ABSTRACT
Badminton footwork has been characterised with jump-landing, cross step, side side and lunges, which requires movement agility to facilitate on-court performance. A novel badminton shoe design with systematic increase of lateral wedge hardness (Asker C value of 55, 60, 65, and 70) was developed and investigated in this study, aiming to analyse the dose–response effect of incremental wedge hardness on typical badminton footwork. Stance time and joint stiffness were employed to investigate the footwork performance, and the factorial Statistical non-Parametric Mapping and Principal Component Analysis (PCA) were used to quantify the biomechanical responses over the stance. As reported, shorter contact times (decreased by 8.9%–13.5%) and increased joint stiffness (in side step) of foot-ankle complex were found, suggesting improved footwork stability and agility from increased hardness. Time-varying differences were noted during the initial landing and driving-off phase of cross and side steps and drive-off returning of lunges, suggesting facilitated footwork performance. The reconstructed modes of variations from PCA further deciphered the biomechanical response to the wedge dosage, especially during drive-off, to understand the improved footwork agility and stability.
Introduction
Badminton is one of the most popular racquet sports, which has attracted millions of populations around the world (Phomsoupha & Laffaye, Citation2015; Yu & Mohamad, Citation2022). Due to the characteristics of high-intensity and intermittent motions as the fastest racquet sport (Alder et al., Citation2019; Phomsoupha & Laffaye, Citation2015), badminton playing populations showed significant benefits of improved overall health quality, a reduced ratio of all-cause and cardiovascular-disease mortality (Oja et al., Citation2017). An efficient on-court badminton performance was reported as the primary goal of both recreational and athletic cohorts (Mei et al., Citation2017), which required coordination of the upper extremity, torso, and lower extremity, and physical capability of fast, short-duration, and high-intensity power output (Alder et al., Citation2019; Manrique & González-Badillo, Citation2003; Ooi et al., Citation2009).
Efficient badminton footwork motions, such as jump-landing, orthogonal and diagonal lunges, cross and side steps, rapid cutting and turning, would assist the coordination of kinetic chain in the upper extremities with handwork and racket to hit shuttle and score (Phomsoupha & Laffaye, Citation2015; Valldecabres et al., Citation2020). As reported, excellent execution of footwork would enable rapid movement into the appropriate position for the shuttle returning (Kuntze et al., Citation2010; Yu & Mohamad, Citation2022). A recent study of on-court movements during the Badminton World Championship found that the diagonal footwork across the court took up the main part among elite badminton players (Valldecabres et al., Citation2020), especially the lunges following the cross and side steps. The lunge footwork was previously investigated as a particular interest to improve performance and prevent injury (Cronin et al., Citation2003; Lam et al., Citation2020; Lee & Loh, Citation2019; Mei et al., Citation2017). Consequently, specific factors, such as fatigue conditions (Herbaut & Delannoy, Citation2020; Valldecabres et al., Citation2020), athletic level (Mei et al., Citation2017), footwear design and property (Hong et al., Citation2016; Lam et al., Citation2017, Citation2018; Wei et al., Citation2009), physiological and motor demand (Cronin et al., Citation2003; Kuntze et al., Citation2010) were elaborated and discussed to achieve the goal.
Considering the high intensity and agility characteristics, certain properties of badminton footwear, such as impact absorption, upper elasticity (wrapping), midsole torsion, and outsole traction are required to facilitate performance improvement and injury prevention (Chen et al., Citation2022; Hong et al., Citation2016; Lam et al., Citation2017; Mei et al., Citation2014; Shen et al., Citation2022). Three key components, specifically shoe comfort, injury prevention, and performance, were proposed (Reinschmidt & Nigg, Citation2000) thus employed to evaluate sports shoes (Mei et al., Citation2014), with further highlighted features of lateral stability, torsion stiffness, impact cushion, and traction to meet the demand of court sports shoes. In specifics, the lateral stability, defining as the ability to resist over supination or inversion in the foot-ankle complex (Reinschmidt & Nigg, Citation2000) and prevention of ankle sprain during cutting (Tik-Pui Fong et al., Citation2007), was a crucial property, considering the high ratio of diagonal footwork, such as side-step cutting and cross-step turnings, and lunges as documented in the literature (Kuntze et al., Citation2010; Lam et al., Citation2017; Mei et al., Citation2017; Valldecabres et al., Citation2020; Yu et al., Citation2021). Lateral stability was further combined with torsional stiffness, especially for the court sport shoes, to resist the twisting and over inversion and provide stable support during cutting or change of direction movements (Bagehorn et al., Citation2023; Graf & Stefanyshyn, Citation2013; Martin et al., Citation2023; Zifchock et al., Citation2017). A recent study on modulating the lateral midsole of badminton shoes to investigate the cutting (change of direction) performance found that higher ground reaction forces and shorter contact times with modified sole structure (Chen et al., Citation2022), may be a predictor of improved cutting performance on the court. While the study lacked a systematic analysis of dose–response effect from the lateral wedge design, which has been widely used in orthotics to revise foot malalignment (Telfer et al., Citation2013).
Badminton shoes, as a unique functional footwear in court sports, should meet the demands of perceived comfort and sufficient support from the perspectives of biomechanical output, athletic performance, and injury prevention. Badminton footwear with a novel lateral wedge of systematically incremental hardness was developed in this study, which may assist the footwork stability and mechanical alignment of the foot-ankle complex during stance. This study was firstly aimed to investigate the dose–response influence of the incremental wedge stiffness on the biomechanical performance of typical badminton footwork, specifically, the forecourt lunges (of the right limb), and the diagonal side-step and cross-step footwork (of the left limb). It was hypothesized that the biomechanical parameters in the ankle and subtalar joints would change significantly with the systematically increased hardness of the lateral wedge in the midsole. The dose–response effect was further deciphered and quantified using advanced statistics of Factorial Statistical non-Parametric Mapping to check the significance (Trama et al., Citation2021) and Principal Component Analysis (PCA) (Yu, Mei et al., Citation2021) for the time-varying biomechanical parameters during stance.
Materials and methods
Participants
A total of 15 university-level male badminton players (age: 26 ± 2.24 yrs, mass: 68.3 ± 4.5 kg, height: 1.76 ± 0.42 m, BMI: 22.13 ± 0.66 kg/m^2, years of playing: 7 ± 0.66 yrs) were recruited for this study, following the inclusion criteria, (1) right-side dominant (as determined with the dominant hand for badminton racquet), (2) experience of participating the provincial University badminton competition (athletic level defined as the regional elite or national level division-II by the coach), and (3) preferred shoe size of 42 (EU) and foot length of 260 ± 5 mm (which is the same as the size of badminton footwear). The sample size was calculated using GPower v3.1 (Faul et al., Citation2007) with an ANOVA F test for repeated measures within factors of a lateral wedge with incremental hardness, with effect size (f) of 0.5, a-level of 0.05 and power value of 0.996. No lower extremity injuries or foot disorders were reported prior to six-month before the experiment for all badminton players. The study was approved by the ethics committee from the research institute in the University (RAGH20220518), and all participants were informed of the test objectives, procedures, and requirements with written consent.
Footwear
The footwear employed for the biomechanical tests in this study was the Li-Ning YT-01 badminton shoe, with a uniform size of 42 (EU). As illustrated in , a forefoot lateral wedge of irregular trapezoid shape in the midsole was designed with an incremental hardness of 60, 65, and 70 (Asker C), compared to the currently standardised hardness of 55 (Asker C). The rearfoot and forefoot stack heights were 34 mm and 17 mm, respectively. The geometrical specifications of the lateral wedge included the lateral arc length of 75 mm, trapezoid with a lateral and medial length of 72 mm and 51 mm (respectively), and width of 23 mm (one-third of the forefoot width). The experimental shoes were identical in the materials and upper design and are defined as LN55, LN60, LN65, and LN70 as per the Asker C hardness. The perception of shoe comfort was measured using a previously established 150 mm VAS (Visual Analogue Scale) scoring system (Mei et al., Citation2018; Mündermann et al., Citation2002), specifically including the Overall Comfort (1), Forefoot Comfort (2), Forefoot Upper Stability (3), Forefoot Wrapping (4), Midfoot Support (5), Forefoot Torsion (6), Forefoot Cushioning (7), Forefoot Lateral Stiffness (8), Weight (9), and Comprehensive Property (10).
Experimental protocol
The typical badminton footwork includes forehand and backhand lunges to the right-forward (RF) and left-forward (LF) court and cross-step (CS) from the backhand rear court and side-step (SS) from the forehand rear court. More specific details of the four typical footwork and lab setup are illustrated in . All participants are experienced badminton players with right-sided dominance for racquet-grasp and right leg performing lunges and left leg performing cross or side steps, as badminton footwork typically involves unilateral hand and foot (Yu & Mohamad, Citation2022; Yu et al., Citation2021). Specifically, all badminton players initiated the RF and LF lunges with a split step, stepping up the left foot, followed by the right leg and foot for lunges to the right forecourt or the left forecourt. The lunging stance includes heel landing, loading acceptance, and drive-off, which was defined according to a previous study (Kuntze et al., Citation2010). Players initiated the cross-step (CS) with an overhead clear in the backhand rear-court, chasse step, followed by crossing the left leg with heel landing, drive, and push-off, and finished with lunging of the right leg. The side-step (SS) was initiated with a clear in the forehand rear-court, chasse step, followed by side-stepping (cutting) the right leg with heel landing, drive, and push-off, and finished with lunging of the right leg. Thus, the lower limb of interest for lunging footwork was the right side (RF, LF), and the side or cross -step footwork was the left side (SS, CS) in the current study.
Figure 2. Illustration of lab-simulated court setup (left) and the typical badminton footwork of RF, LF, CS and SS (right).
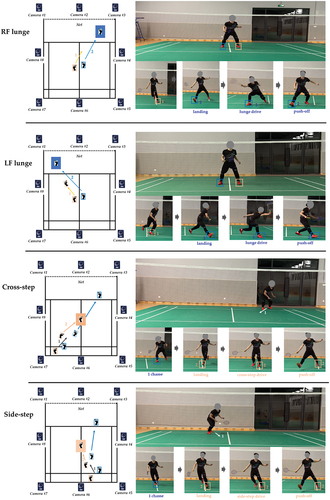
Once confirming the typical badminton footwork, a lab-simulated badminton court facilitated with an 8-camera Vicon motion capture system and synchronously connected AMTI force plates, was set up for the biomechanical experiment to record the markers’ positions and ground reaction forces during badminton footwork (Mei et al., Citation2017; Yu et al., Citation2021). The data collection frequency was set at 200 Hz and 1000 Hz, respectively. The marker set model in this study included markers to both acromion of the torso, bilateral ASIS and PSIS of the pelvis, 4-marker cluster to the lateral aspect of both thighs, medial and lateral knee epicondyles, 4-marker cluster to the lateral aspect of both shank, medial and lateral ankle malleoli, posterior calcaneus, anterior toe-tip, medial M1 and lateral M5 of the bilateral lower limb. The model was employed and validated in our previous study of badminton directional lunges (Yu et al., Citation2021), and other similar data-driven musculoskeletal modelling studies (Mei et al., Citation2019; Rajagopal et al., Citation2016).
The lab setup included a badminton net and stick-hang shuttlecock in the target region for lunges, cross, and side-steps to mimic real court situations. The distance of preparing the position for the RF and LF lunges to the force plate was 2.5 times the leg length of each participant (Yu et al., Citation2021). The SS and CS mimicked a real court distance as shown in .
Prior to the data collection, badminton players were required to perform warm-up and lab court familiarisation practice with randomly selected footwear for 10 min. The four typical footwork was conducted as per the standard during training and visually supervised by an experienced coach. The approaching velocity was defined as the speed from initiated position to the foot contact of forceplate (Lam et al., Citation2017), which was manually controlled with stopping watch by the coach. During the experiment, all badminton players would randomly wear the four pairs of badminton shoes to conduct the footwork without informing the difference (single-blind). During the data collection, a static trial was firstly collected for each pair of badminton footwear, then performed the RF, LF, CS, or SS footwork in self-selected order. The interval time of 10 min between each shoe pair was controlled to minimise fatigue influence. Marker trajectories and ground reaction forces from three successful trials for each footwork were collected with footwear of LN55, LN60, LN65, and LN70 for post-data processing.
Data processing
The target limb for RF and LF footwork was the right limb, and the target limb for CS and SS footwork was the left limb. This study aimed to investigate the influence of incremental lateral wedge hardness on badminton footwork performance. Thus, the stance phase, as a close chain, was focused to analyse the contact times, joint angles, joint moments, and joint stiffness, which was defined from the threshold of 20N in vertical ground reaction force (Yu, Mei et al., Citation2021). The approaching velocity was also controlled with stopping watch as the biomechanical parameters are sensitive to velocity. The velocity was calculated using the resultant speed of the bilateral ASIS and PSIS markers in the pelvis.
The collected marker trajectories and ground reaction force data were visually inspected, and gap filling was performed with pattern fill in Vicon Nexus software. The raw data were then stored as C3D files for processing with customised Matlab script, to generate “trc” and “mot” files with a zero-phase fourth-order Butterworth low-pass filtered frequency at 6 Hz for markers and 30 Hz for forces (Mei et al., Citation2019; Yu et al., Citation2021)
A musculoskeletal OpenSim model with upgraded knee abduction/adduction and internal/external rotation DoFs (degrees of freedom) was employed in this study for data processing as per a standardised pipeline (Rajagopal et al., Citation2016). The generic model was firstly scaled to match the anthropometrics of each participant with anatomically adjusted inertia and moment arms. Inverse kinematics was then performed to calculate joint angles of the hip, knee and ankle, which were then employed to compute the joint moments following the Inverse Dynamics algorithm. The joint stiffness was calculated using the change of moment (∆M) divided by the change of angles (or range of motion) (RoM) during stance.
Statistical analysis
Prior to statistical analysis, the discrete parameters, including the VAS scores of perceived shoe comfort, approaching velocity, contact times, joint range of motion, and joint stiffness, were firstly checked with Shapiro–Wilk normality distribution, then repeated measures ANOVA were run with post hoc Tukey test. The results of VAS comfort are presented in the Supplementary Materials (1).
Due to the 1-dimensional feature, the waveform data of joint angles and moments were firstly interpolated with a cubic spline, registering into 101 data-point to represent the stance (100%) (Mei et al., Citation2021). Three trials of each footwork were averaged to avoid inter-trial variations for each player with four pairs of badminton shoes. Considering the time-varying features of angles and moments from the four conditions with different badminton shoes (LN55, LN60, LN65, LN70), a recently developed fctSnPM (factorial Statistical non-Parametric Mapping) (Trama et al., Citation2021), upgrading from SPM1d (Pataky, Citation2010), with the main advantage of not requiring data in a Gaussian distribution, was employed to check the statistical difference among the incremental lateral wedge hardness on footwork biomechanics. The effect size was firstly checked, followed by further post hoc tests with the Bonferroni correction of six comparison factors (with p = 0.05/6, 0.0083). There were several 10/a permutations for each test (Trama et al., Citation2021). The effect size (ES) was defined as trivial when smaller than 0.2, small when between 0.2 and 0.5, medium when between 0.5 and 0.8, and larger when greater than 0.8 (Trama et al., Citation2023), and presented in the Supplementary Materials (2).
To further quantify the dose–response effect of incremental wedge stiffness, a statistical principal component analysis (PCA) was modelled for the time-varying joint angles and moments, to further reveal the influence of lateral wedge hardness on the biomechanical performance during typical badminton footwork. In this study, the variations of main PCs and accumulation in percentage were presented, followed by presenting key variances in the upper (+) (95th percentile, high) and lower (▽) (5th percentile, low) limits of the first PC against the mean waveforms (Ramsay & Silverman, Citation2005; Warmenhoven et al., Citation2021; Yu; Mei et al., Citation2021). All the statistical analysis and modelling was run using Matlab R2020a (The MathWorks Inc., MA, United States).
Results
Discrete parameters
The approaching velocity during the RF and LF lunges was recorded at around 2.0 m/s, while the approaching velocity during the CS and SS footwork was recorded at around 2.5 m/s. The range of motion (ROM) in the ankle and subtalar joints showed no significance during the RF, LF, CS, and SS footwork with the badminton shoes of incremental lateral wedge hardness. While the perceived shoe comfort of the VAS scores in the 10-item was included in the Supplementary Materials (1).
illustrated the comparison of stance time of typical footwork with mean, standard deviation, median, and quartiles. Specifically, the stance time (unit in second, 95% confidence interval, CI) during RF lunge was 0.66 ± 0.04 [95%CI: 0.64–0.67], 0.66 ± 0.3 [95%CI: 0.64–0.7], 0.64 ± 0.04 [95%CI: 0.61–0.64], and 0.61 ± 0.02 [95%CI: 0.6–0.62] for LN55, LN60, LN65, and LN70, respectively. Compared to the mean of LN55 and LN60, the stance time was reduced by 3.9% (LN65, p = 0.001) and 7.9% (LN70, p = 0.004).
Figure 3. Comparison of stance time during typical badminton footwork with incremental lateral wedge hardness, with highlighted mean values connected in blue lines, median values in black lines, minimal and maximal values, and 1st quartile and 3rd quartiles in box.
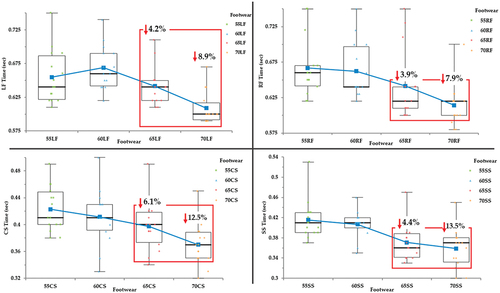
As for the LF lunge, the stance time was 0.66 ± 0.04 [95%CI: 0.62–0.69], 0.66 ± 0.03 [95%CI: 0.64–0.69%], 0.64 ± 0.02 [95%CI: 0.62–0.65], and 0.6 ± 0.02 [95%CI: 0.59–0.62], respectively. Similarly, an approximate reduction of 4.2% (LN65, p = 0.021) and 8.9% (LN70, p = 0.0007) was found while comparing to LN55 and LN60 during stance.
As for the CS footwork, the stance time was 0.42 ± 0.03 [95%CI: 0.4–0.45], 0.41 ± 0.04 [95%CI: 0.39–0.43], 0.39 ± 0.03 [95%CI: 0.37–0.42], and 0.37 ± 0.03 [95%CI: 0.35–0.39]. Compared to the mean of LN55 and LN60, an approximate reduction of 6.1% (LN65, p = 0.304) and 12.5% (LN70, p = 0.373) was observed.
The SS footwork, the stance time was 0.41 ± 0.03 [95%CI: 0.39–0.43], 0.4 ± 0.02 [95%CI: 0.4–0.42], 0.37 ± 0.03 [95%CI: 0.34–0.39], and 0.35 ± 0.03 [95%CI: 0.33–0.38]. An approximate reduction of 4.4% (LN65, p = 0.019) and 13.5% (LN70, p = 0.29) was found compared to the LN55 and LN60 during SS footwork.
In terms of the joint stiffness, the statistical significance was observed only during the SS footwork with incremental wedge hardness (), without finding a difference from the other footwork. Specifically, the LN70 had greater ankle flexion-extension stiffness (4.98 ± 2.47, [95%CI: 3.03–7.71]) compared to LN65 (3.84 ± 1.57, [95%CI: 2.46–5.13], p = 0.021), LN60 (3.66 ± 1.22, [95%CI: 2.4–4.87], p = 0.014), and LN55 (3.62 ± 1.23, [95%CI: 2.68–4.34], p = 0.001), respectively. The subtalar joint stiffness of LN70 (2.34 ± 1.0, [95%CI: 1.58–3.2]) was greater than LN60 (2.01 ± 0.49, [95%CI: 1.67–2.49], p = 0.02) and LN55 (2.13 ± 0.59, [95%CI: 1.74–2.56], p = 0.013). The LN65 (2.39 ± 0.98, [95%CI: 1.79–3.27]) was also increased significantly compared to LN60 (p = 0.019) and LN55 (p = 0.021).
Factorial statistical non-parametric mapping (fctSnpm)
In the current study, the dose–response effect on biomechanics in the foot-ankle complex was primarily investigated and reported, while the statistics of the hip and knee joints are available in our online project repository, and the effect size (ES) waveforms are attached in the Supplementary Materials (2). Ankle dorsi and plantar flexion angles showed no difference in response to the increased lateral wedge hardness. The CS and SS reported statistical differences in the heel landing and foot push-off phases during stance (). For the CS step, the wedge stiffness affected the ankle dorsiflexion angles during 0–12% (F = 4.54, p < 0.05) and 43–100% (F = 4.54, p < 0.05) of the stance. Specifically, LN55 and LN70 differed at 0–12% (p = 0.0083, t* = 5.09) and 46–100% (p = 0.0083, t* = 5.09), LN60 differed at landing (1–5%) from LN65 (p = 0.0083, t* = 5.16), while LN60 and LN70 differed at 76–100% (p < 0.0083, t* = 4.31), and LN65 and LN70 differed at 1–12% (p − 0.0083, t* = 4.39) and 90–100% (p = 0.0083, t* = 4.39). As observed during SS footwork, difference during the 1–17% (F = 4.189, p < 0.05) and 54–100% (F = 4.189, p < 0.05) of stance was found, with significance observed between LN55 and LN60 at 13–18% (p = 0.0083, t* = 4.02), LN55 and LN70 at 86–100% (p = 0.0083, t* = 4.81), LN60 and LN70 at 1–16% (p = 0.0083, t* = 5.38) and 78–100% (p = 0.0083, t* = 5.38), and LN65 and LN70 at 1–8% (p = 0.0083, t* = 4.58).
Figure 5. Factorial SnPM statistics of ankle dorsi (+) – plantar (-) flexion angles during LF, RF, CS and SS footwork with badminton shoes of incremental lateral wedge hardness (stiffness). Notes: highlighted regions of significant cluster in blue, and bicolour transverse bar of significance between two conditions with upper color larger than the lower.
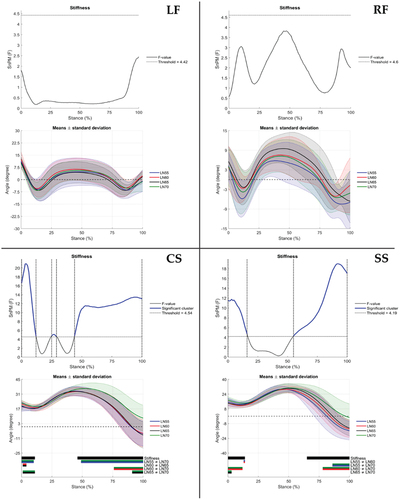
In terms of the ankle dorsi and plantar flexion moments (), the incremental stiffness showed influence at 72–82% of LF (F = 5.18, p < 0.05), 1–4%, 9–13% and 31–86% of RF (F = 5.26, p < 0.05), 9–36%, and 67–94% of CS (F = 5.32, p < 0.05), and 16–19% and 35–46% of SS (F = 4.72, p < 0.05). In specifics, no significance was found between shoe conditions during the LF step, while during the RF step the LN55 and LN60 differed at 2–4% and 46–84% (p = 0.0083, t* = 4.26), LN55 and LN65 differed at 36–81% (p = 0.0083, t* = 4.36), and LN55 and LN70 differed at 44–84% (p = 0.0083, t* = 4.35). For the CS footwork, LN55 and LN70 differed at 11–38% (p = 0.0083, t* = 4.02), and LN65 and LN70 differed at 11–20% and 69–83% (p = 0.0083, t* = 4.55). The LN55 and LN70 differed at 29–45% (p = 0.0083, t* = 4.305), and LN60 and LN70 differed at 16–25% (p = 0.0083, t* = 4.282) in the SS footwork.
Figure 6. Factorial SnPM statistics of ankle dorsi (+) – plantar (-) flexion moments during LF, RF, CS, and SS footwork with badminton shoes of incremental lateral wedge hardness (stiffness). Notes: highlighted regions of the significant cluster in blue, and bicolor transverse bar of significance between two conditions with the upper color larger than the lower.
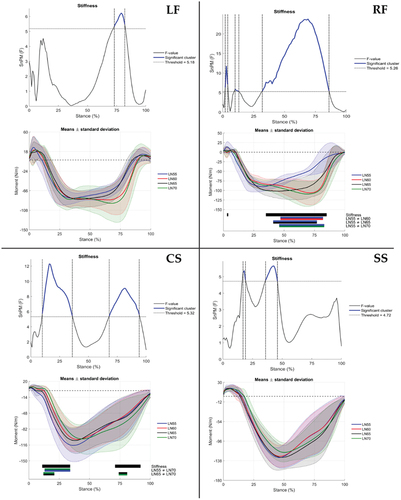
While for the subtalar inversion and eversion angles (), the increased wedge stiffness showed no significance in the LF step and inter-shoe differences, only subtle influence was found during 3–7% (F = 4.476, p < 0.05) of RF step without reporting inter-shoe differences. While the stiffness affected the subtalar angles during 3–37% and 92–100% of CS footwork (F = 3.854, p < 0.05), particularly between the LN70 and LN65 at 4–34% (p = 0.0083, t* = 5.9). The incremental stiffness also affected the SS footwork during 42–82% (F = 3.574, p < 0.05), with specific differences observed between LN55 and LN65 at 70–86% (p = 0.0083, t* = 3.494), LN55 and LN70 at 14–48% (p = 0.0083, t* = 4.157), LN60 and LN65 at 55–74% (p = 0.0083, t* = 3.103), and LN65-LN70 at 18–57% (p = 0.0083, t* = 3.497).
Figure 7. Factorial SnPM statistics of subtalar inversion (+) - eversion (-) angles during LF, RF, CS, and SS footwork with badminton shoes of incremental lateral wedge hardness (stiffness). Notes: highlighted regions of the significant cluster in blue, and bicolor transverse bar of significance between two conditions with the upper color larger than the lower.
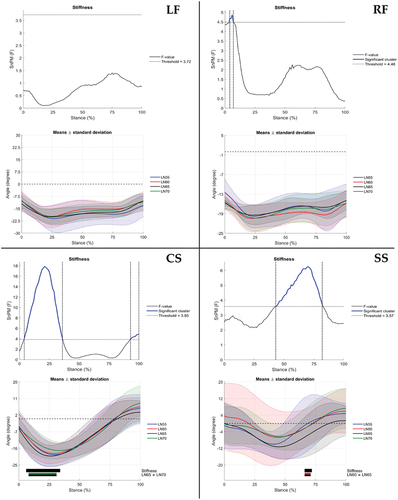
As illustrated in , the difference in the subtalar joint moments was noted in the four typical badminton footwork, specifically in the LF step, the incremental stiffness of the lateral wedge showed a difference at 45–85% (F = 5.22, p < 0.05), and LN55 was smaller than LN70 during 90–94% (p = 0.0083, t* = 5.5). The RF step showed a difference over the stance, during 1–5%, 5–11%, and 18–85% (F = 5.4, p < 0.05). Subtalar moment of LN55 was smaller than LN60 (p = 0.0083, t* = 4.62) at 1–5% and 19–83%, smaller than LN65 (p = 0.0083, t* = 4.76) at 8–13% and 23–70%, and smaller than LN70 (p = 0.0083, t* = 5.56) at 1–3%, 22–33%, and 42–81%. As for the CS footwork, the incremental stiffness showed effect during 4–38% and 64–80% (F = 4.39, p < 0.05), with specific dose–response of a larger subtalar moment with LN55 than LN70 at 9–36% (p = 0.0083, t* = 5.08), LN60 was greater than LN70 at 9–20% (p = 0.0083, t* = 5.02), and LN65 was greater than LN70 at 10–27% (p = 0.0083, t* = 4.28). The incremental stiffness had influence during 4–6%, 11–48%, and 97–100% of stance in the SS footwork (F = 5.69, p < 0.05). Difference between LN55 and LN65 was observed at 0–2%, 4–11%, and 14–20% (p = 0.0083, t* = 4.69), between LN55 and LN70 at 15–18% and 26–44% (p = 0.0083, t* = 5.23), between LN60 and LN70 at 15–45% (p = 0.0083, t* = 5.17), and between LN65 and LN70 at 4–6%, 8–55%, and 97–100% (p = 0.0083, t* = 5.47).
Figure 8. Factorial SnPM statistics of the subtalar inversion (+) – eversion (-) moment during LF, RF, CS, and SS footwork with badminton shoes of incremental lateral wedge hardness (stiffness). Notes: highlighted regions of the significant cluster in blue, and bicolor transverse bar of significance between two conditions with the upper color larger than the lower.
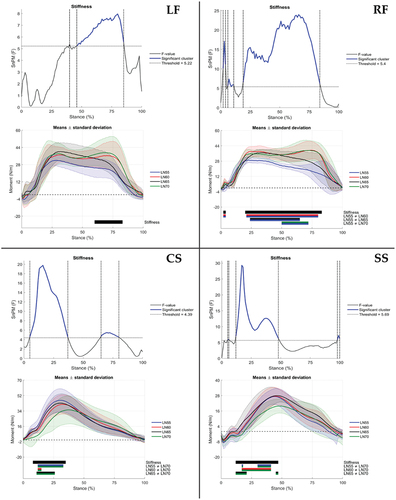
Principal component analysis (PCA)
A PCA modelling was conducted to reduce the dimensionality of the joint motion (angles) and loading (moments) during typical badminton footwork with badminton footwear of incremental lateral wedge hardness. The mean angles and moments in waveforms, PC scores and variations of PC2 and PC3 were included in the Supplementary Materials (3). As observed from , the main variances of ankle angles were observed in the LF (88.07%) and RF (76.78%) during stance with the upper limit over the lower limit as the increased wedge hardness. While key variations in the CS (85.45%) and SS (84.94%) footwork showed similarity during the early half (10–50%) of the stance with a lower limit over the upper limit, and the later (50–100%) stance showed opposite.
Figure 9. PCA modelling of ankle angles during LF, RF, CS, and SS footwork, with bar graphs highlighting the percentage explanation and accumulation, and contribution of the upper limit (‘+’) and lower limit (‘▽’) against the mean angle waveforms.
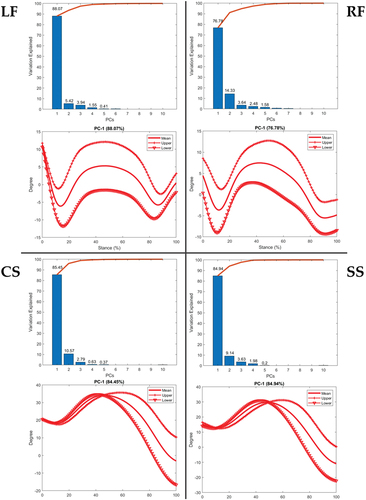
The main modes (PC1) of variances in ankle moments explained 62.16% (LF), 61.98% (RF), 77.44% (CS), and 83.28% (SS) with increased wedge hardness (). Specifically, the wedge hardness affected the ankle moments across the stance of the LF step with the upper limit over the lower limit, while the RF step showed the variance during 30–100% of the stance with the upper over the lower limit. Similarities of the upper over the lower limit were observed in the CS and SS footwork during 20–100% and 25–100%, respectively.
Figure 10. PCA modelling of ankle moments during LF, RF, CS, and SS footwork, with bar graphs highlighting the percentage explanation and accumulation, and contribution of the upper limit (‘+’) and lower limit (‘▽’) against the mean moment waveforms.
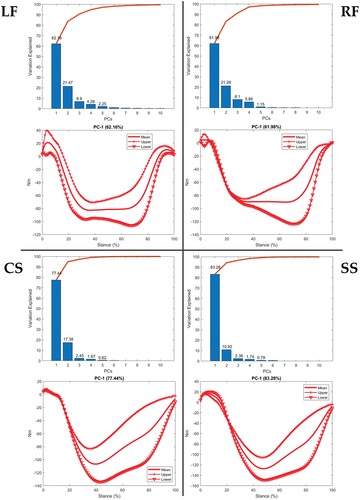
As for the subtalar angles (), the similarity across the four typical footwork (LF, RF, CS, and SS) was noted that the primary component (PC1) explained 79.93% (LF), 86.1% (RF), 93.09% (CS), and 76.44% (SS), showing the upper limit over the lower limit as a response to increased lateral wedge hardness. While observing the subtalar moments (), the PC1 accounted for 70.87% (LF), 60.92% (RF), 69.08% (CS), and 61.82% (SS) of variations. LF step had the upper limit over the lower limit across the stance, while RF had an upper limit over the lower limit during 20–100% of the stance. CS footwork had upper over the lower limit during 10–90%, while SS footwork had upper over lower limit during the entire stance.
Discussion
In the current study, badminton shoes with a novel lateral wedge design of incremental hardness (with a systematic increase from Asker C hardness of 55, 60, 65, and 70) were developed and tested while performing typical badminton footwork, which was aimed to improve footwork stability, agility, and performance. As the findings indicated, decreased contact times during the stance of the LF and RF lunges and CS and SS footwork were observed, while increased joint stiffness in the ankle complex was reported. These may be predictors of improved footwork performance. A further dose–response effect was quantified and reported via factorial SnPM statistical analysis, and certain instances of joint kinematics and kinetics were found, such as subtalar joint during initial landing and driving-off of CS and SS footwork, and mid-stance of RF and LF steps. The PCA modelling reported key components of variances as a response to the increased wedge hardness during specific instances over stance, such as variations of ankle angles across the stance of RF and LF steps but variances of subtalar angles in the later stance of CS and SS footwork. All these biomechanical parameters combined suggested a certain response to the incremental dose of lateral wedge hardness in badminton shoes.
Understanding the dose–response influence of sports footwear or orthotics on sports performance is challenging as the variant factors are difficult to control and standardise. Due to the manufacturing complexity and difficulty, previous studies mainly investigated the dose effect of orthotic insoles, which were relatively easier to fabricate. Several example of investigating the biomechanical response of flatfoot, pronated foot (rearfoot inversion or eversion), and knee osteoarthritis during walking or running gait were conducted to test the efficacy (Costa et al., Citation2021; Telfer et al., Citation2013; Zhang et al., Citation2022). Considering the inclined wedge design in orthotics, the realignment of the kinetic chain in the foot-ankle complex and lower extremity was obtained, thus showing promise in altering biomechanical performance. In terms of sports footwear, the novel design with a systematic increase of material hardness in the badminton shoes is the first study to investigate the dose–response effect, without changing wedge structure, geometry, and kinetic alignment. The quantification of discrete parameters and time-varying parameters as biomechanical responses to the systematic dose was explicitly reported.
Footwork performance in badminton is often referred as the ability to execute steps with high agility, such as the ability to lunge and return to starting position or even the next motion (Cronin et al., Citation2003). Considering the consistent approaching velocity with literature (T. L.-W. Chen et al., Citation2022; Lam et al., Citation2018; Valldecabres et al., Citation2018), the stance time was taken to quantify the improved performance. Consequently, a maximal reduction of contact times was observed as hardness increased during the footwork of LF by 8.9%, RF by 7.9%, CS by 12.5% and SS by 13.5% while using LN70 compared to LN55 as example. The non-significant joint ROM across the different footwork and badminton footwear conditions suggested that agility and flexibility were not affected by the systematic increase of lateral wedge hardness. The joint stiffness was another evidence of improved joint stability as the systematic increase of wedge hardness (Jang et al., Citation2021), especially the significantly increased ankle and subtalar joint stiffness during the SS (side-step) footwork. Although no significance in the ROM was observed, the increased joint moments from SS footwork may enhance the joint stiffness. The execution of SS footwork in this study was similar to the cutting manoeuvres, which was documented with a relatively high ratio of ankle inversion sprain (Bagehorn et al., Citation2023; Herbaut & Delannoy, Citation2020). The increased stability in the foot-ankle complex benefiting from the incremental wedge hardness may also be a predictor of improved footwork performance, due to the reduced deformation of the midsole to prevent forefoot inversion and assist torsional support (Graf & Stefanyshyn, Citation2012). Considering the torsional stiffness, the increased hardness in the lateral wedge might change the property of torsional stiffness in the experimental footwear, which was not evaluated in this study. This may be due to that all participants reported no significance in the perceived torsion from the VAS, and they had similar athletic capability. However, while looking at the joint stiffness of the 1st and 3rd quartile, especially under the LN65 and LN70 conditions, several athletes showed no response to the increased wedge hardness, suggesting the existence of respondent and non-respondent participants in the athletes. Whilst, the torsional properties were not provided in this study, it was proposed that future research may investigate the influence of providing appropriate footwear properties (i.e., torsional stiffness) based on the athletic and physical capability of each individual on athletic performance and injury risks.
To further investigate the dose–response effect from the systematic increase of lateral wedge hardness, we employed a popular statistical analysis approach of factorial SnPM (Trama et al., Citation2021) and PCA (Yu, Mei et al., Citation2021) to quantify the difference and features in the time-varying parameters during stance. The ankle kinematics of LF and RF footwork showed no significance, suggesting that joint ROM and agility were not influenced and thus not limited by the wedge hardness. Differences were observed while conducting CS and SS footwork, especially during initial landing and driving-off instances (Kuntze et al., Citation2010). While considering the landing patterns from lateral heel shifting to lateral forefoot, the differences were easily explained with increased wedge hardness in the lateral aspect of the midsole. The drive-off performance was improved with the stability in the ankle joint from the facilitated lateral “wall” of badminton shoes with a novel design in this study. The ankle plantarflexion moment improved greatly during the drive-off phase as well in both LF and RF steps, suggesting that the systematically increased wedge hardness may assist the returning of lunge towards the starting position on the court to execute the next movement (Lee & Loh, Citation2019; Mei et al., Citation2017; Yu & Mohamad, Citation2022).
The subtalar joint, as an extension of the shank, played an important role in aligning the kinetic chain of the lower extremity, even the trunk and torso (centre of mass) (Huang et al., Citation2014; Lin et al., Citation2015). Particularly, a less subtalar eversion found in the LN70 may suggest more stable support during the initial landing with a lateral wedge in the CS footwork, which was observed during the push-off of SS footwork with lateral support, similar to the cutting manoeuvres (Davis et al., Citation2009; Graf & Stefanyshyn, Citation2013). Another important finding of the subtalar moment during the drive-off of LF and RF steps should be noted, which may be explained by the above-mentioned plantarflexion moment. These changes may facilitate a rapid return to the starting position for the next movement and suggest improved footwork agility as responding to the systematically incremental wedge hardness. Smaller subtalar moments as observed with badminton shoes of greater wedge hardness during the CS and SS footwork, especially in the early half stance, may suggest fewer supination-pronation motions during landing and increased anti-torsional stiffness (Graf & Stefanyshyn, Citation2013; Stacoff et al., Citation1989).
Apart from the statistical inference and significance with the badminton shoes of incremental wedge hardness, the PCA modelling typically reduced the high dimensionality data, extracted, and reconstructed the key variances (Brandon et al., Citation2013), especially the angles and moments during stance. As observed, a systematic increase of the wedge hardness altered the magnitude (upper against lower limits) of ankle dorsi and plantar flexion angles and moments during LF and RF steps, apart from main variances during the RF driving-off return. These variances of ankle plantar flexion moments were also similar in the CS and SS footwork (angles shifted between lower and upper limits during drive-off), which suggested that the increased wedge hardness may assist the output of ankle plantar flexors moment to reduce the stance time for next execution and improve the footwork agility. Similar patterns of variances across stance were found in the subtalar angles but key variations of moments were observed during drive-off in LF and RF steps and initial till midstance of CS and SS footwork. Knowledge of the reconstructed principal component modes over the stance highlighted incremental angle and moment responses to the systematic increase of wedge dose, which was employed to understand other biomechanical scenarios of force impact profiles (Yu, Mei et al., Citation2021), technique features (Navandar et al., Citation2022), fatigue influence (Bruce et al., Citation2017), and injuries mechanism (O’connor & Bottum, Citation2009).
Several limitations should be considered before acknowledging the findings of this study. Firstly, the acute response from an incremental dose of wedge hardness was investigated using musculoskeletal modelling. Badminton athletes may adapt to certain footwear conditions while executing footwork, and the muscular activation in the lower extremity, particularly the shank, was not analysed, which may reveal further response. Secondly, this study of badminton footwork was conducted and executed in a lab-simulated badminton court, without mimicking the real-scenario badminton competition with randomised movements and potential fatigue effect. Lastly, the modification in the lateral wedge might change the torsional stiffness of the footwear, which was not quantified in the current study. Noting that female players were not evaluated and the sex difference in athletic performance, both their biomechanics and demand for footwear material properties may be different. Future studies shall consider investigating the mechanical patterns of female athletes and design sex-specific footwear.
Conclusion
This study developed a novel badminton shoe with a systematic increase of lateral wedge hardness in the midsole. To our knowledge, it was the first study to investigate the dose–response effect on typical badminton footwork with quantification of discrete parameters (contact time and joint stiffness) and time-varying angles and moments during stance. Increased footwork agility and joint stability were found as the wedge increased, suggesting improved footwork performance. Further explicit differences and variances, such as initial landing and driving-off phases, may add up knowledge on the mechanism of contribution from incremental wedge hardness. The specific design and biomechanical profiles being documented and reported in this study may provide implications for future footwear to fulfil the functional demand of court sports.
Supplemental Material
Download Zip (9.4 MB)Acknowledgments
Authors would like to thank all badminton athletes for participating in this study. We appreciate the support of badminton footwear with the systematic design of incremental wedge hardness from Li Ning (China) Sports Goods Company Limited.
Disclosure statement
No potential conflict of interest was reported by the author(s).
Data availability statement
The contributing data in the current study are available from our project repository (doi: 10.17608/k6.auckland.22638745; doi: 10.17608/k6.auckland.22638760).
Supplementary material
Supplemental data for this article can be accessed online https://doi.org/10.1080/02640414.2023.2257513.
Additional information
Funding
References
- Alder, D. B., Broadbent, D. P., Stead, J., & Poolton, J. (2019). The impact of physiological load on anticipation skills in badminton: From testing to training. Journal of Sports Sciences, 37(16), 1816–1823. https://doi.org/10.1080/02640414.2019.1596051
- Bagehorn, T., Lysdal, F. G., Jakobsen, L., de Zee, M., & Kersting, U. G. (2023). Medio-lateral and lateral edge friction in indoor sports shoes. Footwear Science, 15(1), 17–27. https://doi.org/10.1080/19424280.2022.2127921
- Brandon, S. C. E., Graham, R. B., Almosnino, S., Sadler, E. M., Stevenson, J. M., & Deluzio, K. J. (2013). Interpreting principal components in biomechanics: Representative extremes and single component reconstruction. Journal of Electromyography and Kinesiology, 23(6), 1304–1310. https://doi.org/10.1016/j.jelekin.2013.09.010
- Bruce, O., Moull, K., & Fischer, S. (2017). Principal components analysis to characterise fatigue-related changes in technique: Application to double under jump rope. Journal of Sports Sciences, 35(13), 1300–1309. https://doi.org/10.1080/02640414.2016.1221523
- Chen, C.-H., Condello, G., Chen, W.-H., Liu, T.-H., Tessitore, A., Shiang, T.-Y., & Liu, C. (2022). Effects of a novel inclined-adaptive footwear on change-of-direction performance in male athletes. Gait & Posture, 94, 189–194. https://doi.org/10.1016/j.gaitpost.2022.03.010
- Chen, T. L.-W., Wang, Y., Wong, D. W.-C., Lam, W.-K., & Zhang, M. (2022). Joint contact force and movement deceleration among badminton forward lunges: A musculoskeletal modelling study. Sports Biomechanics, 21(10), 1249–1261. https://doi.org/10.1080/14763141.2020.1749720
- Costa, B. L., Magalhães, F. A., Araújo, V. L., Richards, J., Vieira, F. M., Souza, T. R., & Trede, R. (2021). Is there a dose-response of medial wedge insoles on lower limb biomechanics in people with pronated feet during walking and running? Gait & Posture, 90, 190–196. https://doi.org/10.1016/j.gaitpost.2021.09.163
- Cronin, J., McNair, P. J., & Marshall, R. N. (2003). Lunge performance and its determinants. Journal of Sports Sciences, 21(1), 49–57. https://doi.org/10.1080/0264041031000070958
- Davis, E. M., Landry, S. C., & Nigg, B. M. (2009). Torsion of the foot in low cut basketball shoes in four cutting movements. Footwear Science, 1(sup1), 65–67. https://doi.org/10.1080/19424280902977335
- Faul, F., Erdfelder, E., Lang, A.-G., & Buchner, A. (2007). G*power 3: A flexible statistical power analysis program for the social, behavioral, and biomedical sciences. Behavior Research Methods, 39(2), 175–191. https://doi.org/10.3758/BF03193146
- Graf, E. S., & Stefanyshyn, D. (2013). The effect of footwear torsional stiffness on lower extremity kinematics and kinetics during lateral cutting movements. Footwear Science, 5(2), 101–109. https://doi.org/10.1080/19424280.2013.789561
- Graf, E. S., & Stefanyshyn, D. J. (2012). The shifting of the torsion axis of the foot during the stance phase of lateral cutting movements. Journal of Biomechanics, 45(15), 2680–2683. https://doi.org/10.1016/j.jbiomech.2012.08.025
- Herbaut, A., & Delannoy, J. (2020). Fatigue increases ankle sprain risk in badminton players: A biomechanical study. Journal of Sports Sciences, 38(13), 1560–1565. https://doi.org/10.1080/02640414.2020.1748337
- Hong, Y., Lam, W. K., Wang, S., & Cheung, J. T. M. (2016). Changes in comfort perception and direction change performance of badminton shoes with extensive usage time. Footwear Science, 8(1), 13–17. https://doi.org/10.1080/19424280.2015.1130753
- Huang, M.-T., Lee, H.-H., Lin, C.-F., Tsai, Y.-J., & Liao, J.-C. (2014). How does knee pain affect trunk and knee motion during badminton forehand lunges? Journal of Sports Sciences, 32(7), 690–700. https://doi.org/10.1080/02640414.2013.848998
- Jang, J., Song, K., & Wikstrom, E. A. (2021). Dynamic joint stiffness of the ankle in chronic ankle instability patients. Gait and Posture, 86, 199–204. https://doi.org/10.1016/j.gaitpost.2021.03.021
- Kuntze, G., Mansfield, N., & Sellers, W. (2010). A biomechanical analysis of common lunge tasks in badminton. Journal of Sports Sciences, 28(2), 183–191. https://doi.org/10.1080/02640410903428533
- Lam, W.-K., Wong, D. W.-C., & Lee, W.-C.-C. (2020). Biomechanics of lower limb in badminton lunge: A systematic scoping review. PeerJ, 8, e10300. https://doi.org/10.7717/peerj.10300
- Lam, W. K., Ding, R., & Qu, Y. (2017). Ground reaction forces and knee kinetics during single and repeated badminton lunges. Journal of Sports Sciences, 35(6), 587–592. https://doi.org/10.1080/02640414.2016.1180420
- Lam, W. K., Lee, K. K., Park, S. K., Ryue, J., Yoon, S. H., Ryu, J., & Li, Y. (2018). Understanding the impact loading characteristics of a badminton lunge among badminton players. PLoS ONE, 13(10), e205800. https://doi.org/10.1371/journal.pone.0205800
- Lam, W. K., Ryue, J., Lee, K. K., Park, S. K., Cheung, J. T. M., Ryu, J., & Padulo, J. (2017). Does shoe heel design influence ground reaction forces and knee moments during maximum lunges in elite and intermediate badminton players? PLoS ONE, 12(3), e0174604. https://doi.org/10.1371/journal.pone.0174604
- Lee, J. J. J., & Loh, W. P. (2019). A state-of-the-art review on badminton lunge attributes. Computers in Biology and Medicine, 108, 213–222. https://doi.org/10.1016/j.compbiomed.2019.04.003
- Lin, C.-F., Hua, S.-H., Huang, M.-T., Lee, H.-H., & Liao, J.-C. (2015). Biomechanical analysis of knee and trunk in badminton players with and without knee pain during backhand diagonal lunges. Journal of Sports Sciences, 33(14), 1429–1439. https://doi.org/10.1080/02640414.2014.990492
- Manrique, D. C., & González-Badillo, J. J. (2003). Analysis of the characteristics of competitive badminton. British Journal of Sports Medicine, 37(1), 62–66. https://doi.org/10.1136/bjsm.37.1.62
- Martin, C., Touzard, P., Horvais, N., Puchaud, P., Kulpa, R., Bideau, B., & Sorel, A. (2023). Influence of shoe torsional stiffness on foot and ankle biomechanics during tennis forehand strokes. European Journal of Sport Science, 23(6), 914–924. https://doi.org/10.1080/17461391.2022.2153232
- Mei, Q., Graham, M., & Gu, Y. (2014). Biomechanical analysis of the plantar and upper pressure with different sports shoes. International Journal of Biomedical Engineering and Technology, 14(3), 181–191. https://doi.org/10.1504/IJBET.2014.059668
- Mei, Q., Gu, Y., Fu, F., & Fernandez, J. (2017). A biomechanical investigation of right-forward lunging step among badminton players. Journal of Sports Sciences, 35(5), 457–462. https://doi.org/10.1080/02640414.2016.1172723
- Mei, Q., Gu, Y., Sun, D., & Fernandez, J. (2018). How foot morphology changes influence shoe comfort and plantar pressure before and after long distance running? Acta of Bioengineering and Biomechanics, 20(2), 179–186. https://doi.org/10.5277/ABB-01112-2018-02
- Mei, Q., Gu, Y., Xiang, L., Baker, J. S., & Fernandez, J. (2019). Foot pronation contributes to altered lower extremity loading after long distance running. Frontiers in Physiology, 10(May), 573. https://doi.org/10.3389/fphys.2019.00573
- Mei, Q., Xiang, L., Li, J., Fernandez, J., & Gu, Y. (2021). Analysis of running ground reaction forces using the one-dimensional statistical Parametric Mapping (SPM1d). Journal of Medical Biomechanics, 36(5), 684–691. https://doi.org/10.16156/j.1004-7220.2021.05.004
- Mei, Q., Zhang, Y., Li, J., & Rong, M. (2014). Different sole hardness for badminton movement. Journal of Chemical and Pharmaceutical Research, 6(6), 632–634.
- Mündermann, A., Nigg, B. M., Stefanyshyn, D. J., & Humble, R. N. (2002). Development of a reliable method to assess footwear comfort during running. Gait and Posture, 16(1), 38–45. https://doi.org/10.1016/S0966-6362(01)00197-7
- Navandar, A., Kipp, K., & Navarro, E. (2022). Hip and knee joint angle patterns and kicking velocity in female and male professional soccer players: A principal component analysis of waveforms approach. Journal of Sports Sciences, 40(17), 1919–1930. https://doi.org/10.1080/02640414.2022.2121022
- O’connor, K. M., & Bottum, M. C. (2009). Differences in cutting knee mechanics based on principal components analysis. Medicine and Science in Sports and Exercise, 41(4), 867–878. https://doi.org/10.1249/MSS.0b013e31818f8743
- Oja, P., Kelly, P., Pedisic, Z., Titze, S., Bauman, A., Foster, C., Hamer, M., Hillsdon, M., & Stamatakis, E. (2017). Associations of specific types of sports and exercise with all-cause and cardiovascular-disease mortality: A cohort study of 80 306 British adults. British Journal of Sports Medicine, 51(10), 812–817. https://doi.org/10.1136/bjsports-2016-096822
- Ooi, C. H., Tan, A., Ahmad, A., Kwong, K. W., Sompong, R., Ghazali, K. A. M., Liew, S. L., Chai, W. J., & Thompson, M. W. (2009). Physiological characteristics of elite and sub-elite badminton players. Journal of Sports Sciences, 27(14), 1591–1599. https://doi.org/10.1080/02640410903352907
- Pataky, T. C. (2010). Generalized n-dimensional biomechanical field analysis using statistical parametric mapping. Journal of Biomechanics, 43(10), 1976–1982. https://doi.org/10.1016/j.jbiomech.2010.03.008
- Phomsoupha, M., & Laffaye, G. (2015). The Science of badminton: Game characteristics, anthropometry, physiology, visual fitness and Biomechanics. Sports Medicine, 45(4), 473–495. https://doi.org/10.1007/s40279-014-0287-2
- Rajagopal, A., Dembia, C. L., DeMers, M. S., Delp, D. D., Hicks, J. L., & Delp, S. L. (2016). Full-body musculoskeletal model for muscle-driven simulation of human Gait. IEEE Transactions on Biomedical Engineering, 63(10), 2068–2079. https://doi.org/10.1109/TBME.2016.2586891
- Ramsay, J. O., & Silverman, B. W. (2005). Functional data analysis 2nd edition. Springer New York. https://doi.org/10.1007/B98888
- Reinschmidt, C., & Nigg, B. M. (2000). Current issues in the design of running and court shoes. Sportverletzung · Sportschaden, 14(3), 72–81. https://doi.org/10.1055/s-2000-7866
- Shen, S., Lam, W.-K., Teng, J., Jia, S.-W., Baker, J. S., Ugbolue, U. C., Fekete, G., & Gu, Y. (2022). Gender and leg-dominance differences in shoe properties and foot injuries in badminton: A cross-sectional survey. Journal of Foot and Ankle Research, 15(1), 26. https://doi.org/10.1186/s13047-022-00531-w
- Stacoff, A., Kaelin, X., Stuessi, E., & Segesser, B. (1989). The torsion of the foot in running. International Journal of Sport Biomechanics, 5(4), 375–389. https://doi.org/10.1123/ijsb.5.4.375
- Telfer, S., Abbott, M., Steultjens, M. P. M., & Woodburn, J. (2013). Dose–response effects of customised foot orthoses on lower limb kinematics and kinetics in pronated foot type. Journal of Biomechanics, 46(9), 1489–1495. https://doi.org/10.1016/j.jbiomech.2013.03.036
- Tik-Pui Fong, D., Hong, Y., & Xian Li, J. (2007). Cushioning and lateral stability functions of cloth sport shoes. Sports Biomechanics, 6(3), 407–417. https://doi.org/10.1080/14763140701491476
- Trama, R., Hautier, C., & Blache, Y. (2021). fctSnpm: Factorial ANOVA and post-hoc tests for statistical nonParametric Mapping in MATLAB. Journal of Open Source Software, 6(63), 3159. https://doi.org/10.21105/joss.03159
- Trama, R., Hautier, C., Blache, Y., Bertucci, W., Chiementin, X., & Hintzy, F. (2023). Intra-cycle analysis of muscle vibration during cycling. Sports Biomechanics, 22(4), 554–566. https://doi.org/10.1080/14763141.2022.2083010
- Valldecabres, R., Casal, C. A., Chiminazzo, J. G. C., & de Benito, A. M. (2020). Players’ on-court movements and contextual variables in badminton World Championship. Frontiers in Psychology, 11, 1567. https://doi.org/10.3389/fpsyg.2020.01567
- Valldecabres, R., De Benito, A. M., Littler, G., & Richards, J. (2018). An exploration of the effect of proprioceptive knee bracing on biomechanics during a badminton lunge to the net, and the implications to injury mechanisms. PeerJ, 6, e6033. https://doi.org/10.7717/peerj.6033
- Valldecabres, R., Richards, J., & de Benito, A.-M. (2020). The effect of match fatigue in elite badminton players using plantar pressure measurements and the implications to injury mechanisms. Sports Biomechanics, 00, 1–18. https://doi.org/10.1080/14763141.2020.1712469
- Warmenhoven, J., Bargary, N., Liebl, D., Harrison, A., Robinson, M., Gunning, E., & Hooker, G. (2021). PCA of waveforms and functional PCA: A primer for Biomechanics. Journal of Biomechanics, 116, 110106. https://doi.org/10.1016/j.jbiomech.2020.110106
- Wei, Y., Liu, Y., Tian, M., & Fu, W. (2009). Effects of different footwear on the metatarsophalangeal joint during push-off in criticial badminton footwork. Journal of Medical and Biological Engineering, 29(4), 172–176.
- Yu, L., Mei, Q., Mohamad, N. I., Gu, Y., & Fernandez, J. (2021). An exploratory investigation of patellofemoral joint loadings during directional lunges in badminton. Computers in Biology and Medicine, 132(5), 104302. https://doi.org/10.1016/j.compbiomed.2021.104302
- Yu, L., Mei, Q., Xiang, L., Liu, W., Mohamad, N. I., István, B., Fernandez, J., & Gu, Y. (2021). Principal component analysis of the running ground reaction forces with different speeds. Frontiers in Bioengineering and Biotechnology, 9, 629809. https://doi.org/10.3389/fbioe.2021.629809
- Yu, L., & Mohamad, N. I. (2022). Development of badminton-specific footwork training from traditional physical exercise to novel intervention approaches. Physical Activity and Health, 6(1), 219–225. https://doi.org/10.5334/paah.207
- Zhang, X., Lam, W.-K., & Vanwanseele, B. (2022). Dose-response effects of forefoot and arch orthotic components on the center of pressure trajectory during running in pronated feet. Gait & Posture, 92, 212–217. https://doi.org/10.1016/j.gaitpost.2021.11.033
- Zifchock, R., Sulley, M., Helton, G., Freisinger, G., Wilson, R., Blackmon, W., & Goss, D. (2017). Quantification of torsional stiffness in running footwear: Proposed methodology. Footwear Science, 9(3), 121–126. https://doi.org/10.1080/19424280.2017.1342703