ABSTRACT
This study examined the immediate effects of multidirectional elastic tape (MET) on passive ankle joint torque in healthy adults. A randomised crossover trial evaluated four tape conditions (no-tape-NT, low-tension-LT, medium-tension-MT, and high-tension-HT) at two angular speeds on peak dorsiflexion torque, low- (stiffness 1) and high-torque stiffness (stiffness 2), area under the loading curve (AUC) and hysteresis. Twenty-two adults completed the study (17 females; mean (SD): age 26.0 (6.9) years, height 1.7 (0.1) m, body mass 71.1 (20.2) kg. There was no significant condition-by-speed interaction for any ankle torque variable. There was a significant main effect of condition on peak dorsiflexion torque, stiffness 1, and AUC, but not stiffness 2 or hysteresis. Post-hoc tests revealed that peak dorsiflexion torque, stiffness 1 and AUC were significantly lower in the NT condition, compared to the three taped conditions, and between the LT and HT conditions, though the effect sizes were considered small. MET applied with increasing levels of pre-tension, led to a small and incremental increase in stretch resistance and elastic energy stored (range 5.5% to 12.5%) during passive ankle dorsiflexion. Importantly, effect sizes were small and may not translate to measurable improvements in muscle-tendon unit performance during dynamic exercise.
1 Introduction
Therapeutic tape is used to manage and reduce the risk of musculoskeletal injury. There are two broad types of therapeutic tape – rigid tape and elastic tape. Rigid tape is characterised by near-linear material properties (Siegler et al., Citation2011) that does not stretch, and is therefore used to restrict joint motion and deload underlying soft tissues (Hug et al., Citation2014; Obst et al., Citation2019). In contrast, elastic tape is characterised by non-linear (hyperelastic) mechanical properties with distinct low- and high-force stiffness values, like that of tendons and ligaments (Boonkerd & Limroongreungrat, Citation2016; Siegler et al., Citation2011). Because of these differences, the application of elastic tape over muscles, tendons, and joints has been purported to augment the mechanical properties of the underlying muscle and/or joint through changes in passive stiffness and hysteresis (McNeill & Pedersen, Citation2016). To this end, elastic tape is typically applied over the target muscle-tendon unit with the muscle in a shortened position, such that tape begins to tension as the target muscle-tendon is lengthened, thereby contributing to positive muscle work by converting of potential elastic energy to kinetic energy during tape recoil (McNeill & Pedersen, Citation2016).
In vivo, the passive mechanical properties of a joint or muscle-tendon unit (MTU) can be assessed using passive loading and unloading torque – angle relations (Magnusson, Citation1998) for the estimation of maximal passive torque and angle, energy stored, and stiffness (Nordez et al., Citation2006). The capacity for elastic tape applied over the skin to change the passive mechanical properties of a joint or underlying muscle likely varies according to the mechanical properties of the tape itself, which differ between commercial brands (Boonkerd & Limroongreungrat, Citation2016; Cheatham & Baker, Citation2021; Matheus et al., Citation2017), as well as the method (e.g., pre-tension), amount (e.g., length and layers), and location of the tape. For example, maximal stretch values reported by manufacturers vary from 140% for Kinesio® tape, 180% for Rocktape®, and greater than 200% for Dynamic tape® and GripIt Active®. Furthermore, most elastic tapes (e.g., Kinesio® tape, Rocktape®) are designed to stretch longitudinally (i.e., unidirectional elastic tape), whereas more recent products (e.g., Dynamic tape® and GripIt Active®) stretch both longitudinally and transversely (i.e., multidirectional elastic tape – MET) which may provide additional mechanical benefits during multiplanar and multi-joint movements, and when underlying muscle dimensions change in response to stretch and/or contraction.
Several studies have examined the effects of commercial MET in healthy (Bittencourt et al., Citation2017; Silva et al., Citation2021) and injured populations (Alahmari et al., Citation2020; Hill et al., Citation2022; Robinson et al., Citation2019). In healthy female adults, Silva et al., (Citation2021) found that MET had no effect on gluteus medius activation and did not improve functional performance during a triple hop and 6-m timed hop test. In contrast, MET decreased the frontal knee projection angle by approximately 5 deg during the single leg squat in female volley players (Bittencourt et al., Citation2017). In individuals with chronic lower back pain, MET provided short-term improvements in trunk extensor endurance, though no concomitant changes were found for pain, disability, mobility, or kinesiophobia (Alahmari et al., Citation2020). In individuals with greater trochanteric pain syndrome, MET reduced pain as well as the hip adduction moment, internal rotation, and pelvic drop during walking (Robinson et al., Citation2019). Finally, in people with lateral elbow tendinopathy MET resulted in a small reduction is muscle activity of selected forearm extensor muscles during gripping, compared to no-tape (Hill et al., Citation2022).
While these studies provide some evidence for a possible neurophysiological and/or biomechanical effect of MET during dynamic and complex multi-joint movements, only one study examined the effect of elastic tape on the passive mechanical properties of a joint (Siegler et al., Citation2011). This study showed that a custom hyperelastic tape applied over the lateral ankle reduced the post-exercise increase in ankle flexibility, compared to rigid tape, and therefore may provide greater protection to the ankle (Siegler et al., Citation2011). Unfortunately, because the study did not include a pre-exercise no-tape condition, it is not known, whether the elastic tape inherently changed the passive mechanical properties of the ankle joint. Furthermore, manufacturers of elastic tape recommend that elastic tape be stretched sufficiently to “take up the slack” in the tape during application. This is highly subjective as the amount of stretch applied will differ between practitioners, and even within the same practitioner at different time points. Differences in the amount of pre-stretch may therefore influence the biomechanical effect of the elastic tape. Finally, because elastic tape is considered viscoelastic, its effect on joint biomechanics may be dependent on the speed of angular displacement (Siegler et al., Citation2011).
It is therefore not clear whether the application of MET across a joint can change the passive mechanical properties of that joint, including low- and high-force torque stiffness, hysteresis, and area under the loading curve (AUC). Such information would complement existing material testing of elastic tape (Boonkerd & Limroongreungrat, Citation2016; Cheatham & Baker, Citation2021; Matheus et al., Citation2017), and provide important mechanistic evidence for the proposed biomechanical effects of MET, as suggested by the manufacturers (McNeill & Pedersen, Citation2016). This study, therefore, aimed to investigate the effect of MET applied over the posterior lower leg at three levels of tension (high, medium, and low) on the passive mechanical properties of the ankle joint assessed during dorsiflexion-plantar flexion at two joint velocities (slow and fast). We hypothesised that MET would increase the resistance to passive ankle dorsiflexion and joint stiffness, so that for a given angular displacement more energy would be stored during the MET condition(s) compared to the no-tape condition. We also hypothesised that such changes would be dependent on the amount of tension applied to the MET and, based on the viscoelastic behaviour of the ankle joint and the MET, the speed at which the ankle was passively moved.
2 Materials and methods
2.1 Study design
A randomised repeated measures design was used to examine the effect of MET stretched to 105% (LT), 120% (MT) and 135% (HT) of its resting length, compared to no tape (NT), on the passive mechanical properties of the ankle joint measured at two angular velocities – slow (5 deg/sec) and fast (20 deg/sec). Each participant attended a 1.5-h session. All testing was completed on the right ankle. A random sequence generator (https://www.random.org/sequences/) was used to assign the order of each tape condition and speed of passive movement.
2.2 Participants
Twenty-two healthy, recreationally active volunteers (17 females; mean (SD): age 26.0 (6.9) years, height 1.7 (0.1) m, mass 71.1 (20.2) kg, BMI 23.9 (5.4) kg/m2) participated. Participants were excluded if they had experienced pain in the past 6 months that required them to see a healthcare professional or had a history of significant injury to the right lower limb or lower back. Participants were also excluded if they reported neurological, metabolic, or systemic conditions, or skin allergies to adhesives. All participants provided written informed consent, and all procedures were approved by CQUniversity Human Research Ethics Committee (#2021–021).
2.3 Tape conditions
An experienced physiotherapist (LJH) trained in the application of MET applied each tape condition using two layers of 7.5 cm wide ActiveTape® V2 (StrapIt Sports Medical Supplies Pty Ltd., Victoria, Australia) composed of technical nylon fabric, waterproofing agents, and adhesive. Prior to application, the leg was shaved to improve tape adhesion and the participant was positioned prone with their right knee extended (0 deg) and right ankle maximally plantar flexed. Two strips of tape were placed over the posterior calf-ankle-foot, such that ends of the tape (~10 cm) were applied without tension to ensure adhesion, minimise the risk of skin traction injuries, and reduce tape lift-off. The distal end was applied over the sole of the foot three quarters the distance from the head of first metatarsal to the heel () and the proximal end was applied from the mid-shank (). The distance between the end of the distal end to the middle of the posterior aspect of the shank was divided by 1.05, 1.20, and 1.35 to determine the respective tape lengths for the LT, MT, and HT conditions (). One additional strip of tape was applied horizontally over the Achilles tendon (from medial to lateral malleolus) to minimise tape bowstringing during the passive motion trials ().
2.4 Isokinetic dynamometry
Participants were positioned supine with their knee in full extension (0 deg) and approximately half of their right tibia off the end of the plinth. The lateral malleolus was aligned with the axis of the isokinetic dynamometer (Biodex Medical Systems, Inc. NY, USA) (Muir et al., Citation1999). The foot was secured to the ankle attachment via two adjustable restraints. A goniometer was used to locate the participants’ neutral ankle position (shank-foot angle = 90 deg), recorded as 0 deg on the Biodex. Joint torque data were reported relative to this position (i.e., plantar flexion torque = negative, dorsiflexion torque = positive). Where possible, the range of motion limits were set to 50 deg plantar flexion (i.e., −50 deg) and 20 deg dorsiflexion (i.e., 20 deg). Individuals unable to reach these limits were assigned limits based on their maximal stretch tolerance determined by passively rotating their ankle. Prior to the experimental trials the ankle joint was pre-conditioned using 10 passive plantar flexion-dorsiflexion cycles (NT) at an angular velocity of 5 deg/s (Salsich et al., Citation2000). For the experimental trials 10 passive ankle rotations were completed at each speed, with a 2-min rest between sets. A 5-min washout period was allowed between each tape condition.
Joint torque (Nm), position (deg), and angular speed (deg/sec) were recorded throughout each trial at 2000 Hz. Surface electromyography (EMG) was recorded for the medial gastrocnemius, lateral gastrocnemius, and tibialis anterior according to SENIAM guidelines (Hermens et al., Citation1999). Prior to electrode (Trigno Sensors, Delsys Inc., Natick, MA, USA) application, the skin was shaved, abraded, and cleaned with an alcohol wipe. EMG data were sampled at 1111 Hz via the Trigno Base Station (ADInstruments, Dunedin, NZ). The raw EMG signals were monitored during the passive movements to ensure no active muscle contraction occurred. Trials with clear evidence of muscle contraction were discarded and then re-collected. All data were synchronised and recorded using LabChart (Version 8.1.13, ADInstruments, Dunedin, New Zealand).
2.5 Data analysis
All data were analysed using MatLab (R2020a, MathWorks, Inc., Natick, MA, USA). Joint torque and position data were filtered using a 4th order Butterworth zero lag filter with 6 Hz cut-off. Filtered torque-angle data of each loading (plantar flexion to dorsiflexion) and unloading (dorsiflexion to plantar flexion) cycle were interpolated to 101 points and averaged over the 10 cycles for subsequent analysis. The following discrete measures were derived from the averaged torque-angle data: passive stiffness 1, passive stiffness 2, AUC, and hysteresis. Passive stiffness was defined according to the methods of Salsich et al (Salsich et al., Citation2000); where stiffness 1 represented the slope of the linear regression applied to the first half of the passive torque-angle curve (i.e., 50 deg plantar flexion to 15 deg plantar flexion), and stiffness 2 refers to the slope of the linear regression applied to the second half of the passive torque-angle curve (i.e., 15 deg plantar flexion to 20 deg dorsiflexion) (). Model fit was assessed using the coefficient of determination (R2) and root mean square error (RMSE): averaged over all trials: stiffness 1 [mean (SD) R2 = 0.9757 (0.0031) and RMSE = 0.2623 (0.0361)] and stiffness 2 [mean (SD) R2 = 0.9659 (0.0026) and RMSE = 0.4737 (0.0413)]. The AUC was computed as the integral of the torque-angle data for the loading phase and reflects the amount of energy stored during passive dorsiflexion (Nordez et al., Citation2009). Hysteresis was calculated as the relative difference (%) between the AUC for the loading and unloading cycles, and reflects the relative amount of energy lost or dissipated as heat during cyclic loading (Nordez et al., Citation2009).
Figure 2. Example trial torque-angle data (dorsiflexion = negative, plantar flexion =positive) for one participant (#1) showing the 10 loading (upper most) and unloading (lower most) curves. Stiffness 1 (solid line) represents the slope of the line of best fit for the initial 50% of the loading curve. Stiffness 2 (dashed line) represents the slope of the line of best fit for the final 50% of the loading curve.
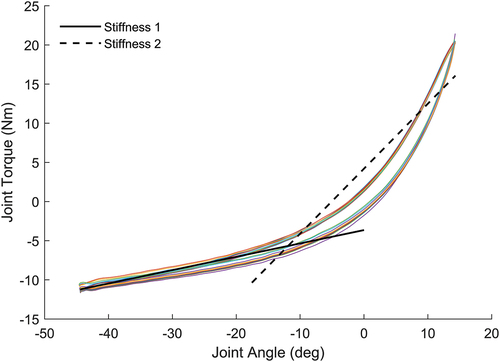
2.6 Statistical analysis
Statistical analysis of discrete measures was conducted using SPSS Version 26 (IBM, Armonk, NY, USA). All discrete measures met the assumptions of normality and homogeneity of variance assessed using the Shapiro – Wilk test and Levene’s test, respectively. A two-way repeated measures analysis of variance (ANOVA) was used with a factor of tape condition (i.e., NT, LT, MT, and HT) and a factor of joint speed (i.e., slow, and fast). The dependent variables included peak DF torque, stiffness 1, stiffness 2, AUC, and hysteresis. Where a significant condition-by-speed interaction or main effect of condition was found, appropriate post-hoc tests were undertaken using Bonferroni adjustments for multiple comparisons. Where a significant main effect of speed was found the omnibus results are reported, but no post-hoc comparisons were reported or discussed as we were only interested in the condition-by-speed interaction and main effect of condition. Interaction and main effects are reported using the F-statistic, p-value, and two effect size estimates (partial eta squared, ηp2 and generalised eta squared, ηg2), the latter of which can be compared against the partial eta benchmarks provided by Cohen (Cohen, Citation2013) (i.e., 0.01 = small, 0.06 = medium, 0.14 = large) (Lakens, Citation2013).
The effect of tape condition at slow and fast angular speed on the interpolated torque-data (0–100%) was conducted using a 1-D parametric statistical parametric mapping (SPM) ANOVA with repeated measures (Pataky, Citation2010). Where a significant main effect of condition was detected, a-priori planned pairwise comparisons were performed between NT and each of the taped conditions using paired t-tests with Bonferroni adjustments for multiple comparisons. SPM analyses were implemented in MATLAB using opensource SPM1D code (www.spm1d.org). Statistical significance for all tests was defined as α = 0.05.
3 Results
There were no adverse events associated with the application of tape at any tension. Group mean (SD) angular displacements ranged from −47.5 (3.5) deg to 19.0 (3.0) deg. Group means for the discrete measures of passive mechanical properties of the ankle joint are shown in . There was no significant condition-by-speed interaction (F3,60 = 0.714, p = 0.548, ηp2 = 0.034, ηg2 = 0.0026) or main effect of speed for peak DF torque (F1,20 = 0.138, p = 0.715, ηp2 = 0.007, ηg2 = 0.00003); however, there was a main effect of condition (F3,60 = 3.488, p = 0.021, ηp2 = 0.149, ηg2 = 0.0087). Post-hoc tests show that peak DF torque was higher in the HT compared to LT (p = 0.01), with no other significant pairwise comparisons (). There was no significant condition-by-speed interaction (F3,60 = 0.287, p = 0.835, ηp2 = 0.014, ηg2 = 0.00013) or main effect of speed for stiffness 1 (F1,20 = 4.21, p = 0.054, ηp2 = 0.174, ηg2 = 0.0027); however, there was a main effect of condition (F3,60 = 18.743, p < 0.001, ηp2 = 0.484, ηg2 = 0.014). Post-hoc tests show that stiffness 1 was higher in all taped conditions, compared to NT, and higher in HT compared to LT (). There was no significant condition-by-speed interaction (F3,60 = 1.360, p = 0.264, ηp2 = 0.064, ηg2 = 0.0002) or main effect of condition for stiffness 2 (F3,60 = 2.109, p = 0.109, ηp2 = 0.095, ηg2 = 0.0018) but there was a main effect of speed (F1,20 = 31.56, p < 0.001, ηp2 = 0.612, ηg2 = 0.005). There was no significant condition-by-speed interaction for AUC (F3,60 = 0.385, p = 0.764, ηp2 = 0.019, ηg2 = 0.00017); however, there was a main effect of condition (F3,60 = 13.380, p < 0.001, ηp2 = 0.401, ηg2 = 0.017) and speed (F1,20 = 8.04, p = 0.01, ηp2 = 0.287, ηg2 = 0.0029). Post-hoc tests show that AUC was higher in MT and HT compared to NT, and higher in HT compared to LT (). There was no significant condition-by-speed interaction (F3,60 = 0.998, p = 0.400, ηp2 = 0.048, ηg2 = 0.0019) or main effect of condition (F3,60 = 2.420, p = 0.075, ηp2 = 0.108, ηg2 = 0.0014) for hysteresis, though there was a main effect of speed (F1,20 = 41.94, p < 0.001, ηp2 = 0.677, ηg2 = 0.075).
Table 1. Group mean data for all passive mechanical properties of the ankle joint measured at two angular speeds.
Table 2. Post-hoc comparisons for the main effect of tape condition on the passive mechanical properties of the ankle joint.
The one-way repeated measures SPM ANOVA showed a significant main effect of tape condition on joint torque for the fast angular speed only (). Post-hoc planned pairwise comparisons show that ankle joint torque during the loading cycles was higher for each of the tape conditions compared to NT (). These differences were most evident for the HT condition, wherein, ankle joint torque was significantly higher across most (~70%) of the loading cycle. There were no differences during unloading between NT and LT, and NT and MT, and only a marginal difference between NT and HT over ~ 5% of unloading cycle.
Figure 3. Top panel - ensemble average with SD (shaded) for ankle torque measured during passive dorsiflexion (loading = 0–50%) and passive plantar flexion (unloading = 50–100%) at 20 deg/sec for each tape condition. Bottom panel – SPM one-way ANOVA results with horizontal dashed line depicting the critical threshold for significance (α = 0.05). Shaded regions represent a statistically significant effect of tape condition on passive ankle torque.
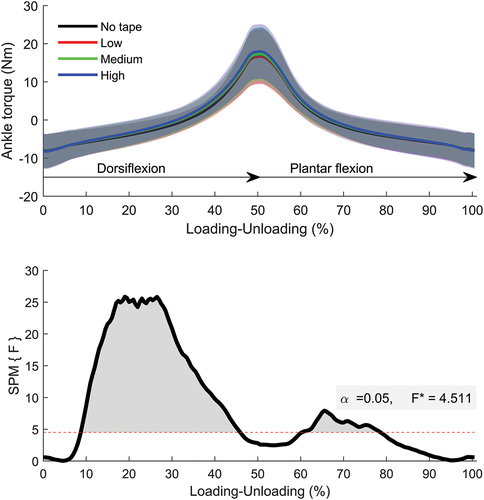
Figure 4. Ensemble average with SD (shaded) for ankle torque measured during passive dorsiflexion (loading = 0–50%) and passive plantar flexion (unloading = 50–100%) at 20 deg/sec. Each plot depicts the post-hoc paired t-test SPM analysis comparing the no-tape to each of the taped conditions (low tension – left, medium tension – middle, and high tension – right). Statistical differences with Bonferroni corrections (α = 0.02) are depicted by the shaded boxes.
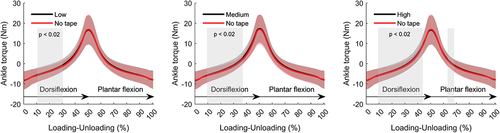
4 Discussion
This is the first study to investigate the effect of MET on the passive mechanical properties of the ankle joint. Our results suggest that MET applied over the posterior leg and foot alters the ankle torque–angle relationship during passive dorsiflexion; however, the effects were small, isolated to the low-torque region, and not affected by the speed of joint motion, and only marginally affected by the amount of pre-tension applied to the tape. MET increased stiffness 1, and AUC, as well as passive torque during loading compared to the no-tape condition. There was no effect of MET on passive torque during unloading and so although there was a small corresponding increase in hysteresis with increasing levels of tape tension this effect was not statistically significant. Together, our results suggest that MET may enable a small amount of additional elastic energy to be stored during passive ankle dorsiflexion; however, this energy is not fully recovered during passive plantar flexion and may be lost as heat.
4.1 Effect of MET on passive ankle torque
Consistent with our hypothesis, we found that MET increased the resistance to stretch as evidenced by an increase in passive dorsiflexion torque, stiffness 1 (low-torque stiffness), and AUC. Our results are broadly consistent with a previous study that reported lower “flexibility” (deg/Nm) of ankle inversion-eversion with the application of hyperelastic athletic taping, compared to no-tape (Siegler et al., Citation2011). In the current study, MET increased ankle torque during passive dorsiflexion over approximately 70% of the range of motion (i.e., from 35 deg plantar flexion to 15 deg dorsiflexion) and peaked between 40 deg and 20 deg plantarflexion corresponding to the lower region of the passive ankle torque-angle curve (Kato et al., Citation2005). Not surprisingly, stiffness 1, which represented the slope of lower torque region, increased with the application of MET. In contrast, there was no effect of MET on the high torque region (i.e., >10–12 Nm or > 10 deg dorsiflexion) or stiffness 2. Our results, therefore, suggest that the passive effects of MET on the ankle may be limited to the low torque region of the passive torque-angle curve, approximating the toe-region of the typical stress–strain curve for soft-tissues. Previous studies suggest the relative contribution of muscle to tendon elongation is greater within the lower passive torque region, compared to the higher passive torque region, whereby tendon elongation predominates (Blazevich et al., Citation2012; Kato et al., Citation2005). As such, MET may have the potential to influence muscle elongation during passive lengthening of the MTU but may have less influence on MTU lengthening at higher passive torques, wherein elongation of the tendon predominates. Nevertheless, without concomitant measures of muscle and/or tendon elongation, we can only speculate as to the potential influence MET may have on MTU elongation. Further, the magnitude of effect of MET was small, with an average increase in stiffness 1 ranging from 0.011 Nm/deg (NT vs LT @ 5 deg/sec) and 0.025 Nm/mm (NT vs HT @ 20 deg/sec), or 10% and 17%, respectively. These relative changes in low-torque stiffness are also lower than that reported by Siegler et al (Siegler et al., Citation2011) during passive ankle inversion, wherein, hyperelastic tape increased passive inversion stiffness by ~ 30%, compared to no-tape. While methodological differences between studies, including the direction of movement, tape material, and stiffness measurement, may explain the differences in the magnitude of effect of the tape, our results do suggest that MET can increase the passive stiffness of the ankle (albeit at very low loads), which may provide some protection to the ankle during low-load dynamic activities (Siegler et al., Citation2011).
The AUC reflects the amount of energy stored during passive loading whereas, hysteresis reflects the proportion of that stored energy dissipated as heat during passive unloading (Nordez et al., Citation2009). Our data show that MET increased AUC but had no effect on hysteresis, suggesting that during passive loading MET allows for more energy to be stored; however, the amount of energy lost during passive unloading as heat remained mostly unchanged. Whether the small increase in stored energy with MET could be recovered during a subsequent muscle contraction (i.e., stretch shorten contraction) is not known. Nevertheless, the amount of additional elastic energy stored during passive stretch was small (range = 5.5% − 12.5%) and may not be sufficient to change muscle energetics or work output during active stretch shorten contractions. While no studies have examined the effect of elastic tape on joint work during active stretch shorten cycle contractions or tasks, there is some evidence that elastic tape (e.g., KinesiotapeTM) can increase peak concentric and eccentric joint torque in healthy (Fratocchi et al., Citation2013; Yeung & Yeung, Citation2016; Yeung et al., Citation2015) and osteoarthritic populations (Anandkumar et al., Citation2014). These changes in joint torque could reflect enhanced rate of force development (Huang et al., Citation2011), independent of changes in muscle activation or electromechanical delay (Hsieh et al., Citation2007; Williams et al., Citation2012; Yeung et al., Citation2015); however, such evidence is not conclusive (Chang et al., Citation2010; Fu et al., Citation2008; Williams et al., Citation2012). It is therefore possible that enhanced active torque output with the application of elastic tape could reflect the conversion of added elastic energy from the tape to kinetic energy during active muscle shortening. However, it should be noted that the effects of elastic tape on joint stiffness and AUC in the current study, and active torque production in other studies are very small (Williams et al., Citation2012), and any potential effects on performance or injury recovery should be considered with caution.
4.2 Effect of MET pre-tension on passive ankle torque
We found a significant main effect of tape condition on passive ankle dorsiflexion torque, stiffness 1, and AUC; however, this was mostly driven by differences between the no-tape and the taped conditions (LT, MT, and HT), rather than differences between the taped conditions, for which the only significant differences were between HT and LT. The SPM analysis of the torque-angle data also show that with increasing levels of tension the effect of MET was statistically significant over a larger region of the loading curve. Nevertheless, while not conclusive, our data provide some support that higher levels of tape pre-tension are marginally associated with higher levels of passive joint torque, stiffness 1, and AUC. It is possible that other forms or application techniques (e.g., layering) of elastic tape may provide more definitive results, and that the effect of pre-tension may be more evident during active conditions. However, the only other study to examine the effect of pre-tension on elastic tape (KinesiotapeTM) reported no effect of tape tension on active knee extension torque (Shakeri et al., Citation2017). Therefore, while passive torque, stiffness 1, and AUC, generally increased with higher levels of pre-tension, the effects were small and would likely have negligible effect on dynamic ankle function.
4.3 Effect of speed on passive ankle torque
There was no significant speed-by-condition interaction for any of the discrete measures of passive ankle torque. Given that elastic tape, including the MET used in the current study (ActiveTape® V2), is considered viscoelastic (Matheus et al., Citation2017; Siegler et al., Citation2011), we expected the resistance to passive ankle dorsiflexion would be dependent on both angular speed (i.e., rate dependence) and the level of pre-tension. While the mean differences between angular speeds for stiffness 1, stiffness 2, and AUC generally increased with higher tape tensions, the magnitude of these differences were too small and/or inconsistent to observe a speed-by-condition interaction for these discrete measures. However, our SPM analyses of the torque data between NT and each of the taped conditions were significant for the fast speed trials, but not the slow speed trials, providing some evidence that the effect of MET on passive ankle mechanics may be influenced by the speed of stretch. It is possible that the inclusion of additional and/or higher angular speeds and/or the use tape layering could have improved the likelihood of observing a significant speed-by-condition interaction effect for the discrete measures of passive ankle torque. Nevertheless, based on the current study there is no clear and consistent evidence that the application of MET over the posterior calf and foot alters the passive rate-dependent properties of the ankle joint.
4.4 Methodological considerations
There are several methodological considerations when interpreting the results of this study. First, although we observed several statistically significant effects on tape condition on measures of passive ankle torque, the effect sizes were small (all ηg2 <0.02) limiting the strength of the conclusions. Second, we estimated low- and high-torque stiffness as the average slope of the first and second halves of the loading phase, respectively. While this method has been used previously (Salsich et al., Citation2000) it is possible that measurements of passive stiffness over different regions of the torque-angle curve may have resulted in different outcomes for this variable. However, we analysed the entire torque-angle curve using SPM statistics and these results suggest that the effects of MET on passive ankle torque may be reduced when torques exceed 10–12 Nm. Third, we only investigated the effect of MET on passive mechanical properties of the ankle at two angular velocities and a single knee position. Further studies are needed to investigate the effect of MET on active torque production and muscle activation patterns at higher angular velocities and during cyclic stretch shorten contractions, consistent with dynamic exercise. Fourth, we chose to apply the MET according to the manufacturers guidelines and using a double layering method. It is possible that the application of different tape configurations, including the use of multi-layering (3 or more layers), may result in different outcomes. Finally, we included young adults with no current or previous history of pain or injury, and so are results may not be generalisable to other populations, with neuromuscular musculoskeletal injury and/or impairments for which MET may be used clinically.
5 Conclusion
The application of MET over the posterior calf and foot, applied according to the manufacturer’s guidelines, altered the passive mechanical properties of the ankle during passive dorsiflexion, as evidenced by an increase in low-torque stiffness and area under the loading curve. These effects were independent of the speed of passive stretch. While MET appears to increase the amount of elastic energy stored during passive ankle dorsiflexion, it is unclear if this energy would be recovered during active muscle contraction. Future studies are required to examine whether the effects of MET on the passive mechanical properties of the ankle translate to measurable changes in active muscle-tendon function.
Supplementary Material_Table 1.docx
Download MS Word (14 KB)Acknowledgments
We acknowledge StrapIt Sports Medical Supplies for supplying the tape used in the study.
Disclosure statement
No potential conflict of interest was reported by the authors.
Supplementary material
Supplemental data for this article can be accessed online https://doi.org/10.1080/02640414.2023.2283291
Additional information
Funding
References
- Alahmari, K. A., Rengaramanujam, K., Reddy, R. S., Samuel, P. S., Tedla, J. S., Kakaraparthi, V. N., Ahmad I. (2020). The immediate and short-term effects of dynamic taping on pain, endurance, disability, mobility and kinesiophobia in individuals with chronic non-specific low back pain: A randomized controlled trial. PLoS One, 15(9), e0239505–e. https://doi.org/10.1371/journal.pone.0239505
- Anandkumar, S., Sudarshan, S., & Nagpal, P. (2014). Efficacy of kinesio taping on isokinetic quadriceps torque in knee osteoarthritis: A double blinded randomized controlled study. Physiotherapy Theory and Practice, 30(6), 375–383. https://doi.org/10.3109/09593985.2014.896963
- Bittencourt, N., Leite, M., Zuin, A., Pereira, M., Gonçalves, G., & Signoretti, S. (2017). Dynamic taping and high frontal plane knee projection angle in female volleyball athletes. British Journal of Sports Medicine, 51(4), 297. https://doi.org/10.1136/bjsports-2016-097372.36
- Blazevich, A. J., Cannavan, D., Waugh, C. M., Fath, F., Miller, S. C., & Kay, A. D. (2012). Neuromuscular factors influencing the maximum stretch limit of the human plantar flexors. Journal of Applied Physiology: Respiratory, Environmental and Exercise Physiology, 113(9), 1446–1455. https://doi.org/10.1152/japplphysiol.00882.2012
- Boonkerd, C., & Limroongreungrat, W. (2016). Elastic therapeutic tape: Do they have the same material properties? Journal of Physical Therapy Science, 28(4), 1303–1306. https://doi.org/10.1589/jpts.28.1303
- Chang, H.-Y., Chou, K.-Y., Lin, J.-J., Lin, C.-F., & Wang, C.-H. (2010). Immediate effect of forearm Kinesio taping on maximal grip strength and force sense in healthy collegiate athletes. Physical Therapy in Sport, 11(4), 122–127. https://doi.org/10.1016/j.ptsp.2010.06.007
- Cheatham, S. W., & Baker, R. (2021). A clinically relevant method of quantifying the mechanical properties of RockTape® kinesiology tape at different elongation lengths. Journal of Sport Rehabilitation, 30(1), 173–176. https://doi.org/10.1123/jsr.2019-0261
- Cohen, J. (2013). Statistical power analysis for the behavioral sciences. Routledge.
- Fratocchi, G., DiMattia, F., Rossi, R., Mangone, M., Santilli, V., & Paoloni, M. (2013). Influence of Kinesio taping applied over biceps brachii on isokinetic elbow peak torque. A placebo controlled study in a population of young healthy subjects. Journal of Science & Medicine in Sport / Sports Medicine Australia, 16(3), 245–249. https://doi.org/10.1016/j.jsams.2012.06.003
- Fu, T.-C., Wong, A. M., Pei, Y.-C., Wu, K. P., Chou, S.-W., & Lin, Y.-C. (2008). Effect of Kinesio taping on muscle strength in athletes—a pilot study. Journal of Science & Medicine in Sport / Sports Medicine Australia, 11(2), 198–201. https://doi.org/10.1016/j.jsams.2007.02.011
- Hermens, H. J., Merletti, R., & Freriks, B. (1999). SENIAM : European recommendations for surface electromyography. Roessingh Research and Development.
- Hill, C. E., Heales, L. J., Stanton, R., Holmes, M. W. R., & Kean, C. O. (2022). Effects of multidirectional elastic tape on forearm muscle activity and wrist extension during submaximal gripping in individuals with lateral elbow tendinopathy: A randomised crossover trial. Clin Biomech (Bristol, Avon), 100, 105810. https://doi.org/10.1016/j.clinbiomech.2022.105810
- Hsieh, T., Wu, P., Liao, J., Kuo, T., Wu, T., Huang, C., Chang, SK, Chai, H, Su, FC. (2007). Does elastic taping on the triceps surae facilitate the ability of vertical jump? Journal of Biomechanics, 40, S412. https://doi.org/10.1016/S0021-9290(07)70407-4
- Huang, C.-Y., Hsieh, T.-H., Lu, S.-C., & Su, F.-C. (2011). Effect of the Kinesio tape to muscle activity and vertical jump performance in healthy inactive people. Biomedical Engineering Online, 10(1), 70. https://doi.org/10.1186/1475-925X-10-70
- Hug, F., Ouellette, A., Vicenzino, B., Hodges, P. W., & Tucker, K. (2014). Deloading tape reduces muscle stress at rest and during contraction. Medicine & Science in Sports & Exercise, 46(12), 2317–2325. https://doi.org/10.1249/MSS.0000000000000363
- Kato, E., Oda, T., Chino, K., Kurihara, T., Nagayoshi, T., Fukunaga, T., & Kawakami, Y. (2005). Musculotendinous factors influencing difference in ankle joint flexibility between women and men. International Journal of Sport and Health Science, 3(Special_Issue_2005), 218–225. https://doi.org/10.5432/ijshs.3.218
- Lakens, D. (2013). Calculating and reporting effect sizes to facilitate cumulative science: a practical primer for t-tests and ANOVAs. Frontiers in Psychology, 4, 4. https://doi.org/10.3389/fpsyg.2013.00863
- Magnusson, S. P. (1998). Passive properties of human skeletal muscle during stretch maneuvers. Scandinavian Journal of Medicine & Science in Sports, 8(2), 65–77. https://doi.org/10.1111/j.1600-0838.1998.tb00171.x
- Matheus, J. P. C., Zille, R. R., Gomide Matheus, L. B., Lemos, T. V., Carregaro, R. L., & Shimano, A. C. (2017). Comparison of the mechanical properties of therapeutic elastic tapes used in sports and clinical practice. Physical Therapy in Sport, 24, 74–78. https://doi.org/10.1016/j.ptsp.2016.08.014
- McNeill, W., & Pedersen, C. (2016). Dynamic tape. Is it all about controlling load? Journal of Bodywork and Movement Therapies, 20(1), 179–188. https://doi.org/10.1016/j.jbmt.2015.12.009
- Muir, I. W., Chesworth, B. M., & Vandervoort, A. A. (1999). Effect of a static calf-stretching exercise on the resistive torque during passive ankle dorsiflexion in healthy subjects. The Journal of Orthopaedic and Sports Physical Therapy, 29(2), 106–115. https://doi.org/10.2519/jospt.1999.29.2.106
- Nordez, A., Casari, P., Mariot, J. P., & Cornu, C. (2009). Modeling of the passive mechanical properties of the musculo-articular complex: Acute effects of cyclic and static stretching. Journal of Biomechanics, 42(6), 767–773. https://doi.org/10.1016/j.jbiomech.2008.12.019
- Nordez, A., Cornu, C., & McNair, P. (2006). Acute effects of static stretching on passive stiffness of the hamstring muscles calculated using different mathematical models. Clinical Biomechanics, 21(7), 755–760. https://doi.org/10.1016/j.clinbiomech.2006.03.005
- Obst, S., Heales, L., Hrelja, Z., Ishri, P., Wesche, J., & Barber, L. (2019). The effect of deloading tape on medial gastrocnemius muscle fascicle behaviour during dynamic exercise. Journal of Sports Sciences, 37(16), 1874–1883. https://doi.org/10.1080/02640414.2019.1600225
- Pataky, T. C. (2010). Generalized n-dimensional biomechanical field analysis using statistical parametric mapping. Journal of Biomechanics, 43(10), 1976–1982. https://doi.org/10.1016/j.jbiomech.2010.03.008
- Robinson, N. A., Spratford, W., Welvaert, M., Gaida, J., & Fearon, A. M. (2019). Does dynamic tape change the walking biomechanics of women with greater trochanteric pain syndrome? A blinded randomised controlled crossover trial. Gait & Posture, 70, 275–283. https://doi.org/10.1016/j.gaitpost.2019.02.031
- Salsich, G. B., Mueller, M. J., & Sahrmann, S. A. (2000). Passive ankle stiffness in subjects with diabetes and peripheral neuropathy versus an age-matched comparison group. Physical Therapy, 80(4), 352–362. https://doi.org/10.1093/ptj/80.4.352
- Shakeri, S., Khademi-Kalantari, K., & Akbarzade Baghban, A. (2017). Effect of the level of tension and the extent of coverage of Kinesio tape on the knee extensor torque in healthy young people. The Scientific Journal of Rehabilitation Medicine, 6(4), 123–131.
- Siegler, S., Marchetto, P., DJM, I. V., & Gadikota, H. R. (2011). A composite athletic tape with hyperelastic material properties improves and maintains ankle support during exercise. Journal of Orthopaedic & Sports Physical Therapy, 41(12), 961–968. https://doi.org/10.2519/jospt.2011.3476
- Siegler, S., Marchetto, P., Murphy, I. D., & Gadikota, H. R. (2011). A composite athletic tape with hyperelastic material properties improves and maintains ankle support during exercise. The Journal of Orthopaedic and Sports Physical Therapy, 41(12), 961–968. https://doi.org/10.2519/jospt.2011.3476
- Silva, R. O., Carlos, F. R., Morales, M. C., Emerick, V., Teruyu, A. I., & Valadão, V. M. A., Carvalho, LC, Lobato, DF. (2021). Effect of two dynamic tape™ applications on the electromyographic activity of the gluteus medius and functional performance in women: A randomized, controlled, clinical trial. Journal of Bodywork and Movement Therapies, 25, 212–217. https://doi.org/10.1016/j.jbmt.2020.11.018
- Williams, S., Whatman, C., Hume, P. A., & Sheerin, K. (2012). Kinesio taping in treatment and prevention of sports injuries. Sports Medicine, 42(2), 153–164. https://doi.org/10.2165/11594960-000000000-00000
- Yeung, S. S., & Yeung, E. W. (2016). Acute effects of Kinesio taping on knee extensor peak torque and stretch reflex in healthy adults. Medicine (Baltimore), 95(4), e2615. https://doi.org/10.1097/MD.0000000000002615
- Yeung, S. S., Yeung, E. W., Sakunkaruna, Y., Mingsoongnern, S., Hung, W. Y., Fan, Y. L., Iao, HC. (2015). Acute effects of Kinesio taping on knee extensor peak torque and electromyographic activity after exhaustive isometric knee extension in healthy young adults. Clinical Journal of Sport Medicine: Official Journal of the Canadian Academy of Sport Medicine, 25(3), 284–290. https://doi.org/10.1097/JSM.0000000000000132