals throughout the study included maximal oxygen uptake, maximal heart rate, oxygen pulse, maximal minute power, and mean power output sustained during all-out tests over 2000 m and 6000 m on a rowing ergometer. These measures were used to evaluate adaptations in physiological and performance characteristics of rowers to long-term training. The two studied rowers underwent annual physiological assessments in our laboratory from 2005 to 2016. Because of their subjective feelings of fatigue at the time of the assessment, they were not able to complete their last physiological assessment in 2016, so the physiological data from 2016 (i.e., the final assessment point of a 12-year follow-up) are missing from this report. The annual assessment was performed during preparatory periods, either in late November, December, or early January. The tests were always conducted in the morning hours, between 8:30 and 11:00 am. The study was approved by the Committee for Scientific Research and Ethics of the Faculty of Kinesiology at the University of Zagreb.
The rowers were given 10 minutes to warm up by combining ergometer rowing (Concept2 Model C (from 2005 to 2010) and Model D (from 2011 to 2016), Morrisville, VT, USA) and stretching in accordance with their usual habits. The rowers were then equipped with the necessary instrumentation and sat quietly for 1 min on the ergometer before starting the exercise. The exercise started at a power output of 150 W, which the rowers maintained for 3 min. The rowers then increased their power output incrementally by 25 W every minute until volitional exhaustion or until the output was reduced by 10 W of the target power for five consecutive strokes. Each rower chose his optimal stroke rate for each power output stage. The drag factor for all tests was freely set by the rowers after consultation with their coach and ranged between 120 and 135. For physiological tests carried out from 2005 to 2012, we used a stationary rowing ergometer. From 2013 onwards, after discussion with the rowers’ coach and to provide “on-water-feeling” during physiological testing, the rowing ergometer was placed on horizontal slides that allowed the ergometer to move back and forth during the rowing stroke.
Gas-exchange data were measured breath-by-breath using a Quark b2 metabolic measurement cart (Cosmed, Rome, Italy) and stored in the memory of a PC for later analysis. Before each test, the gas analyzers were calibrated using gases (CO2 and O2) of known concentration, and the flow meter was calibrated using a 3-L syringe. The same procedures were repeated immediately after each test to verify the readings. Heart rate was monitored using the short-range Polar radio telemetry system (Polar Electro, Kempele, Finland). During off-line processing, data were recorded as means for 30-s intervals, and the highest values for physiological variables were taken the highest mean of two consecutive 30-s intervals. The 2000-m and 6000-m rowing ergometer performance data were collected at annual competitions organized either by the national rowing federation or by the rowing club to which the rowers belong. These competitions are held between December and February of each year and generally no more than eight weeks from physiological testing dates. All 2000-m rowing ergometer performance data analyzed for this study were achieved on a stationary Concept2 (model D) rowing ergometer, while 6000-m rowing ergometer performance data were achieved either on a stationary (2005–2012) or on a Concept2 model D positioned on a Concept2 slide (2013–2016). Ergometer performance data were collected in form of performance times that were later converted to the mean power output values using a conversion calculator provided on the manufacturer’s website.
Training
The training routines of rowers during preparatory and competition periods over the last monitored phase of the study were consistent. In short, the training consisted of approximately 12 training sessions per week, which amounted to a training volume of about 24 h·wk−1. Of these 12 weekly sessions, nine were typically endurance-based, and three were strength-based sessions performed in the weight room. Endurance-based training was predominately performed as specific rowing training on the water or, during the colder months of the year, on a rowing ergometer. However, cross-training (e.g., cycling, running, cross-country skiing, and training on various indoor simulators) was used for up to 30% of the endurance-based sessions even during the competition periods. About 80% to 85% of the total endurance-training time was spent at training intensities that could be characterized as low to moderate (HR in the range 50–85% of the HRmax). The remaining 15–20% of the total endurance-training time was spent at intensities at or above 85% of HRmax. There were 2 weekly training sessions with intensities at or above 85% of HRmax during the preparatory periods, and 3–4 weekly training sessions during the competition periods.
Reliability of measures
Between-days test–retest reliability of measures derived from the ramp-wise exercise test on a rowing ergometer, conducted as previously described, was recently assessed in our laboratory with the relative technical error of measurement. In a sample of eight national-level rowers (mean ± SD: age 22 ± 2 years, body mass 83 ± 4 kg, stature 185 ± 4 cm) who performed two identical exercise tests 3–7 days apart, V̇O2max, HRmax, oxygen pulse and MMW had technical errors of measurement of 3.0%, 1.5%, 4.0%, and 3.8%, respectively. The reliability of performance of a 2000-m all-out test on a rowing ergometer has been previously established by Schabort, Hawley, Hopkins, and Blum (Citation1999). The authors determined the variability of performance for individual rowers, expressed as a coefficient of variation for mean power output, to be 2.0% (95% CI: 1.3 to 3.1%). Corresponding reliability data for 6000-m ergometer performance are not available.
Statistics and calculations
All reported data in the study are presented as mean values calculated from both rowers. Simple regression was used to identify the relation between changes in physiological variables (i.e., maximal heart rate and oxygen pulse) and age. Microsoft Excel software (Microsoft Corporation, Redmond, WA, USA) was used to process and report the data.
Results and discussion
This case study describes the physiological and performance development of two heavyweight men rowers over a 12-year period and across three phases of their careers: from competing as prospective rowers at the junior – and early-senior levels (age 15–16 to 19–20), through to achieving world-class status by winning a world championship title and a silver medal at the Olympic Games (age 19–20 to 23–24), to maintaining the world-class status by winning three consecutive world championship titles as well as a gold medal at the Olympic Games (age 23–24 to 27–28). Over the 12 – year study period, the rowers competed exclusively in sculling boats on major competitions. From 2005 to 2008 they competed in various sculling boats; from 2009 to 2013 they were members of a quadruple-scull squad, and in 2014 they formed a double-scull squad and competed together as a crew until the end of the 2016 season.
Anthropometry
The rowers’ basic physical characteristics were: stature for both rowers, 189 cm and, at the time when the study commenced in 2005, they were already at 98% of their adult stature. Their mean body mass ranged between 93 and 97 kg over the last two phases of the study which is an ~8% increase from the time the study commenced. Also, over the last two phases of the study, body fat mean value typically ranged between 10% and 12% as estimated from skinfold thicknesses, corresponding to a mean lean body mass of approximately 85–86 kg (with small fluctuations outside this range).
Physiological measures
Maximal oxygen uptake, as expressed in l·min−1 and indicated in ), gradually increased from the beginning of the study by approximately 0.3 l·min−1 per year up to the crew mean value of 6.99 l·min−1 in 2011, when the rowers’ mean age was 22. With minor fluctuations, V̇O2max was then maintained until the last completed physiological assessment in 2015, when the rowers’ mean age was 26. This last physiological assessment in 2015 also yielded the study’s highest crew mean V̇O2max value of 7.09 l·min−1. The point at which V̇O2max, over a multi-year period plateaus in world-class endurance athletes tends to be from 20 to 25 years of age. At that point, it is assumed that an athlete has neared his peak potential, after which further substantial increases in V̇O2max are difficult to achieve. In a recent multi-year study on a world-class lightweight rower (Nybo et al., Citation2014), the winner of five medals at five consecutive Olympic Games, the authors reported V̇O2max leveling off at around 24–25 years of age (at a value of about 5.9 l·min−1) with minimal fluctuations thereafter.
Figure 1. Changes in maximal oxygen uptake (Figure 1a), maximal heart rate (Figure 1b), and oxygen pulse (Figure 1(c) versus age in our study participants. Markers are mean values for both rowers.
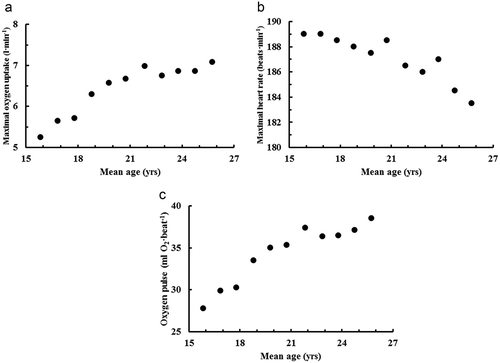
While maintaining world-class status by winning three consecutive world championship titles and a gold medal at the Olympic Games (2013–2016), the rowers’ V̇O2max values were 6.86 l·min−1 in 2013, 6.87 l·min−1 in 2014 and 7.09 l·min−1 in 2015 (physiological data for 2016 were not collected). These values are greater than those reported for other top-caliber rowers, winners of Olympic gold medals. Specifically, Lacour et al. (Citation2009) reported that a 2000 Olympic champion sweep-oar rower recorded V̇O2max of about 6.3 l·min−1 in the winter months preceding his gold-medal race in the summer. This is ~10% lower value than the rowers in our study. Ingham, Carter, Whyte, and Doust (Citation2007) reported an even lower mean value (5.9 l·min−1) for a group of eight Olympic champion rowers. Values that are similar to the exceptional V̇O2max values of our studied rowers were reported by Godfrey, Ingham, Pedlar, and Whyte (Citation2005) for a 2004 Olympic champion rower (unspecified event). He recorded a V̇O2max value of ~6.8 l·min−1; however, the rower was tested during the peak competition period just eight weeks before the Olympics.
Age-related reductions in V̇O2max are well documented. Reductions occur after about 20 years of age both in non-athletes and athletes (Tanaka & Seals, Citation2008). Endurance athletes have a more pronounced decline with aging than sedentary individuals (Pimentel, Gentile, Tanaka, Seals, & Gates, Citation2003). While further annual physiological monitoring will be necessary to address this issue, no reduction in V̇O2max occurred in our studied athletes up to 26 years of age. These two case reports of Olympic champion rowers are encouraging in that they suggest that intense endurance training (typical for elite endurance athletes) counters the common age-induced reduction of V̇O2max. Specifically, Lacour et al. (Citation2009) reported that an Olympic champion rower maintained a high V̇O2max continuously up until the end of his international career at the age of 32, while Nybo et al. (Citation2014) reported that high V̇O2max can be maintained throughout a rower’s career to as late as age 40. The ability to maintain a high V̇O2max late in the third decade of life influences some rowers’ ability to compete at top international standard even when approaching (or in some instances exceeding) age 40.
Other changes in physiological variables included a gradual reduction in HRmax from 188 – 189 beats·min−1 during the first 4-year phase to about 184 beats·min−1 in the last two years of physiological testing (2014–2015). Monitored over 11 years, this corresponds to a decline in HRmax of about 0.5 beats·year−1 (regression equation: HRmax = 197.3–0.47 x age, standard error of the estimate (SEE) = 0.8, coefficient of variation (CV) = 0.4; )). The phenomenon of an age-induced reduction in HRmax is well established (Tanaka, Monahan, & Seals, Citation2001), and it occurs even in elite endurance athletes, despite their engagement in intense endurance training. A five-time Olympic medal winner rower decreased their HRmax by about 0.9 beats·year−1 from ages 19 to 40 (Nybo et al., Citation2014). In another Olympic champion rower, HRmax decreased from 185 to 182 beats·min−1 over six years of his competitive career (Lacour et al., Citation2009). This is a decrease of approximately 0.5 beats·year−1 (over the age span of 26 to 32 years), which closely resembles the rate of decline in the present case study. The reduction in HRmax with age is notable because it is often considered to be a primary cause for the general decline in V̇O2max both in athletes and non-athletes. In the present study, our athletes displayed a decline in HRmax of about 0.5 beats·year−1 but this decline over the 11-year period was not associated with a reduction in V̇O2max. A physiological explanation could be that max can be reached at a non-maximal heart rate (Mortensen et al., Citation2005).
A noteworthy observation is the maintenance of V̇O2max during the last several years of the study, given the decline in HRmax. For V̇O2max values to remain constant despite the decrease in HRmax, the Fick equation dictates that either stroke volume of the heart must increase or the arteriovenous oxygen difference of the active muscles must expand. While we cannot draw any conclusions about the arteriovenous difference, the most likely compensatory mechanism is increased SV. Indeed, in contrast to the decline in HRmax, oxygen pulse, which serves as an indirect indicator of SV, increased over the 11 years by approximately 1 ml O2·beat−1·yr−1 (regression equation: oxygen pulse = 13.5 + 0.99 x age, SEE = 1.3, CV = 3.8; )). At the mean age of 26, it had a mean value of 38.8 ml O2·beat−1, which was the highest value over the course of the study. The only other study involving top-caliber rowers that reported oxygen pulse values was Nybo et al. (Citation2014). A five-time Olympic medalist (a lightweight rower) was studied for over more than 20 years of his elite career, and his oxygen pulse values increased steadily to about 34–35 ml O2·beat−1 at the age of 27–28. He then managed to maintain the values in this range for over a decade until the end of his elite career at age 40. His highest individual oxygen pulse value was recorded during his last visit to the laboratory as an elite athlete at age 40.
Performance measures
Maximal minute power, derived from the ramp exercise test, improved by 29% (from 413 W to 531 W) over the monitored period (). Most importantly, the difference between MMW over the 11 years of assessment, and the mean power output sustained during all-out 2000-m test on a rowing ergometer, also over the same 11-year period, was only ~6 W, with individual differences on an annual basis never exceeding ~15 W. This observation, along with those of Ingham et al. (Citation2013), who reported an identical ~6 W difference between MMW and 2000-m power in a cross-sectional study involving trained rowers, and the earlier findings of Bourdin et al. (Citation2004), who reported a difference between “peak power” and 2000-m power of ~13 W in a large group of highly-trained rowers, supports MMW as a valid and useful measure in monitoring rowing performance. Additionally, unlike determination of V̇O2max that tends to require complex laboratory equipment, MMW could be easily derived from a ramp-wise test for which only a rowing ergometer is needed. This likely presents a further advantage for coaches monitoring changes in the performance capability of their rowers.
Figure 2. Improvements in maximal minute power, derived from the ramp exercise test, versus age. Markers are mean values for both rowers.
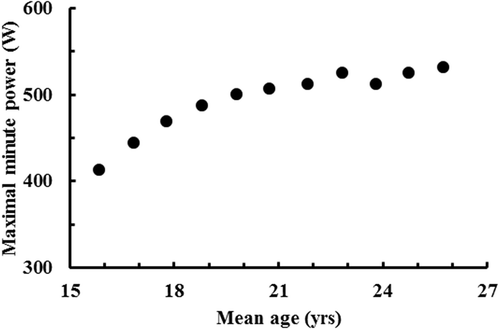
A 12-year improvement of 28% and 33% occurred in mean power output sustained over 2000 m and 6000 m on a rowing ergometer, respectively (). It should be noted that the highest values for the mean power output, both for 2000 and 6000-m tests, were recorded during the penultimate year of the assessment, when the rowers’ mean age was 26 years. These mean values of 542 W for 2000 m and 465 W for 6000 m, achieved during the 2015 season, corresponded to mean performance times of 5 min 45.8 s for 2000 m and 18 min 11.5 s for 6000 m, and include personal best times for both rowers in both ergometer tests. While V̇O2max plateaus after about 22 years of age, rowing ergometer performance improves (with minor fluctuations) steadily over the monitored period. It is encouraging for the future of the crew that the last two years of the assessment provided the best single 12-year mean performance times both for 2000-m and 6000-m ergometer performance. These improvements over the last few years of the assessment are largely independent of changes in V̇O2max, as the value was stable from 2011, when the rowers were 22 years old. Other physiological and performance factors, possibly related to the production of energy by anaerobic energy pathways not detectable by oxygen consumption, and improved rowing technique, could explain this observation.
Conclusion
Based on the annual testing lasting more than a decade, this case study described the physiological and performance development of two rowing athletes who represent the true elites of their sport. Their V̇O2max (l·min−1) gradually increased to approximately 22 years of age and plateaued at approximately 7 l·min−1 with minimal fluctuations thereafter. However, while the V̇O2max remained stable over the last few years of the assessment, the variables that influence it changed. Specifically, while the age-related decline in maximal heart rate was about 0.5 beats·year−1 over the course of 11 years, oxygen pulse, which serves as an indirect measure of the stroke volume, correspondingly increased by about 1 ml O2·beat−1·yr−1, allowing the athletes to maintain exceptionally high V̇O2max. The maximal minute power of the studied rowers, derived each year from their ramp-wise tests, closely resembled the corresponding mean power output sustained during the 2000-m all-out tests on a rowing ergometer. The findings contribute to the body of knowledge on athletes representing the true elites of their respective sports.
Disclosure statement
No potential conflict of interest was reported by the authors.
References
- Bassett, D. R., Jr., & Howley, E. T. (2000). Limiting factors for maximum oxygen uptake and determinants of endurance performance. Medicine and Science in Sports and Exercise, 32(1), 70–84. doi:10.1097/00005768-200001000-00012
- Bird, S., & Davidson, R. C. R. (1997). Physiological testing guidelines (3rd ed.). Leeds, UK: British Association of Sport and Exercise Science.
- Bourdin, M., Messonnier, L., Hager, J. P., & Lacour, J. R. (2004). Peak power output predicts rowing ergometer performance in elite male rowers. International Journal of Sports Medicine, 25(5), 368–373. doi:10.1055/s-2004-815844
- Godfrey, R. J., Ingham, S. A., Pedlar, C. R., & Whyte, G. P. (2005). The detraining and retraining of an elite rower: A case study. Journal of Science and Medicine in Sport, 8(3), 314–320. doi:10.1016/S1440-2440(05)80042-8
- Ingham, S. A., Carter, H., Whyte, G. P., & Doust, J. H. (2007). Comparison of the oxygen uptake kinetics of club and olympic champion rowers. Medicine and Science in Sports and Exercise, 39(5), 865–871. doi:10.1249/mss.0b013e31803350c7
- Ingham, S. A., Pringle, J. S., Hardman, S. L., Fudge, B. W., & Richmond, V. L. (2013). Comparison of step-wise and ramp-wise incremental rowing exercise tests and 2000-m rowing ergometer performance. International Journal of Sports Physiology and Performance, 8(2), 123–129. doi:10.1123/ijspp.8.2.123
- Lacour, J. R., Messonnier, L., & Bourdin, M. (2009). Physiological correlates of performance. Case study of a world-class rower. European Journal of Applied Physiology, 106(3), 407–413. doi:10.1007/s00421-009-1028-3
- Mikulic, P. (2011). Maturation to elite status: A six-year physiological case study of a world champion rowing crew. European Journal of Applied Physiology, 111(9), 2363–2368. doi:10.1007/s00421-011-1870-y
- Mortensen, S. P., Dawson, E. A., Yoshiga, C. C., Dalsgaard, M. K., Damsgaard, R., Secher, N. H., & Gonzalez-Alonso, J. (2005). Limitations to systemic and locomotor limb muscle oxygen delivery and uptake during maximal exercise in humans. Journal of Physiology, 566(Pt 1), 273–285. doi:10.1113/jphysiol.2005.086025
- Nybo, L., Schmidt, J. F., Fritzdorf, S., & Nordsborg, N. B. (2014). Physiological characteristics of an aging Olympic athlete. Medicine and Science in Sports and Exercise, 46(11), 2132–2138. doi:10.1249/MSS.0000000000000331
- Pimentel, A. E., Gentile, C. L., Tanaka, H., Seals, D. R., & Gates, P. E. (2003). Greater rate of decline in maximal aerobic capacity with age in endurance-trained than in sedentary men. Journal of Applied Physiology (1985), 94(6), 2406–2413. doi:10.1152/japplphysiol.00774.2002
- Schabort, E. J., Hawley, J. A., Hopkins, W. G., & Blum, H. (1999). High reliability of performance of well-trained rowers on a rowing ergometer. Journal of Sports Sciences, 17(8), 627–632. doi:10.1080/026404199365650
- Secher, N. H. (2000). Rowing. In R. J. Shephard & P. O. Astrand (Ed.), Endurance in sport (pp. 836–843). Oxford: Blackwell Science.
- Tanaka, H., Monahan, K. D., & Seals, D. R. (2001). Age-predicted maximal heart rate revisited. Journal of the American College of Cardiology, 37(1), 153–156. doi:10.1016/S0735-1097(00)01054-8
- Tanaka, H., & Seals, D. R. (2008). Endurance exercise performance in masters athletes: Age-associated changes and underlying physiological mechanisms. Journal of Physiology, 586(1), 55–63. doi:10.1113/jphysiol.2007.141879