ABSTRACT
We present a case study detailing cognitive performance, functional neuroimaging, and effects of a hypothesis-driven treatment in a 10-year-old girl diagnosed with complete, isolated corpus callosum agenesis. Despite having average overall intellectual abilities, the girl exhibited profound surface dyslexia and dysgraphia. Spelling treatment significantly and persistently improved her spelling of trained irregular words, and this improvement generalized to reading accuracy and speed of trained words. Diffusion weighted imaging revealed strengthened intrahemispheric white matter connectivity of the left temporal cortex after treatment and identified interhemispheric connectivity between the occipital lobes, likely facilitated by a pathway crossing the midline via the posterior commissure. This case underlines the corpus callosum’s critical role in lexical reading and writing. It demonstrates that spelling treatment may enhance interhemisphericconnectivity in corpus callosum agenesis through alternative pathways, boosting the development of a more efficient functional organization of the visual word form area within the left temporo-occipital cortex.
1. Introduction
Dual-route models of written language processing enable us to specify damaged and preserved reading and writing processes in patients with written language disorders (Coltheart et al., Citation2001; Perry et al., Citation2010). These models distinguish between two independent but interactive procedures or “routes” for word processing. The distinction lies in how phonology is translated into orthography and vice versa. The nonlexical route of reading is thought to process regular words and nonwords by converting graphemes into their associated phonemes to produce a phonological output. In this context, orthographically regular words with a predictable relationship between graphemes and phonemes can be read or written correctly by applying analytic mechanisms to convert graphemes into phonemes and vice versa. Hence, in nonlexical spelling, phonemes are converted into their corresponding graphemes, irrespective of possible irregularities. By employing learned mappings between sounds and letters, nonlexical reading and writing enable correct outputs for regular words (e.g., LEG) and nonwords, but may result in regularization errors for inconsistent irregular words (e.g., YACHT spelled YOT and read as YATCHED). In contrast, the lexical reading and writing route (analogous to a dictionary look up procedure) should identify all familiar words, regardless of irregularity, by activating their stored representation in the orthographic and phonological lexicons. This activates the corresponding whole-word, retrieved directly from the lexicons. While the lexical route is believed to expedite the reading and writing process, nonlexical reading and writing can support the processing of every word, even unknown ones, especially when the lexical route is damaged and even when the orthography is transparent (consistent mapping between graphemes-phonemes, and vice versa; Folk & Rapp, Citation2004; Provazza et al., Citation2022; Rapp et al., Citation2002).
Dual-route models were originally conceived to explain the patterns of impaired reading and writing resulting from brain injury (Marshall & Newcombe, Citation1973). Two patient groups have important theoretical relevance here, since they demonstrate a double dissociation in impairment and sparing patterns, providing evidence for the existence of independent lexical and nonlexical routes susceptible to independent damage. Patients with phonological dyslexia and/or dysgraphia demonstrate a striking dissociation of performance captured by poor reading and/or writing of nonwords whilst retaining the ability to process words, consistent with a selective impairment to the nonlexical route. Conversely, patients with surface dyslexia and/or dysgraphia demonstrate the reverse dissociation pattern captured by a selective difficulty in reading and/or writing of irregular words with preservation of nonwords, reflecting an impairment to the lexical route. These patients often make regularization errors.
Whilst most of the surface dyslexic patients described in the literature involve adult stroke patients, representing acquired cases, there are a few case studies documenting developmental surface dyslexia (Broom & Doctor, Citation1995; Castles, Citation1996; Castles & Coltheart, Citation1996; Coltheart et al., Citation1983; Friedmann & Lukov, Citation2008; Gvion & Friedmann, Citation2016; Judica et al., Citation2002; Kohnen et al., Citation2018; Lukov et al., Citation2014; Manis et al., Citation1996; McArthur et al., Citation2013; Spinelli et al., Citation1997; Wybrow & Hanley, Citation2015; Zoccolotti et al., Citation1999) and dysgraphia (Brunsdon et al., Citation2005; Hanley & Sotiropoulos, Citation2018; Hepner et al., Citation2017; Sotiropoulos & Hanley, Citation2017), including some cases in languages with nonalphabetic scripts such as Chinese and Japanese (Ho et al., Citation2007; Wang & Yang, Citation2014). The double dissociations in impairment and sparing, respectively, of lexical and nonlexical routes observed in patients with phonological versus surface dyslexia/dysgraphia support the distinction between the two reading and writing procedures, indicating selectivity of impairment not only in skilled readers and writers, but also in the development of lexical and nonlexical procedures (Castles, Citation1996).
Neuroimaging studies indicate that nonlexical and lexical processes also rely on partially distinct brain areas. Lexical processes are associated with a ventral pathway in the left occipito-temporal cortex (Cohen & Dehaene, Citation2004; Dehaene & Cohen, Citation2011; Graves et al., Citation2010; Hoffman et al., Citation2015; Ludersdorfer et al., Citation2015; Taylor et al., Citation2013). This area, also known as the visual word form area (VWFA), responds preferentially to words and pseudowords relative to random letter strings or other objects and is believed to be involved in mapping orthographic information to phonological whole word representations (Joubert et al., Citation2004; Kubota et al., Citation2019; Planton et al., Citation2019). Lesion studies have revealed that damage to the left occipito-temporal cortex results in visual word recognition deficits (Norton et al., Citation2015), which may extend to non-orthographic visual tasks such as pattern discrimination, object, and face processing as observed in lesion (Roberts et al., Citation2013; Roberts et al., Citation2015) and developmental (Giofrè et al., Citation2019; Provazza et al., Citation2019) studies across orthographic depth (Provazza et al., Citation2022). Similarly, neuroimaging studies on the neural substrates for spelling have identified the left occipito-temporal cortex to be involved in the retrieval of orthographic whole-word representations (Ludersdorfer et al., Citation2015; Rapp & Dufor, Citation2011), while lesion-deficit correlation studies have associated lesions within the left lingual, fusiform, and parahippocampal gyri with lateral extension to the inferior temporal gyrus with surface dysgraphia (Purcell et al., Citation2014; Rapcsak & Beeson, Citation2004; Rapp et al., Citation2016; Tsapkini & Rapp, Citation2010). Nonlexical processes, on the other hand, seem to involve a dorsal pathway within the left perisylvian cortex including the inferior frontal gyrus, operculum, precentral gyrus, insula, superior temporal gyrus, and supramarginal gyrus (Alexander et al., Citation1992; Henry et al., Citation2007; Rapcsak et al., Citation2009; Roeltgen et al., Citation1983). These areas are critical for mapping graphemes to phonemes and vice versa, and also provide the neural substrate of phonological short-term memory (DeMarco et al., Citation2017; Henry et al., Citation2007; Hickok & Poeppel, Citation2007; Joubert et al., Citation2004).
The cause/s of dyslexia have been the subject of scientific debate for many years. Following the discovery of reduced phonological awareness in many dyslexic children (Bradley & Bryant, Citation1978; Snowling, Citation1981), researchers suggested that the core deficit of developmental dyslexia is a specific impairment in the representation, storage and/or retrieval of speech sounds (Reed, Citation1989; Vellutino et al., Citation2000; Werker & Tees, Citation1987). However, alternative theories have emerged, such as the magnocellular theory that posits a visual processing deficit (Bosse et al., Citation2007; Valdois et al., Citation2004), and the rapid auditory processing theory, which suggests a reduced perception of rapidly varying sounds (Tallal, Citation1980; Tallal et al., Citation1993).
We hypothesize that reduced communication between hemispheres can significantly impact reading and spelling abilities, leading to slowed reading and potentially underdeveloped lexical and sublexical routes. This theory, known as the interhemispheric deficit theory (Badzakova-Trajkov et al., Citation2005; Bradshaw et al., Citation2020; Monaghan & Shillcock, Citation2008), emphasizes the important role of the corpus callosum in reading and writing. The corpus callosum, the major interhemispheric white matter tract, contributes to executive functions, psychomotor speed, and higher language functions (Hinkley et al., Citation2016). Moreover, it is involved in the neural organization and hemispheric specialization of cognition (Friederici & Alter, Citation2004). In a recent functional magnetic resonance imaging (fMRI) study in healthy children, we described the integrative function of the corpus callosum within the language network, allowing improved interhemispheric functional connectivity and enhanced language abilities (Bartha-Doering et al., Citation2021a).
In typically developing children, reading is lateralized to the left hemisphere, where networks of occipito-temporal areas support the storage and retrieval of written forms in the VWFA (Dehaene & Dehaene-Lambertz, Citation2016; Strother et al., Citation2017). However, the right hemisphere also contributes to reading, albeit to a lesser extent. Initially, it has access to both orthographic and phonological information during word recognition and can store orthographic forms for high-frequency nouns (Behrmann & Plaut, Citation2020; Halderman, Citation2011; Iacoboni & Zaidel, Citation1996). Information from the right hemisphere is transmitted through the splenium, the most posterior portion of the corpus callosum, to the VWFA (Binder & Mohr, Citation1992; Molko et al., Citation2002). Thus, reading engages both hemispheres, and interhemispheric interaction plays an important role in the process (Artuvan Korkmaz et al., Citation2022).
Interaction between hemispheres during written word recognition has been studied using visual half-field paradigms. In typically developed individuals, recognition of words presented in the right visual field is faster and more accurate than of words presented in the left visual field (Bradshaw et al., Citation2020; Hellige, Citation1993). This recognition advantage is thought to reflect the additional processing costs associated with transferring information from a word presented to the left visual field in the right hemisphere and then via callosal connections to the left VWFA (Ellis, Citation2004; Zaidel et al., Citation1990). When identical word stimuli are presented bilaterally, recognition accuracy and speed increase, indicating cooperative interactions between the hemispheres (Hellige, Citation1993). This bilateral advantage effect has only been observed for words (Hasbrooke & Chiarello, Citation1998; Mohr & Pulvermuller, Citation2002), but not for unfamiliar nonwords (Hellige et al., Citation1989; Mohr et al., Citation2000; Mohr et al., Citation2007). A case report of a split-brain patient who does not demonstrate this effect underlines the important role of interhemispheric interaction through the corpus callosum for reading (Mohr et al., Citation1994).
In dyslexic children, visual half-field studies have shown abnormalities in the interhemispheric transfer of information, coordination, and timing (Bradshaw et al., Citation2020; Henderson et al., Citation2007; Kinsbourne, Citation2003; Paulesu et al., Citation1996; Vandermosten et al., Citation2012a; Vandermosten et al., Citation2012b; Žarić et al., Citation2017). Moreover, individuals with dyslexia often exhibit deficits in motor tasks which require interhemispheric transfer, such as tactile transfer tasks and bimanual coordination (Daini et al., Citation2018; Fabbro et al., Citation2001; Gross-Glenn & Rothenberg, Citation1984; Moore et al., Citation1995). These observations highlight the important role of the corpus callosum in reading. Interestingly, some studies reported abnormalities in the volume and size of the corpus callosum in dyslexic individuals (Duara et al., Citation1991; Hynd et al., Citation1995; Rumsey et al., Citation1996), while others failed to demonstrate any difference in callosal measures (Larsen et al., Citation1992; Pennington et al., Citation1999)
A patient group that could provide more insight on the role of the corpus callosum in reading and spelling are those with agenesis of the corpus callosum (ACC). ACC is a rare congenital brain malformation characterized by a partial or complete absence of the corpus callosum. Studies of patients with ACC frequently report a favourable cognitive outcome with overall intellectual abilities that fall within the normal to low average range (Moutard et al., Citation2012; Siffredi et al., Citation2013). However, detailed neuropsychological examinations often reveal deficits in higher-level cognitive functions, including slower reaction times and processing speed (Marco et al., Citation2012), abstract reasoning and problem solving (Hinkley et al., Citation2012), arithmetic (Siffredi et al., Citation2018), social cognition (Brown & Paul, Citation2000; Turk et al., Citation2010), and communicative abilities (Brown et al., Citation2005; Paul et al., Citation2003). Critically, written language abilities are rarely tested. Siffredi et al. (Citation2018) reported word reading and spelling in the low average range in a group of children with ACC, though detailed individual results were not provided. Temple et al. (Citation1990) described two children who showed phonological difficulties in reading.
We hypothesized that reduced communication between hemispheres has a significant impact on the development of reading and spelling abilities following ACC (Hypothesis 1). The results of cognitive examinations and neuroimaging findings generated a second hypothesis that was subsequently tested via an individualized, model-driven treatment study using behavioural and neuroimaging measures.
2. Case presentation
2.1. Case history
WR, a 10-year-old girl, was referred to the Neuropediatric Outpatient Unit of the Department of Pediatrics and Adolescent Medicine at the Medical University of Vienna in 2020. Her parents expressed concerns about ongoing difficulties in the acquisition of written language skills despite the absence of any notable oral language deficits. Additionally, a parental interview revealed attentional and learning difficulties in school, as well as challenges in acquiring bimanual coordination skills such as swimming and riding a bike. WR had a history of different therapies, including occupational therapy, speech therapy, and auditory processing and perception therapy. She attended an inclusive school.
Upon neurological examination, WR showed signs of dyspraxia, a developmental motor coordination deficit, and complete left-handedness (EHI—100; Oldfield, Citation1971). No hemianopsia was observed. Subsequent magnetic resonance imaging (MRI) revealed a complete, isolated ACC with typical colpocephaly, a disproportionate prominence of the occipital horns of the lateral ventricles ().
Figure 1. Magnetic resonance imaging reveals a complete, isolated agenesis of the corpus callosum with colpocephaly; neurological convention.
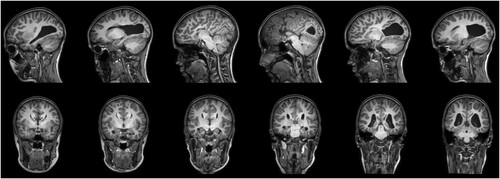
WR was first enrolled in an ongoing study on ACC (Bartha-Doering et al., Citation2021b). As part of this, fMRI was performed, revealing bilateral language localization during a semantic decision paradigm. Detailed information regarding the fMRI paradigm and the language localization pattern can be found in the Supplementary Information (see Note 1 and Supplementary Figure S1). Subsequently, ethical approval was obtained from the Ethics Committee of the Medical University of Vienna for the present study. Written, informed consent was obtained from a parent prior to WR’s inclusion in each study.
2.2. Cognitive background information
Comprehensive cognitive testing was performed over four testing sessions within a two-week period. Raw scores from standardized tests were transformed into age-adjusted z-scores. According to clinical conventions, z-scores ranging from −1 to +1 were considered average, scores above +1 as above-average, scores below −1 as below-average, and scores below −2 as reduced. Experimental tests were also administered to explore specific cognitive abilities in more detail. Results of the neuropsychological examination are displayed in .
Table 1. Cognitive background information.
The Wechsler Intelligence Scale for Children WISC-V (Wechsler, Citation2017) indicated overall average intellectual abilities. However, there was impairment in flexibility of thinking on a visuomotor sequencing test (Trail Making Test; Delis et al., Citation2001), and below-average fluid reasoning in a matrix reasoning test (WISC-V). Additionally, a computerized test of attentional performance (KiTAP; Zimmermann et al., Citation2002) revealed below average performance in alertness and go/nogo tasks, as well as impaired flexibility. The Digit span Test (WISC-V) showed average scores in verbal short-term and working memory. The Verbaler Lern- und Merkfähigkeitstest (Helmstaedter et al., Citation2001), the German equivalent of the Auditory Verbal Learning Test (Lezak, Citation1995), exhibited below-average learning efficiency of a list of words. Short- and long-term recall, were above average and significantly better than her own performance during word list learning, suggesting attentional rather than amnestic deficits. The analysis and mental manipulation of spatial forms, as tested by the WISC-V Block Design test and Visual Puzzles test, were within the average range. Furthermore, line orientation was normal. Copying a complex figure (Rey Osterrieth Complex Figure Test; Osterrieth, Citation1944) showed below-average visuoconstructive abilities and difficulties with planning and global level processing, with the patient adopting a part-by-part strategy and neglecting the overall “gestalt” of the figure. Immediate and delayed recall of the figure was below-average and average respectively, suggesting attentional problems with intact memory abilities. Further visuospatial tests revealed visuoperceptual deficits in specific domains: visual scanning (tested with the KiTAP) showed a lack of search strategy with a high number of missing targets; line bisection exhibited a mean 8.5% deviation towards the left side in each line, suggesting right visuospatial neglect.
2.3. Spoken language abilities
The neurolinguistic investigation of spoken language abilities indicated average to above-average abilities in auditory perception and production tests (). The Token Test for Children TTFC-2 (McGhee et al., Citation2007) revealed average comprehension of spoken instructions, and the Sprachstandserhebungstest SET 5–10 (Petermann, Citation2018) showed average auditory comprehension of words and sentences, and above-average comprehension of stories. Expressive vocabulary for nouns, words, and adverbs/adjectives was tested with the Wortschatz—und Wortfindungstest WWT (Glück, Citation2011) and the SET 5–10 and fell within the average range. Story telling abilities (SET 5-10) were found to be above-average. Syntactic abilities were measured with the Reception of Grammar Test TROG (Bishop, Citation2003) and the SET 5–10 and revealed above-average syntactic processing, singular/plural formation, and recognition of incorrect sentences. Phonemic memory span for vowels, syllables, words, and sentences, assessed with the Phonematischer Gedächtnistest PHOG (Gruner et al., Citation2013), was average. In addition, the Regensburger Wortflüssigkeitstest RWT (Aschenbrenner et al., Citation2001) revealed average semantic word fluency.
Table 2. Spoken language abilities.
3. Orthographic processing
The Lese- und Rechtschreibtest SLRT-II (Moll & Landerl, Citation2010) was administered for a standardized assessment of reading and spelling abilities. The reading subtest of the SLRT-II measures efficiency in automated, direct word recognition and letter-to-sound conversion within one minute, focusing on reading fluency and speed. WR’s scores were below—2 standard deviations, as she could only read six regular words per minute (five correctly) and seven pseudowords per minute due to a slow letter-by-letter reading strategy. In the spelling subtest of the SLRT-II, WR was presented with a written sentence missing a word, both visually and auditorily, and asked to fill in the blank by dictation. Whilst she correctly spelled the two words with regular orthographic forms, all 22 irregular words were spelled incorrectly. In the reading comprehension subtest of the Aachener Aphasietest (Huber et al., Citation1983), WR had to match written words with regular grapheme-phoneme correspondence to one of four pictures, respectively. Although her reading was very slow, her comprehension of the read words was flawless.
A more detailed evaluation of reading and spelling was performed using experimental tests. displays the results of these tests. We furthermore compared WR’s performance on these experimental tests to the performance of three typically developing girls, matched for age (control group age mean 9.67 years, SD 0.47, range 9-10) using the Crawford modified t-test (Crawford & Howell, Citation1998; Crawford & Garthwaite, Citation2002). WR was able to identify all letters out of a set of letters, signs, and figures, and could match letters in different fonts. Naming and spelling of single letters was flawless. Spelling of regular words and pseudowords was slightly inferior to the control group, characterized by a letter-by-letter strategy, resulting in slow performance but with few errors. In contrast, spelling of words with irregular phoneme-grapheme correspondence was severely impaired with phonologically plausible spelling errors accounting for 85% of errors, sometimes combined with omissions and visual letter misidentification confusions of d/p or d/b (examples are displayed in ). Reading of regular words and pseudowords was very slow, but accurate with few self-corrections or errors. Reading of words with irregular grapheme-phoneme correspondence was errorful, producing almost exclusively regularizations and a slower speed due to self-corrections and hesitations (e.g., CLOWN [klˈa͡ʊn] was read as [klˈɔvn], REGIE [ʁəʒˈi] was read as [rˈeːɡɪə]). Nevertheless, once WR successfully read the words, her comprehension was good.
Table 3. Orthographic processing
Table 4. Examples of spelling errors.
4. Interim conclusions and hypothesis 2
While spoken language comprehension, vocabulary, verbal, fluency, syntactic processing, and phonemic memory were normal, WR exhibited extremely slow reading of regular and irregular words and pseudowords. This finding confirmed hypothesis 1 regarding the important role of the corpus callosum in reading.
Interestingly, WR’s written language testing revealed an additional deficit primarily affecting accuracy in reading and spelling of irregular words, indicative of surface dyslexia and dysgraphia. Thus, we formulated hypothesis 2, suggesting that reduced access to and/or an underdeveloped VWFA may be responsible for the observed surface dyslexia and dysgraphia in this patient. Based on previous research by Glezer et al. (Citation2015), which demonstrated selective increases in orthographic representations in the VWFA through word learning, and Purcell et al. (Citation2019) who reported enhanced neural differentiation within the left ventral occipitotemporal cortex after spelling treatment in acquired dysgraphia, hypothesis 2 led us to assume that a spelling treatment targeting and strengthening lexical representations would facilitate reading and spelling of irregular words.
We therefore developed an individual, model-driven treatment based on previous treatment studies on surface dyslexia and dysgraphia (Brunsdon et al., Citation2005; Lutz, Citation2016; Schmalzl & Nickels, Citation2006). Since some of them showed that using visual mnemonics could be of additional benefit (Brunsdon et al., Citation2005; De Partz et al., Citation1992; Schmalzl & Nickels, Citation2006), we incorporated visual mnemonics in our design. We used behavioural and neuroimaging methods to evaluate treatment effects with the following objectives:
Objective 2a: To determine whether spelling treatment improved the spelling of trained irregular words more than untrained irregular words. We predicted that the spelling treatment would lead to a greater increase in the number of correctly written trained irregular words compared to untrained irregular words after a training-free interval (hypothesis 2a).
Objective 2b: To assess whether the treatment also affected reading. We predicted that the treatment would influence not only spelling accuracy but also reading accuracy and speed of the trained words, as improved storage and access to irregular orthographic word forms may impact reading (hypothesis 2b).
Objective 2c: To investigate whether there was a treatment effect on white matter connectivity. Given the strong correlation between white matter tissue properties and reading proficiency, we predicted that successful treatment would enhance both intrahemispheric (Yeatman et al., Citation2011) temporo-occipital white matter connectivity, and also interhemispheric connectivity through alternative pathways in ACC (Dougherty et al., Citation2007; Siffredi et al., Citation2019; Tovar-Moll et al., Citation2014) (hypothesis 2c).
5. Spelling treatment and results
Treatment sessions were overseen by a speech therapist in training (BB) under the supervision of an experienced speech therapist (SMJ). Pre- and post-treatment examinations were conducted by an experienced clinical linguist (LBD). Due to the COVID pandemic, spelling training was performed remotely at WR’s home, with the assistance of her mother and the use of a web camera to monitor the girl’s writing. Each spelled word was recorded, and the original documents containing the written words were received after the sessions by mail.
5.1. Treatment items and schedule
Before treatment, WR’s spelling was assessed using 180 orthographically irregular words, of which 166 were incorrect. From these, 160 words were selected. Word frequences and regularities were derived from German word corpora (Goldhahn et al., Citation2012; Institut für Deutsche Sprache, Citation2012). WR’s reading accuracy for these words was 64%. The words were then divided into two groups matched for word frequency (X2(3) = 6.951, p = 0.073), number of syllables (X2(3) = 3.402, p = 0.334), and degree of orthographic irregularity (X2(2) = 0.619, p = 0.734), resulting in two groups of 80 words each. One group comprised the training words, the other group comprised the untrained words for assessing treatment effects. The training group comprised of 8 lists with 10 words each. Trained and untrained words were not tested again before training began.
Each list was trained for five days, with a break day in-between and after the training days (). This procedure was repeated for lists 1 to 4, resulting in training of 40 words within one treatment block. On the first day of the following week, each week’s list was tested with spelling to dictation. After block 1 and a 4-week interval without training, spelling and reading of all trained words as well as the corresponding 40 untrained words were tested. Subsequently, the procedure was repeated for lists 5–8 (). At the end, 4-week post-treatment results were obtained of 80 trained words and 80 untrained words.
5.2. Training sessions
One training session lasted approximately 30 minutes and involved the following steps for each of the ten training items. On the first day, the written training word was displayed on the screen, together with the corresponding line drawing of the object. The therapist read the word aloud, and WR was asked to copy the written word. Visual presentation of objects and words was then stopped, and WR folded the paper to ensure she could not see the copied word. Next, WR was asked to write the word again, first from memory, and then a second time after dictation. If correct, the therapist proceeded to the next training item. If incorrect, the therapist repeated the previous six steps. If incorrect again, the card was presented once more, but the therapist then proceeded to the next training item.
5.3. Statistical analyses
Analyses were conducted using SPSS Statistics 24 (IBM). McNemar Chi-Square Tests (χ²) and paired t-tests were performed to test for differences in spelling and reading accuracy and speed between trained and untrained words.
5.4. Treatment effect on spelling accuracy
After the treatment and a training-free interval of four weeks, spelling accuracy of trained words significantly increased from 0% pre-treatment to 51% post-treatment (). Moreover, WR showed improvement in the spelling of untrained words, with accuracy increasing from 0% pre-treatment to 15% post-treatment. However, the accuracy of spelling for trained words was significantly better than for untrained words (χ² p < 0.001; hypothesis 2a).
Table 5. Effect of treatment on spelling and reading of irregular words.
5.5. Treatment effect on Reading accuracy and speed
Reading accuracy and speed were evaluated before and four weeks after treatment. Since word irregularity was based on phoneme-grapheme correspondence rather than grapheme-phoneme correspondence, and German is a transparent orthography, reading accuracy of orthographically irregular words was much better than spelling accuracy prior to treatment. However, reading was exceptionally slow with a mean speed of 13 s per word prior to treatment ().
After treatment, reading accuracy and speed of the trained words were significantly better compared to the untrained ones (pre-treatment versus post-treatment accuracy: χ² p = 0.034; speed: paired t-test p < 0.001; hypothesis 2b). In addition, reading speed of the untrained words significantly improved after treatment (pre-treatment vesus post-treatment paired t-test p = 0.016).
5.6. Follow-up investigation of spelling and Reading one year after treatment
One year after treatment, reading and spelling of trained and untrained words were retested. Spelling accuracy of trained words was lower compared to the last assessment, but still significantly higher than that of untrained words (trained words 31/80, untrained words 16/80, χ² p < 0.001).
Reading accuracy results remained unchanged one year after treatment. Compared to pre-treatment, there was a significant improvement of reading accuracy for trained words (77/80, χ² p = 0.037), but not for untrained words (47/80, χ² p = 0.267). Reading speed continued to be significantly better than pre-treatment (paired t-test p < 0.001) and even improved further one year after treatment compared to the last assessment for both trained (mean 9.73, SD 3.72) and untrained words (mean 11.17, SD 3.74).
6. Treatment effects on white matter connectivity
6.1. MRI data acquisition
MRI acquisitions were performed using a 3 T Philips Achieva scanner with a 32-channel head coil. High resolution T1 images (Repetition time (TR)/Time to echo (TE) 8.1/3.7 ms, flip angle 8°, slice thickness 1 mm, 320 × 320 × 180 matrix, voxel size 0.75 × 0.75 × 1 mm, field of view 240 mm) were acquired as anatomical structural images. Functional MRI data were acquired using an echo planar imaging (EPI) sequence with a duration of five minutes (TR/TE 1000/25 ms, flip angle 65°, slice thickness 2.5 mm, 96 × 96 × 51 matrix, voxel size 2.3 × 2.3 × 2.5 mm, field of view 220.80 mm). For white matter structural analysis, multi-shell diffusion MRI (dMRI) data (TR/TE 3150/89 ms, flip angle 90°, slice thickness 2 mm, 144 × 144 × 66 matrix, voxel size 1.56 × 1.56 × 2 mm, b-values: 0, 1000, 2000, field of view 224.64 mm) were acquired.
6.2. Preprocessing and analysis of structural connectome
Diffusion-weighted images (DWI) underwent denoising and removal of Gibbs’ ringing artifacts (Tournier et al., Citation2019). Subsequently, we performed unwarping and removal of eddy currents using FSL’s eddy tool (Smith et al., Citation2004). This was followed by upsampling of DWI images and template mask to a 1.25 mm isotropic voxel size. Next, correction of field inhomogeneities for the DWI volumes was carried out using N4 algorithm implemented in ANTs (Tournier et al., Citation2019), and fiber orientation densities were computed with the Tournier algorithm based on multi-shell, multi-tissue constrained spherical deconvolution model (Tournier et al., Citation2013). Finally, normalization of the generated fiber orientation densities was performed.
Whole-brain tractography was performed creating 20 million streamlines. Streamlines optimization and refining was done using the tcksift2 algorithm as provided in MRtrix3 (Smith et al., Citation2012). Cortical and subcortical segmentation of T1-weighted images into 84 anatomical region (42 in each hemisphere) was performed using Freesurfer image analysis. These 84 brain regions were then automatically merged into lobe-based regions based on the Desikan-Killiany cortical atlas (Dale et al., Citation1999; Desikan et al., Citation2006; Fischl et al., Citation2002), resulting in six regions per hemisphere: frontal lobe, temporal lobe, insula, parietal lobe, occipital lobe, and cingulate cortex. Connectivity indices were then calculated by dividing the number of streamlines connecting two brain regions by the volume of the two regions using the “scale_invnodevol”-option implemented in MRtrix3. Hence, a connectivity index of 3, for example, represents three streamlines per mm3 brain region volume. This analysis allows a within-subject volume-independent comparison of connectivity indices between regions and investigations. Average structural connectivity of each region was investigated by calculating the mean connectivity index of this region to all other regions. Statistical analysis of pre- to post-treatment connectivity changes of average structural connectivities of each region to all other regions was performed using paired t-test (two-tailed), corrected for multiple comparisons by applying the Benjamini-Hochberg procedure. Cohen’s d was used as a measure of effect size.
6.3. Treatment effects
DWI measurements before and after treatment revealed two statistically significant findings: (1) a significant increase from pre- to post-treatment in the average white matter connectivity of the left temporal lobe, and (2) a significant decrease from pre- to post-treatment in the average white matter connectivity of the right cingulate cortex (hypothesis 2c, ). Post-hoc examination of the left temporal lobe changes (depicted in c) with values reported in Table S1) indicated that largest changes involved increased connectivity between the temporal lobe and the occipital lobe, while post-hoc examination of the right cingulate cortex changes indicated that largest changes involved decreased connectivity between the cingulate cortex and the occipital and the parietal lobe (note, however, that these changes were not statistically evaluated).
Figure 3. (a and b) Pre- and post-treatment white matter connectivity; The colour bar represents the connectivity indices (streamlines per mm3 brain region volume) from blue (connectivity index 0) to yellow (connectivity index ≥3); (c) White matter connectivity changes after treatment. The colour bar represents the pre- to post-treatment changes of connectome indices from blue (connectivity index decreased by -2) to yellow (connectivity index increased by +1). Note: FL, frontal lobe; CC, cingulate cortex; OL, occipital lobe; TL, temporal lobe; PL, parietal lobe; IN, insula.
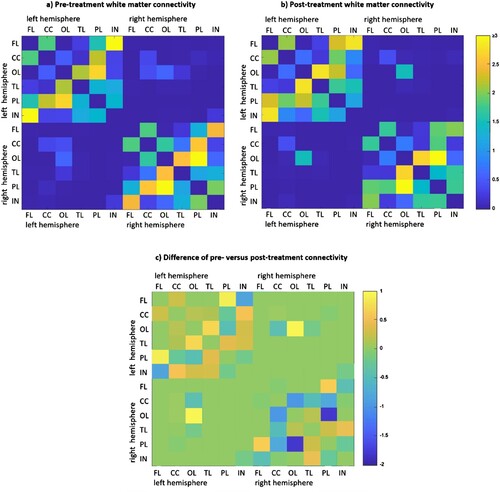
Table 6. Pre- to post-treatment differences of average structural connectivities of each region to all other regions.
In terms of interhemispheric connectivity, an increase in white matter connectivity between left and right occipital lobes was observed (c), Table S1), which, although not statistically evaluated, is suggestive as there was no evidence of other changes in interhemispheric connectivity. visualizes white matter connectivity between the hemispheres through the anterior and posterior commissures, which could possibly provide the basis for the observed changes in interhemispheric connectivity between occipital cortices, although this was not statistically confirmed.
Figure 4. White matter connectivity of the left and right occipital lobes before and after treatment, overlaid on the individual structural T1, neurological convention. The occipital lobes showed connectivity to ipsilateral temporal and frontal brain regions. Interhemispheric connectivity was possible through the anterior and posterior commissure. The colour-coding of tractography pathways is based on a standard red-green-blue (RGB) code that was applied to the vector in each brain area to show the spatial locations of terminal regions of each pathway (red for right-left, blue for cranial-caudal, and green for anterior-posterior). As pre- and post-treatment T1 images were taken at two different measurement time points, the orientation of the head within the scanner was not identical, and slices differed to some degree.
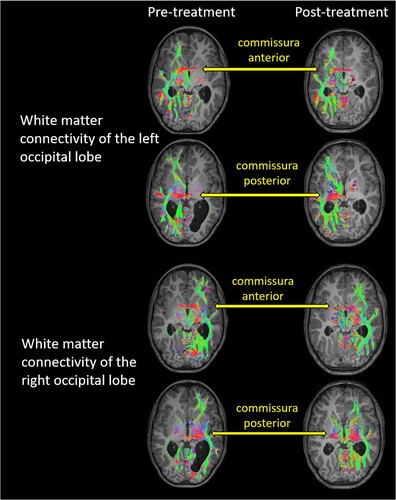
7. Discussion
This case report examines the cognitive performance, functional neuroimaging, and hypothesis-driven treatment outcomes in a 10-year-old girl with a complete, isolated CCA. Despite displaying average overall intellectual abilities, the girl exhibited a profound surface dyslexia and dysgraphia, characterized by a selective difficulty in reading and writing of orthographically irregular words. Treatment targeting spelling resulted in significant and persistent improvements in spelling, reading accuracy, and reading speed of trained orthographically irregular words compared to untrained words. Moreover, post-treatment analyses revealed a significant increase in ipsilateral white matter connectivity of the left temporal cortex, predominantly driven by increased ipsilateral connectivity of the temporal lobe with the occipital lobe, and a significant decrease of right hemisphere cingulate cortex connectivity. These findings are discussed against the background of the interhemispheric deficit theory of dyslexia.
7.1. The role of the corpus callosum in reading
WR had two observable reading deficits: First, she showed a slow letter-by-letter reading that affected all stimuli (regular words, irregular words, pseduowords). Second, she revealed impaired reading (and spelling) accuracy of orthographically irregular words. We hypothesize that both deficits can be attributed to the ACC: The corpus callosum is believed to play a major role in the hemispheric specialization of cognitive functions, especially language functions (Bartha-Doering et al., Citation2021a; Hines et al., Citation1992; Josse et al., Citation2008; Mandl et al., Citation2023). WR’s fMRI revealed bilateral auditory language representation and a left-handedness. This is a typical finding in complete ACC, where atypical language representations are found more often, together with ambidexterity or left-handedness (Bartha-Doering et al., Citation2021b). We hypothesize that the absence of the corpus callosum not only prevented a lateralization of the language system, but also affected the development of the VWFA. With an underdeveloped or dysfunctional VWFA, WR's brain lacked the ability to store orthographic whole-word informations, reflected by the patient’s surface dyslexia and dysgraphia.
Without access to an orthographic lexicon, WR’s brain might have processed orthographic information serially; hence, her reliance on a letter-by-letter reading approach might also be attributed to a dysfunctional VWFA. Indeed, previous research has linked letter-by-letter reading to VWFA damage (Binder & Mohr, Citation1992; Hanley & Kay, Citation1996; Saffran & Coslett, Citation1998) or deafferentiation (Cohen et al., Citation2004), indicating the absence of orthographic word representations necessary for rapid whole-word recognition. On the other hand, this does not explain WR’s letter by letter reading of regular words and pseudowords. Furthermore, previous studies have described letter-by-letter readers who can read irregular words correctly (Patterson & Kay, Citation1982). Alternative explanations for letter-by-letter reading have been offered which involve the failure of parallel processing due to splenial damage (Friedman & Alexander, Citation1984), a right-hemisphere reading mechanism (Shallice & Saffran, Citation1986), and visuospatial impairment during letter identification (Farah & Wallace, Citation1991; Kay & Hanley, Citation1991), all falling into a general category of “prelexical deficit” accounts of letter-by-letter reading (Rapp & Caramazza, Citation1991).
However, if the absence of the corpus callosum was responsible for WR’s observed deficits, the question arises of why surface dyslexia and dysgraphia have not been more commonly reported in children with ACC. We propose several reasons that may contribute to the scarcity of previous reports on these issues: (1) verbal IQ results are often reported, but studies specifically examining reading and spelling in individuals with ACC are limited (Bayram et al., Citation2020; Siffredi et al., Citation2013; Siffredi et al., Citation2018; Wright & Booth, Citation2023); (2) if written language skills are assessed, examinations are often not detailed enough, potentially overlooking important information about lexical and nonlexical routes of written language processing (Fischer et al., Citation1992; Moutard et al., Citation2003); (3) many studies on ACC include patients with partial ACC who may retain some interhemispheric connections (Brown et al., Citation1999; Panos et al., Citation2001; Wright & Booth, Citation2023); (4) individuals with ACC often present with broader intellectual impairments rather than isolated cognitive deficits, possibly masking specific symptoms (Finlay et al., Citation2000; Moutard et al., Citation2012; Siffredi et al., Citation2013; Stickles et al., Citation2002); (5) previous studies on ACC typically involve older adults, who may have had time to develop strategies for lexical reading and writing (Brown et al., Citation1999; Brown & Paul, Citation2000). Regarding the last point, it is worth considering whether WR might eventually develop word-form storage and lexical reading skills without treatment, albeit later in life. Thus, it is plausible to discuss whether the observed deficits could be viewed as a delay rather than a permanent impairment. However, the lack of reports on adults with ACC exhibiting persistent surface dyslexia and dysgraphia may support the notion that our assessments captured WR in a state of delayed written language development due to the lack of hemispheric specialization. To definitively address this question, more detailed investigations of reading and spelling are necessary in younger children with complete ACC.
Overall, in line with previous researchers, we propose that dyslexia is a highly heterogeneous disorder (Castles & Friedmann, Citation2014; McArthur et al., Citation2013). Whilst many children with developmental dyslexia present with phonological deficits, there are cases of developmental surface dyslexia where individuals show no phonological processing impairments. The language deficit in our patient, WR, was very specific and not of a phonological nature; she struggled profoundly with reading and writing words with irregular grapheme-phoneme correspondence, yet demonstrated close to normal accuracy with regular grapheme-phoneme conversion in words and nonwords. In addition, WR did not have a history of language developmental delay and displayed no impairments in comprehension, syntax, or word finding. Her verbal working memory was within the average range. Thus, we hypothesize, albeit speculatively, that in at least some children with developmental surface dyslexia and dysgraphia, dysfunctional interhemispheric transfer may underlie their difficulties, and further investigation of the corpus callosum through neuroimaging could provide insightful.
7.2. Effects of treatment—what do they tell us?
Eight weeks of spelling training significantly improved the spelling of trained words compared to untrained words. Furthermore, a cross modality transfer effect of spelling training to reading accuracy and speed of trained compared to untrained words could be observed. These treatment effects were reflected in changes in WR’s white matter connectivity. Statistical analyses and post-hoc evaluations of DWI revealed significant increases of white matter pathways in the left temporal lobe, driven by increased ipsilateral connectivity of the temporal lobe with the occipital lobe and the parietal lobe with the insula, and significant decreases of the right cingulate lobe connectivity, driven by reduced connectivity with the right parietal and occipital lobe. In addition, increases (although not statistically evaluated) in interhemispheric connectivity between occipital lobes were observed.
The transfer effect of the spelling treatment to reading abilities aligns with previous studies in developmental dyslexia that used single modality training and showed transfer effects of training in both directions: reading training improved spelling (Brunsdon et al., Citation2002), and spelling practice led to improved reading (Brunsdon et al., Citation2005; Kohnen et al., Citation2008). Accordingly, a unified lexicon of orthographic representations for both reading and spelling has been proposed (Purcell et al., Citation2014; Rapp & Lipka, Citation2011; Tsapkini & Rapp, Citation2010), supported by functional neuroimaging studies (Purcell et al., Citation2017), studies on neurotypical individuals (Basso et al., Citation1999; Ouellette, Citation2010), and on neurologically impaired readers and spellers (Angelelli et al., Citation2010; Behrmann & Bubb, Citation1992; Coltheart & Funnell, Citation1987; Friedman & Hadley, Citation1992). However, other studies have suggested two independent lexica (Campbell, Citation1987; Scott & Byng, Citation1989; Weekes & Coltheart, Citation1996) or separate lexica that share information (Jones & Rawson, Citation2016; Monsell, Citation1987). The reading improvement observed in our patient may have been influenced by the visual presentation of training words during the copying process. When the girl was first asked to copy the word form, this may have permitted silent reading in addition to copying. Furthermore, seeing her own written words provided visual feedback, which may have contributed to her reading skill development. Hence, we cannot exclude the impact of visual presentation on her reading abilities.
The left hemisphere white matter connectivity changes after treatment were observed in brain regions typically relevant for spelling and reading: spelling training significantly enhanced ipsilateral white matter connectivity of the left temporal lobe predominantly to the occipital lobe, but also to the posterior lobe and insula. These findings align with previous studies in children with developmental reading deficits (Banfi et al., Citation2019), in typical adults (Cheema et al., Citation2023), and in adults with acquired spelling and reading deficits (Joubert et al., Citation2004; Kubota et al., Citation2019; Planton et al., Citation2019) that show the relevance of the left occipitotemporal white matter pathways for spelling and reading and support current models of reading processes involving a ventral stream within the left temporo-occipital cortex for accessing familiar words encoded in lexical memory.
Remarkably, WR also exhibited increased interhemispheric connectivity between the occipital lobes after treatment. DW images exhibited white matter streamlines connecting the right and left hemispheres through the anterior and posterior commissures suggesting that in the absence of the corpus callosum, interhemispheric connectivity was established through the anterior and posterior commissure. This finding corroborates previous studies describing anomalous neural pathways crossing the midline via anterior and posterior commissures in individuals with ACC (Siffredi et al., Citation2019; Tovar-Moll et al., Citation2014). It has been shown that in children with ACC, larger posterior commissure volumes are associated with better selective attentional abilities (Siffredi et al., Citation2019).
WR showed a statistically significant decrease in white matter connectivity of the right cingulate cortex after treatment. A statistical evaluation of the single connectome indices was not possible, however, post-hoc analyses of right hemisphere connectome indices suggest that this decrease was caused by a reduced connectivity between the right cingulate cortex and ipsilateral occipital, temporal, and parietal cortices. A striking connectivity decrease was in addition detected between the right occipital and parietal lobe.
The increase of left hemisphere and decrease of right hemisphere connectivity observed in WR after treatment is similar to the pattern of age-related functional connectivity changes during typical development (Weiss-Croft & Baldeweg, Citation2015). In typically developing children, evolving language abilities are accompanied by an increased specialization of language-associated areas towards the left hemisphere, reducing the need for right hemisphere engagement (Szaflarski et al., Citation2006). Additonally, several studies in healthy children and children with developmental or acquired language deficits showed an association of left-sided language lateralization and different language abilities, including reading comprehension (Bartha-Doering et al., Citation2019; Berl et al., Citation2010; de Guibert et al., Citation2011; Everts et al., Citation2009; Lillywhite et al., Citation2009; Raja Beharelle et al., Citation2010). WR’s fMRI revealed bilateral language processing before treatment. The treatment might have induced an increased involvement of the left hemisphere during language processing, including an increased involvement of left ventral pathways in spelling and reading processes, and a reduced engagement of right hemisphere homologues. Nevertheless, since no post-treatment fMRI was conducted in WR, this assumption remains speculative.
The effects of treatment shed light on the neuroplasticity of the brain in children with ACC. Despite WR initially struggling to spell fewer than 10% of orthographically irregular words correctly, spelling training significantly improved her performance to 50% (36% more than matched untrained words), and this improvement persisted a year later. This underscores the clinical significance of tailored treatment for reading and spelling in children with ACC, offering hope for caregivers and affected individuals that substantial cognitive improvement is achievable through personalised therapy. Furthermore, it highlights the brain’s plasticity, even in the absence of a corpus callosum, particularly in areas related to the visual word form.
7.3. Limitations
Above all, it is essential to recognize that this is a single case report. Cubelli and Della Sala (Citation2017) argue that the single case study approach is the appropriate methodology for drawing inferences about the functional architecture of cognitive processes in studies involving brain-damaged patients. Moreover, single case reports permit the observation of unexpected phenomena, such as the surface dyslexia in our patient. Thus, we firmly believe that individual case studies, such as ours, play a significant role in advancing cognitive neuroscience. Nevertheless, it is important to acknowledge a limitation inherent in this being a single case study: Due to the lack of standardized methods to statistically analyze single subject DTI, an experimental analysis was performed. We based this analysis on previous studies and reported the exact methods and results to improve replicability, but this experimental approach has to be kept in mind when interpreting the findings. Additionally, employing a customized fMRI paradigm to explore neural activation during reading both pre- and post-treatment would have substantially enhanced our hypotheses and interpretations. Hence, we propose future research in ACC to not only assess spelling and reading abilities but also to investigate the associated brain regions using customized fMRI and DTI. This will enable a deeper exploration of the role of the corpus callosum in spelling and reading.
7.4. Conclusions and outlook
This case report of a girl with ACC and surface dysgraphia and dyslexia contributes to our understanding of the crucial role of the corpus callosum in written language acquisition, supporting the interhemispheric deficit theory of dyslexia. Aditionally, it demonstrates that spelling treatment has the potential to enhance interhemispheric white matter connectivity in individuals with ACC through alternative pathways. This improvement may allow for the development of a more efficient functional organization of the visual word form area within the left temporo-occipital cortex.
Ethics approval
Ethics approval was obtained from the Ethics Committee of the Medical University of Vienna for the present study. A parent gave written, informed consent prior to inclusion.
CN-FA 28.23.R4_SUPPLEMENTARY FILE.docx
Download MS Word (340.4 KB)Acknowledgements
We are very grateful to WR and her family for their enthusiasm, patience, invested time, and interest in this study.
Data availability
Fully anonymized data from this study can be made available on request.
Disclosure statement
No potential conflict of interest was reported by the author(s).
References
- Alexander, M. P., Friedman, R. B., Loverso, F., & Fischer, R. S. (1992). Lesion localization of phonological agraphia. Brain and Language, 43(1), 83–95. https://doi.org/10.1016/0093-934X(92)90022-7
- Angelelli, P., Marinelli, C. V., & Zoccolotti, P. (2010). Single or dual orthographic representations for reading and spelling? A study of Italian dyslexic-dysgraphic and normal children. Cognitive Neuropsychology, 27(4), 305–33. https://doi.org/10.1080/02643294.2010.543539
- Artuvan Korkmaz, H., Ari, F., Doganay Erdogan, B., & Kalaycıoğlu, C. (2022). Interhemispheric transfer time differs between fast and slow healthy adult readers. Neuropsychologia, 169, 108205. https://doi.org/10.1016/j.neuropsychologia.2022.108205
- Aschenbrenner, S., Tucha, O., & Lange, K. W. (2001). Regensburger Wortfluessigkeitstest. Göttingen: Testzentrale.
- Badzakova-Trajkov, G., Hamm, J. P., & Waldie, K. E. (2005). The effects of redundant stimuli on visuospatial processing in developmental dyslexia. Neuropsychologia, 43(3), 473–8. https://doi.org/10.1016/j.neuropsychologia.2004.06.016
- Banfi, C., Koschutnig, K., Moll, K., Schulte-Körne, G., Fink, A., & Landerl, K. (2019). White matter alterations and tract lateralization in children with dyslexia and isolated spelling deficits. Human Brain Mapping, 40(3), 765–776. https://doi.org/10.1002/hbm.24410
- Bartha-Doering, L., Kollndorfer, K., Schwartz, E., Fischmeister, F. P. S., Alexopoulos, J., Langs, G., Prayer, D., Kasprian, G., & Seidl, R. (2021a). The role of the corpus callosum in language network connectivity in children. Developmental Science, 24(2), e13031. https://doi.org/10.1111/desc.13031
- Bartha-Doering, L., Novak, A., Kollndorfer, K., Schuler, A.-L., Kasprian, G., Langs, G., Schwartz, E., Fischmeister, F. P. S., Prayer, D., & Seidl, R. (2019). Atypical language representation is unfavorable for language abilities following childhood stroke. European Journal of Paediatric Neurology, 23(1), 102–116. https://doi.org/10.1016/j.ejpn.2018.09.007
- Bartha-Doering, L., Schwartz, E., Kollndorfer, K., Fischmeister, F. P. S., Novak, A., Langs, G., Werneck, H., Prayer, D., Seidl, R., & Kasprian, G. (2021b). Effect of corpus callosum agenesis on the language network in children and adolescents. Brain Structure and Function, 226(3), 701–713. https://doi.org/10.1007/s00429-020-02203-6
- Basso, A., Burgio, F., & Prandoni, P. (1999). Acquisition of output irregular orthographic representations in normal adults: An experimental study. Journal of the International Neuropsychological Society, 5(5), 405–12. https://doi.org/10.1017/S1355617799555033
- Bayram, A. K., Kütük, M. S., Doganay, S., Özgün, M. T., Gümüş, H., Başbuğ, M., Kumandaş, S., Canpolat, M., & Per, H. (2020). An analysis of 109 fetuses with prenatal diagnosis of complete agenesis of corpus callosum. Neurological Sciences, 41(6), 1521–1529. https://doi.org/10.1007/s10072-019-04224-4
- Behrmann, M., & Bubb, D. (1992). Surface dyslexia and dysgraphia: Dual routes, single lexicon. Cognitive Neuropsychology, 9(3), 209–251. https://doi.org/10.1080/02643299208252059
- Behrmann, M., & Plaut, D. C. (2020). Hemispheric organization for visual object recognition: A theoretical account and empirical evidence. Perception, 49(4), 373–404. https://doi.org/10.1177/0301006619899049
- Benton, A. L., Varney, N. R., & Hamsher, K. D. (1978). Visuospatial judgment. A clinical test. Archives of Neurology, 35(6), 364–7. https://doi.org/10.1001/archneur.1978.00500300038006
- Berl, M. M., Duke, E. S., Mayo, J., Rosenberger, L. R., Moore, E. N., VanMeter, J., Ratner, N. B., Vaidya, C. J., & Gaillard, W. D. (2010). Functional anatomy of listening and Reading comprehension during development. Brain and Language, 114(2), 115–25. https://doi.org/10.1016/j.bandl.2010.06.002
- Binder, J. R., & Mohr, J. P. (1992). The topography of callosal reading pathways. A case-control analysis. Brain, 115(Pt 6), 1807–26. https://doi.org/10.1093/brain/115.6.1807
- Bishop, D. V. M. (2003). Test for reception of grammar (TROG - 2). London: Harcourt Assessment.
- Bosse, M. L., Tainturier, M. J., & Valdois, S. (2007). Developmental dyslexia: The visual attention span deficit hypothesis. Cognition, 104(2), 198–230. https://doi.org/10.1016/j.cognition.2006.05.009
- Bradley, L., & Bryant, P. E. (1978). Difficulties in auditory organisation as a possible cause of reading backwardness. Nature, 271(5647), 746–7. https://doi.org/10.1038/271746a0
- Bradshaw, A. R., Bishop, D., & Woodhead, Z. (2020). Testing the interhemispheric deficit theory of dyslexia using the visual half-field technique. Quarterly Journal of Experimental Psychology, 73(7), 1004–1016. https://doi.org/10.1177/1747021819895472
- Broom, Y. M., & Doctor, E. A. (1995). Developmental surface dyslexia: A case study of the efficacy of a remediation programme. Cognitive Neuropsychology, 12(1), 69–110. https://doi.org/10.1080/02643299508251992
- Brown, W. S., Jeeves, M. A., Dietrich, R., & Burnison, D. S. (1999). Bilateral field advantage and evoked potential interhemispheric transmission in commissurotomy and callosal agenesis. Neuropsychologia, 37(10), 1165–80. https://doi.org/10.1016/S0028-3932(99)00011-1
- Brown, W. S., & Paul, L. K. (2000). Cognitive and psychosocial deficits in agenesis of the corpus callosum with normal intelligence. Cognitive Neuropsychiatry, 5(2), 135–157. https://doi.org/10.1080/135468000395781
- Brown, W. S., Symingtion, M., VanLancker-Sidtis, D., Dietrich, R., & Paul, L. K. (2005). Paralinguistic processing in children with callosal agenesis: Emergence of neurolinguistic deficits. Brain and Language, 93(2), 135–9. https://doi.org/10.1016/j.bandl.2004.09.003
- Brunsdon, R., Coltheart, M., & Nickels, L. (2005). Treatment of irregular word spelling in developmental surface dysgraphia. Cognitive Neuropsychology, 22(2), 213–51. https://doi.org/10.1080/02643290442000077
- Brunsdon, R. K., Hannan, T. J., Coltheart, M., & Nickels, L. (2002). Treatment of lexical processing in mixed dyslexia: A case study. Neuropsychological Rehabilitation, 12(5), 385–418. https://doi.org/10.1080/09602010244000174
- Campbell, R. (1987). One or two lexicons for Reading and writing words: Can misspellings shed any light? Cognitive Neuropsychology, 4(4), 487–499. https://doi.org/10.1080/02643298708252049
- Castles, A. (1996). Cognitive correlates of developmental surface dyslexia: A single case study. Cognitive Neuropsychology, 13(1), 25–50. https://doi.org/10.1080/026432996382051
- Castles, A., & Coltheart, M. (1996). Cognitive correlates of developmental surface dyslexia: A single case study. Cognitive Neuropsychology, 13(1), 25–50. https://doi.org/10.1080/026432996382051
- Castles, A., & Friedmann, N. (2014). Developmental dyslexia and the phonological deficit hypothesis. Mind & Language, 29(3), 270–285. https://doi.org/10.1111/mila.12050
- Cheema, K., Sweneya, S., Craig, J., Huynh, T., Ostevik, A. V., Reed, A., & Cummine, J. (2023). An investigation of white matter properties as they relate to spelling behaviour in skilled and impaired readers. Neuropsychological Rehabilitation, 33(6), 989–1017. https://doi.org/10.1080/09602011.2022.2053168
- Cohen, L., & Dehaene, S. (2004). Specialization within the ventral stream: The case for the visual word form area. Neuroimage, 22(1), 466–76. https://doi.org/10.1016/j.neuroimage.2003.12.049
- Cohen, L., Henry, C., Dehaene, S., Martinaud, O., Lehéricy, S., Lemer, C., & Ferrieux, S. (2004). The pathophysiology of letter-by-letter Reading. Neuropsychologia, 42(13), 1768–80. https://doi.org/10.1016/j.neuropsychologia.2004.04.018
- Coltheart, M., & Funnell, E. (1987). Reading and writing: One lexicon or two? In D. A. Allport, D. G. Mackay, W. Prinz, & E. Scherer (Eds.), Language and production: Relationships between listening, speaking, reading, and writing (pp. 313–339). London: Academic Press.
- Coltheart, M., Masterson, J., Byng, S., Prior, M., & Riddoch, J. (1983). Surface dyslexia. The Quarterly Journal of Experimental Psychology Section A, 35(3), 469–495. https://doi.org/10.1080/14640748308402483
- Coltheart, M., Rastle, K., Perry, C., Langdon, R., & Ziegler, J. (2001). DRC: A dual route cascaded model of visual word recognition and Reading aloud. Psychological Review, 108(1), 204–56. https://doi.org/10.1037/0033-295X.108.1.204
- Crawford, J. R., & Garthwaite, P. H. (2002). Investigation of the single case in neuropsychology: Confidence limits on the abnormality of test scores and test score differences. Neuropsychologia, 40(8), 1196–208. https://doi.org/10.1016/S0028-3932(01)00224-X
- Crawford, J. R., & Howell, D. C. (1998). Comparing an individual's test score against norms derived from small samples. The Clinical Neuropsychologist, 12(4), 482–486. https://doi.org/10.1076/clin.12.4.482.7241
- Cubelli, R., & Della Sala, S. (2017). Looking back to go forward: Promoting single case studies. Cortex, 97, A1–A3. https://doi.org/10.1016/j.cortex.2017.09.023
- Daini, R., De Fabritiis, P., Ginocchio, C., Lenti, C., Lentini, C. M., Marzorati, D., & Lorusso, M. L. (2018). Revisiting strephosymbolie: The connection between interhemispheric transfer and developmental dyslexia. Brain Sciences, 8(4), https://doi.org/10.3390/brainsci8040067
- Dale, A. M., Fischl, B., & Sereno, M. I. (1999). Cortical surface-based analysis. I. Segmentation and surface reconstruction. Neuroimage, 9, 179–94.
- de Guibert, C., Maumet, C., Jannin, P., Ferré, J.-C., Tréguier, C., Barillot, C., Le Rumeur, E., Allaire, C., & Biraben, A. (2011). Abnormal functional lateralization and activity of language brain areas in typical specific language impairment (developmental dysphasia). Brain, 134(10), 3044–58. https://doi.org/10.1093/brain/awr141
- Dehaene, S., & Cohen, L. (2011). The unique role of the visual word form area in reading. Trends in Cognitive Sciences, 15(6), 254–62. https://doi.org/10.1016/j.tics.2011.04.003
- Dehaene, S., & Dehaene-Lambertz, G. (2016). Is the brain prewired for letters? Nature Neuroscience, 19(9), 1192–3. https://doi.org/10.1038/nn.4369
- Delis, D. C., Kaplan, E., & Kramer, J. H. (2001). Delis-Kaplan executive function system: Examiner’s manual. The Psychological Corporation.
- DeMarco, A. T., et al. (2017). Neural substrates of sublexical processing for spelling. Brain and Language, 164, 118–128. https://doi.org/10.1016/j.bandl.2016.10.001
- De Partz, M., Seron, X., & Vn der Linden, M. (1992). Re-education of a surface dysgraphia with a visual imagery strategy. Cognitive Neuropsychology, 9(5), 369–401. https://doi.org/10.1080/02643299208252065
- Desikan, R. S., et al. (2006). An automated labeling system for subdividing the human cerebral cortex on MRI scans into gyral based regions of interest. Neuroimage, 31(3), 968–80. https://doi.org/10.1016/j.neuroimage.2006.01.021
- Dougherty, R. F., et al. (2007). Temporal-callosal pathway diffusivity predicts phonological skills in children. Proceedings of the National Academy of Sciences, 104(20), 8556–61. https://doi.org/10.1073/pnas.0608961104
- Duara, R., Kushch, A., Gross-Glenn, K., Barker, W. W., Jallad, B., Pascal, S., Loewenstein, D. A., Sheldon, J., Rabin, M., Levin, B., & Lubs, H. (1991). Neuroanatomic differences between dyslexic and normal readers on magnetic resonance imaging scans. Archives of Neurology, 48(4), 410–6. https://doi.org/10.1001/archneur.1991.00530160078018
- Ellis, A. W. (2004). Length, formats, neighbours, hemispheres, and the processing of words presented laterally or at fixation. Brain and Language, 88(3), 355–66. https://doi.org/10.1016/S0093-934X(03)00166-4
- Everts, R., Lidzba, K., Wilke, M., Kiefer, C., Mordasini, M., Schroth, G., Perrig, W., & Steinlin, M. (2009). Strengthening of laterality of verbal and visuospatial functions during childhood and adolescence. Human Brain Mapping, 30(2), 473–483. https://doi.org/10.1002/hbm.20523
- Fabbro, F., Pesenti, S., Facoetti, A., Bonanomi, M., Libera, L., & Lorusso, M. L. (2001). Callosal transfer in different subtypes of developmental dyslexia. Cortex, 37(1), 65–73. https://doi.org/10.1016/S0010-9452(08)70558-6
- Farah, M. J., & Wallace, M. A. (1991). Pure alexia as a visual impairment: A reconsideration. Cognitive Neuropsychology, 8(3-4), 313–334. https://doi.org/10.1080/02643299108253376
- Finlay, D. C., Peto, T., Payling, J., Hunter, M., Fulham, W. R., & Wilkinson, I. (2000). A study of three cases of familial related agenesis of the corpus callosum. Journal of Clinical and Experimental Neuropsychology, 22(6), 731–42. https://doi.org/10.1076/jcen.22.6.731.962
- Fischer, M., Ryan, S. B., & Dobyns, W. B. (1992). Mechanisms of interhemispheric transfer and patterns of cognitive function in acallosal patients of normal intelligence. Archives of Neurology, 49(3), 271–7. https://doi.org/10.1001/archneur.1992.00530270085023
- Fischl, B., Salat, D. H., Busa, E., Albert, M., Dieterich, M., Haselgrove, C., van der Kouwe, A., Killiany, R., Kennedy, D., Klaveness, S., Montillo, A., Makris, N., Rosen, B., & Dale, A. M. (2002). Whole brain segmentation: Automated labeling of neuroanatomical structures in the human brain. Neuron, 33(3), 341–55. https://doi.org/10.1016/S0896-6273(02)00569-X
- Folk, J. R., & Rapp, B. (2004). Interaction of lexical and sublexical information in spelling: Evidence from nonword priming. Applied Psycholinguistics, 25(4), 565–585. https://doi.org/10.1017/S0142716404001274
- Friederici, A. D., & Alter, K. (2004). Lateralization of auditory language functions: A dynamic dual pathway model. Brain and Language, 89(2), 267–76. https://doi.org/10.1016/S0093-934X(03)00351-1
- Friedman, R. B., & Alexander, M. P. (1984). Pictures, images, and pure alexia: A case study. Cognitive Neuropsychology, 1(1), 9–23. https://doi.org/10.1080/02643298408252014
- Friedman, R. B., & Hadley, J. A. (1992). Letter-by-letter surface alexia. Cognitive Neuropsychology, 9(3), 185–208. https://doi.org/10.1080/02643299208252058
- Friedmann, N., & Lukov, L. (2008). Developmental surface dyslexias. Cortex, 44(9), 1146–60. https://doi.org/10.1016/j.cortex.2007.09.005
- Giofrè, D., Toffalini, E., Provazza, S., Calcagnì, A., Altoè, G., & Roberts, D. J. (2019). Are children with developmental dyslexia all the same? A cluster analysis with more than 300 cases. Dyslexia, 25(3), 284–295. https://doi.org/10.1002/dys.1629
- Glezer, L. S., Kim, J., Rule, J., Jiang, X., & Riesenhuber, M. (2015). Adding words to the brain's visual dictionary: Novel word learning selectively sharpens orthographic representations in the VWFA. The Journal of Neuroscience, 35(12), 4965–72. https://doi.org/10.1523/JNEUROSCI.4031-14.2015
- Glück, C. W. (2011). Wortschatz- und Wortfindungstest für 6- bis 10-Jährige. München: Elsevier.
- Goldhahn, D., Eckart, T., & Quasthoff, U. (2012). Building large monolingual dictionaries at the Leipzig corpora collection: From 100 to 200 languages. Proceedings of the 8th International Language Resources and Evaluation (LREC'12), 759–765.
- Graves, W. W., Desai, R., Humphries, C., Seidenberg, M. S., & Binder, J. R. (2010). Neural systems for Reading aloud: A multiparametric approach. Cerebral Cortex, 20(8), 1799–815. https://doi.org/10.1093/cercor/bhp245
- Gross-Glenn, K., & Rothenberg, S. (1984). Evidence for deficit in interhemispheric transfer of information in dyslexic boys. International Journal of Neuroscience, 24(1), 23–35. https://doi.org/10.3109/00207458409079531
- Gruner, E., Zeller, M. S., & Fleck, C. (2013). Phonematischer Gedächnistest (PHOG). Testverfahren zur Überprüfung der phonematischen Gedächtnisleistung bei Kindern, Jugendlichen und Erwachsenen nach Gruner. Bern: Verlag Hans Huber.
- Gvion, A., & Friedmann, N. (2016). A principled relation between Reading and naming in acquired and developmental anomia: Surface dyslexia following impairment in the phonological output lexicon. Frontiers in Psychology, 7, 340. https://doi.org/10.3389/fpsyg.2016.00340
- Halderman, L. K. (2011). Evidence for right hemisphere phonology in a backward masking task. Brain and Language, 119(3), 232–7. https://doi.org/10.1016/j.bandl.2011.05.005
- Hanley, J. R., & Kay, J. (1996). Reading speed in pure alexia. Neuropsychologia, 34(12), 1165–74. https://doi.org/10.1016/0028-3932(96)00039-5
- Hanley, J. R., & Sotiropoulos, A. (2018). Developmental surface dysgraphia without surface dyslexia. Cognitive Neuropsychology, 35(5-6), 333–341. https://doi.org/10.1080/02643294.2018.1468317
- Hasbrooke, R. E., & Chiarello, C. (1998). Bihemispheric processing of redundant bilateral lexical information. Neuropsychology, 12(1), 78–94. https://doi.org/10.1037/0894-4105.12.1.78
- Hellige, J. B. (1993). Hemispheric asymmetry: What’s right and what’s left. Cambridge: Harvard University Press.
- Hellige, J. B., Taylor, A. K., & Eng, T. L. (1989). Interhemispheric interaction when both hemispheres have access to the same stimulus information. Journal of Experimental Psychology: Human Perception and Performance, 15(4), 711–22. https://doi.org/10.1037/0096-1523.15.4.711
- Helmstaedter, C., Lendt, M., & Lux, S. (2001). Verbaler Lern- und Merkfaehigkeitstest VLMT. Göttingen: Beltz Test.
- Henderson, L., Barca, L., & Ellis, A. W. (2007). Interhemispheric cooperation and non-cooperation during word recognition: Evidence for callosal transfer dysfunction in dyslexic adults. Brain and Language, 103(3), 276–91. https://doi.org/10.1016/j.bandl.2007.04.009
- Henry, M. L., Beeson, P. M., Stark, A. J., & Rapcsak, S. Z. (2007). The role of left perisylvian cortical regions in spelling. Brain and Language, 100(1), 44–52. https://doi.org/10.1016/j.bandl.2006.06.011
- Hepner, C., McCloskey, M., & Rapp, B. (2017). Do reading and spelling share orthographic representations? Evidence from developmental dysgraphia. Cognitive Neuropsychology, 34(3-4), 119–143. https://doi.org/10.1080/02643294.2017.1375904
- Hickok, G., & Poeppel, D. (2007). The cortical organization of speech processing. Nature Reviews Neuroscience, 8(5), 393–402. https://doi.org/10.1038/nrn2113
- Hines, M., Chiu, L., McAdams, L. A., Bentler, P. M., & Lipcamon, J. (1992). Cognition and the corpus callosum: Verbal fluency, visuospatial ability, and language lateralization related to midsagittal surface areas of callosal subregions. Behavioral Neuroscience, 106(1), 3–14. https://doi.org/10.1037/0735-7044.106.1.3
- Hinkley, L. B. N., Marco, E. J., Brown, E. G., Bukshpun, P., Gold, J., Hill, S., Findlay, A. M., Jeremy, R. J., Wakahiro, M. L., Barkovich, A. J., Mukherjee, P., Sherr, E. H., & Nagarajan, S. S. (2016). The contribution of the corpus callosum to language lateralization. The Journal of Neuroscience, 36(16), 4522–33. https://doi.org/10.1523/JNEUROSCI.3850-14.2016
- Hinkley, L. B. N., Marco, E. J., Findlay, A. M., Honma, S., Jeremy, R. J., Strominger, Z., Bukshpun, P., Wakahiro, M., Brown, W. S., Paul, L. K., Barkovich, A. J., Mukherjee, P., Nagarajan, S. S., & Sherr, E. H. (2012). The role of corpus callosum development in functional connectivity and cognitive processing. PLoS One, 7(8), e39804. https://doi.org/10.1371/journal.pone.0039804
- Ho, C. S.-H., Chan, D. W., Chung, K. K. H., Lee, S.-H., & Tsang, S.-M. (2007). In search of subtypes of Chinese developmental dyslexia. Journal of Experimental Child Psychology, 97(1), 61–83. https://doi.org/10.1016/j.jecp.2007.01.002
- Hoffman, P., Lambon Ralph, M. A., & Woollams, A. M. (2015). Triangulation of the neurocomputational architecture underpinning reading aloud. Proc Natl Acad Sci U S A, 112, E3719–28.
- Huber, W., et al. (1983). Aachener Aphasie Test. Göttingen: Hogrefe.
- Hynd, G. W., Hall, J., Novey, E. S., Eliopulos, D., Black, K., Gonzalez, J. J., Edmonds, J. E., Riccio, C., & Cohen, M. (1995). Dyslexia and corpus callosum morphology. Archives of Neurology, 52(1), 32–8. https://doi.org/10.1001/archneur.1995.00540250036010
- Iacoboni, M., & Zaidel, E. (1996). Hemispheric independence in word recognition: Evidence from unilateral and bilateral presentations. Brain and Language, 53(1), 121–40. https://doi.org/10.1006/brln.1996.0040
- Institut für Deutsche Sprache. (2012). Deutsches Referenzkorpus / Archiv der Korpora geschriebener Gegenwartssprache. Mannheim: Institut für Deutsche Sprache.
- Jones, A. C., & Rawson, K. A. (2016). Do reading and spelling share a lexicon? Cognitive Psychology, 86, 152–84. https://doi.org/10.1016/j.cogpsych.2016.02.003
- Josse, G., Seghier, M. L., Kherif, F., & Price, C. J. (2008). Explaining function with anatomy: Language lateralization and corpus callosum size. The Journal of Neuroscience, 28(52), 14132–9. https://doi.org/10.1523/JNEUROSCI.4383-08.2008
- Joubert, S., Beauregard, M., Walter, N., Bourgouin, P., Beaudoin, G., Leroux, J.-M., Karama, S., & Lecours, A. R. (2004). Neural correlates of lexical and sublexical processes in reading. Brain and Language, 89(1), 9–20. https://doi.org/10.1016/S0093-934X(03)00403-6
- Judica, A., Luca, M. D., Spinelli, D., & Zoccolotti, P. (2002). Training of developmental surface dyslexia improves Reading performance and shortens eye fixation duration in reading. Neuropsychological Rehabilitation, 12(3), 177–197. https://doi.org/10.1080/09602010244000002
- Kay, J., & Hanley, R. (1991). Simultaneous form perception and serial letter recognition in a case of letter-by-letter Reading. Cognitive Neuropsychology, 8(3-4), 249–273. https://doi.org/10.1080/02643299108253374
- Kinsbourne, M. (2003). The corpus callosum equilibrates the cerebral hemispheres. In E. Zaidel, & M. Iacoboni (Eds.), The parallel brain: The cognitive neuroscience of the corpus callosum (pp. 271–281). Cambridge: MIT Press.
- Kohnen, S., Nickels, L., Coltheart, M., & Brunsdon, R. (2008). Predicting generalization in the training of irregular-word spelling: Treating lexical spelling deficits in a child. Cognitive Neuropsychology, 25(3), 343–75. https://doi.org/10.1080/02643290802003000
- Kohnen, S., Nickels, L., Geigis, L., Coltheart, M., McArthur, G., & Castles, A. (2018). Variations within a subtype: Developmental surface dyslexias in English. Cortex, 106, 151–163. https://doi.org/10.1016/j.cortex.2018.04.008
- Kubota, E. C., Joo, S. J., Huber, E., & Yeatman, J. D. (2019). Word selectivity in high-level visual cortex and reading skill. Developmental Cognitive Neuroscience, 36, 100593. https://doi.org/10.1016/j.dcn.2018.09.003
- Larsen, J. P., Hoien, T., & Odegaard, H. (1992). Magnetic resonance imaging of the corpus callosum in developmental dyslexia. Cognitive Neuropsychology, 9(2), 123–134. https://doi.org/10.1080/02643299208252055
- Lezak, M. D. (1995). Neuropsychological assessment (3rd ed). Oxford: University Press.
- Lillywhite, L. M., Saling, M. M., Simon Harvey, A., Abbott, D. F., Archer, J. S., Vears, D. F., Scheffer, I. E., & Jackson, G. D. (2009). Neuropsychological and functional MRI studies provide converging evidence of anterior language dysfunction in BECTS. Epilepsia, 50(10), 2276–2284. https://doi.org/10.1111/j.1528-1167.2009.02065.x
- Ludersdorfer, P., Kronbichler, M., & Wimmer, H. (2015). Accessing orthographic representations from speech: The role of left ventral occipitotemporal cortex in spelling. Human Brain Mapping, 36(4), 1393–406. https://doi.org/10.1002/hbm.22709
- Lukov, L., et al. (2014). Dissociations between developmental dyslexias and attention deficits. Front Psychol, 5, 1501.
- Lutz, L., (2016). MODAK - Modalitätenaktivierung in der Aphasietherapie. Berlin, Heidelberg: Springer.
- Mandl, S., et al.. (2023). Larger corpus callosum volume is favorable for theory of mind development in healthy children. Cerebral Cortex, 33(23), 11197–11205. https://doi.org/10.1093/cercor/bhad353
- Manis, F. R., Seidenberg, M. S., Doi, L. M., McBride-Chang, C., & Petersen, A. (1996). On the bases of two subtypes of development dyslexia. Cognition, 58(2), 157–195. https://doi.org/10.1016/0010-0277(95)00679-6
- Marco, E. J., Harrell, K. M., Brown, W. S., Hill, S. S., Jeremy, R. J., Kramer, J. H., Sherr, E. H., & Paul, L. K. (2012). Processing speed delays contribute to executive function deficits in individuals with agenesis of the corpus callosum. Journal of the International Neuropsychological Society, 18((03|3)), 521–9. https://doi.org/10.1017/S1355617712000045
- Marshall, J. C., & Newcombe, F. (1973). Patterns of paralexia: A psycholinguistic approach. Journal of Psycholinguistic Research, 2(3), 175–99. https://doi.org/10.1007/BF01067101
- McArthur, G., Kohnen, S., Larsen, L., Jones, K., Anandakumar, T., Banales, E., & Castles, A. (2013). Getting to grips with the heterogeneity of developmental dyslexia. Cognitive Neuropsychology, 30(1), 1–24. https://doi.org/10.1080/02643294.2013.784192
- McGhee, R. L., Ehrler, D. J., & DiSimoni, F. (2007). TTFC-2. The Token Test for Children. Austin, Texas: ProEd.
- Mohr, B., Endrass, T., Hauk, O., & Pulvermuller, F. (2007). ERP correlates of the bilateral redundancy gain for words. Neuropsychologia, 45(9), 2114–24. https://doi.org/10.1016/j.neuropsychologia.2007.01.015
- Mohr, B., & Pulvermuller, F. (2002). Redundancy gains and costs in cognitive processing: Effects of short stimulus onset asynchronies. Journal of Experimental Psychology: Learning, Memory, and Cognition, 28(6), 1200–23. https://doi.org/10.1037/0278-7393.28.6.1200
- Mohr, B., Pulvermüller, F., Cohen, R., & Rockstroh, B. (2000). Interhemispheric cooperation during word processing: Evidence for callosal transfer dysfunction in schizophrenic patients. Schizophrenia Research, 46(2-3), 231–9. https://doi.org/10.1016/S0920-9964(00)00020-7
- Mohr, B., Pulvermüller, F., Rayman, J., & Zaidel, E. (1994). Interhemispheric cooperation during lexical processing is mediated by the corpus callosum: Evidence from the split-brain. Neuroscience Letters, 181(1-2), 17–21. https://doi.org/10.1016/0304-3940(94)90550-9
- Molko, N., Cohen, L., Mangin, J. F., Chochon, F., Lehéricy, S., Bihan, D. L., & Dehaene, S. (2002). Visualizing the neural bases of a disconnection syndrome with diffusion tensor imaging. Journal of Cognitive Neuroscience, 14(4), 629–36. https://doi.org/10.1162/08989290260045864
- Moll, K., & Landerl, K. (2010). Lese- und Rechtschreibtest SLRT II. Huber.
- Monaghan, P., & Shillcock, R. (2008). Hemispheric dissociation and dyslexia in a computational model of Reading⋆. Brain and Language, 107(3), 185–93. https://doi.org/10.1016/j.bandl.2007.12.005
- Monsell, S. (1987). Nonvisual orthographic processing and the orthographic input lexicon. In M. Coltheart (Ed.), Attention and performance XII: Reading (pp. 299–323). Hove, UK: Lawrence Erlbaum Associates.
- Moore, L. H., et al. (1995). Bimanual coordination in dyslexic adults. Neuropsychologia, 33(6), 781–93. https://doi.org/10.1016/0028-3932(95)00019-Y
- Moutard, M.-L., Kieffer, V., Feingold, J., Kieffer, F., Lewin, F., Adamsbaum, C., Gélot, A., Campistol i Plana, J., van Bogaert, P., André, M., & Ponsot, G. (2003). Agenesis of corpus callosum: Prenatal diagnosis and prognosis. Child's Nervous System, 19(7-8), 471–6. https://doi.org/10.1007/s00381-003-0781-6
- Moutard, M., Kieffer, V., Feingold, J., Lewin, F., Baron, J., Adamsbaum, C., Gélot, A., Isapof, A., Kieffer, F., & de Villemeur, T. B. (2012). Isolated corpus callosum agenesis: A ten-year follow-up after prenatal diagnosis (How are the children without corpus callosum at 10 years of age?). Prenatal Diagnosis, 32(3), 277–83. https://doi.org/10.1002/pd.3824
- Norton, E. S., Beach, S. D., & Gabrieli, J. D. (2015). Neurobiology of dyslexia. Current Opinion in Neurobiology, 30, 73–8. https://doi.org/10.1016/j.conb.2014.09.007
- Oldfield, R. C. (1971). The assessment and analysis of handedness: The Edinburgh inventory. Neuropsychologia, 9(1), 97–113. https://doi.org/10.1016/0028-3932(71)90067-4
- Osterrieth, P. A. (1944). Le test de copie d'une figure complexe; contribution à l'étude de la perception et de la mémoire. Archives de psychologie, 30, 286–356.
- Ouellette, G. (2010). Orthographic learning in learning to spell: The roles of semantics and type of practice. Journal of Experimental Child Psychology, 107(1), 50–8. https://doi.org/10.1016/j.jecp.2010.04.009
- Panos, P. T., Porter, S. S., Panos, A., Gaines, R. N., & Erdberg, P. S. (2001). An evaluation of a case of agenesis of the corpus callosum with Rourke's nonverbal learning disorder model. Archives of Clinical Neuropsychology, 16(5), 507–21. https://doi.org/10.1093/arclin/16.5.507
- Patterson, K., & Kay, J. (1982). Letter-by-letter Reading: Psychological descriptions of a neurologial syndrome. The Quarterly Journal of Experimental Psychology Section A, 34(3), 411–41. https://doi.org/10.1080/14640748208400852
- Paul, L. K., Van Lancker-Sidtis, D., Schieffer, B., Dietrich, R., & Brown, W. S. (2003). Communicative deficits in agenesis of the corpus callosum: Nonliteral language and affective prosody. Brain and Language, 85(2), 313–24. https://doi.org/10.1016/S0093-934X(03)00062-2
- Paulesu, E., Frith, U., Snowling, M., Gallagher, A., Morton, J., Frackowiak, R. S. J., & Frith, C. D. (1996). Is developmental dyslexia a disconnection syndrome? Brain, 119(Pt 1), 143–57. https://doi.org/10.1093/brain/119.1.143
- Pennington, B. F., Filipek, P. A., Lefly, D., Churchwell, J., Kennedy, D. N., Simon, J. H., Filley, C. M., Galaburda, A., Alarcon, M., & DeFries, J. C. (1999). Brain morphometry in reading-disabled twins. Neurology, 53(4), 723–9. https://doi.org/10.1212/WNL.53.4.723
- Perry, C., Ziegler, J. C., & Zorzi, M. (2010). Beyond single syllables: Large-scale modeling of Reading aloud with the Connectionist Dual Process (CDP++) model. Cognitive Psychology, 61(2), 106–51. https://doi.org/10.1016/j.cogpsych.2010.04.001
- Petermann, F. (2018). Sprachstandserhebungstest für Kinder im Alter zwischen 5 und 10 Jahren. 3. aktualisierte und teilweise neu nomierte Auflage. Göttingen: Hogrefe.
- Planton, S., Chanoine, V., Sein, J., Anton, J.-L., Nazarian, B., Pallier, C., & Pattamadilok, C. (2019). Top-down activation of the visuo-orthographic system during spoken sentence processing. Neuroimage, 202, 116135. https://doi.org/10.1016/j.neuroimage.2019.116135
- Provazza, S., Adams, A.-M., Giofrè, D., & Roberts, D. J. (2019). Double trouble: Visual and phonological impairments in English dyslexic readers. Frontiers in Psychology, 10, 2725. https://doi.org/10.3389/fpsyg.2019.02725
- Provazza, S., Carretti, B., Giofrè, D., Adams, A.-M., Montesano, L., & Roberts, D. (2022). Shallow or deep? The impact of orthographic depth on visual processing impairments in developmental dyslexia. Annals of Dyslexia, 72(1), 171–196. https://doi.org/10.1007/s11881-021-00249-7
- Purcell, J. J., Jiang, X., & Eden, G. F. (2017). Shared orthographic neuronal representations for spelling and Reading. Neuroimage, 147, 554–567. https://doi.org/10.1016/j.neuroimage.2016.12.054
- Purcell, J. J., Shea, J., & Rapp, B. (2014). Beyond the visual word form area: The orthography-semantics interface in spelling and reading. Cognitive Neuropsychology, 31(5-6), 482–510. https://doi.org/10.1080/02643294.2014.909399
- Purcell, J. J., Wiley, R. W., & Rapp, B. (2019). Re-learning to be different: Increased neural differentiation supports post-stroke language recovery. Neuroimage, 202, 116145. https://doi.org/10.1016/j.neuroimage.2019.116145
- Raja Beharelle, A., et al. (2010). Left hemisphere regions are critical for language in the face of early left focal brain injury. Brain, 133(6), 1707–16. https://doi.org/10.1093/brain/awq104
- Rapcsak, S. Z., & Beeson, P. M. (2004). The role of left posterior inferior temporal cortex in spelling. Neurology, 62(12), 2221–9. https://doi.org/10.1212/01.WNL.0000130169.60752.C5
- Rapcsak, S. Z., Beeson, P. M., Henry, M. L., Leyden, A., Kim, E., Rising, K., Andersen, S., & Cho, H. (2009). Phonological dyslexia and dysgraphia: Cognitive mechanisms and neural substrates. Cortex, 45(5), 575–91. https://doi.org/10.1016/j.cortex.2008.04.006
- Rapp, B. C., & Caramazza, A. (1991). Spatially determined deficits in letter and word processing. Cognitive Neuropsychology, 8(3-4), 275–311. https://doi.org/10.1080/02643299108253375
- Rapp, B., & Dufor, O. (2011). The neurotopography of written word production: An fMRI investigation of the distribution of sensitivity to length and frequency. Journal of Cognitive Neuroscience, 23(12), 4067–81. https://doi.org/10.1162/jocn_a_00109
- Rapp, B., Epstein, C., & Tainturier, M. J. (2002). The integration of information across lexical and sublexical processes in spelling. Cognitive Neuropsychology, 19(1), 1–29. https://doi.org/10.1080/0264329014300060
- Rapp, B., & Lipka, K. (2011). The literate brain: The relationship between spelling and Reading. Journal of Cognitive Neuroscience, 23(5), 1180–97. https://doi.org/10.1162/jocn.2010.21507
- Rapp, B., Purcell, J., Hillis, A. E., Capasso, R., & Miceli, G. (2016). Neural bases of orthographic long-term memory and working memory in dysgraphia. Brain, 139(2), 588–604. https://doi.org/10.1093/brain/awv348
- Reed, M. A. (1989). Speech perception and the discrimination of brief auditory cues in reading disabled children. Journal of Experimental Child Psychology, 48(2), 270–92. https://doi.org/10.1016/0022-0965(89)90006-4
- Roberts, D. J., Lambon Ralph, M. A., Kim, E., Tainturier, M.-J., Beeson, P. M., Rapcsak, S. Z., & Woollams, A. M. (2015). Processing deficits for familiar and novel faces in patients with left posterior fusiform lesions. Cortex, 72, 79–96. https://doi.org/10.1016/j.cortex.2015.02.003
- Roberts, D. J., Woollams, A. M., Kim, E., Beeson, P. M., Rapcsak, S. Z., & Lambon Ralph, M. A. (2013). Efficient visual object and word recognition relies on high spatial frequency coding in the left posterior fusiform gyrus: Evidence from a case-series of patients with ventral occipito-temporal cortex damage. Cerebral Cortex, 23(11), 2568–80. https://doi.org/10.1093/cercor/bhs224
- Roeltgen, D. P., Sevush, S., & Heilman, K. M. (1983). Phonological agraphia: Writing by the lexical-semantic route. Neurology, 33(6), 755–65. https://doi.org/10.1212/WNL.33.6.755
- Rumsey, J. M., Casanova, M., Mannheim, G. B., Patronas, N., DeVaughn, N., Hamburger, S. D., & Aquino, T. (1996). Corpus callosum morphology, as measured with MRI, in dyslexic men. Biological Psychiatry, 39(9), 769–75. https://doi.org/10.1016/0006-3223(95)00225-1
- Saffran, J. R., & Coslett, H. B. (1998). Implicit vs. Letter-by-letter reading in pure alexia: A tale of two systems. Cognitive Neuropsychology, 15(1-2), 141–165. https://doi.org/10.1080/026432998381249
- Schmalzl, L., & Nickels, L. (2006). Treatment of irregular word spelling in acquired dysgraphia: Selective benefit from visual mnemonics. Neuropsychological Rehabilitation, 16(1), 1–37. https://doi.org/10.1080/09602010443000218
- Scott, C., & Byng, S. (1989). Computer assisted remediation of a homophone comprehension disorder in surface dyslexia. Aphasiology, 3(3), 301–320. https://doi.org/10.1080/02687038908248996
- Shallice, T., & Saffran, E. (1986). Lexical processing in the absence of explicit word identification: Evidence from a letter-by-letter Reader. Cognitive Neuropsychology, 3(4), 429–458. https://doi.org/10.1080/02643298608252030
- Siffredi, V., Anderson, V., Leventer, R. J., & Spencer-Smith, M. M. (2013). Neuropsychological profile of agenesis of the corpus callosum: A systematic review. Developmental Neuropsychology, 38(1), 36–57. https://doi.org/10.1080/87565641.2012.721421
- Siffredi, V., Anderson, V., McIlroy, A., Wood, A. G., Leventer, R. J., & Spencer-Smith, M. M. (2018). A neuropsychological profile for agenesis of the corpus callosum? Cognitive, academic, executive, social, and behavioral functioning in school-Age children. Journal of the International Neuropsychological Society, 24(5), 445–455. https://doi.org/10.1017/S1355617717001357
- Siffredi, V., Wood, A. G., Leventer, R. J., Vaessen, M., McIlroy, A., Anderson, V., Vuilleumier, P., & Spencer-Smith, M. M. (2019). Anterior and posterior commissures in agenesis of the corpus callosum: Alternative pathways for attention processes? Cortex, 121, 454–467. https://doi.org/10.1016/j.cortex.2019.09.014
- Smith, S. M., Jenkinson, M., Woolrich, M. W., Beckmann, C. F., Behrens, T. E. J., Johansen-Berg, H., Bannister, P. R., De Luca, M., Drobnjak, I., Flitney, D. E., Niazy, R. K., Saunders, J., Vickers, J., Zhang, Y., De Stefano, N., Brady, J. M., & Matthews, P. M. (2004). Advances in functional and structural MR image analysis and implementation as FSL. Neuroimage, 23(Suppl 1), S208–19. https://doi.org/10.1016/j.neuroimage.2004.07.051
- Smith, R. E., Tournier, J.-D., Calamante, F., & Connelly, A. (2012). Anatomically-constrained tractography: Improved diffusion MRI streamlines tractography through effective use of anatomical information. Neuroimage, 62(3), 1924–38. https://doi.org/10.1016/j.neuroimage.2012.06.005
- Snowling, M. J. (1981). Phonemic deficits in developmental dyslexia. Psychological Research, 43(2), 219–34. https://doi.org/10.1007/BF00309831
- Sotiropoulos, A., & Hanley, J. R. (2017). Lexical decision performance in developmental surface dysgraphia: Evidence for a unitary orthographic system that is used in both Reading and spelling. Cognitive Neuropsychology, 34(3-4), 144–162. https://doi.org/10.1080/02643294.2017.1368468
- Spinelli, D., Angelelli, P., De Luca, M., Di Pace, E., Judica, A., & Zoccolotti, P. (1997). Developmental surface dyslexia is not associated with deficits in the transient visual system. Neuroreport, 8(8), 1807–12. https://doi.org/10.1097/00001756-199705260-00003
- Stickles, J., Schilmoeller, G., & Schilmoeller, K. (2002). A 23-year review of communication development in an individual With agenesis of the corpus callosum. International Journal of Disability, Development and Education, 49(4), 367–383. https://doi.org/10.1080/1034912022000028349
- Strother, L., et al. (2017). An fMRI study of visual hemifield integration and cerebral lateralization. Neuropsychologia, 100, 35–43. https://doi.org/10.1016/j.neuropsychologia.2017.04.003
- Szaflarski, J. P., Schmithorst, V. J., Altaye, M., Byars, A. W., Ret, J., Plante, E., & Holland, S. K. (2006). A longitudinal functional magnetic resonance imaging study of language development in children 5 to 11 years old. Annals of Neurology, 59(5), 796–807. https://doi.org/10.1002/ana.20817
- Tallal, P. (1980). Auditory temporal perception, phonics, and Reading disabilities in children. Brain and Language, 9(2), 182–98. https://doi.org/10.1016/0093-934X(80)90139-X
- Tallal, P., Miller, S., & Fitch, R. H. (1993). Neurobiological basis of speech: A case for the preeminence of temporal processing. Annals of the New York Academy of Sciences, 682(1), 27–47. https://doi.org/10.1111/j.1749-6632.1993.tb22957.x
- Taylor, J. S. H., Rastle, K., & Davis, M. H. (2013). Can cognitive models explain brain activation during word and pseudoword reading? A meta-analysis of 36 neuroimaging studies. Psychological Bulletin, 139(4), 766–791. https://doi.org/10.1037/a0030266
- Temple, C. M., Jeeves, M. A., & Vilarroya, O. O. (1990). Reading in callosal agenesis*1. Brain and Language, 39(2), 235–53. https://doi.org/10.1016/0093-934X(90)90013-7
- Tournier, J. D., Calamante, F., & Connelly, A. (2013). Determination of the appropriate b value and number of gradient directions for high-angular-resolution diffusion-weighted imaging. NMR in Biomedicine, 26(12), 1775–86. https://doi.org/10.1002/nbm.3017
- Tournier, J.-D., Smith, R., Raffelt, D., Tabbara, R., Dhollander, T., Pietsch, M., Christiaens, D., Jeurissen, B., Yeh, C.-H., & Connelly, A. (2019). MRtrix3: A fast, flexible and open software framework for medical image processing and visualisation. Neuroimage, 202, 116137. https://doi.org/10.1016/j.neuroimage.2019.116137
- Tovar-Moll, F., Monteiro, M., Andrade, J., Bramati, I. E., Vianna-Barbosa, R., Marins, T., Rodrigues, E., Dantas, N., Behrens, T. E. J., de Oliveira-Souza, R., Moll, J., & Lent, R. (2014). Structural and functional brain rewiring clarifies preserved interhemispheric transfer in humans born without the corpus callosum. Proceedings of the National Academy of Sciences, 111(21), 7843–8. https://doi.org/10.1073/pnas.1400806111
- Tsapkini, K., & Rapp, B. (2010). The orthography-specific functions of the left fusiform gyrus: Evidence of modality and category specificity. Cortex, 46(2), 185–205. https://doi.org/10.1016/j.cortex.2009.02.025
- Turk, A. A., Brown, W. S., Symington, M., & Paul, L. K. (2010). Social narratives in agenesis of the corpus callosum: Linguistic analysis of the Thematic Apperception Test. Neuropsychologia, 48(1), 43–50. https://doi.org/10.1016/j.neuropsychologia.2009.08.009
- Valdois, S., Bosse, M. L., & Tainturier, M. J. (2004). The cognitive deficits responsible for developmental dyslexia: Review of evidence for a selective visual attentional disorder. Dyslexia, 10(4), 339–63. https://doi.org/10.1002/dys.284
- Vandermosten, M., Boets, B., Poelmans, H., Sunaert, S., Wouters, J., & Ghesquiere, P. (2012a). A tractography study in dyslexia: Neuroanatomic correlates of orthographic, phonological and speech processing. Brain, 135(3), 935–48. https://doi.org/10.1093/brain/awr363
- Vandermosten, M., Boets, B., Wouters, J., & Ghesquière, P. (2012b). A qualitative and quantitative review of diffusion tensor imaging studies in Reading and dyslexia. Neuroscience & Biobehavioral Reviews, 36(6), 1532–52. https://doi.org/10.1016/j.neubiorev.2012.04.002
- Vellutino, F. R., Scanlon, D. M., & Lyon, G. R. (2000). Differentiating between difficult-to-remediate and readily remediated poor readers. Journal of Learning Disabilities, 33(3), 223–38. https://doi.org/10.1177/002221940003300302
- Wang, L. C., & Yang, H. M. (2014). Classifying Chinese children with dyslexia by dual-route and triangle models of Chinese Reading. Research in Developmental Disabilities, 35(11), 2702–13. https://doi.org/10.1016/j.ridd.2014.07.001
- Wechsler, D. (2017). Wechsler Intelligence Scale for Children - Fifth Edition. Deutsche Bearbeitung von Franz Petermann. Frankfurt am Main: Pearson.
- Weekes, B., & Coltheart, M. (1996). Surface dyslexia and surface dysgraphia: Treatment studies and their theoretical implications. Cognitive Neuropsychology, 13(2), 277–315. https://doi.org/10.1080/026432996382033
- Weiss-Croft, L. J., & Baldeweg, T. (2015). Maturation of language networks in children: A systematic review of 22years of functional MRI. Neuroimage, 123, 269–81. https://doi.org/10.1016/j.neuroimage.2015.07.046
- Werker, J. F., & Tees, R. C. (1987). Speech perception in severely disabled and average reading children. Canadian Journal of Psychology / Revue Canadienne de Psychologie, 41(1), 48–61. https://doi.org/10.1037/h0084150
- Wright, A., & Booth, R. (2023). Neuropsychological profiles of children with agenesis of the corpus callosum: A scoping review. Developmental Medicine & Child Neurology, 65(9), 1141–1149. https://doi.org/10.1111/dmcn.15532
- Wybrow, D. P., & Hanley, J. R. (2015). Surface developmental dyslexia is as prevalent as phonological dyslexia when appropriate control groups are employed. Cognitive Neuropsychology, 32(1), 1–13. https://doi.org/10.1080/02643294.2014.998185
- Yeatman, J. D., Dougherty, R. F., Rykhlevskaia, E., Sherbondy, A. J., Deutsch, G. K., Wandell, B. A., & Ben-Shachar, M. (2011). Anatomical properties of the arcuate fasciculus predict phonological and Reading skills in children. Journal of Cognitive Neuroscience, 23(11), 3304–17. https://doi.org/10.1162/jocn_a_00061
- Zaidel, E., Clarke, J., & Suyenobu, B. (1990). Hemispheric independence: A paradigm case for cognitive neuroscience. In A. B. Scheibel, & A. F. Wechsler (Eds.), Neurobiology of higher cognitive functions (pp. 297–355). New York: Guilford Press.
- Žarić, G., Correia, J. M., Fraga González, G., Tijms, J., van der Molen, M. W., Blomert, L., & Bonte, M. (2017). Altered patterns of directed connectivity within the reading network of dyslexic children and their relation to reading dysfluency. Developmental Cognitive Neuroscience, 23, 1–13. https://doi.org/10.1016/j.dcn.2016.11.003
- Zimmermann, P., Gondan, M., & Fimm, B. (2002). KiTAP: Test for attentional performance in children. Herzogenrath: Psytest.
- Zoccolotti, P., De Luca, M., Di Pace, E., Judica, A., Orlandi, M., & Spinelli, D. (1999). Markers of developmental surface dyslexia in a language (Italian) with highgrapheme–phoneme correspondence. Applied Psycholinguistics, 20(2), 191–216. doi:10.1017/S0142716499002027