ABSTRACT
This study investigated the short-term responses of step characteristics in sprinters and team-sports players under different bend conditions. Eight participants from each group completed 80 m sprints in four conditions: banked and flat, in lanes two and four (L2B, L4B, L2F, L4F). Groups showed similar changes in step velocity (SV) across conditions and limbs. However, sprinters produced significantly shorter ground contact times (GCT) than team sports players in L2B and L4B for both left (0.123 s vs 0.145 s and 0.123 s vs 0.140 s) and right steps (0.115 s vs 0.136 s and 0.120 s vs 0.141 s) (p > 0.001–0.029; ES = 1.15–1.37). Across both groups, SV was generally lower in flat conditions compared to banked (Left: 7.21 m/s vs 6.82 m/s and Right: 7.31 m/s vs 7.09 m/s in lane two), occurring due to reduced step length (SL) rather than step frequency (SF), suggesting that banking improves SV via increased SL. Sprinters produced significantly shorter GCT in banked conditions that led to non-significant increases in SF and SV, highlighting the importance of bend sprinting specific conditioning and training environments representative of indoor competition for sprint athletes.
Introduction
Curvilinear running is relevant for many sports and maintaining high running speed around bends with tight radii leads to increases in performance. For example, sprints during official soccer matches are rarely along a linear path (Fitzpatrick et al., Citation2019), with approximately 85% being curvilinear (Caldbeck, Citation2019) and along a curve radius of 3.5–11 m (Brice et al., Citation2004). Within track and field, long sprint athletes (200–400 m) are required to run around bends of varying radii on both outdoor and indoor facilities. Furthermore, the use of banked bends is common in indoor athletic facilities, where tracks are typically 200 m rather than 400 m. The lateral banking enables athletes to maintain higher running speeds compared to flat curves of similar radii (Greene, Citation1987).
It is commonly accepted that performance, kinematic and kinetic variables are affected when sprinting around a bend compared to linear sprinting (Alt et al., Citation2015; Chang & Kram, Citation2007; Churchill et al., Citation2015, Citation2016; Smith et al., Citation2006). Performance and step characteristics have also been shown to be affected by tightening radii (Chang & Kram, Citation2007; Churchill et al., Citation2019). For example, the effect of lane radius between the inside lanes and outer lanes has been proposed to be between 0.123 and 0.23 s over a 200 m race on an outdoor track (Churchill et al., Citation2019; Greene, Citation1985; Quinn, Citation2009). Chang and Kram (Citation2007) observed significant reductions in step length as the bend radii became smaller (1.0 m−6.0 m). In contrast, Churchill et al. (Citation2019) reported that reductions in mean race speed decreased mainly due to reductions in step frequency as the radius of the bend decreased (38.5 m−45.1 m). While these differing results could be attributed to the difference in the bend radii used, Churchill et al. (Citation2019) also reported that the effects of tighter bend radii typical of outdoor competition were not consistent at a group level, with greater variability in performance between participants. This finding indicates that athletes may possess differing abilities for maintaining sprint performance on tighter radii. Ohnuma et al. (Citation2018) grouped well-trained sprinters as “good” and “poor” bend runners based on an ad-hoc division of the difference in velocity between the straight and the bend. Their results showed reductions in sprinting speed, step length, and flight distance when bend sprinting in the “poor” group only. This suggests that even within a well-trained population, the ability to maintain velocity on the bend varies between athletes.
The potential difference in performance between athletes when negotiating tight-bend radii has greater bearing during indoor competition where radii are smaller (Usherwood & Wilson, Citation2006). The increased effect of bend radii on indoor sprint performance led to 200 m indoor events being abandoned by the International Association of Athletics Federations in 2004. The tighter radii of indoor bends may place a greater challenge on the athlete’s coordinative structures through having to generate greater turning forces with each step and therefore have a greater impact on step characteristics and performance outcome. Ryan & Harrison (Citation2003) reported reduced step lengths (SLs) and flight times (FTs) but increased step frequency (SF) and longer ground contact times (GCT) on banked indoor bends (lanes 1 and 4) compared to flat outdoor bends (lanes 1 and 8), suggesting the effect of lateral banking and radius impacts SL and SF differently. Nonetheless, the comparison of banked indoor radii and flat outdoor radii means that the change in lateral banking may have led to differences in step characteristics rather than the difference in lane radius.
To compensate for tighter bend radii, indoor athletic facilities have incorporated lateral banking (Greene, Citation1987). Lateral banking minimises the amount of body lateral lean required during bend sprinting, meaning that the lower limbs are in a better position to generate ground reaction forces. For example, during bend sprints with a radius of 2.5 m, Luo and Stefanyshyn (Citation2012), found that laterally wedged footwear aligned the ankle in a more neutral position (less eversion), enabling greater force production and improving curved sprint speed. Nevertheless, only inside (left) step data is reported. Previous research has highlighted that the inside (left) step is more affected by the bend than the outside (right), with trends for reduced SF as a result of longer GCTs (Alt et al., Citation2015; Churchill et al., Citation2015, Citation2019). Nonetheless, this is direction-specific due to the athletic competition occurring in a counter-clockwise direction.
Other than track and field, athletes are required to run in a curvilinear manner in multiple team sports (Fitzpatrick et al., Citation2019; Gomez-Carmona et al., Citation2021; Nian, Citation2021). Recent findings showed that soccer players sprint marginally faster (2.45 s ± 0.11 s) during a curved sprint in comparison to a linear sprint (2.47 ± 0.13 s) around a 9.5 m radius (Filter et al., Citation2020). The authors also suggested that the inside leg appeared to be more affected than the outside limb (Filter et al., Citation2020). Smith et al. (Citation2006) suggested that overall force production was reduced on a 5 m radius at 5.4 m/s compared to straight running at matched speed and that the reduced inside limb force production accounted for this reduced force (compared to the outside), postulating that the outside limb contributes more to the turning of the centre of mass. However, Churchill et al. (Citation2015) found in lane 2 (37.72 m) of an outdoor track that the inside (left) step contributes 1.6º more turning (defined as the change in angle of consecutive flight displacement vectors on either side of the foot in contact with the ground) than the right step. Nonetheless, despite some anecdotal evidence for a greater impact on the left step during indoor bend sprinting (Bezodis & Gittoes, Citation2008), and for soccer players sprinting curvilinearly (Filter et al., Citation2020), research is yet to investigate the relationship between bend radius, lateral banking, and inter-limb differences in both well-trained sprinters and team sports players.
Therefore, the aim of this study was to examine the short-term responses to bend sprinting in flat and banked bend conditions on step characteristics in well-trained and team sports athletes. The overall purpose was to provide original insights into the mechanical adaptations that both sprint athletes and team sports athletes undergo when exposed to the constraints of different bend conditions to inform training prescription. The identification of the mechanisms for performance reduction with tighter radii and unbanked bends can enable practitioners to focus training to target these areas. It was hypothesised that the effect of the conditions would affect both groups similarly (H1). Secondly, sprint athletes would demonstrate significantly faster step velocities (SVs) through a combination of greater SF and SL than the team sports group (H2). Thirdly, SV would be significantly reduced when sprinting on flat bends in comparison to banked bends (H3), and when on tighter radii compared to less tight radii for both left and right steps (H4). Finally, it was hypothesised that right SV would be greater than left SV across conditions as a result of shorter ground contact times (GCT) (H5).
Materials and methods
Following institutional ethical approval, eight sprinters and eight team sports athletes provided informed consent. Characteristics for all participants including their 200 m personal best (PB) are presented in . Participants were well-trained long sprinters (200–400 m) who had competed on a banked indoor track within the last 6 months or team sports players (rugby, soccer, cricket) who regularly sprinted in those games.
Table 1. Mean ± standard deviations (SD) of participant characteristics: age, height, mass and 200 m personal best (PB).
Experimental setup
Twenty-four optoelectronic cameras (Vicon, Oxford, 250 Hz) were set up to capture two consecutive steps on the bend across lanes two and four (). Participants were prepared with an adapted plug-in gait marker set previously validated for bend sprinting (Judson et al., Citation2020a), with additional technical marker clusters. A 200 m indoor track capable of raising and lowering the bend to be banked or flat and radii of 13.98 m for lane two and 15.94 m for lane four was used for testing. Two sprints were undertaken at 85% of their perceived maximum in both lane two and four when the bend was banked and flat (L2B, L4B, L2F, L4F); thus, participants were asked to undertake eight 80 m sprints. These were undertaken at submaximal velocity and were selected due to the injury risk associated with maximum velocity on flat bends of small radii (Ryan & Harrison, Citation2003). To avoid the effects of fatigue, recovery time was self-selected, but typically lasted 5 to 10 min, similar to previous sprint research (Douglas et al., Citation2020; Exell et al., Citation2017), with testing carried out in a randomised counter-balanced order. Kinematic data were captured at the apex of the curve 50–60 m into the sprint.
Data processing
Kinematic marker trajectories were labelled using Vicon Nexus (v2.9.3) and gap filled when necessary using both rigid body (for gaps in marker cluster trajectories) and pattern functions. Additionally, when no suitable trajectory was appropriate for pattern gap filling, the spline function was used. Rigid-body and pattern gap-filling were used where appropriate with the spline function only utilised for gaps <10 frames (0.04 s). Data were exported for further processing using Visual 3D software (C-Motion Inc, Germantown, USA). Custom MATLAB (MathWorks, USA, 2018a) code was used to determine foot contact events: touchdown was identified using peak vertical accelerations of the toe marker, and toe-off was identified as the first frame after the minimum toe position (Nagahara & Zushi, Citation2013). Step characteristics were defined in line with Churchill et al. (Citation2015).
Statistical analysis
IBM SPSS Statistics (Version 27, SPSS Inc, Chicago Illinois, USA) was used to undertake statistical analyses. A linear mixed effects model including fixed effects of limb, condition and athlete group and a random effect of athlete were utilised (Limb*Condition*Athlete Group) for each variable, and Bonferroni corrections were applied for post-hoc pairwise difference testing. Most sprint research has used averaged scores or selected the fastest trial rather than examining individual trials, so the use of linear mixed effects models, which enable assessment of trial level data, allows a more sensitive approach that more accurately models within-participant variance (Speelman & McGann, Citation2013). Nonetheless, in order to determine pairwise differences, mean data for step characteristics were averaged across the two sprint trials for each participant in each condition. Group descriptive data (mean ± SD) were calculated for all variables and checked for normal distribution using the Shapiro–Wilk statistic (p > 0.05). To identify any differences between limbs within a condition, post-hoc paired-t tests were run, whilst for differences between sprint and team-sport groups, independent t-tests were run. Significance was set at p < 0.05 for interactions and main effects, whilst for post-hoc comparisons 0.025 was used to reflect the one-tailed hypotheses (H2–H5). Effect sizes were calculated for Hedges G pairwise differences (small = 0.2, medium = 0.5, large = 0.8).
Results
Interactions
The mean ± SD results for the combined data set (sprinters and team sports groups together) across all conditions are presented in . Linear mixed effect modelling revealed no significant 3-way interactions (Limb * Condition * Athlete Group) (all F = 0.23–1.234; p = 0.302–0.88). Significant interactions were observed for Limb * Condition in SV (F (3, 204.011) = 3.752; p = 0.012), SF (F (3,206.016) = 5.198; p = 0.002), GCT (F (3, 205.99) = 3.37; p = 0.019), and FT (F (3, 205.99) = 3.37; p = 0.019) but not for SL (F (3,204.289) = 1.408; p = 0.241). No Limb * Athlete Group interactions were observed for any variable (all F = 0.12–2.91; p = 0.089–0.73). No Condition * Athlete Group interactions were observed for SV (F (3, 204.153) = 0.655; p = 0.580), SL (F (3, 204.568) = 0.540; p = 0.655), SF (F (3, 206.199) = 0.713; p = 0.545) or FT (F (3, 206.37) = 1.67; p = 0.174); however, there was a Condition * Athlete Group interaction for GCT (F (3, 206.198) = 3.981; p = 0.009).
Table 2. Mean ± SD for all variables across conditions for left (L) and right (R) steps (team sports group and sprinters group data have been grouped together).
Main effects
Group
No significant main effects were observed for Group: SV (p = 0.234), SL (p = 0.567), SF (p = 0.460), GCT (p = 0.125), or FT (p = 0.16). shows Team Sports group versus Sprint group for SV, SF and GCT for the left and right steps. Post-hoc independent t-tests revealed sprinters produced shorter GCTs for both left and right steps than team sport group in the banked conditions (p > 0.001–0.029; ES = 1.15–1.37), whilst on the flat conditions no significant differences were found (p > 0.05; ES = 0.72–1.33). No differences were observed between groups across any lane for left or right step for SV (p > 0.05; ES = 0.06–0.88), SF (p < 0.05; ES = 0.02–0.68), SL (p > 0.05; ES = 0.05–0.43) and FT (p > 0.05; ES = 0.29-1.43).
Figure 2. Mean ± SD step velocity (top row), step frequency (second row) and ground contact time (bottom row) for sprinters (light grey) versus team sports (dark grey). Left hand side figures (a, c, e) show values for the left step and right-hand side figures (b, d, f) show values for the right step. Note. p < 0.05 and ** denotes p < 0.001 between sprint athletes and team-sports group.
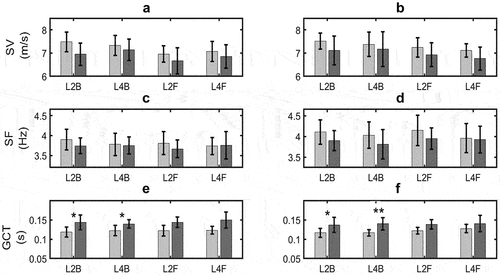
Condition
Significant main effects were observed for condition in SV (p < 0.001), SL (p < 0.001), GCT (p < 0.001) and FT (p = 0.021) but not SF (p = 0.361).
The effect of condition on the left step
Post-hoc comparisons revealed that for SV, both L2B and L4B were greater than both L2F and L4F (all p < 0.05; ES = 0.47-0.87). SF in L2B was greater than in L2F (p = 0.017; ES = 0.32), whilst for SL, both L2B and L4B were greater than both L2F and L4F (all p < 0.05; ES = 0.56-0.67). No pairwise differences were observed for GCT or FT (p > 0.05; ES = 0.122-0.289).
The effect of condition on the right step
For the right step, SV in L2B was greater than in L4F (p = 0.017; ES = 0.50), whilst L4B were greater than L4F (p = 0.041; ES = 0.44). For SF, no pairwise differences were observed between conditions. SL in L2B and L4B was greater than L2F (p = 0.002; ES = 0.68 and p = 0.001; ES = 0.71, respectively). FT in L4B was greater than L2F (p = 0.046; ES = 0.65) with no pairwise differences observed for FT in the other lanes (p > 0.05; ES = 0.06–0.58) or GCT across all conditions (p > 0.05; ES = 0.04–0.60).
Limb
Significant main effects for Limb were observed for SV (p < 0.001), SF (p < 0.001), GCT (p = 0.001) and FT (p = 0.001) but not for SL (p = 0.708). Significant pairwise differences, direction and magnitude of effect size are reported in . No significant differences were observed between left and right SV in L2B, L4B and L4F, FT in L4F and SL or GCT across all lanes (all p > 0.05; ES < 0.51).
Table 3. Test statistics and magnitude of effect size for significant within lane left versus right comparisons.
Discussion
The aim of the study was to examine the short-term responses to different bend conditions on step characteristics in well-trained sprinters and team-sports players. No variables showed a significant three-way interaction between athlete group, limb and condition (all p > 0.05). These results suggest that both participant groups underwent similar responses to the differing bend conditions, and that both participant groups experienced the same effect on each limb as a result of the conditions. Therefore, the first hypothesis is accepted (H1). Previous research has highlighted that team sports athletes often move in a curvilinear fashion (Fitzpatrick et al., Citation2019; Gomez-Carmona et al., Citation2021; Nian, Citation2021) and achieve similar velocities during a bend sprint in comparison to a linear sprint (Filter et al., Citation2020). This is potentially one of the reasons that the team sports athletes were competent at adapting to the different bend conditions. Nonetheless, the sprint athletes produced shorter GCTs than the team sports group for both steps in both L2B and L4B, with this resulting in non-significantly greater SF and SV in the banked conditions (). Thus, H2 can be rejected. Previous research has highlighted that elite sprinters apply greater forces more rapidly during early stance, with this leading to shorter GCT and ultimately faster velocities (Clark & Weyand, Citation2014). This indicates that improving team-sports athletes’ ability to apply force during early stance when bend sprinting would lead to reduced GCTs and thus, faster SVs. Faster SV during bend sprinting could have impact on sporting performance (Caldbeck, Citation2019). Furthermore, this has greater bearing due to the fact that radii of curvilinear sprints typically occur along a curve radius of 3.5–11 m (Brice et al., Citation2004), in comparison to the 13.98 m for lane two and 15.94 m for lane four radii tested in this study. Interestingly, sprinters produced significantly shorter GCTs only in the banked conditions, suggesting that negotiating a banked bend requires specific conditioning and familiarisation. Thus, it could be beneficial to ensure sufficient bend sprint training is undertaken on banked bends prior to indoor competition.
For the left step, results showed SV in both flat conditions to be significantly lower compared to the banked equivalents. Reduced SV occurred through a combination of reduced SL and SF in lane 4 but only reduced SF in lane 2 (). Therefore, for the effect of banking, H3 can be accepted. Lateral banking has previously been shown to affect peak sprinting velocities (Greene, Citation1987; Luo & Stefanyshyn, Citation2012) and competition times (Barnes & Malcata, Citation2017). For example, Luo and Stefanyshyn (Citation2012) found a 4.3% (p = 0.0001) increase in curved sprinting speed when wearing laterally raised footwear in comparison to a flat shoe (recreating a laterally banked surface of 7.5°). The increase in sprint speed occurred as a result of shorter inside limb GCTs and increased SF (Luo & Stefanyshyn, Citation2012), suggesting that SL would have been similar between conditions. Additionally, during cutting manoeuvres on a 10-degree bank, Wannop et al. (Citation2014) found lower inversion angles and reductions in frontal and transverse plane angular impulses, whilst reporting increased angular impulse in the sagittal plane. The authors postulated that the banking assisted in force generation during plantar-flexion which in turn may lead to improved performance. Nonetheless, the methods employed by Luo and Stefanyshyn (Citation2012) and Wannop et al. (Citation2014) are not generalisable to conditions representative of indoor competition and so results should be interpreted with caution. Nonetheless, lateral banking appears to improve SV by enabling athletes to maintain greater SL and SF. However, the reduction in SV in flat conditions appears to occur through larger reductions in SL (larger ES and significant differences in both L2 and L4). Therefore, strategies to improve athlete’s ability to maintain SL on flat and tighter bends could be beneficial to performance. Whilst sprinting linearly, Hunter et al. (Citation2004) report that long step length sprinters achieve significantly larger flight distances through combination of a significantly greater angle at take-off and vertical velocity at take-off. Furthermore, Weyand et al. (Citation2000) highlight that faster running speeds occur through generation of greater vertical ground reaction forces rather than more rapid leg movements. Nonetheless, research is yet to propose mechanisms for improving force production on the bend and ultimately SL. Sprint athletes should ensure that training reflects the nature of competition, for example if competing indoors on a banked track, incorporating bend sprints on banked bends of varying radii in preparation.
For both the left and right steps, no significant differences were found between lane 2 and lane 4, flat or banked. Therefore, for the effect of lane radius, H4 is rejected. Previous research on outdoor athletics tracks found reduced left SV in lane 2 compared to lane 8 as a result of for a reduced SF (Churchill et al., Citation2019). For the right step, reductions in SV due to radius resulted from non-significant reductions in both SL and SF (Churchill et al., Citation2019). On smaller radii, Chang and Kram (Citation2007) found peak velocity to reduce with each decrease in radius (6 m: 5.66 ± 0.08 m/s to 1 m: 2.99 ± 0.07 m/s) through reductions in left and right SL from 1.53 ± 0.1 m (left) and 1.70 ± 0.10 (right) on a 6 m radius to 0.77 ± 0.02 m (left) and 0.80 ± 0.04 (right) on a 1 m radius, whilst SF remained similar. On banked indoor bends, Bezodis and Gittoes (Citation2008) report left SV decreases of 0.2% in lane 4 compared to lane 1, with this coming from a combination of SF and SL. For the right step, Bezodis and Gittoes (Citation2008) reported an increase of 1.5% in SV in lane 4 compared to lane 1. This occurred due to a larger SL but lower SF. Therefore, the interaction of SL and SF appears to be different between left and right steps on differing radii. Bezodis and Gittoes’s (Citation2008) study contained a limited sample size of four well-trained sprinters and so results cannot be generalised to non-trained sprinters such as team-sports athletes. The present study found that no significant differences were observed between lanes 2 and 4, it is possible that the difference in radii (1.96 m) is not sufficiently large to see meaningful difference in step characteristics.
Right SV was greater than left in all conditions except L4F, however only in L2F did this reach statistical significance. A similar pattern emerged for SF, where right SF was significantly greater in L2B, L2F and L4F. Faster right steps occurred due to shorter FT than left in all conditions except for L4F (). Consequently, the fifth hypothesis is partially accepted (H5) due to the right step achieving faster SV as a result of shorter FT rather than GCT. These findings agree with previous research in that the left steps have lower SF (Churchill et al., Citation2016) but contrast to the mechanism for reduced SF. For example, previous research has found reduced left SF to occur as a result of longer GCTs rather than FTs (Alt et al., Citation2015; Churchill et al., Citation2015, Citation2019). Nonetheless, the longer left FT observed in the present study suggests that it took participants longer to reposition the left limb in preparation for touchdown, potentially as a result of the more altered kinematics during the left step across the lower extremity during bend sprinting (Alt et al., Citation2015; Churchill et al., Citation2015; Judson et al., Citation2020b). On the other hand, Ishimura and Sakurai (Citation2016) found both GCT and FT to be longer for the left step in comparison to the right step during maximal sprints on a 43.51 m radius. It has been suggested that longer left GCTs occur due to the left step generating medial-lateral forces in order to follow the path of the bend (Churchill et al., Citation2016), with previous research demonstrating that on tighter radii these requirements are greater (Chang & Kram, Citation2007). Nevertheless, these were carried out on flat bends of either too small or too large radii for comparison; thus, the lateral banking may reduce the disproportionate effect on GCT for the left step by aligning the ankle in a more neutral position (Greene, Citation1987), enabling more efficient force production during stance (Luo & Stefanyshyn, Citation2012) and thus, reducing the GCT. No differences in SL were observed between limbs across conditions, supporting previous anecdotal research where SF contributes to reductions in left SV on indoor radii (Bezodis & Gittoes, Citation2008).
Team sports athletes struggled to maintain short GCT on tight and banked bends compared to sprinters. To improve bend sprinting performance, it may be beneficial for team sports athletes to undertake high-velocity sprints on radii representative of game situations, such as the bend sprint test established by Filter et al. (Citation2020), using the arc of the penalty area. In addition, research has suggested that focusing on bend-specific strength and conditioning exercises, such as aiming to increase plantar flexion force production with a body lean and in internally rotated positions may be beneficial for performance (Judson et al., Citation2020b; Luo & Stefanyshyn, Citation2012). Further, the laterally resisted split squat has been proposed to closely mimic the demands of change of direction tasks via reorientation of the force vector (Maddy, Citation2020). Due to the similar multi-planar requirements between bend sprinting and change of direction, the laterally resisted split squat has the potential to act as a more specific training exercise to help prepare and develop athletes for the demands of bend sprinting. Wearable resistance may also provide an additional training stimulus during bend sprinting, as has been shown to lead to improvements (or minimise reductions due to detraining) in linear sprint performance (Feser, Bayne, et al., Citation2021; Feser, Korfist, et al., Citation2021). Furthermore, tethered or assisted sprinting may have the potential to improve bend sprint performance (Chang & Kram, Citation2007). However, more research is needed to confirm these methods.
This investigation is not without limitations. For example, the standardisation of velocity was not achievable due to the effect different lane conditions had on velocity (Greene, Citation1987). Furthermore, despite encouraging plenty of rest between trials, eight 80 m sub-maximal sprints may induce fatigue that may start to impact quality of the trials. To counter the effect of fatigue, the order of trials was randomly generated for every participant and sufficient rest was taken between trials. The team sport players may have been less effectively able to regulate their effort than the sprint group (i.e., 85% of perceived maximum); thus; for comparisons between groups, the average of the two trials was taken for further analysis. The sprint group included six males (200 m personal best = 23.35 ± 0.86 s) and two females (200 m personal best = 25.49 ± 0.91 s); thus, the direct comparison between sprint and team-sports groups may have been influenced by this difference in participant characteristics. Nonetheless, the aim of the study was to investigate the short-term responses to bend sprinting and not male versus female differences; thus, females were not excluded from participation in this study.
Conclusion
This study is the first to report step characteristics during bend sprinting in well-trained sprinters and athletes who regularly sprint as part of their sport (team sports athletes). Team sports athletes responded to conditions in the same manner as sprinters for all variables; however, sprint athletes produced significantly shorter GCTs in the banked conditions in comparison to the team sports athletes, leading to non-significant increases in SF and SV. These results suggest that the sprinters’ physical preparation improves capacity to generate force more rapidly, leading to shorter GCTs on banked bends of tight radii and non-significant increases in SF and SV. Furthermore, familiarity with sprinting on banked bends appears to be beneficial due to the fact sprinters had competed on banked indoor bends previously (within 6 months prior to testing). Therefore, this study highlights the importance of adaptation to a less familiar movement pattern for sprint athletes, suggesting that improved bend sprinting performance on banked bends requires specific conditioning and familiarity with running on banked bends representative of indoor competition. Whilst previous literature has found faster SV or performance times on banked bends (Barnes & Malcata, Citation2017; Greene, Citation1987; Luo & Stefanyshyn, Citation2012), this study provides new understanding of the mechanism driving increased SV on banked bends. Greater SV on the banked bends was brought about by increased SL for the left and right steps, with a trend for similar SF observed between banked and flat conditions. This suggests that strategies to maintain step length on flat and tight radii have the potential to improve sprinting performance under these conditions. Consistent with previous research, the left step was more affected by the bend conditions than the right step; however, the reduced left SF was brought about by greater FT rather than increased GCT. Future research should focus on the more detailed kinematics of bend sprinting on flat and banked radii in order to further understand the demands of bend conditions typical of indoor competition.
Disclosure statement
No potential conflict of interest was reported by the author(s).
Additional information
Funding
References
- Alt, T., Heinrich, K., Funken, J., & Potthast, W. (2015). Lower extremity kinematics of athletics curve sprinting. Journal of Sports Sciences, 33(6), 552–560. https://doi.org/10.1080/02640414.2014.960881
- Barnes, K. R., & Malcata, R. (2017). Conversion index for running on different indoor track and field facility types. International Journal of Performance Analysis in Sport, 17(4), 375–384. https://doi.org/10.1080/24748668.2017.1346453
- Bezodis, I. N., & Gittoes, M. J. R. (2008). Bilateral differences in step characteristics when sprinting on the straight and banked bend of an indoor 200 m track. Proceedings of the 26th International Conference on Biomechanics in Sports, July 14–18, Seoul, Korea (pp. 673–676).
- Brice, P., Smith, N., & Dyson, R. (2004). Frequency of curvilinear motion during competitive soccer play. Communications to the fourth congress of science and football. Journal of Sports Sciences, 22, 504. https://doi.org/10.4324/9780203412992
- Caldbeck, P. (2019). Contextual Sprinting in Football. Impress [ DSportExSci thesis] John Moores University:
- Chang, Y.-H., & Kram, R. (2007). Limitations to maximum running speed on flat curves. Journal of Experimental Biology, 210(6), 971–982. https://doi.org/10.1242/jeb.02728
- Churchill, S. M., Salo, A. I. T., & Trewartha, G. (2015). The effect of the bend on technique and performance during maximal effort sprinting. Sports Biomechanics, 14(1), 106–121. https://doi.org/10.1080/14763141.2015.1024717
- Churchill, S. M., Trewartha, G., Bezodis, I. N., & Salo, A. I. T. (2016). Force production during maximal effort bend sprinting: Theory vs reality: Force production during bend sprinting. Scandinavian Journal of Medicine & Science in Sports, 26(10), 1171–1179. https://doi.org/10.1111/sms.12559
- Churchill, S. M., Trewartha, G., & Salo, A. I. T. (2019). Bend sprinting performance: New insights into the effect of running lane. Sports Biomechanics, 18(4), 437–447. https://doi.org/10.1080/14763141.2018.1427279
- Clark, K. P., & Weyand, P. G. (2014). Are running speeds maximized with simple-spring stance mechanics? Journal of Applied Physiology, 117(6), 604–615. https://doi.org/10.1152/japplphysiol.00174.2014
- Douglas, J., Pearson, S., Ross, A., & McGuigan, M. (2020). Reactive and eccentric strength contribute to stiffness regulation during maximum velocity sprinting in team sport athletes and highly trained sprinters. Journal of Sports Sciences, 38(1), 29–37. https://doi.org/10.1080/02640414.2019.1678363
- Exell, T., Irwin, G., Gittoes, M., & Kerwin, D. (2017). Strength and performance asymmetry during maximal velocity sprint running. Scandinavian Journal of Medicine & Science in Sports, 27(11), 1273–1282. https://doi.org/10.1111/sms.12759
- Feser, E. H., Bayne, H., Loubser, I., Bezodis, N. E., & Cronin, J. B. (2021). Wearable resistance sprint running is superior to training with no load for retaining performance in pre-season training for rugby athletes. European Journal of Sport Science, 21(7), 967–975. https://doi.org/10.1080/17461391.2020.1802516
- Feser, E. H., Korfist, C., Lindley, K., Bezodis, N. E., Clark, K., & Cronin, J. (2021). The effects of lower-limb wearable resistance on sprint performance in high school American football athletes: A nine-week training study. International Journal of Sports Science & Coaching, 16(5), 1187–1195. https://doi.org/10.1177/17479541211003403
- Filter, A., Olivares-Jabalera, J., Santalla, A., Morente-Sánchez, J., Robles-Rodríguez, J., Requena, B., & Loturco, I. (2020). Curve sprinting in soccer: Kinematic and neuromuscular analysis. International Journal of Sports Medicine, 1144–3175. https://doi.org/10.1055/a-1144-3175
- Fitzpatrick, J. F., Linsley, A., & Musham, C. (2019). Running the curve: A preliminary investigation into curved sprinting during football match-play. Sports Performance & Science Reports, 55(1), 1–3. Retrieved 10 Feburary, 2022, from https://sportperfsci.com/
- Gomez-Carmona, C. D., Reina, M., Pino-Ortega, J., & Ibanez, S. J. (2021). Effect of isolated vs in-game curvilinear displacements in multi-location external workload profile. A case study in semi-professional basketball players. Journal of Sport Psychology, 30(2), 309–317. Retrieved 23 August, 2022, from https://www.rpd-online.com/index.php/rpd/article/view/382
- Greene, P. R. (1985). Running on flat turns: Experiments, theory, and applications. Journal of Biomechanical Engineering, 107(2), 96–103. https://doi.org/10.1115/1.3138542
- Greene, P. R. (1987). Sprinting with banked turns. Journal of Biomechanics, 20(7), 667–680. https://doi.org/10.1016/0021-92908790033-9
- Hunter, J. P., Marshall, R. N., & Mcnair, P. J. (2004). Interaction of step length and step rate during sprint running. Medicine & Science in Sports & Exercise, 36(2), 261–271. https://doi.org/10.1249/01.MSS.0000113664.15777.53
- Ishimura, K., & Sakurai, S. (2016). Asymmetry in determinants of running speed during curved sprinting. Journal of Applied Biomechanics, 32(4), 394–400. https://doi.org/10.1123/jab.2015-0127
- Judson, L. J., Churchill, S. M., Barnes, A., Stone, J. A., Brookes, I. G. A., & Wheat, J. (2020a). Measurement of bend sprinting kinematics with three-dimensional motion capture: A test–retest reliability study. Sports Biomechanics, 19(6), 761–777.
- Judson, L. J., Churchill, S. M., Barnes, A., Stone, J. A., Brookes, I. G. A., & Wheat, J. (2020b). Kinematic modifications of the lower limb during the acceleration phase of bend sprinting. Journal of Sports Sciences, 38(3), 336–342. https://doi.org/10.1080/02640414.2019.1699006
- Luo, G., & Stefanyshyn, D. (2012). Ankle moment generation and maximum-effort curved sprinting performance. Journal of Biomechanics, 45(16), 2763–2768. https://doi.org/10.1016/j.jbiomech.2012.09.010
- Maddy, D. (2020). Analyzing change-of-direction and the laterally resisted split squat. Boise State University.
- Nagahara, R., & Zushi, K. (2013). Determination of foot strike and toe-off event timing during maximal sprint using kinematic data. International Journal of Sport and Health Science, 11, 96–100. https://doi.org/10.5432/ijshs.201318
- Nian, H. (2021). Curvilinear running demands for a fullback in rugby league. Journal of Australian Strength and Conditioning, 29(1), 21–27. retrieved 23 August, 2022, from https://www.strengthandconditioning.org/jasc-29-1/2930-from-the-field-case-study-curvilinear-running-demands-for-a-fullback-in-rugby-league
- Ohnuma, H., Tachi, M., Kumano, A., & Hirano, Y. (2018). How to maintain maximal straight path running speed on a curved path in sprint events. Journal of Human Kinetics, 62(1), 23–31. https://doi.org/10.1515/hukin-2017-0175
- Quinn, M. D. (2009). The effect of track geometry on 200- and 400-m sprint running performance. Journal of Sports Sciences, 27(1), 19–25. https://doi.org/10.1080/02640410802392707
- Ryan, G. J., & Harrison, A. J. (2003). Technical adaptations of competitive sprinters induced by bend running. New Studies in Athletics, 18(4), 57–70. Retrived 10 Feburary, 2022, from https://worldathletics.org/nsa
- Smith, N., Dyson, R., Hale, T., & Janaway, L. (2006). Contributions of the inside and outside leg to maintenance of curvilinear motion on a natural turf surface. Gait & Posture, 24(4), 453–458. https://doi.org/10.1016/j.gaitpost.2005.11.007
- Speelman, C. P., & McGann, M. (2013). How mean is the mean? Frontiers in Psychology, 4, 4. https://doi.org/10.3389/fpsyg.2013.00451
- Usherwood, J. R., & Wilson, A. M. (2006). Accounting for elite indoor 200 m sprint results. Biology Letters, 2(1), 47–50. https://doi.org/10.1098/rsbl.2005.0399
- Wannop, J. W., Graf, E. S., & Stefanyshyn, D. J. (2014). The effect of lateral banking on the kinematics and kinetics of the lower extremity during lateral cutting movements. Human Movement Science, 33, 97–107. https://doi.org/10.1016/j.humov.2013.07.020
- Weyand, P. G., Sternlight, D. B., Bellizzi, M. J., & Wright, S. (2000). Faster top running speeds are achieved with greater ground forces not more rapid leg movements. Journal of Applied Physiology, 89(5), 1991–1999. https://doi.org/10.1152/jappl.2000.89.5.1991