ABSTRACT
This study sought to detail and compare the in-ride nutritional practices of a group of professional cyclists with type 1 diabetes (T1D) under training and racing conditions. We observed seven male professional road cyclists with T1D (Age: 28 ± 4 years, HbA1c: 6.4 ± 0.4% [46 ± 4 mmol.mol−1], VO2max: 73.9 ± 4.3 ml.kg −1.min−1) during pre-season training and during a Union Cycliste Internationale multi-stage road cycling race (Tour of Slovenia). In-ride nutritional, interstitial glucose, and performance variables were quantified and compared between the two events. The in-ride energy intake was similar between training and racing conditions (p = 0.909), with carbohydrates being the major source of fuel in both events during exercise at a rate of 41.9 ± 6.8 g.h−1 and 45.4 ± 15.5 g.h−1 (p = 0.548), respectively. Protein consumption was higher during training (2.6 ± 0.6 g.h−1) than race rides (1.9 ± 0.9 g.h−1; p = 0.051). A similar amount of time was spent within the euglycaemic range (≥70-≤180 mg.dL−1): training 77.1 ± 32.8% vs racing 73.4 ± 3.9%; p = 0.818. These data provide new information on the in-ride nutritional intake in professional cyclists with T1D during different stages of the competitive season.
Introduction
The enormous physical demands of professional road cycling require a carefully planned feeding strategy before, during, and after exercise. Failure to achieve sufficient carbohydrate intake while riding can result in endogenous hepatic and skeletal muscle glycogen depletion as well as a reduction in blood glucose concentrations, with impact on performance (Ørtenblad et al., Citation2013). In order to maintain high carbohydrate oxidation rates and attenuate liver glycogen breakdown during prolonged (>1 hour) endurance exercise, exogenous carbohydrates orally ingested at rates of at least 30–60 g.h−1 up to 90 g.h−1 during exercise are recommended by various international organisations and expert consensus documents (ACSM, Citation2016; Jeukendrup, Citation2014; Stellingwerff & Cox, Citation2014).
The typical pancreatic response to reduce insulin secretion during prolonged exercise is compromised in people with type 1 diabetes (T1D); hence, blood insulin concentrations are instead reflective of the dosing and analogue type of exogenous insulin previously injected. For those with T1D, the effects of relative hyperinsulinaemia and exercise-enhanced muscle cell glucose uptake can combine to exceed the rate at which glucose can be endogenously produced, potentiating hypoglycaemia (McCarthy, Deere et al., Citation2020), with associated consequences on energy metabolism (McCarthy, Pitt et al., Citation2020) and cycling performance (Hill et al., Citation2017). To avoid hypoglycaemia, in addition to maintaining performance, exogenous fuelling is required. Conversely, athletes with T1D who consume such high amounts of carbohydrates to fuel performance in competitive events must also consider the risk of hyperglycaemia.
To coincide optimal training status with the competitive season, different in- and out-of-ride nutritional strategies can be utilised. In athletes without T1D, strategies that focus on restricting nutritional intake such as: training when fasted before and during exercise; “train low, compete high”; and “sleep low, train low”, are prevalent to elicit maximal metabolic adaptations from training and maintain appropriate body weight (Impey et al., Citation2018). By contrast, the aforementioned guidelines recommend high quantities of in-ride carbohydrate consumption during prolonged exercise (ACSM, Citation2016; Jeukendrup, Citation2014; Stellingwerff & Cox, Citation2014). The applicability of these nutritional strategies in people with T1D has been recently reviewed, highlighting the potential risk that drastically changing carbohydrate intake throughout the season may have on effectively managing blood glucose and avoiding hypoglycaemia (Scott et al., Citation2019). Ultimately, there is limited understanding of the nutritional requirements for prolonged exercise in people with T1D. The current evidence base is lacking in samples of professional athletes, a cohort who have higher demands in terms of performance and nutrition during training and races (Gawrecki et al., Citation2018; Mattsson et al., Citation2019; Murillo et al., Citation2010; Sane et al., Citation1988; Scott et al., Citation2020; Van Dijk et al., Citation2016).
Collapsing the results of multiple studies, carbohydrate consumption in people with T1D performing long distance endurance events has been shown to average 50 ± 18 g.h−1 (Riddell et al., Citation2020). This rate of consumption is somewhat lower than the considered maximum muscle carbohydrate oxidation rate achievable via absorption of single transportable carbohydrates (i.e. 60 g.h−1) (Jeukendrup & Jentjens, Citation2000) and deviates below the upper recommendations for carbohydrate consumption for healthy individuals (90 g.h−1) achieved via multiple transporter carbohydrates (Jeukendrup, Citation2014).
Though we have recently profiled the glycaemic and insulin therapy demands of professional road cyclists during pre-season training (McCarthy, Eckstein et al., Citation2020) and competitive stage racing (Moser, Dietrich et al., Citation2020), there remains a dearth in information that characterises the in-ride nutrition of athletes with T1D during training compared to racing in the competitive season. Hence, the aim of this study was to detail and compare the in-ride nutritional practices of a group of professional cyclists with T1D under non-competitive pre-season training and competitive racing conditions.
Materials and methods
Study design
This was a secondary, cross-over, pooled analysis of data collected from two observational, prospective studies (DRKS.de: DRKS00019923, DRKS00019928). The studies were carried out in accordance with the Declaration of Helsinki and International Conference on Harmonisation Good Clinical Practice. Ethical approval was granted by Swansea University research ethics committee (Ethics Committee Reference number: 2018–140). All participants provided written informed consent prior to any study-related activities.
Participant characteristics
Seven male athletes with T1D from one professional cycling team who undertook nine days of pre-season training as part of a performance camp (January 2019, Altea, Spain) and competed in a 5-day competitive stagerace (June 2019, Tour of Slovenia, Slovenia 2.HC level race [stage race in the second tier of competitive road cycling]) volunteered to participate in this research study. During the training camp, cyclists performed a cardio-pulmonary exercise test (CPET) to volitional exhaustion on their bicycle (Colnago, C60) attached to a cycle trainer (Cyclus 2, RBM elektronik-automation GmbH, Germany) as previously described (McCarthy, Eckstein et al., Citation2020). The anthropometric and diabetes characteristics alongside the CPET results of participants are presented in . All riders were using multiple daily injections of insulin therapy via pen administration consisting of a range of bolus (bold) and basal insulins (Training: n = 6 FiaspTM, n = 1 NovorapidTM, n = 4 LantusTM, n = 2 LevemirTM, n = 1 ToujeoTM; Race: n = 7 FiaspTM, n = 4 LantusTM, n = 2 LevemirTM, n = 1 ToujeoTM).
Table 1. Anthropometric, diabetes and maximum cardiopulmonary exercise test performance characteristics of study participants (n = 7).
Cycle training regime
Between the 18th and 27th of January 2019, riders attended a pre-season training camp in Altea, Spain. During the camp, riders performed eight training rides over nine days on roads through mostly mountainous terrain with one day dedicated to CPET. Riders covered approximately 1290 km over the eight days of road training (average temperature 12.4°C). During training camp, riders performed all sessions on an individual time-trial basis with individualised training zones prescribed by the exercise physiology team.
Cycle racing regime
Later in the racing season, the same seven riders competed in the Tour of Slovenia - a 5-day Union Cycliste Internationale (UCI) road-cycle race held between the 19th and 23rd of June 2019. The Tour of Slovenia included 18 teams, consisting of five UCI World Tour teams, eight UCI professional Continental teams, five UCI Continental teams, and one national team. Riders covered approximately 823 km over the five consecutive days of road racing (average temperature 26.2°C).
Profiling of performance and physiological responses during exercise
Athletes rode with a mobile power-meter (Pioneer, USA) and a cycle computer (Wahoo, Wahoo fitness, USA) mounted on their bike that monitored physiological and performance metrics during exercise (i.e., power, cadence, velocity, distance and duration). Heart rate (HR) was measured via a portable monitor strapped onto the chest (Wahoo, Wahoo Fitness, USA). Cycle metrics were logged and retrospectively uploaded via a computerised cloud-based package (Training PeaksTM, USA) that facilitated data analysis.
Continuous glucose monitoring
In each period of data collection, riders were provided with unblinded real-time continuous glucose monitors ([rtCGM] G6, Dexcom Inc., San Diego, California). The subcutaneous sensor was worn on the posterior upper arm or on the hip, depending on rider preference. On the final day of both the training and racing events, each rider’s rtCGM data were downloaded from the online Dexcom Clarity™ platform.
Nutritional tracking and macronutrient reporting
During exercise, dietary intake was recorded via the provision of individualised rider feed bags containing a selection of items routinely used by the team throughout the cycling calendar. Before each cycling session, riders’ pre-race food was quantified. Food and drinks handed from the team cars during the race were recorded as well as food given to each rider at the feed zone(s). Food items in the riders’ pockets not consumed at the end of each race was quantified and subtracted from that given out. These data were verified by recall by the athletes following each stage. When there was uncertainty, for example, where one athlete collected numerous items from the team car to distribute to their teammates, food items were split evenly between riders. Nutrition content for each product was quantified using the Nutrition Facts labels. Individual drinks and gels were classified as liquid snacks while all other individual foods were classed as solid snacks (plain water was excluded from this analysis).
Data analysis
All descriptive and statistical analyses were carried out using SPSS 26.0 statistical software (SPSS, Chicago, Illinois, USA). Alpha was set at 0.05, and statistical significance was accepted when p ≤ alpha. Differences between training versus racing data were assessed via paired sample t-tests. Single stepwise linear regression was used to explore relationships between in-ride nutritional intake, glycaemia, and exercise performance variables.
rtCGM data as percentage time spent were stratified into pre-defined glycaemic ranges in accordance with a recent international consensus statement (Battelino et al., Citation2019): level 2 hypoglycaemia (<54 mg.dL−1 [<3.0 mmol.L−1]), level 1 hypoglycaemia (≥54 – ≤69 mg.dL−1 [≥3.0 – ≤3.8 mmol.L−1]), euglycaemia (≥70 – ≤180 mg.dL−1 [≥3.9 – ≤10.0 mmol.L−1]), level 1 hyperglycaemia (≥181 – ≤250 mg.dL−1 [≥10.1 – ≤13.9 mmol.L−1]) and level 2 hyperglycaemia (>250 mg.dL−1 [>13.9 mmol.L−1]).
Results
Performance metrics and heart rate responses to exercise
The means of riders’ performance variables of exercise are presented in . Despite equivalent exercise durations, power outputs, and cycling cadences, riders traversed further distances at quicker speeds with higher HR responses under racing conditions than during the training sessions ().
Table 2. The performance variables of exercise under pre-season training and racing conditions.
In-ride energy intake and macronutrient contributions
Carbohydrate intake was similar during exercise under both training and racing conditions (). Irrespective of the exercising event, carbohydrate contributed most to the total energy intake during cycling (>80% in both conditions).
Table 3. In-ride energy and macronutrient intake of professional road cyclists with type 1 diabetes under pre-season training and competitive road cycle racing conditions (n = 7).
Protein intake during exercise was higher during training in absolute amounts (p = 0.046) and when expressed as a % contribution to the total energy intake, yet fell outside significance when intake was relativised to time (). There was no difference in fat intake between events. Linear regression revealed that total in-ride carbohydrate intake was considered a potential predictor of power output during exercise (β = 0.411, p < 0.001) with an R2 of 0.169.
Rate and form of fuel intake during cycling
Riders consumed an average of 9 ± 3 vs 8 ± 1 (p = 0.310) snacks per ride, equating to 2 ± 1 vs 2 ± 0 (p = 0.088) snacks per hour, in racing and training, respectively. A preference to consume snacks classed as solid vs snacks classed as fluid was shown during training (p < 0.001), while there was no preference in snack type usage during race conditions (p = 0.282). details the percentage contribution of number of solid and liquid foods to the total number of in-ride sacks both in racing and training conditions.
Figure 1. Number of snacks consumed per ride and relative contribution of Fluid and Solid snacks. Percentage contributions of snack type usage between the two event types were statistically significant (p < 0.001). Data expressed as averages of riders in each event with SEM bars.
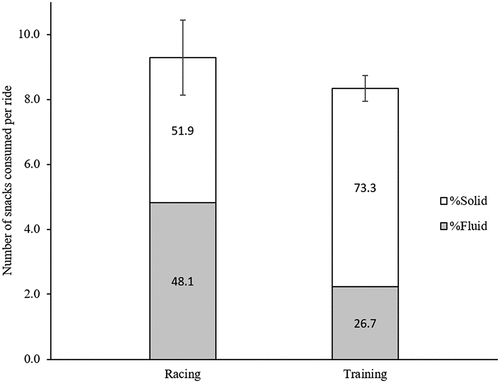
Fluid snacks had no protein or fat content and fewer calories on average compared to solid snacks in both racing (Fluid 71.5 ± 8.9 vs Solid 134.6 ± 12.0 kcals, p < 0.001) and training (Fluid 65.9 ± 22.7 vs Solid 133.0 ± 10.26 kcals, p < 0.001) events. Riders favoured a carbohydrate-rich branded sports drink and a branded sports gel during the race, whereas a sports bar and the same sports drink were the most common snacks consumed during training. Other popular snacks included fruit and biscuits. Out of the top five preferred snacks in training, three of the same snacks were also used during the race (9 out of a combined total of 15 different snacks were the same in both events).
In-ride glycaemia
outlines the interstitial glucose (iG) responses to exercise under training and racing conditions; there were no differences between glycaemic responses in the two events.
Table 4. Glycaemic responses to exercise under training and racing conditions.
Discussion
This study sought to characterise the in-ride nutritional practices of professional cyclists with T1D and compare these data under pre-season training and competitive racing conditions. Cycling load was similar in terms of volume and intensity parameters between training and competition rides; however, increased cycling speed and higher heart rates reflect the greater exertion performed during race events. The findings reveal a key role for carbohydrates in supporting cycling performance with in-ride energy intake similar between racing and training environments. Riders consumed ~43 g carbohydrates per hour of cycling (~0.7 g.kg−1.h−1) and the amount of carbohydrates was a potential predictor variable for cycling power. Despite differences in exercise intensity, riders spent a comparable amount of time within the euglycaemic range in training and racing.
Energy intake and composition
We found a similar energy intake during exercise between training and racing conditions, with a heavy emphasis (>80% of total energy intake during exercise in both events) on carbohydrates as the predominant source of fuel. To align with guideline recommendations and maximise performance, it is intuitive that both training and race conditions would reflect high carbohydrate consumption to match the high workload demands in both settings. However, intensity of training fluctuates according to season stage and training periodisation (Stellingwerff et al., Citation2007); hence, the dietary intake observed during the high-volume training camp is more likely to match the high volume and intensity of a stage race than other periods of the athlete training schedule (e.g., general training, rest and recovery; Scott et al., Citation2019). Indeed, cross-sectional data from multiple studies suggest a wide range of daily carbohydrate consumption in athletes with type 1 diabetes, yet reflect only acute dietary strategies (Riddell et al., Citation2020).
We also observed that in-ride carbohydrate intake was considered a potential, albeit weak, predictor of power output during exercise. As muscle glycogen stores deplete during prolonged exercise, blood glucose becomes the major source of carbohydrates for working skeletal muscle tissue (Coggan & Coyle, Citation1991; Coyle et al., Citation1986). Hence, as endogenous glucose production via hepatic gluconeogenesis is rate-limited, the ingestion of exogenous carbohydrate during the later stages of exercise is the predominant means of ensuring blood glucose availability and oxidation. With this in mind, the ergogenic potential of carbohydrate supplementation during prolonged exercise in postponing fatigue and enhancing muscular performance is well established (Coggan & Coyle, Citation1991, Citation1987; Coyle et al., Citation1986; Temesi et al., Citation2011) and recognised by its inclusion in several sports nutrition consensus guidelines (ACSM, Citation2016; Thomas et al., Citation2016). It should be noted that in athletes with (and without) T1D carbohydrate consumption during exercise may not be consistent throughout the training season as different nutritional strategies can help elicit different training adaptations (Scott et al., Citation2019).
Adherence to in-ride carbohydrate consumption guidelines
International position statement guidelines advocate the intake of 30–90 g.h−1 of exercise to enhance or maintain performance during endurance exercise lasting longer than 60 minutes (ACSM, Citation2016). While this applies to people without T1D, it is apparent from our data that the riders with T1D met these recommendations, consuming 41.9 g.h−1 of carbohydrates during training rides and 45.4 g.h−1 of carbohydrates during competition. Furthermore, recent research detailing the nutritional requirements of elite athletes with T1D advocates consuming 0.4–1.3 g.kg−1.h−1 to prevent hypoglycaemia and enhance performance (Riddell et al., Citation2020). The riders from the current study consumed approximately 0.6 g.kg−1.h−1, falling within the guidance specific to those with T1D. While there is scope for the mechanism of the ergogenic effect of carbohydrate supplementation to differ between exercise modalities (Tsintzas & Williams, Citation1998), previous literature has described similar ingestion rates in endurance running, skiing, and walking (Riddell et al., Citation2020).
It is clear that, in these cyclists, nutritional intake must be carefully balanced between two major factors 1) the need to support optimal performance and 2) the management of glycaemic excursions. Hence, beyond the ergogenic potential of carbohydrate in aiding sports performance, carbohydrate prioritisation during exercise in the present study may reflect a glycaemic management strategy used by riders to support glucose both within and beyond the exercising event. Indeed, carbohydrate ingestion during exercise can evoke a sparing effect on hepatic glucose production, thereby reducing the extent of liver glycogen depletion. We have previously shown higher rates of nocturnal hypoglycaemia in professional cyclists with T1D compared to day-time hypoglycaemia, the consequence of which was an increased chance of experiencing a further hypoglycaemic event during the following day’s ride (McCarthy, Eckstein et al., Citation2020). Given the direct links between blood glucose and muscular performance, as well as the clinical repercussions of hypoglycaemia (McCarthy, Pitt et al., Citation2020), nutritional strategies that help mitigate risk are critical in those with T1D.
Selection of in-ride fuelling
There was a greater consumption of fluid versus solid types of carbohydrate snacks during race conditions than during training sessions. Previous research has suggested that using fluid-based snacks is an efficacious strategy in endurance events in maintaining hydration status during the ride whilst being convenient to consume macronutrients (Von Duvillard et al., Citation2004). Individual preference of avoiding solid foods which contained more fat, protein, and fibre, may also be made in the effort to avoid gastrointestinal issues (De Oliveira et al., Citation2014); however, the preference for gels and drinks in our observations may be due to the higher ambient temperature under racing conditions in these observations. Despite an overall preference towards fluid drinks during the race, riders exhibited continuity in their fuelling preferences from training in terms of the number and amount (carbohydrate mass) of carbohydrate snacks consumed. Future research that determines the exact timing of food consumption during rides, alongside insulin and rtCGM data, would enhance our understanding of exogenous fuelling for (prolonged) exercise. This information, in addition to the type and quantity of food we have described, could help clarify the allocation of nutritional intake in the context of the anticipation of declining blood glucose; reactions to feelings of fatigue, hypoglycaemia, or hunger; or the implementation of a nutrition strategy for performance.
Time in range
Riders spent a commendable amount of time in the range during exercise (>70% in both groups), with euglycaemic mean interstitial glucose concentrations observed in both conditions. These values fall well within the clinical recommendations for the amount of time spent with sensor glucose in target range (i.e., >70% of daily values within 70–180 mg.dL−1 [3.9–10.0 mmol.L−1]; Battelino et al., Citation2019) as well as exercise-specific position statements advocating the maintenance of sensor glucose between 90 and 180 mg.dL−1 (5.0–10.0 mmol.L−1) during exercise in people with T1D (Moser, Riddell et al., Citation2020). That riders in the present study achieved these targets during successive days’ worth of ~4.5-hour daily rides at intensities of >70% HRmax is particularly admirable given the difficulties of maintaining normoglycaemia and avoiding hypoglycaemia during prolonged intense exercise even in those without T1D (Hill et al., Citation2017).
We observed one rider who spent considerably more time in hyperglycaemic ranges during training compared to other riders. Under race conditions, the same rider averaged high-normoglycaemic concentrations (166 mg.dL−1) and spent a comparable time in range to others in the group. Despite being close to the hyperglycaemic threshold (180 mg.dL−1) in both conditions, the tendency to spend time above this threshold in hyperglycaemia during training may explain the greater standard deviations in the training group rtCGM metrics. This example highlights the importance of individual monitoring in the racing group or self-reflection for solo athletes to identify methods for improved in-ride glucose management.
Unsurprisingly, loss of glycaemia during exercise constitutes the leading barrier dissuading regular participation for people with T1D (Brazeau et al., Citation2008). In these data, the management of glycaemia during prolonged exercise in T1D is achievable without detriment to acute glycaemic control and may offer encouragement to the wider T1D community when looking to undertake regular physical activity.
Strengths and limitations
The strength of this work is the provision of data that lends insight into the in-ride nutrition of a unique cohort of elite professional cyclists with type 1 diabetes during two differing periods of the racing calendar and their comparison. Marrying nutrition information with rtCGM data provides additional relevance to glucose management during exercise performance; however, further research would benefit from identifying the timing of in-ride nutrition. The small sample size of the study is similar to that of other studies in elite athletes in this area, yet we acknowledge this limits the power of statistical comparison.
Conclusion
We have characterised the in-ride nutritional fuelling of athletes with T1D athletes and shown that carbohydrate intake in training and competition to be similar, albeit below the upper limits of consumption recommended in healthy individuals. Despite performing prolonged periods of intense activity, riders were able to average euglycaemic glucose concentrations and achieve TIR percentages that meet clinical recommendations. These data provide novel insight into the in-ride nutritional strategies employed by professional cyclists with T1D who successfully manage their glycaemia during prolonged intense exercise events at different stages of the racing calendar, but also show a great individual variability in glycaemic and performance profiles even though the group was small and apparently similar.
Acknowledgments
The authors would like to thank the participants for their willingness to assist in data sharing and collection. We would also like to thank all staff members for their invaluable contributions to data collection at the Team Novo Nordisk rides.
Disclosure statement
SNS, FYF, and KS have been under employment by Team Novo Nordisk Professional Cycling Team.
Additional information
Funding
References
- ACSM. (2016). Nutrition and athletic performance. Medicine & Science in Sports & Exercise, 48(3), 543–568. https://doi.org/10.1249/MSS.0000000000000852
- Battelino, T., Danne, T., Bergenstal, R. M., Amiel, S. A., Beck, R., Biester, T., Bosi, E., Buckingham, B. A., Cefalu, W. T., Close, K. L., Cobelli, C., Dassau, E., DeVries, J. H., Donaghue, K. C., Dovc, K., Doyle III, F. J., Garg, S., Grunberger, G., Heller, S., Heinemann, L., Hirsch, I. B. et al . (2019). Clinical targets for continuous glucose monitoring data interpretation : Recommendations from the international consensus on time in range. Diabetes Care 42 8 :1593–1601. https://doi.org/10.2337/dci19-0028
- Brazeau, A.-S., Rabasa-Lhoret, R., Strychar, I., & Mircescu, H. (2008). Barriers to physical activity among patients with type 1 diabetes. Diabetes Care, 31(11), 2108–2109. https://doi.org/10.2337/dc08-0720
- Coggan, A. R., & Coyle, E. F. (1987). Reversal of fatigue during prolonged exercise by carbohydrate infusion or ingestion. Journal of Applied Physiology, 63(6), 2388–2395. https://doi.org/10.1152/jappl.1987.63.6.2388
- Coggan, A. R., & Coyle, E. F. (1991). Carbohydrate ingestion during prolonged exercise: Effects on metabolism and performance. Exercise and Sport Sciences Reviews, 19(1), 1–40. https://doi.org/10.1249/00003677-199101000-00001
- Coyle, E. F., Coggan, A. R., Hemmert, M. K., & Ivy, J. L. (1986). Muscle glycogen utilization during prolonged strenous exercise when fed carbohydrate. Journal of Applied Physiology, 61(1), 165–172. https://doi.org/10.1152/jappl.1986.61.1.165
- De Oliveira, E. P., Burini, R. C., & Jeukendrup, A. (2014). Gastrointestinal complaints during exercise: Prevalence, etiology, and nutritional recommendations. Sports Medicine, 44(SUPPL.1), 79–85. https://doi.org/10.1007/s40279-014-0153-2
- Gawrecki, A., Zozulinska-Ziolkiewicz, D., Matejko, B., Hohendorff, J., Malecki, M., & Klupa, T. (2018). Safe completion of a trail running ultramarathon by four men with type 1 diabetes. Diabetes Technology & Therapeutics, 20(2), 147–152. https://doi.org/10.1089/dia.2017.0296
- Hill, N. E., Campbell, C., Buchanan, P., Knight, M., Godsland, I. F., & Oliver, N. S. (2017). Biochemical, physiological and psychological changes during endurance exercise in people with type 1 Diabetes. Journal of Diabetes Science and Technology, 11(3), 529–536. https://doi.org/10.1177/1932296816671956
- Impey, S. G., Hearris, M. A., Hammond, K. M., Bartlett, J. D., Louis, J., Close, G. L., & Morton, J. P. (2018). Fuel for the work required: A theoretical framework for carbohydrate periodization and the glycogen threshold hypothesis. Sports Medicine, 48(5), 1031–1048. https://doi.org/10.1007/s40279-018-0867-7
- Jeukendrup, A. (2014). A step towards personalized sports nutrition: Carbohydrate intake during exercise. Sports Medicine, 44(SUPPL.1), S25–S33. https://doi.org/10.1007/s40279-014-0148-z
- Jeukendrup, A. E., & Jentjens, R. (2000). Oxidation of carbohydrate feedings during prolonged exercise. Sports Medicine, 29(6), 407–424. https://doi.org/10.2165/00007256-200029060-00004
- Mattsson, S., Jendle, J., & Adolfsson, P. (2019). Carbohydrate loading followed by high carbohydrate intake during prolonged physical exercise and its impact on glucose control in individuals with diabetes type 1—an exploratory study. Frontiers in Endocrinology, 10(August), 1–8. https://doi.org/10.3389/fendo.2019.00571
- McCarthy, O., Deere, R., Churm, R., Dunseath, G. J., Jones, C., Eckstein, M. L., Williams, D. M., Hayes, J., Pitt, J., Bain., S. C., Moser, O., Bracken, R. M., et al. (2020). Extent and prevalence of post-exercise and nocturnal hypoglycemia following peri-exercise bolus insulin adjustments in individuals with type 1 diabetes. Nutrition, Metabolism, and Cardiovascular Diseases, 31 1 , 227–236. https://doi.org/10.1016/j.numecd.2020.07.043
- McCarthy, O., Eckstein, M. L., Scott, S. N., Fontana, F. Y., Christiansen, M. P., Stettler, C., Fisher, M., Bode, B., Riddell, M. C., Hayes, C., Lagrou, P. L., Southerland, P., Moser, O., Bracken, R. M., et al (2020). Glycemic responses to strenuous training in male professional cyclists with type 1 diabetes: A prospective observational study. BMJ Open Diabetes Research & Care, 8(1), 1–9. https://doi.org/10.1136/bmjdrc-2020-001245
- McCarthy, O., Pitt, J., Churm, R., Dunseath, G. J., Jones, C., Bally, L., Nakas, C. T., Deere, R., Eckstein, M. L., Bain, S. C., Moser, O., & Bracken, R. M. (2020). Metabolomic, hormonal and physiological responses to hypoglycemia versus euglycemia during exercise in adults with type 1 diabetes. BMJ Open Diabetes Research & Care, 8(1), 1–10. https://doi.org/10.1136/bmjdrc-2020-001577
- Moser, O., Dietrich, M., McCarthy, O., Bracken, R. M., & Eckstein, M. L. (2020). Bolus insulin dose depends on previous-day race intensity during 5 days of professional road-cycle racing in athletes with type 1 diabetes: A prospective observational study. Diabetes, Obesity and Metabolism, 22(10), 1714–1721. https://doi.org/10.1111/dom.14083
- Moser, O., Riddell, M. C., Eckstein, M. L., Adolfsson, P., Rabasa-Lhoret, R., van den Boom, L., Gillard, P., Nørgaard, K., Oliver, N. S., Zaharieva, D. P., Battelino, Tadej, de Beaufort, C., Bergenstal, R. M., Buckingham, B., Cengiz, E., Deeb, A., Heise, T., Heller, S., Kowalski, A. J., Leelarathna, L., Mathieu, C. et al . (2020). Glucose management for exercise using continuous glucose monitoring (CGM) and intermittently scanned CGM (isCGM) systems in type 1 diabetes: Position statement of the European Association for the Study of Diabetes (EASD) and of the International Society f. Diabetologia 63 12 :2501–2520. https://doi.org/10.1111/pedi.13105
- Murillo, S., Brugnara, L., & Novials, A. (2010). One year follow-up in a group of half-marathon runners with type-1 diabetes treated with insulin analogues. The Journal of Sports Medicine and Physical Fitness, 50(4), 506–510. PMID: 21178938.
- Ørtenblad, N., Westerblad, H., & Nielsen, J. (2013). Muscle glycogen stores and fatigue. The Journal of Physiology, 591(18), 4405–4413. https://doi.org/10.1113/jphysiol.2013.251629
- Riddell, M. C., Scott, S. N., Fournier, P. A., Colberg, S. R., Gallen, I. W., Moser, O., Stettler, C., Yardley, J. E., Zaharieva, D. P., Adolfsson, P., & Bracken, R. M. (2020). The competitive athlete with type 1 diabetes. Diabetologia, 63(8), 1475–1490. https://doi.org/10.1007/s00125-020-05183-8
- Sane, T., Helve, E., Pelkonen, I., & Koivisto, V. A. (1988). The adjustment of diet and insulin dose during long-term endurance exercise in Type 1 (insulin-dependent) diabetic men. Diabetologia, 31(1), 35–40. https://doi.org/10.1007/BF00279130
- Scott, S. N., Anderson, L., Morton, J. P., Wagenmakers, A. J. M., & Riddell, M. C. (2019). Carbohydrate restriction in type 1 diabetes: A realistic therapy for improved glycaemic control and athletic performance? Nutrients, 11(5), 1–28. https://doi.org/10.3390/nu11051022
- Scott, S. N., Christiansen, M. P., Fontana, F. Y., Stettler, C., Bracken, R. M., Hayes, C. A., Fisher, M., Bode, B., Lagrou, P. H., Southerland, P., & Riddell, M. C. (2020). Evaluation of factors related to glycemic management in professional cyclists with type 1 diabetes over a 7-day stage race. Diabetes Care, 43(5), 1142–1145. https://doi.org/10.2337/dc19-2302
- Stellingwerff, T., Boit, M. K., & Res, P. T. (2007). Nutritional strategies to optimize training and racing in middle-distance athletes. Journal of Sports Sciences, 25 S1 , S17–S28. https://doi.org/10.1080/02640410701607213
- Stellingwerff, T., & Cox, G. R. (2014). Systematic review : Carbohydrate supplementation on exercise performance or capacity of varying durations 1. Applied Physiology, Nutrition, and Metabolism, 39(9), 998–1011. https://doi.org/10.1139/apnm-2014-0027
- Temesi, J., Johnson, N. A., Raymond, J., Burdon, C. A., & O’Connor, H. T. (2011). Carbohydrate ingestion during endurance exercise improves performance in adults. The Journal of Nutrition, 141(5), 890–897. https://doi.org/10.3945/jn.110.137075
- Thomas, D. T., Erdman, K. A., & Burke, L. M. (2016). Position of the academy of nutrition and dietetics, Dietitians of Canada, and the American College of Sports Medicine: Nutrition and athletic performance. Journal of the Academy of Nutrition and Dietetics, 116(3), 501–528. https://doi.org/10.1016/j.jand.2015.12.006
- Tsintzas, K., & Williams, C. (1998). Human muscle glycogen metabolism during exercise. Sports Medicine, 25(1), 7–23. https://doi.org/10.2165/00007256-199825010-00002
- van Dijk, J. W., Eijsvogels, T. M., Nyakayiru, J., Schreuder, T. H. A., Hopman, M. T., Thijssen, D. H., & van Loon, L. J. C. (2016). Glycemic control during consecutive days with prolonged walking exercise in individuals with type 1 diabetes mellitus. Diabetes Research and Clinical Practice, 117, 74–81. https://doi.org/10.1016/j.diabres.2016.04.053
- Von Duvillard, S. P., Braun, W. A., Markofski, M., Beneke, R., & Leithäuser, R. (2004). Fluids and hydration in prolonged endurance performance. Nutrition, 20(7–8), 651–656. https://doi.org/10.1016/j.nut.2004.04.011