ABSTRACT
This study aimed to compare the significant turning demands of English Premier League soccer match play relative to playing position using LiDAR technology. Turning data were collected from an English Premier League soccer team (2020–2021 season; 18 fixtures) using a Sportlight® LiDAR tracking system. Turns were tracked during match play, sub-categorised by entry speed (<3.0, 3.0–5.5, 5.5–7.0 and >7.0 m/s) and turning angle (Low: 20–59°; Medium: 60–119°; High: 120–180°). Turning metric frequencies were compared between playing positions (centre backs, full-backs, central midfielders, wide midfielders, and central forwards). On average, per match, central midfielders performed more total turns (~38 vs ~18–27), turns with entry speeds <3.0 (~15 vs ~7–10) and 3.0–5.5 m/s (~21 vs ~8–15) and low (~4 vs ~1–2), medium (~10 vs ~3–6) and high angled turns (~24 vs ~12–18) compared to all other playing positions (p ≤ 0.001, d = 0.96–2.74). Approximately, 90% of turns during matches were performed with entry speeds <5.5 m/s and ~63–70% were high angled turns. This study provides unique insights into the turning demands of English Premier League soccer matches , which can be used to inform position-specific physical preparation strategies, turning testing battery selection, agility drill construction, and rehabilitation and return to play standards.
1. Introduction
Soccer is a high-intensity intermittent sport whereby players perform numerous locomotor, technical, and tactical actions for attacking and defensive purposes (Bradley & Ade, Citation2018). Our understanding of traditional high-intensity locomotor activities in soccer, such as the distances and frequency of high-speed running, sprints, accelerations, and decelerations, has improved over recent years (Bradley & Ade, Citation2018; Harper et al., Citation2019; Nassis et al., Citation2020; Novak et al., Citation2021), particularly due to the advancements in wearable and non-wearable player tracking devices and software (Nassis et al., Citation2020; Novak et al., Citation2021; Taberner et al., Citation2019). However, soccer players may transition from the aforementioned actions by performing a turning manoeuvre (synonymous with change of direction [COD]; Bloomfield et al., Citation2007; Bradley & Ade, Citation2018). Turning actions preceding sprinting have been reported to be associated with contextually decisive moments in soccer (Caldbeck, Citation2020; Faude et al., Citation2012). Turns are considered important from an attacking and defensive standpoint, as they are associated with goal scoring, supporting team-mates in possession, creating and denying space, tackling, and pressing opponents (Ade et al., Citation2016; Bloomfield et al., Citation2007; Bradley & Ade, Citation2018). Because soccer turning actions are linked to key performance outcomes (Caldbeck, Citation2020; Faude et al., Citation2012; Martínez Hernández et al., Citation2022), have the propensity to elicit high mechanical loading (Dos’Santos et al., Citation2018; Dos’ Santos et al., Citation2021; Kalkhoven et al., Citation2021), tissue damage (Harper & Kiely, Citation2018; McBurnie et al., Citation2021) and fatigue (Harper & Kiely, Citation2018; McBurnie et al., Citation2021), and can be a non-contact injury mechanism (Della Villa et al., Citation2020; Serner et al., Citation2019), understanding the turning demands of soccer match play is of great interest to practitioners to better inform physical preparation and assessment strategies. This is particularly pertinent in the English Premier League where the physical demands of soccer match play have evolved (Barnes et al., Citation2014) and are envisaged to rise over the next 10 years (Harper et al., Citation2021; Nassis et al., Citation2020).
Turning activity has traditionally been assessed during soccer match play using visual observations and notational (video) analysis (Ade et al., Citation2016; Bloomfield et al., Citation2007; Morgan et al., Citation2021; Nedelec et al., Citation2014; Robinson et al., Citation2011). Bloomfield et al. (Bloomfield et al., Citation2007) previously reported that English Premier League soccer players perform ~600 turns (i.e., qualitative evaluation of movement based on circle) of 0–90° (i.e., ¼ circle) and ~100 turns of 90–180° (i.e., ¼–½ circle) per match, inclusive of low to high-intensity actions, with defenders and strikers performing more turns than midfielders. Robinson et al. (Robinson et al., Citation2011) later reported that English Premier League soccer players perform ~80 turns (i.e., qualitative path change with two relatively straight movements with a turn in the middle of them) of 45–135° and ~20 turns of 135–180° containing an action ≥4.0 m/s per match, with midfielders performing the greatest frequency of turns compared to other positional groups. Conversely, Ade et al. (Ade et al., Citation2016), using the high-intensity movement programme classification (similar to Bloomfield et al. (Bloomfield et al., Citation2007)), reported centre backs performed the greatest 0–90° turning frequency in English Premier League soccer compared to other positional groups. More recently, however, Morgan et al. (Morgan et al., Citation2021) established that English Premier League academy soccer players performed ~300 turns (i.e., qualitative path change caused by an identifiable plant of a leg leading to change in path travelled) per match with no position-related differences. Interestingly, 77% of turns were 0–90° compared to 23% of turns which were >90° (Morgan et al., Citation2021), but it is worth noting that these included low to high-intensity efforts pre and post turn (excluding walking). While the aforementioned research has provided further insights into the turning demands of soccer match play, notational and qualitative analysis is subjective and time-consuming which restricts its feasibility in practice for turn and load monitoring. Additionally, these studies only provide a limited representation of the seasonal demands of turning activity, with specific English Premier League turning research 7–17 years old (Ade et al., Citation2016; Bloomfield et al., Citation2007; Robinson et al., Citation2011), and therefore unlikely to be representative of the turning demands of the modern game.
Wearable technologies have recently been implemented for soccer turn and load monitoring, which may provide novel insights into instantaneous speed and turning angle (Baptista et al., Citation2018; Granero-Gil, Bastida-Castillo et al., Citation2020; Granero-Gil, Gómez-Carmona et al., Citation2020; Kai et al., Citation2021). Granero-Gil et al. (Granero-Gil, Bastida-Castillo et al., Citation2020; Granero-Gil, Gómez-Carmona et al., Citation2020) examined the turning demands of a Russian Premier League soccer team over the course of season using a device with embedded accelerometers, gyroscopes, and global positioning system. The authors reported ~600 turns were performed during soccer match play, of which ~60 and ~15 was performed at high (>16 km/hr; 4.44 m/s) and maximum intensity (>21 km/hr; 5.83 m/s), respectively, but the authors did not clearly define their turn classification criteria and definition nor delineate where and how speed was obtained during the turn. Additionally, position-related differences were observed in the turning activities (Granero-Gil, Gómez-Carmona et al., Citation2020); however, it is worth noting that they did not provide specific information regarding turning angle. As the biomechanical demands of turning are angle- and velocity dependent (Dos’Santos et al., Citation2018; Dos’ Santos et al., Citation2021), and turning angle and entry speed can have distinct implications for the potential technique, strength requirements, braking and propulsive requirements, muscle activation, mechanical loading and potential tissue damage (Dos’Santos et al., Citation2018; Dos’ Santos et al., Citation2021; Kalkhoven et al., Citation2021; McBurnie et al., Citation2021), understanding the turning demands of match play with respect to angle and entry speed can be considered highly important to better inform physical preparation of soccer players. To our best knowledge, using a local positioning system wearable device, only one study (Kai et al., Citation2021) has examined the turning activity of soccer match play with respect to angle and instantaneous speed, reporting no position-related differences in turns (i.e., calculated based on speed, acceleration, jerk, and directional angle utilising x-y coordinate data), over 15° ranges, with the majority of turns performed with speeds at the onset of turn <5.0 m/s. However, this study was limited to Japanese collegiate and amateur soccer players, was only representative of nine matches for turns which contained an acceleration ≥2 m/s2, and utilised a wearable technology. Some players are reluctant to wear devices for load monitoring purposes, thus athlete compliance can be a problematic issue when using the aforementioned technology. Nevertheless, adopting a similar approach to Kai et al. (Kai et al., Citation2021) with respect to turning angle and speed, further data is needed pertaining to turning of English Premier League soccer match play.
One solution to accurately quantify the turning demands of soccer match play is using light detection and ranging technology (LiDAR) technology. This emerging technology emits infrared lasers to measure distances and locations of objects relative to the LiDAR sensor and has been recently employed in other industries, such as transportation (Williams et al., Citation2013), ecological sciences (Eitel et al., Citation2016), and motion tracking (Clark et al., Citation2019; Dewan et al., Citation2016). Sportlight Technology Ltd has recently developed a LiDAR player tracking system for the continuous quantification of key physical metrics (i.e., total distance, high-speed running, sprint speed, accelerations, decelerations, turns) based on the distance and location of the player. This system has been recently validated against the gold standard of three-dimensional motion analysis during football-specific movements (i.e., jogging, linear sprinting, curved sprinting, and turns of various angles; Bampouras & Thomas, Citation2022), with trivial to small differences in time spent during different speed zones (d = 0.04–0.26) observed, and root mean square error values of 0.04–0.014 m/s and 0.16–0.7 m/s2 for velocity and acceleration data, respectively. Importantly, this system only consists of a single unit which can be placed in indoor and outdoor environments for player tracking. As such, due to its potential portability, and the fact that it does not require athletes to wear a device, the Sportlight® system appears to be a non-invasive and attractive solution for continuous player tracking and monitoring of turning during competitive match play.
To our best knowledge, no study has quantified the turning demands of English Premier League soccer match play with accurate and comprehensive information pertaining to angle and entry speed. Additionally, mixed observations have been observed in relation to position-related differences in turning activity during soccer match play (Ade et al., Citation2016; Baptista et al., Citation2018; Bloomfield et al., Citation2007; Granero-Gil, Gómez-Carmona et al., Citation2020; Kai et al., Citation2021; Morgan et al., Citation2021; Robinson et al., Citation2011). This could potentially be attributed to differences in turn classifications adopted within the literature. For example, researchers via observational analysis have included low to high intensity turning movements (Bloomfield et al., Citation2007), low to high intensity turning movements with a clear foot plant (excluding walking; Morgan et al., Citation2021), and observations of turns with an action ≥4 m/s (Robinson et al., Citation2011). Additionally, researchers using objective methods such as accelerometers, gyroscopes and global positioning system have provided unclear turning classification criteria (Granero-Gil, Bastida-Castillo et al., Citation2020; Granero-Gil, Gómez-Carmona et al., Citation2020), while Kai et al. (Kai et al., Citation2021) using local positioning system wearable device quantified turns which contained an acceleration ≥2 m/s2. Furthermore, most studies which have quantified turns have included turns performed at low intensity (Baptista et al., Citation2018; Bloomfield et al., Citation2007; Granero-Gil, Gómez-Carmona et al., Citation2020; Morgan et al., Citation2021) which are likely to elicit limited mechanical loading, fatigue, and tissue damage (Dos’Santos et al., Citation2018; Dos’ Santos et al., Citation2021; Harper & Kiely, Citation2018; Kalkhoven et al., Citation2021; McBurnie et al., Citation2021). Conversely, significant turns, which in the current study are described as “an occurrence where a player completes a deceleration (≤ −2 m/s2), an angle change in direction of travel (≥20°) and a subsequent acceleration (≥2 m/s2) within a 1 second duration” are likely to be of greater interest to practitioners due to their importance for decisive match-related outcomes such as goal scoring and assists (Caldbeck, Citation2020; Faude et al., Citation2012; Martínez Hernández et al., Citation2022), and their potential to generate greater mechanical loading, particularly knee joint loading (Dos’Santos et al., Citation2018; Dos’ Santos et al., Citation2021; Kalkhoven et al., Citation2021), fatigue (Harper & Kiely, Citation2018; McBurnie et al., Citation2021), and tissue damage (Harper & Kiely, Citation2018; McBurnie et al., Citation2021) from the potentially damaging nature of significant turning.
Therefore, the aim of this study, was to present and compare the significant turning demands of English Premier League soccer match play relative to playing position using LiDAR technology. It was hypothesised that positional differences would exist in turning activity, with central midfielders performing the greatest turning frequency. It is expected that the results of the research will improve our understanding of the physical demands of soccer match play and can be used to assist practitioners in the development of physical preparation strategies, position-specific agility and multidirectional speed (MDS) drill construction, turning testing battery selection and return to play protocols for rehabilitating athletes.
2. Methods
2.1 Research design
This study used a longitudinal between-subject comparative design, whereby turn metrics were compared between soccer players’ positional groups of an English Premier League soccer team.
2.2 Match analysis and player data
Turning data were collected from a single English Premier League soccer team over 18 home fixtures during the 2020–2021 season using a Sportlight® LiDAR tracking system (Sportlight®, Oxford, UK; LiDAR). This system included a single sensor head consisting of a high-resolution LiDAR sensor sampling 1.2 million spatial readings/second over a 200 m range at 10 Hz, which was mounted in the football stand approximately 7 m above the pitch, close to the halfway line. The LiDAR system tracked all movement on the pitch by determining a ground plane (the pitch), a background model (static objects), and a foreground model (all moving points within the LIDAR data) using a proprietary software. Clusters of foreground points were identified to localize players. The proprietary software then identified the centre of each cluster, which has been shown to provide accurate positional information when compared with a 3D motion capture system using a four-marker pelvis model (Bampouras & Thomas, Citation2022). Additionally, three cameras collected high-resolution imagery (Sony IMX253, 12.4MPx, 10fps synchronized with the LiDAR data) which was used as the input to an artificial intelligence system for temporal tracking of individual clusters and to re-identify players from previously collected imagery. Gait-neutralised velocity was calculated by filtering the raw velocity data with a fourth order low-pass Butterworth filter (1 Hz cut-off; Bampouras & Thomas, Citation2022). Gait-neutralised acceleration was defined as a change in the gait-neutralised velocity over time (Bampouras & Thomas, Citation2022). The output from the Sportlight® system then provided positional information and derived metrics for turning.
A total of 22 players (age: 29.8 ± 3.1 years; height: 1.83 ± 0.04 m; mass: 83.5 ± 6.6 kg) were tracked during match play multiple times and were analysed across five positions similar to previous research (Ade et al., Citation2016): centre back (CB: n = 5), full-back (FB: n = 5), central midfielder (CM: n = 7), wide midfielder (WM: n = 8) and central forward (CF: n = 8). These observations were collected over 18 home fixtures (3 wins, 6 draws, and 9 losses) on an outdoor natural grass pitch, with a consistent 4-4-2 playing formation. If a starting player was substituted by a like-for-like replacement, the data were still treated as one observation, similar to previous procedures (Caldbeck, Citation2020). This resulted in 36 total observations per position group. The number of substitutions by playing position over the 18 fixtures was as follows: FBs one substitution, CBs no substitutions, WMs 13 substitutions over 10 matches; CMs four substitutions over three matches and CFs 15 substitutions over 13 matches. Ethical approval was granted by the university institutional review board (ID:39846), and the soccer team provided informed written consent to publish the data for research purposes.
2.3 Turning metrics
Players’ significant turns were tracked during match play, which was defined as an occurrence where a player completed a deceleration (≤−2 m/s2), an angle change in direction of travel (≥20°), and a subsequent acceleration (≥2 m/s2) within a 1 second duration. These thresholds are typically used to classify accelerations and decelerations above a moderate intensity level during team-sport match play (Delves et al., Citation2021; Newans et al., Citation2019). The significant turns were further sub-categorised by entry speed (<3.0 m/s, 3.0–5.5 m/s, 5.5–7.0 m/s and >7.0 m/s) and turning angle (Low: 20–59°; Medium: 60–119°; and High: 120–180°). The entry speed was classified as the instantaneous speed of the player upon initiation of the deceleration (i.e., when a player’s deceleration exceeded ≤ −2 m/s2 threshold). The angle of turn was calculated as the angle between the deceleration and acceleration vector in the horizontal plane based on coordinates of the centre of the cluster (i.e., estimated whole-body centre of mass; Bampouras & Thomas, Citation2022). The frequency of total significant turns and sub-categories of entry speed and turning angle were calculated for each playing position, with the average, standard deviation, maximum and minimum values also calculated.
2.4 Statistical analysis
Statistical analyses were performed using JAMOVI software (V1.6.7.0, Sydney, Australia; The jamovi project, Citation2021) and Microsoft Excel (version 2016, Microsoft Corp., Redmond, WA, USA). Normality for all variables was assessed using a Shapiro–Wilks test and confirmed that all variables did not meet the assumptions for a parametric test. The coefficient of variation (CV%) was calculated as SD/mean × 100 for each observation and then averaged across all observations to indicate intra-positional match to match variability (Ade et al., Citation2016). Turn metrics were compared across positions using a Kruskal–Wallis test with Dwass-Steel-Critchlow-Fligner pairwise comparisons in cases of significant differences. Epsilon squared effect sizes were also calculated for all Kruskal–Wallis tests. Standardized differences were calculated for all pairwise comparisons using Cohen’s d effect sizes (ES) and the following formula: Cohen d = M1 – M2/σ pooled, where σ pooled = the square root of (σ12 + σ22/2; Cohen, Citation1977) and interpreted as trivial (≤0.19), small (0.20–0.59), moderate (0.60–1.19), large (1.20–1.99), very large (2.00–3.99), and extremely large (≥4.00; Hopkins, Citation2002). Statistical significance was defined p < 0.05 for all tests. The average of all 36 observations per playing position was used for analysis, thus the presented data reflects average turning frequency per match. Finally, the proportion of total turns was split by turning angle and entry speed.
3. Results
Descriptive statistics for turn metrics for each positional group are presented in . The results of the Kruskal–Wallis test, pairwise comparisons with effect sizes, and match-to-match variability are presented in .
Table 1. Descriptive and positional group comparison for turning metrics.
A significant effect of positional group for all turning metrics was observed (). Specifically, CMs performed significantly more total turns, more turns with entry speeds of <3.0 m/s and 3.0–5.5 m/s, and low, medium and high angled turns compared to all other playing positions (moderate to very large ES; ). CMs also performed significantly more turns with entry speeds of 5.5–7.0 m/s compared to CBs and CFs (moderate ES; ).
WMs performed significantly more total turns, more turns with entry speeds of 3.0–5.5 m/s and medium and high angled turns compared to FBs, CBs and CFs (moderate to large ES; ). WMs also performed significantly more turns with entry speeds <3.0 m/s compared to CFs, and they also performed more turns with entry speeds of 5.5–7.0 m/s compared to CBs and CFs (moderate ES). Finally, FBs performed significantly more turns with entry speeds of 5.5–7.0 m/s compared to CBs and CFs (moderate ES), while CBs performed significantly less turns with entry speeds >7.0 m/s compared to WM and CMs (moderate ES; ). Match-to-match variability for all turning metrics was high (CV ≥ 20.7%), with large individual and positional variation (, , Supplementary material 1). The proportion of turns by entry speed and angle by positional group are presented in . Generally, ~90% of turns irrespective of playing position were <5.5 m/s, while ~70% of turns were high angled.
Figure 1. Box and whisker plots for Total turns (panel a), Low turns (20–59°) (panel b), Medium turns (60–119°) (panel c) and High turns (120–180°) (panel d). FB: Full backs; CB: Centre back; WM: Wide midfield; CM: Central midfield.
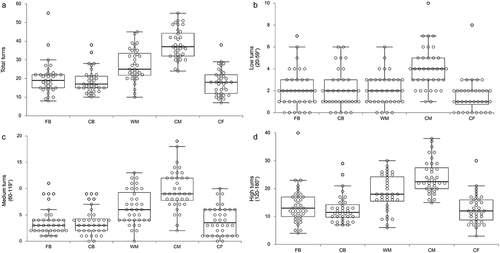
Figure 2. Box and whisker plots for turns with entry speeds <3.0 m/s (panel a), turns 3.0–5.5 m/s (panel b), turns 5.5–7.0 m/s (panel c) and turns >7.0 m/s (panel d). FB: Full backs; CB: Centre back; WM: Wide midfield; CM: Central midfield.
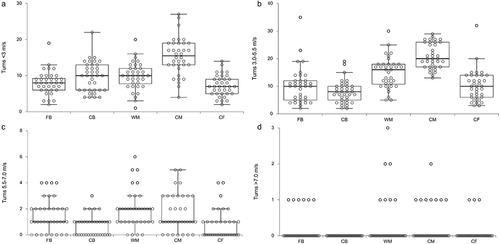
Table 2. Proportion of turns by entry speed and angle across playing position groups.
4. Discussion
This is the first study to comprehensively examine the significant turning demands of English Premier League soccer match play with respect to turning frequency, entry speed, and angle, using LiDAR technology, with previous turning research in this area 7–17 years old and limited to notational analysis (Ade et al., Citation2016; Bloomfield et al., Citation2007; Robinson et al., Citation2011). To our best knowledge, only one study has quantified turning demands in soccer, objectively quantifying both entry speed and angle (Kai et al., Citation2021); however, this study was limited to Japanese collegiate and amateur soccer players. The primary findings of the present study were that, on average, CMs perform double the number of significant turns per match (~38 vs ~19) compared to the other positions (CB, FBs, and CFs), with WMs performing the second most (~27; , ). These findings support the study hypothesis. Additionally, irrespective of playing position, most turns (~90%) during match play were performed with entry speeds <5.5 m/s (, ), while ~63–70% of turns were high angled across all positions (, ). Overall, this study provides unique insights into the physical and turning demands of English Premier League soccer match play, which can be used to assist and help inform position-specific physical preparation strategies, turning testing battery selection, contextually inform agility drill construction, and rehabilitation and return to play standards for soccer practitioners.
From a positional group perspective, CMs performed substantially more total turns, turns with entry speeds of <3.0 m/s and 3.0–5.5 m/s and low, medium and high angled turns compared to all other playing positions (, ). This observation is likely attributable to the dual attacking and defensive roles that CMs have, who therefore have more game involvements compared to the other positional groups who primarily have defensive or attacking responsibilities. Unfortunately, previous research into the turning activities in the English Premier League did not divide defenders and midfielders into central or wide positional groups (Bloomfield et al., Citation2007; Robinson et al., Citation2011), with defenders and strikers observed to perform more total turns than midfielders (Bloomfield et al., Citation2007). But it is worth noting that the turns were inclusive of lower intensity turns. Conversely, Robinson et al. (Robinson et al., Citation2011) only monitored turns that contained an action ≥4.0 m/s and observed that midfielders performed the most turns compared to defenders and strikers. More recently, however, no position-related differences in turns were demonstrated in Japanese soccer players (Kai et al., Citation2021) and English Premier League academy soccer players (Morgan et al., Citation2021), whereas Baptista et al. (Baptista et al., Citation2018) reported that Norwegian WMs and FBs performed significantly more turns >90° than CBs, but unfortunately they did not classify turns of <90°. Ade et al. (Ade et al., Citation2016) observed that English Premier League soccer CBs performed more high-intensity effort 0–90° turns compared to FBs, CMs and WMs, while in Russian Premier League soccer players, CBs were observed to perform the most total turns, whereas CMs and CBs performed the most turns >16 km/hr (>4.44 m/s) and >21 km/hr (>5.83 m/s), respectively (Granero-Gil, Gómez-Carmona et al., Citation2020). Consequently, the lack of consensus on positional group turning activity across the soccer literature is likely attributed to methodological differences, definitions, classifications, inclusion and exclusion criteria of turns (Morgan et al., Citation2021), while player physical capacity and team playing style and tactical differences are likely to influence turning outcomes.
Although, on average, CMs performed a greater number of significant turns compared to CBs, FBs, CFs (~38 vs ~19) and WMs (~38 vs ~27), it is worth noting that large match-to-match variability was displayed, with turning frequencies as low as 7 (CFs) to as high as 55 (WM and FB) observed (, ). While most statistical analysis procedures are based on group mean analysis and comparison, to better prepare players for the physical demands of match play, it is vital to consider the maximum demands (i.e., potential worst-case scenarios; Novak et al., Citation2021). and supplementary material 1 illustrate the variability of turning frequency metrics between fixtures, which are likely attributed to a range of factors including tactical and contextual factors (i.e., playing formation, style of play, attacking and defensive organisation and transition), player physical capacity, and possible fatigue (Novak et al., Citation2021). Nevertheless, the results of this study related to the turning demands have significant implications for the physical preparation strategies of English Premier League soccer players, particularly for CMs who consistently perform the most turns (, ), and FBs and WMs who on occasions also display similar and high turning frequencies to CMs. These players are likely to experience greater mechanical loading, particularly knee joint loading (Dos’Santos et al., Citation2018; Dos’ Santos et al., Citation2021; Kalkhoven et al., Citation2021), fatigue (Harper & Kiely, Citation2018; McBurnie et al., Citation2021), and tissue damage (Harper & Kiely, Citation2018; McBurnie et al., Citation2021) from the potentially damaging nature of significant turning that contain high acceleration and deceleration demands. Thus, CMs, FBs, and WMs must be physically conditioned and robust to tolerate the significant turning demands associated with elite soccer match play compared to other positions to optimise performance and mitigate injury risk. Moreover, from a sports medicine perspective, the average and maximum data presented in this study can be used to provide position-specific return to play standards. This, in turn, may better inform rehabilitation practices of soccer players to ensure they are sufficiently physically prepared to tolerate the competitive turning demands associated with elite match play (Buckthorpe, Citation2021; Taberner et al., Citation2019).
Interestingly, ~90% of turns during soccer match play were performed with entry speeds <5.5 m/s (), whereas turns with entry speeds >7.0 m/s were rarely performed (~1%), with CBs failing to perform a turn at the aforementioned entry speed across 18 matches (). To our best knowledge, only two other research groups have quantified objective measures of instantaneous speed during turning in soccer players (Granero-Gil, Bastida-Castillo et al., Citation2020; Granero-Gil, Gómez-Carmona et al., Citation2020; Kai et al., Citation2021). For example, Granero-Gil et al. (Granero-Gil, Bastida-Castillo et al., Citation2020; Granero-Gil, Gómez-Carmona et al., Citation2020) observed Russian Premier League players during matches performed ~60 and ~15 turns at high (>16 km/hr; 4.44 m/s) and maximum intensity (>21 km/hr; 5.83 m/s), respectively. The researchers tracked turning activity with a wearable device (embedded accelerometers, gyroscopes, and global positioning system); however, they failed to specify how and where speed was calculated during the turn. Conversely, Kai et al. (Kai et al., Citation2021) examined the speed at turn onset (based on x-y coordinates) using a local positioning system and a jerk threshold to verify turn onset (i.e., when jerk changed negative to positive). Similar to our findings, most turns performed by Japanese soccer players were <5.0 m/s, with turns >5.0 m/s rarely observed (maximum 6.99 m/s; Kai et al., Citation2021). Collectively, these findings highlight the importance of turning ability, particularly at low to moderate entry speed (≤5.5 m/s).
Therefore, to better evaluate turning abilities and to better construct turning drills which are more representative of elite soccer match play, practitioners may consider turning tests and constructing and prioritising turning drills (i.e., agility training volume) with low to moderate entry speeds for potentially greater sports specificity. For example, turning ability could be examined using COD speed tests from short approach distances (i.e., 5-m), as the entry speeds for 45° (~5.2 m/s), 90° (~4.5 m/s) and 180° (~4.0 m/s) CODs assessed via 3D motion analysis (Dos’ Santos et al., Citation2021) are similar to the entry speeds observed within the current study. Additionally, to further standardise entry speed assessments during COD, practitioners can use two pairs of electronic timing gates over a two-to-three-metre distance prior to changing direction to calculate average speed (i.e., approximation of entry speed). This approach ensures that athletes fall within a target entry speed which is standardised across participants and for longitudinal monitoring. Practitioners are, however, advised not to completely neglect conditioning and assess players for rare events of turns with higher entry speeds.
Notably, ~63–70% of turns were high angled across all positions (), contrasting with the observations of previous research who generally observe ~70–80% of turns during soccer are 0–90° (Ade et al., Citation2016; Bloomfield et al., Citation2007; Morgan et al., Citation2021) or 45–135° (Robinson et al., Citation2011). Conversely, Baptista et al. (Baptista et al., Citation2018) observed 90–180° turns were more frequently performed than ≥180° turns, but they failed to quantify turns of 0–90°. The aforementioned researchers generally use large angle ranges (90°) when quantifying turns by angle, and they typically determine turning angle through visual inspection which can be susceptible to human error (Krosshaug et al., Citation2007). In contrast, Kai et al. (Kai et al., Citation2021) provided objective turning angle data within 15° ranges, observing 0–15° and 105–135° turns tended to most frequently occur during soccer match play. The differences in total turns and proportion of turns by angle observed in the present study and previous literature (Ade et al., Citation2016; Baptista et al., Citation2018; Bloomfield et al., Citation2007; Kai et al., Citation2021; Morgan et al., Citation2021) are likely attributable to the methodological differences and definitions, classifications, inclusion, and exclusion criteria of turns (Morgan et al., Citation2021). For instance, some researchers (Bloomfield et al., Citation2007; Granero-Gil, Bastida-Castillo et al., Citation2020; Granero-Gil, Gómez-Carmona et al., Citation2020; Morgan et al., Citation2021) when quantifying turns do not use any specific thresholds for speed, acceleration, deceleration, or turn duration, which results in a greater turning frequency observed during soccer match play (~300-700 vs 20–40), in contrast to the present study’s significant turn definition. Moreover, Robinson et al. (Robinson et al., Citation2011) observed a lower turn frequency (~100) when using a minimum 4 m/s threshold for actions during turning, while Kai et al. (Kai et al., Citation2021) reported ~180 turns per match play were observed, with turns having to contain an acceleration ≥2 m/s2 with no restrictions for turn duration for quantification. Collectively, the aforementioned turn classifications differ to the present study which only examined “significant turns” which arguably, based on the deceleration and acceleration thresholds, and turn durations required, may omit the quantification of shallow angled turns with low deceleration due to the angle-velocity trade-off (Dos’Santos et al., Citation2018) and generally limited deceleration demands associated with these tasks (Dos’Santos et al., Citation2018; Hader et al., Citation2015). Further research is therefore required utilising the LiDAR technology to quantify turns using a range of potentially more sensitive threshold (i.e., 1 m/s2) to determine its impact on turn evaluation during soccer match play.
Nonetheless, the results of this study highlight the importance and frequency of high angled turns (as high as 40) in soccer, and these turns are likely to induce greater mechanical loading and potential tissue damage and thus potential injury risk, compared to shallower angled directional changes (Dos’Santos et al., Citation2018; Dos’ Santos et al., Citation2021; Harper & Kiely, Citation2018; Kalkhoven et al., Citation2021; McBurnie et al., Citation2021). Therefore, practitioners should consider developing their athletes’ physical capacity and tissue robustness, particularly eccentric lower-limb strength, to tolerate the greater mechanical loading and braking demands associated with sharper turning to mitigate injury risk and tissue damage (Dos’Santos et al., Citation2018; Dos’ Santos et al., Citation2021; Harper & Kiely, Citation2018; Lloyd & Buchanan, Citation2001; Maniar et al., Citation2020; McBurnie et al., Citation2021). Additionally, practitioners should consider including high angled turning (i.e., COD speed) tests as part of their MDS profiling assessments for soccer players while potentially dedicating larger volumes of MDS training and designing drills to develop high angled turning ability.
It should be noted that the turning data of the present study are representative of the specific team, formation, skill level, and athletes’ physical capacity, and will likely be influenced by the tactics and style of play, contextual factors, and opposition. Therefore, practitioners should be cautious in generalising these findings to other populations; thus, future research is needed investigating the soccer turning demands of different playing levels, ages, and sexes. Additionally, the data were collected during 18 home fixtures during a season with COVID-19 and thus, a shortened physical preparatory period with periods of fixture congestion compared to traditional seasons. Moreover, it should be noted that the turning demands are representative of the turning classification (i.e., definition and criteria) and technology adopted within this study, which differs to previous work (Ade et al., Citation2016; Baptista et al., Citation2018; Bloomfield et al., Citation2007; Granero-Gil, Gómez-Carmona et al., Citation2020; Kai et al., Citation2021; Morgan et al., Citation2021; Robinson et al., Citation2011). For example, arbitrary acceleration (≥2 m/s2) and deceleration (≤−2 m/s2) thresholds, with a 1-s duration, were used to classify turns, which meant turns that did not achieve the criteria were omitted from further analysis in this study. Additionally, the current study did not provide the average playing time for each position, did not distinguish turns of different magnitudes of deceleration and acceleration preceding or following the turn, did not examine exit speed or the specific entry speeds within the angle bands, and the aforementioned thresholds used were arbitrary and not relative to the individual player’s acceleration or deceleration capacities. Moreover, while turning frequency is of interest, further research into the contextual classification of the turning actions is warranted, such as using the high-intensity movement programme classification framework to better help contextualise turning activity in relation to soccer performance (Ade et al., Citation2016; Bradley & Ade, Citation2018; Caldbeck, Citation2020). Finally, future work should consider quantifying the turning demands utilising this technology during training activities to establish whether they are reflective of the physical demands of match play.
Nevertheless, this study provides unique insights into the turning demands of English Premier League soccer match play, utilising an accurate, portable, non-wearable and non-invasive technology for monitoring turning activity during competitive match play. Overall, this study highlights that English Premier League soccer players require the physical capacity and mechanical turning ability to be able to perform turns of various angle (i.e., low, medium, and high) and from a variety of entry speeds to meet the physical demands of soccer match play, though greater training emphasis might be warranted for high angled and low to moderate entry speed turns.
5. Conclusion
This is the first study to comprehensively examine the significant turning demands of English Premier League soccer match play with respect to turning frequency, entry speed, and turning angle using an accurate, portable, non-invasive technology. Generally, on average, CMs perform double the number of significant turns (~38 vs ~19) compared to the other positions (CB, FBs, and CFs) with WMs performing the second most (~27). Additionally, irrespective of playing position, ~90% of turns during match play were performed with entry speeds <5.5 m/s, while ~63–70% of turns were high angled. Collectively, this study provides unique insights into the physical and turning demands of English Premier League soccer match-play, highlighting that soccer players require the physical capacity and turning ability to be able to perform turns of various angles (i.e., low, medium, and high) and from a variety of entry speeds. The results of this study can be used to assist and help inform position-specific physical preparation strategies, turning testing battery selection, contextually inform agility drill construction, and rehabilitation and return to play standards for soccer practitioners.
Supplemental Material
Download MS Word (50.9 KB)Disclosure statement
The study was funded by Sportlight Technology LTD which covered Thomas Dos’Santos’ services for contract research (Project ID: 526556). Ian Cowling and Paul Caldbeck are employed by Sportlight Technology LTD and are involved in the development of the product. Timothy Barry is a sports science consultant for Sportlight Technology LTD. No other potential conflict of interest is reported by the authors.
Supplementary material
Supplemental data for this article can be accessed online https://doi.org/10.1080/02640414.2022.2109355
Additional information
Funding
References
- Ade, J., Fitzpatrick, J., & Bradley, P. S. (2016). High-intensity efforts in elite soccer matches and associated movement patterns, technical skills and tactical actions. Information for position-specific training drills. Journal of Sports Sciences, 34(24), 2205–2214. https://doi.org/10.1080/02640414.2016.1217343
- Bampouras, T. M., & Thomas, N. M. (2022). Validation of a LiDAR-based player tracking system during football-specific tasks. Sports Engineering, 25(1). https://doi.org/10.1007/s12283-022-00372-7
- Baptista, I., Johansen, D., Seabra, A., Pettersen, S. A., & Di Giminiani, R. (2018). Position specific player load during match-play in a professional football club. PloS one, 13(5), e0198115. https://doi.org/10.1371/journal.pone.0198115
- Barnes, C., Archer, D., Hogg, B., Bush, M., & Bradley, P. (2014). The evolution of physical and technical performance parameters in the English Premier League. International Journal of Sports Medicine, 35(13), 1095–1100. https://doi.org/10.1055/s-0034-1375695
- Bloomfield, J., Polman, R., & Donoghue, P. (2007). Physical demands of different positions in FA Premier League soccer. Journal of Sports Science and Medicine, 6 (1) , 63–70.
- Bradley, P. S., & Ade, J. D. (2018). Are current physical match performance metrics in elite soccer fit for purpose or is the adoption of an integrated approach needed? International Journal of Sports Physiology and Performance, 13(5), 656–664. https://doi.org/10.1123/ijspp.2017-0433
- Buckthorpe, M. (2021). Recommendations for movement re-training after ACL reconstruction. Sports Medicine, 51(8), 1601–1618. https://doi.org/10.1007/s40279-021-01454-5
- Caldbeck, P. Contextual Sprinting in Premier League Football. Doctoral Thesis, Liverpool John Moores University, 2020.
- Clark, R. A., Pua, Y.-H., Bower, K. J., Bechard, L., Hough, E., Charlton, P. C., & Mentiplay, B. (2019). Validity of a low-cost laser with freely available software for improving measurement of walking and running speed. Journal of Science and Medicine in Sport, 22(2), 212–216. https://doi.org/10.1016/j.jsams.2018.07.005
- Cohen, J. (1977). Statistical power analysis for the behavioral sciences (rev. Erlbaum.
- Della Villa, F., Buckthorpe, M., Grassi, A., Nabiuzzi, A., Tosarelli, F., Zaffagnini, S., & Della Villa, S. (2020). Systematic video analysis of ACL injuries in professional male football (soccer): Injury mechanisms, situational patterns and biomechanics study on 134 consecutive cases. British Journal of Sports Medicine, 54(23), 1423–1432. https://doi.org/10.1136/bjsports-2019-101247
- Delves, R. I., Aughey, R. J., Ball, K., & Duthie, G. M. (2021). The quantification of acceleration events in elite team sport: A systematic review. Sports Medicine - Open, 7(1), 1–35. https://doi.org/10.1186/s40798-021-00332-8
- Dewan, A., Caselitz, T., Tipaldi, G. D., & Burgard, W. Motion-based detection and tracking in 3d lidar scans. Presented at 2016 IEEE international conference on robotics and automation (ICRA), 2016.
- Dos’ Santos, T., Thomas, C., & Jones, P. A. (2021). The effect of angle on change of direction biomechanics: Comparison and inter-task relationships. Journal of Sports Sciences 39(22) , 2618–2631. https://doi.org/10.1080/02640414.2021.1948258
- Dos’Santos, T., Thomas, C., Comfort, P., & Jones, P. A. (2018). The effect of angle and velocity on change of direction biomechanics: An angle-velocity trade-off. Sports Medicine, 48(10), 2235–2253. https://doi.org/10.1007/s40279-018-0968-3
- Eitel, J. U., Höfle, B., Vierling, L. A., Abellán, A., Asner, G. P., Deems, J. S., Glennie, C. L., Joerg, P. C., LeWinter, A. L., & Magney, T. S. (2016). Beyond 3-D: The new spectrum of lidar applications for earth and ecological sciences. Remote Sensing of Environment, 186(1) , 372–392. https://doi.org/10.1016/j.rse.2016.08.018
- Faude, O., Koch, T., & Meyer, T. (2012). Straight sprinting is the most frequent action in goal situations in professional football. Journal of Sports Sciences, 30(7), 625–631. https://doi.org/10.1080/02640414.2012.665940
- Granero-Gil, P., Bastida-Castillo, A., Rojas-Valverde, D., Gómez-Carmona, C. D., de la Cruz Sánchez, E., & Pino-Ortega, J. (2020). Influence of contextual variables in the changes of direction and centripetal force generated during an elite-level soccer team season. International Journal of Environmental Research and Public Health, 17(3), 967. https://doi.org/10.3390/ijerph17030967
- Granero-Gil, P., Gómez-Carmona, C. D., Bastida-Castillo, A., Rojas-Valverde, D., de la Cruz, E., Pino-Ortega, J., & Núñez, F. J. (2020). Influence of playing position and laterality in centripetal force and changes of direction in elite soccer players. PLoS One, 15(4), e0232123. https://doi.org/10.1371/journal.pone.0232123
- Hader, K., Palazzi, D., & Buchheit, M. (2015). Change of direction speed in soccer: How much braking is enough? Kineziologija, 47 (1) , 67–74 https://hrcak.srce.hr/140253.
- Harper, D. J., Carling, C., & Kiely, J. (2019). High-intensity acceleration and deceleration demands in elite team sports competitive match play: A systematic review and meta-analysis of observational studies. Sports Medicine 49(12) , 1923–1947. https://doi.org/10.1007/s40279-019-01170-1
- Harper, D. J., Clubb, J., Bills, B., Young, M., Stars, C. R., Taberner, M., Carling, C., & Kiely, J. (2021). Elite football of 2030 will not be the same as that of 2020: What has evolved and what needs to evolve? Scandinavian Journal of Medicine & Science in Sports, 31(2), 493–494. https://doi.org/10.1111/sms.13876
- Harper, D. J., & Kiely, J. (2018). Damaging nature of decelerations: Do we adequately prepare players? BMJ Open Sport & Exercise Medicine, 4(1), e000379. https://doi.org/10.1136/bmjsem-2018-000379
- Hopkins, W. G. (2002). A scale of magnitudes for effect statistics. A new view of statistics. http://sportsci.org/resource/stats/effectmag.html
- The jamovi project (2021). jamovi (Version 1.6) [Computer Software]. https://www.jamovi.org
- Kai, T., Hirai, S., Anbe, Y., Takai, Y., & Clemente, F. M. (2021). A new approach to quantify angles and time of changes-of-direction during soccer matches. PloS One, 16(5), e0251292. https://doi.org/10.1371/journal.pone.0251292
- Kalkhoven, J. T., Watsford, M. L., Coutts, A. J., Edwards, W. B., & Impellizzeri, F. M. (2021). Training load and injury: Causal pathways and future directions. Sports Medicine, 51(6) , 1137–1150. https://doi.org/10.1007/s40279-020-01413-6
- Krosshaug, T., Nakamae, A., Boden, B., Engebretsen, L., Smith, G., Slauterbeck, J., Hewett, T. E., & Bahr, R. (2007). Estimating 3D joint kinematics from video sequences of running and cutting maneuvers—assessing the accuracy of simple visual inspection. Gait & Posture, 26(3), 378–385. https://doi.org/10.1016/j.gaitpost.2006.10.010
- Lloyd, D. G., & Buchanan, T. S. (2001). Strategies of muscular support of varus and valgus isometric loads at the human knee. Journal of Biomechanics, 34(10), 1257–1267. https://doi.org/10.1016/S0021-9290(01)00095-1
- Maniar, N., Schache, A. G., Pizzolato, C., & Opar, D. A. (2020). Muscle contributions to tibiofemoral shear forces and valgus and rotational joint moments during single leg drop landing. Scandinavian Journal of Medicine & Science in Sports, 30(9), 1664–1674. https://doi.org/10.1111/sms.13711
- Martínez Hernández, D., Quinn, M., & Jones, P. (2022). Linear advancing actions followed by deceleration and turn are the most common movements preceding goals in male professional soccer. Science and Medicine in Football, 1–9. Published ahead of print, 2022. https://doi.org/10.1080/24733938.2022.2030064
- McBurnie, A. J., Harper, D. J., Jones, P. A., & Dos’Santos, T. (2021). Deceleration Training in Team Sports: Another Potential ‘Vaccine’for Sports-Related Injury? Sports Medicine (Auckland, N.Z.), 52(1), 1–12. https://doi.org/10.1007/s40279-021-01583-x
- Morgan, O. J., Drust, B., Ade, J. D., & Robinson, M. A. (2021). Change of direction frequency off the ball: New perspectives in elite youth soccer. Science and Medicine in Football:, 1–10. https://doi.org/10.1080/24733938.2021.1986635 Published ahead of print, 2021
- Nassis, G. P., Massey, A., Jacobsen, P., Brito, J., Randers, M. B., Castagna, C., Mohr, M., & Krustrup, P. (2020). Elite football of 2030 will not be the same as that of 2020: Preparing players, coaches, and support staff for the evolution Scandinavian Journal of Medicine & Science in Sports 30(6) 962–964. https://doi.org/10.1111/sms.13681
- Nedelec, M., McCall, A., Carling, C., Legall, F., Berthoin, S., & Dupont, G. (2014). The influence of soccer playing actions on the recovery kinetics after a soccer match. Journal of Strength and Conditioning Research, 28(6), 1517–1523. https://doi.org/10.1519/JSC.0000000000000293
- Newans, T., Bellinger, P., Dodd, K., & Minahan, C. (2019). Modelling the acceleration and deceleration profile of elite-level soccer players. International Journal of Sports Medicine, 40(5), 331–335. https://doi.org/10.1055/a-0853-7676
- Novak, A. R., Impellizzeri, F. M., Trivedi, A., Coutts, A. J., & McCall, A. (2021). Analysis of the worst-case scenarios in an elite football team: Towards a better understanding and application. Journal of Sports Sciences, 39(16), 1850–1859. https://doi.org/10.1080/02640414.2021.1902138
- Robinson, G., O’Donoghue, P., & Nielson, P. (2011). Path changes and injury risk in English FA Premier League soccer. International Journal of Performance Analysis in Sport, 11(1) , 40–56. https://doi.org/10.1080/24748668.2011.11868528
- Serner, A., Mosler, A. B., Tol, J. L., Bahr, R., & Weir, A. (2019). Mechanisms of acute adductor longus injuries in male football players: A systematic visual video analysis. British Journal of Sports Medicine, 53(3), 158–164. https://doi.org/10.1136/bjsports-2018-099246
- Taberner, M., Allen, T., & Cohen, D. D. (2019). Progressing rehabilitation after injury: Consider the ‘control-chaos continuum’. BMJ Publishing Group Ltd and British Association of Sport and Exercise Medicine 53(18), 1132–1136. http://dx.doi.org/10.1136/bjsports-2018-100157
- Williams, K., Olsen, M. J., Roe, G. V., & Glennie, C. (2013). Synthesis of transportation applications of mobile LiDAR. Remote Sensing, 5(9), 4652–4692. https://doi.org/10.3390/rs5094652