Abstract
Long-term research on RNA interference led to an unfathomed understanding of the mechanism of siRNA-mediated silencing and finally siRNA has emerged as a powerful therapeutic tool. With siRNAs virtually every gene in the human genome contributing to a disease becomes amenable to regulation, thus opening unprecedented opportunities for drug discovery. siRNA has a well-established role as a tool for in vitro target screening and validation, besides these recent progresses of siRNA delivery in vivo, this has raised more expectations for siRNA-based drugs as the up-and-coming ‘magic bullet’. Although a plethora of articles have been published with siRNA, the fundamentals of siRNA-mediated gene silencing and transforming the functional genomics to novel therapeutics are reviewed in this article with consideration to present hurdles as a new generation challenge.
1. Introduction
Completion of Human Genome Project (HGP), a landmark achievement with understanding for networking and coding of encrypt message of nucleic acid has turned out to be a major breakthrough in every arena of biomedical research. Enormous empathies of HGP have not only led us to understand the molecular aspect of life-threatening disease but also explore the horizon of therapeutic applications. Based on the sequencing of the human genome and blossoming understanding of the molecular causes of diseases, the conjecture of silencing of virulence gene expression appears to be a lucrative approach for handling an array of clinical pathologies. Knowledge about genetic footprint and availability of sequenced genome map for many key model organisms made it possible to silence the gene expression in a sequence-specific manner for investigation of gene function. Small interfering RNA (siRNA) is one of the most recent accessions to the wide repertoire of nucleic acid molecules used to silence gene expression.
Nucleic acid-based therapeutics can symbolize a fundamentally new way to treat most puzzled human disease by addressing targets which are otherwise ‘undruggable’ with existing medicines. The uncovering of long double-stranded RNA (dsRNA) mediated RNAi in the worm and the accompanying demonstration of silencing mediated by small interfering RNA (siRNA), operates in mammalian cells aggrandize research to discover new mechanisms of gene silencing (Elbashir, Lendeckel, & Tuschl, Citation2001; Fire et al., Citation1998). It has revolutionized our understanding of endogenous mechanisms of gene regulation and providing powerful new tools for biological research and drug discovery.
Further in 2002, mRNA-transcript-targeting strategy came to the attention of the non-scientific world by the technique designated as the ‘breakthrough of the year’ by Science (Couzin, Citation2002). Subsequent research has elevated exponentially and as revealed by a number of publications led to a more unfathomed understanding of the underlying mechanism of siRNA-mediated silencing and siRNA has emerged as a potential therapeutic tool in many applications.
2. Fundamentals of gene silencing
Current models of RNAi as shown in Figure suggest that the pathway is driven by siRNA and miRNA which operate at nucleoplasmic to cytoplasmic level (de Fougerolles, Vornlocher, Maraganore, & Lieberman, Citation2007; Kim & Rossi, Citation2007; Meister & Tuschl, Citation2004). Current models of RNAi for miRNA suggest that pre-micro RNA is processed to form initial 70 or more bp long stem loop or short hairpin form of dsRNAs encoded in intragenic region of nucleus by Drosha (He & Hannon, Citation2004). After processing, this dsRNA (>26 bp) are transported to cytoplasm via exportin-5 (Yi, Qin, Macara, & Cullen, Citation2003). In cytoplasm this is further shortened and cleaved by an RNase III like enzyme, termed as Dicer (DCR), while siRNA pathway starts with DCR-mediated cleavage of long double-stranded cytoplasmic RNA to generate 21–23 nt fragments (Bernstein, Caudy, Hammond, & Hannon, Citation2001; Ketting et al., Citation2001). The DCR cleaved double-stranded siRNAs and miRNAs to convert into single-stranded RNAs. This is achieved by RNA-induced silencing complex (RISC) assembly via RNA–protein and protein–protein interactions mainly by RISC loading complex (RLC) (Liu et al., Citation2003). This RISC assembly is initially double-stranded and required its unrolling to be functional RISC assembly. This task is often mediated by multi enzyme complex which include RNA helicase and Argonaute 2 (AGO2) (Liu et al., Citation2004). It is presumed that an ATP dependent RNA helicase recognizes these short duplexes and resolves the siRNA duplex into two single-stranded RNAs but still RNA helicase responsible for this unwinding has not been identified. Once unwound, AGO2 associate with RISC complex and binds to dsRNA. Finally it leads to sequence-specific cleavage of sense strand and gets separated from complex to be activated RISC complex containing guide RNA (Matranga, Tomari, Shin, Bartel, & Zamore, Citation2005). This activated RISC complex then searches for homologous RNA sequences and leads to its cleavage in sequence-specific manner. Any complementary mRNA strand binds to the siRNA and the complex get cleaves at the nucleotides between 10 and 11 of the guide strand from 5′-end. siRNAs have two unusual properties that determine its function are 2 nt 3′-overhangs region and availability of free 5′-phosphate groups. Compared to that miRNA is less specific as it blocks translation of any complementary mRNA strand (Elbashir, Martinez, Patkaniowska, Lendeckel, & Tuschl, Citation2001). Finally, RISC is liberated from the cleaved mRNA and is recycled to perform multiple rounds of catalysis and thus, RISC is most potent in exerting the silencing effect (Coburn & Cullen, Citation2003).
3. siRNA as therapeutics
The prime goal of RNAi-based therapeutics is to achieve efficient gene silencing by site-specific cleavage in mRNA with high degree of accuracy. In existing pathway of silencing it is possible by using two approaches; either by use of viral vectors to express short hairpin RNA (shRNA) that resembles miRNA precursors or alternatively by injection of siRNAs that mimic the dicer cleavage product into the cytoplasm. Significant advantage associated to siRNA is that it can bypass the earlier steps in the RNAi silencing pathway. It is possible to load directly siRNA onto the AGO2-RISC by the use of vectors containing Pol II or Pol III promoters. This is because an RNAi phenomenon is based on sequence recognition by the siRNA, thus mimic response is obtained which can silence other genes with similar sequences. This phenomenon is referred to as an off-target effect, and the growing evidence from large-scale knockdown experiments indicates that the off-target silencing is induced by the base pairing between the seed region at positions 2–8 nt from the 5′ end of the RISC-loaded siRNA strand and its complementary sequences in the 3′ UTR of the unrelated mRNAs. Thus, the concept of siRNA as therapeutics starts with its design and involves in vitro studies to determine its silencing efficacy to off-target effect. As natural siRNA structure is easily susceptible to nuclease attack. It is a better option for targeting by design of new synthetic siRNAs. Present day progress in computational biology made available various tools such as siDirect 2.0 and online design server which helps in designing the synthetic siRNAs (Naito, Yoshimura, Morishita, & Ui-Tei, Citation2009). As synthetic siRNA can mimic the naturally occurring RNAi pathway in more foreseeable and coherent way, it seems to be more attractive as therapeutics. Furthermore as synthetic siRNA enter the RNAi pathway in later stage, they are less prone to degradation by endogenous miRNAs mediated regulation (Grimm et al., Citation2006). As a consequence, synthetic siRNAs emerged as the most advanced therapeutics in preclinical and clinical studies.
4. siRNA as a potent drug
Present challenges for the development of siRNA-based drugs required to overcome various obstacles such as its ease of degradation by endonuclease, stimulation of immunoregulatory response to its stability in human plasma, and various off regulatory mechanism of control (Choung, Kim, Kim, Park, & Choi, Citation2006; Hornung et al., Citation2005; Layzer et al., Citation2004). To transform siRNA to drug, four major facet of candidate molecule has to be evaluated thoroughly, which includes potency, specificity, stability, and pharmacokinetic profile.
4.1. Enhancing specificity through potency
Potency and specificity are related issues. In RNAi screening applications based on siRNA libraries can improve specificity but decrease the efficiency due to off-target effects (Semizarov et al., Citation2003). In vitro experiment is suggesting that siRNAs can easily silence expression of any gene at nanomolar or less concentration and are capable of inducing cleavage of up to 95% of the target mRNA. But the main task is to find out the correct potent one from the list of candidates. This is just like finding a needle in husk. In silico approach by bioinformatics software and algorithms are available to predict effective siRNA based on common features with limitations over in vitro approach (Pei & Tuschl, Citation2006; Reynolds et al., Citation2004). Reports have shown that incorporation of slightly longer siRNA found to have better silencing but longer siRNA is prone to activation of immune response and often more complicated to design (Kim et al., Citation2005; Reynolds et al., Citation2006). It is possible to manipulate strand for better selection by making a single nucleotide substitution at the end of the duplex to alter the relative binding of the ends (Schwarz et al., Citation2003). By designing a siRNA with a mismatch at the 5′ end of the intended active strand that favors its incorporation into RISC, it may be possible to increase the likelihood of identifying a potent duplex (Schwarz et al., Citation2003).
4.2. Specificity
Specificity of siRNAs is an important factor of newly discovered phenotypes from large-scale RNAi screening. The RNAi-mediated gene silencing is highly sequence-specific (Khvorova, Reynolds, & Jayasena, Citation2003). Several off-target effects associated to regulation often put down high specificity of siRNA. The growing evidence from large-scale knockdown experiments indicates that the off-target silencing is induced by the base pairing between the seed region at positions 2–8 nt from the 5′ end of the RISC-loaded siRNA strand and its complementary sequences in the 3′ UTR of the unrelated mRNAs as depicted in Figure (Lin et al., Citation2005; Scacheri et al., Citation2004).
Figure 2. Pictorial representation of naked siRNA structure with its important feature of 7 bp seed region, its cleavage site at position between 10 and 11 plus its two double overhang nucleotide at 3′ end. The passenger strand and the guide strand are represented in blue and orange, respectively.
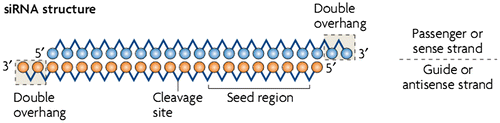
Recently, it was revealed that the capability of siRNAs to induce seed dependent off-target effect is highly correlated to the melting temperature (Tm) of the seed target duplex (Naito et al., Citation2009). Fundamentally, problems can be overcome by limiting Tm of duplex and careful design of siRNA by in silico approach or by chemical modification of native structure.
4.3. Stability
Major hurdles associated to siRNA as drug are the few minutes turn over time of naked siRNA in human plasma and the susceptibility of 3′ end of naked siRNA to exo and endonuclease (Choung et al., Citation2006). Thus, the stability of siRNA-based drugs is a prerequisite for clinical trials. Therefore in order to be successful in the transformation of siRNA from a powerful and well-established tool for manipulating gene expression in mammalian cells into a functional therapeutic molecule, it is necessary to apply chemical modification of the siRNA. Basically all this chemical modification under practice is based on antisense oligonucleotide (ASON) and ribozyme-based therapeutics (Manoharan, Citation1999; Opalinska & Gewirtz, Citation2002). With great array of modification, currently available are the 2′-O-methyl nucleotides and the phosphorothioate (P=S) linkage, in which a non-bridging oxygen of the phosphodiester between adjacent nucleotides in the oligomer chain is replaced by a sulfur atom (Eckstein, Citation2002). Alternation by methylation of 2′-O position of sugar moiety confers the endonuclease resistance, while introduction of sulfur atom at 3′ end protects it against endonuclease as shown in Figure (Allerson et al., Citation2005; de Fougerolles, Manoharan, Meyers, & Vornlocher, Citation2005).
4.4. Pharmacokinetics
Rapid elimination of siRNA by renal excretion and its few minutes of half-life are the major rate-limiting factors to be as a potent drug. Besides this, another associated obstacle is its slow degradation from serum by RNAse i.e. half-life is only 30 min. The half-life of unmodified siRNAs in other body fluids is not available which necessitates the focus research. As blood is considered as highly nuclease rich environment, it necessitates evaluating the stability of siRNA in blood. It is possible to extend half-life of siRNAs under plasma substantially (from minutes to days) by forming complexes with other molecules or by incorporating it into particles to bypass the renal filtration cutoff of ~ 50 kDa (Dykxhoorn & Lieberman, Citation2006). However, these modifications lead to reduced intracellular silencing efficiency. Thus, the appropriate modifications of siRNA to increase the half-life and its resistance towards degradation with same or improved potency are also focused areas for biotherapeutics development.
5. siRNA designing approaches
RNAi-mediated silencing is clearly dependent on the function and specificity of the siRNA. The efficacy of silencing is governed by species-specific distinction. Design, production, and delivery of the functional silencing intermediates largely determine these species-specific distinctions. Thus, the fundamental challenge for successfully implementing RNAi in mammalian systems rests on designing specific and potent siRNAs.
The siRNA design is a truly empirical approach started with screening of phenotype showing better silencing by conventional approach. It further inflates to better efficacy by use of site-specific rational designing and in silico approach. Thus, the understanding of conventional method is the root of further advancements such as more target-specific high-throughput approach, automated designing, and approach of designing in silico environment.
5.1. Conventional designing
The most widely used strategy with conventional designing is selection of 21 base mRNA target sequences by in vitro biochemical studies from Drosophila and mammalian cell lysates (Elbashir, Martinez et al., Citation2001; Martinez, Patkaniowska, Urlaub, Luhrmann, & Tuschl, Citation2002). The conventional method for designing begins with the search of the coding sequence for regions that are most likely to be free of translational or regulatory proteins. Generally it is 100 bases downstream of the start codon and followed by 19 base sequences with 30–70% G + C content sequence motifs characterized by preceding an AA or NA dinucleotide. The subsequent antisense strand would be completely complementary to the target. On average 60–70% of duplexes identified by conventional design method silence their target by at least 50–70% but there exist significant variability in silencing efficiencies (Aza-Blanc et al., Citation2003; Bernstein et al., Citation2001).
5.2. Rational design of siRNA
Duplex functionality and specificity are the main objectives of siRNA design strategies largely determined by G + C content, low internal stability of the 5′ antisense end, and base preference. An adenosine and not cytosine at 19 position of the sense strand is preferred. This probably plays a role in RISC duplex unwinding and activation step. While base preferences at position 10 to uridine, at position 3 to adenosine, and at position 13 to any base except guanosine are thought to play a role in catalytic cleavage of the target (Khvorova et al., Citation2003). Several efforts attempted to check the effect of these attributes alone and with combination. The studies showed that when applied individually, no single attribute is sufficient to ensure silencing. However, when all eight factors are integrated into a single algorithm to evaluate a test panel of 180 duplexes targeting firefly luciferase and human cyclophilin, these eight criteria successfully eliminated all non-functional duplexes.
Conventional design studies by a number of laboratories show that addition of non-or semi-functional siRNA to functional duplexes led to silencing by attenuation (Holen, Amarzguioui, Babaie, & Prydz, Citation2003; McManus et al., Citation2002). This is due to saturation of the silencing machinery. It turns out to be an important rate-limiting step due to the concentration or number of sequences directed against a single or multiple targets. Rationally designed siRNA is the solution to this problem. Significant level of silencing could be achieved with low or sub nanomolar concentrations which shows even on an average or higher potency (and longevity) than that of conventionally designed counterparts.
The importance of rational design related to its off-target effects became clear by microarray analysis which monitors the genome-wide effects of siRNA-induced silencing. However, at closer inspection, the level of off-target down regulation was found 2–3 folds below that of normal gene expression, thus it is functionally insignificant. Moreover among the modulated genes, the target gene remained suppressed while the off-target genes recovered expression over the course of the study. Therefore, these results further support the application of in silico methods that serves to minimize factors contributing to off-target effects such as partial sequence identity, secondary structure, or binding affinities.
5.3. High-throughput and automated design of siRNAs
Large-scale collections of RNAi constructs have been successfully generated for studies in Arabidopsis, C. elegans, Drosophila, mouse cells, and human cells so far. It is now possible that every mRNA in a cell can be screened by knockout in a high-throughput phenotypic or functional screen. An RNAi technique makes it possible to examine the significance of hundreds or thousands of different genes whose expression is known to be up-regulated in a disease. But, still the question to be answered is the identification of interactions that exist between genes and biological pathways that address these logistical issues. Genome-wide siRNA libraries have been synthesized to determine the function of individual genes in C. elegans and Drosophila (DasGupta & Perrimon, Citation2004; Poulin, Nandakumar, & Ahringer, Citation2004). The realistic challenge is the design and production of such 100,000 siRNA duplexes and genome-wide libraries, which requires a combination of good design and informatics tools in a time frame. This could be achieved by a combination of high-throughput approach and automation.
In order to silence each gene in the genome using RNAi, the first step is to create the appropriate library of dsRNA and to recognize this siRNA library. Several different approaches are employed in generating siRNA libraries such as enzymatically generated siRNA library, synthesized siRNA library, and random siRNA library. Enzymatic approach is recently encountered which uses various DNase or restriction enzymes to produce identical fragment of source DNA (such as clone of cDNA library) which is followed by MmeI digestion (i.e. type II restriction endonuclease that cleaves 18 and 20 nt away from its recognition site and leaves a 2-nt 3′overhang.). The fragmented DNA was further converted to a mixture of 20 bp fragments and then attached to the hairpin linker. Finally, a second linker is ligated with the MmeI-generated termini. By using DNA polymerase the short-stranded DNA hair-pin structure is converted to linear DNA with two copies of the inserts in a head-to-head palindrome as shown in Figure (Zhao et al., Citation2005). These fragments are used to generate shRNA expression constructs after cloned adjacent to an RNA Pol III-driven promoter (Shirane et al., Citation2004). This approach allows selection of active siRNAs to target any known or unknown gene. Furthermore, such a library will allow the identification of many new gene functions by using the right selection pressure after introduction of the siRNA library into cells. However, due to the complicated technical manipulations, its use is limited.
Another popular but more expensive approach is synthesized siRNA library design using plausible knowledge from identification of encoded gene against all possible human genes. It is mainly rational designing of siRNA with the use of retroviral-based vector and follows all rules of designing of rational one. Recently siRNA was designed based on neural network algorithm (Huesken et al., Citation2005). This approach has led to a dramatic increase in targets by more than 24,000 human genes. The advantages of the approach include; it contains only useful siRNAs, eliminating most of the non-functional siRNAs, therefore it is an ideal choice to carry out genome-wide screen in mammalian cells. But its downfall is that it relies on current understanding of genome information and the unknown genes and gene variants might be excluded from the starting point.
From moving classical to advanced a more economical approach is to design siRNA randomly against any gene of interest by random siRNA library. The present approach uses plasmid vector containing two convergent RNA pol III promoters to drive the expression of both strands of the siRNA from a single targeting sequence region. Based on this type of vector, a new type of siRNA library is constructed by integrating 19mer fully randomized DNA sequences into siRNA encoding vectors. The most fascinating part of this approach is improved complexity with more economical research but the real challenge is to identify the primary target of functional siRNA (Zhao et al., Citation2005).
A combination of high-throughput and automated strategy is the best possible way to speed up RNAi-based clone construct and its screening. Recently the use of design based on high-throughput synthesis in combination with a Custom Laboratory Information Management System (LIMS) is in practice. Various active partners include companies like Tecan, Perkin Elmer, Wellesley, Hamilton, Reno, Beckman and Fullerton which have designed advance versions of working platform. BioRobot 3000 system from Qiagen allows synthesis of siRNA on dual 96-well plate synthesizers, which enables re-arraying of sense and antisense strand, its molar normalization, and annealing of both strands with real-time monitoring of coupling efficiency.
5.4. In silico designing
The employment of sophisticated in silico procedures that eliminate unnecessary screening is the key advance in the field of RNAi research and therapeutic venues. In silico designing is merely rational deigning with consideration of various factors such as thermodynamic profiling, possible mismatching, %G + C content, and binding affinities. For the same purpose various software like sfold, siDIRECT, and siDirect 2.0 are available. The study is based on some empirical design rule developed by the Tuschl laboratory (Elbashir, Lendeckel et al., Citation2001). These rules are summarized as follows:
siRNA duplexes should be composed of 21 nt sense and 21 nt antisense strands, paired so as to each have a 2 nt 3′ dTdT overhang;
The targeted region is selected from a given cDNA sequence beginning 50 to 100 nt downstream of the start codon (3′ UTRs also have been successfully targeted);
The target motif is selected in the following order of preferences:
NAR (N 17) Y NN, where N is any nucleotide, R is purine (A or G), and Y is pyrimidine (C or U);
AA (N19) TT;
NA (N21);
Nucleotides 1–19 of the sense siRNA strand correspond to positions 3–21 of the 23-nt target motif;
The target sequence is selected to have around 50% G + C content; and
Selected siRNA sequences should be aligned against EST libraries to ensure that only one gene will be targeted.
The best siRNA design has become an intense competition between academic research groups as well as commercial providers of siRNA. Besides it, several servers also allow online designing of siRNA. In general, the existing tools adopt a set of rules and assign each rule an equal or different score, each siRNA sequence is scored against every rule and only those sequences scoring above a predefined point are selected as valid siRNA sequences. Such a simple selection procedure does not discriminate between the critical rules for the validity of a siRNA sequence (must be met) and rules that can only affect the efficiency of the siRNA sequence. Meanwhile, these web-based tools only provide limited flexibility to users and also do not reorganize the selection rules based on preferences of user or recent research data.
6. siRNA and mutation targeting strategies
Mutation, the most studied phenomenon have often found to be associated as a cause of a number of diseases and disorders by either changing, deleting, gaining, or reshuffling of nucleotide or part of genome. Disease or disorder exerts their effect via either gaining of function or by mode of dominant negative. It is possible to overcome effect by designing or targeting siRNA specific to mutant transcript or SNP variant of target transcript. To achieve this task several targeting strategies have been in practice (Figure ) and are described below:
Figure 5. Schematic representation of 4 different siRNA mutation targeting strategies as: (a) Direct Targeting- siRNA design specific to mutant one (b) Indirect targeting – siRNA design as SNP variant of mutant transcript (c) Exon specific – siRNA targeting to only specific exon as blue one shown here (d) Exon skipping – siRNA design to skip exon but boundaries of exon are targeted.
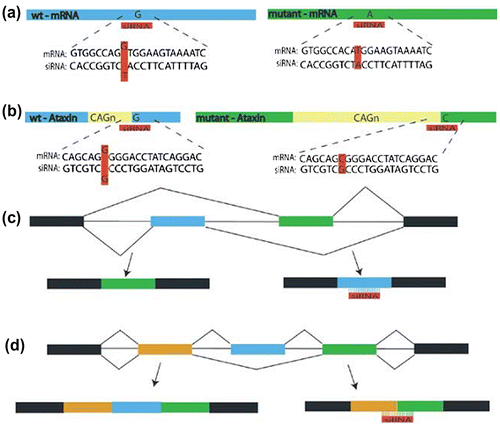
6.1. Direct mutation targeting
It is viable to directly target the siRNA to its mutant transcript which is supported and advantageous by its sequence difference to the wild one. For this purpose, siRNA can be designed to perfectly match its mutant transcript but have a slight mismatch to wild type allele to mediate site-specific degradation of mutant allele. Strategy was successfully employed in cellular model of dominant Tau mutant which causes formation of neurofibrillary tangles and neuronal degeneration in Fronto Temporal Dementia with Parkinsonism (FTDP-17) linked to chromosome 17 (Victor et al., Citation2003). As a strategy, 21–24 nt siRNA was designed in a fashion to target against one of four central positions to four different missense mutants (Figure ). Out of four, only one was successfully found to show efficient silencing. Further improvement of specificity was attempted by constructing siRNA with second mismatch either at upstream or 3′ nucleotide at downstream of 1st construct. As an optimistic result it has shown specific degradation of mutant Tau without any apparent loss of wild type protein (Victor et al., Citation2003).
6.2. Indirect mutational targeting
If mutant transcript is quite similar to wild type as mentioned in direct approach, then it is also plausible to cause degradation of wild type. Therefore, it is better to design siRNA linked to its mutant transcript but as SNP variant which specifically target only linked mutant but not affecting the wild type. This condition is often found in case mutation linked to microsatellite region. As smaller number of repeats are available it is quite difficult to design siRNA.
6.3. Exon-specific targeting
Often splicing mechanism have been reported to wrongly splice exon causing formation of truncated version of target mRNA. This leads to common lethal mutation and finally there is no functional transcript. Some reports also showed that specifically some exon site of gene was not spliced correctly and that leads to improper gene functioning (Faustino & Cooper, Citation2003). Exon-specific siRNA was tested by targeting the variable exons of the Drosophila Dscam exon four cluster in which only one of 12 variable exons are spliced in to mRNA. Instead of using small, 21–23 nt RNA duplexes, ‘RNA triggers’ are composed of dsRNAs corresponding to an entire exon sequence. Both sets of RNA trigger specific and efficient degradation of their respective isoform in Drosophila S2 cells (Celotto & Graveley, Citation2002).
6.4. Exon skipping targeting
Failure of alternative splicing often leads to synthesis of exon with intron or sometimes does not splice some of exon site. Under these circumstances siRNA can design to target only boundaries of exon but not exon (Faustino & Cooper, Citation2003). This aberrant splicing event can lead to the production of dominant negative proteins. It was reported with growth hormone deficiency (GHD) type II, where mutation affects splicing of exon 3 leading to the production of a smaller, 17.5 kDa isoform. In this dominant form of GHD, dominant-negative 17.5 kDa isoform exerts effect on secretion of the biologically active 22 kDa growth hormone isoform. Since the 17.5 kDa isoform results from skipping of exon 3, it can be targeted with siRNAs directed against the unique exons 2–4 junction (Figure (d)). This targeting approach was used to degrade specifically transcripts encoding the 17.5 kDa isoform with no apparent effect on either the 22 kDa isoform or another, smaller 20 kDa isoform (Ryther, Flynt, Harris, Phillips, & Patton, Citation2004).
7. In vivo progress towards use of siRNA
In vitro gene silencing using of siRNA in mouse model to reveal the suppression of hepatic expression was for the first time reported by McCaffrey et al. in 2002 (MaCaffrey et al., Citation2002). The luciferase expressing plasmid constructs of synthetic siRNA were quite anticipating and displayed more than 80% decrease in hepatic luciferase expression. With this finding, researchers have attempted trials for therapeutic role of siRNA using mouse model against fulminant hepatitis in 2003. In vivo progress towards use of siRNA has continuously grown. The first in vivo trial was reported to silence acute liver failure (ALF). About 80% of FAS antibodies treated siRNA found to increase the survival days from 3 days in control to 10 days, which was considered to be a good prognosticating outcome for future clinical trial of siRNA.
Simultaneous research was geared up on pharmacokinetic properties and circumvents challenges of siRNA delivery. Initial studies carried out by various research groups shows that systemic administration of siRNA readily accumulates in liver, spleen, and kidneys, whereas other tissues, including the brain show only moderate to low uptake (Braasch et al., Citation2004). Pharmacokinetic respiratory mucosa as delivery site was found to show more reliable results and also confer advantages of bypassing extensive trapping, metabolism, and excretion by liver and kidneys. Due to higher accessible site to easy uptake of target by inhalation, it seems to be particularly well suited for the uptake of RNAi-based drugs. Systematic siRNA delivery with better pharmacokinetic local administration of siRNAs was tested in a variety of animal studies. For illustration, intraperitoneally delivery of anti(TNF-α) siRNA with LPS for inducing the septic-shock-like syndrome in mice with decreased TNF-α concentration in peritoneal lavage, successfully protected the animals from septic-shock-induced death (Sorensen, Leirdal, & Sioud, Citation2003). In another in vivo study repeated intratumoral administration of siRNA targeting to CSF-1 (colony-stimulating factor-1) in a mammary carcinoma xenograft model led to selective down-regulation of target protein expression in tumor lysates with suppression of tumor growth in mice (Sorensen et al., Citation2003).
Chemical modification of oligonucleotide to enhance its activity and stability was studied in vivo with different route of administration for better cellular uptake with stability (Behlke, Citation2008; Bramsen & Kjems, Citation2011; Gaglione & Messere, Citation2010; Kenski et al., Citation2012). Using chemically synthesized siRNA targeted to RSV (Respiratory Syncytial Virus) or PIV (Para Influenza Virus) by intranasal administration to infected mice were found to prevent the viral replication of RSV and PIV. The titer of RSV and PIV was reduced to 99.98 and 99%, respectively. Following its routine assessment for viral-induced pathology, lungs of animals treated by siRNA were found virtually protected from tissue damage with a pulmonary pathology score similar to uninfected animals. Even when treatment was delayed for days, intranasal siRNA improved pulmonary pathology remarkably and ameliorated clinical symptoms such as respiratory distress. Importantly, this success was a direct consequence of RNAi and was apparently not mediated by triggering a non-specific IFN (interferon) response (Bitko, Musiyenko, Shulyayeva, & Barik, Citation2005).
Various modifications that facilitate entry of siRNA into cells or improve biodistribution of siRNA have been attempted (Manoharan, Citation2002). For example, conjugation of cholesterol to a siRNA can improve serum protein binding and pharmacokinetics. siRNA conjugated with lipophilic derivates of cholesterol, lithocholic acid, or lauric acid has been also synthesized. With Cholesterol conjugated siRNA to the 3′ end of sense strand by means of a pyrrolidine linker (chol–siRNA) has dramatically prolonged in vivo half-life to 95 min (Soutschek et al., Citation2004). In a hallmark study using chol-siRNAs for the first time to target apoB (apolipoprotein B) in mice by means of systematic administered siRNA to liver not only showed diminished apoB protein levels but subsequently lowered blood cholesterol. The silencing of apoB was not limited to liver and serum but also reduced significantly in the jejunum (Soutschek et al., Citation2004). The advance research of lipid-based formulation of siRNA also proved to be useful for in vivo use analogos with non-viral transfection strategies. In one study, systemic administration of synthetic siRNAs complexed with polyethylenimine resulted in delivery to subcutaneous tumor xenograft and Her-2 target for down-regulation in mice (Urban-Klein, Werth, Abuharbeid, Czubayko, & Aigner, Citation2005). Such formulations have reported to cause toxicity in past (Tousignant et al., Citation2000) and often induce a profound IFN response in vitro and in vivo (Judge et al., Citation2005).
Viral-mediated delivery of gene and nucleic acid has been quite significant in the field of biomedical and molecular biology due to its extraordinary replication potential and its suitability as vector for efficient and target-specific delivery. Application of retroviral, adenoviral, and AAV (adeno-associated viral) have been demonstrated successfully. These have recognized its potential and feasibility of genome modification with RNAi in vivo. Very recently, using lentiviral vector via intraspinal injection of shRNA producing plasmids were delivered to neurons in a transgenic mouse model of familial ALS. Results revealed silencing of mutated SOD1 (superoxide dismutase 1) substantially with retarding both the onset and progression rate of the disease (Raoul et al., Citation2005). However, a shortcoming for their potential for employing in clinical use is that retrovirus insert into most genome with poor control of insertion site, which can trigger oncogenic transformation such as in the SCID clinical trials (Hacein-Bey-Abina et al., Citation2003).
In way for searching more sound delivery, rAAV (recombinant AAV) vectors appear to be a good compromise in terms of efficacy and safety for RNAi transfection in vivo. RNAi using rAAV vectors was achieved in a rodent model of spinocerebellar ataxia after intracranial delivery of rAAV shRNA targeting the disease causing ataxin-1, exerting significant improvement in cerebellar pathology and motor performance (Tiemann & Rossi, Citation2009).
8. Status of clinical trials of siRNA
Successful monstrance of siRNA in vivo turns a major break-through in biomedical research and drives a flood of exuberance for exploitation of siRNA-based therapeutics. At present, three different RNAi therapeutics currently under clinical investigation have focused on well validated therapeutic targets, such as the VEGF pathway for the wet form of AMD and on the RSV genome for the treatment of RSV infection, with several more poised to enter trials soon (Nattanan & Thienprasert, Citation2012; Tiemann & Rossi, Citation2009; Vaishnaw et al., Citation2010; Weinstein & Peer, Citation2010).
With affirmative enthusiasm the first ever clinical trial on man by administering an siRNA-based therapeutic was initiated in autumn 2004 by Acuity Pharmaceuticals in patients with AMD (Age-related Macular Degeneration) to target VEGF (Vascular Endothelial Growth Factor). Phase I clinical trial of Cand5 completed successfully by Acuity Pharmaceuticals in October 2005. Cand5 is an unmodified siRNA that targets all VEGF-A spliced isoforms. It has completed a phase II trial in patients with serious progressive wet AMD and has been reported to provide dose-related benefits against several endpoints, including near vision and lesion size. Cand5 is also being tested for efficacy against diabetic macular edema in a phase II trial that began in early 2006.
Independently, Sirna Therapeutics has developed a siRNA targeting VEGF receptor 1 for AMD (Sirna-027) and started a clinical trial a few months later. A single intravitreal dose of Sirna-027 ranging from 100 to 800 μg, appears to be safe and well tolerated with no systemic or local adverse events related to the drug. So far according to the clinical investigation, all patients enrolled in this trial have experienced visual acuity stabilization during their trial participation. Since no dose limiting toxicity has been observed, dose escalation will continue with the objective of delimiting a maximum tolerated dose. This preliminary data are encouraging in terms of tolerability of siRNA compounds and further results regarding the clinical activity reported to stabilize or improve visual acuity in a subset of patient.
Due to absence of nuclease environment and reduced drug dosing requirement pulmonary site turns to be lucrative for the delivery of siRNA. Possible research in this direction has risen up as piecemeal. The first pulmonary studies begin with shoot for treating RSV, a serious neonatal respiratory infection by Alnylam Pharmaceuticals. At present two phase I intranasal trials with ALN-RSV01, an siRNA targeting the viral nucleocapsid gene have been completed in over 100 healthy adult volunteers. ALN-RSV01 was found to be safe and well tolerated. Clinical development of ALN-RSV01 is in the pipeline and experimental infection studies are ongoing.
The world’s first and only antisense drug approved in November 1998 by the FDA was Vitravene®, an oligonucleotide discovered by ISIS and marketed by Novartis Ophthalmics for the treatment of cytomegalovirus retinitis in persons with AIDS enrolled to market as influential AIDS medications. Recently FDA has approved Macugen® (pegaptanib sodium injection), an antagonist and binding blocker of VEGF to its receptor designed to treat neovascular, or wet age-related macular degeneration by Eyetech Pharmaceuticals (Partner Pfizer Inc. will market the drug).
9. Glimpse of siRNA-based drug development programs
Assertive, reliable, safer, and productive monstrance of first siRNA in vivo clinical trials led to proclaimed and involution of nearly 30 clinical trials of 20 unique siRNA/small hairpin RNA (shRNA)-based drugs. This includes participation by 13 biotechnology and pharmaceutical firms and 3 academic-based research centers (Burnett & Rossi, Citation2012; Davidson & McCray, Citation2011; Zhou, Shum, Burnett, & Rossi, Citation2013). In addition, many large pharmaceutical companies have reported active intra group or collaborative RNAi-related drug development projects firmly employed in small molecule drug development.
Many companies have reported preclinical data validating their siRNA drug development projects in vivo in mammalian model systems. In November 2005 during a meeting at the American College of Rheumatology, the Nastech Pharmaceuticals (working with Mayo Clinic) introduced an antiTNF-α siRNA delivered using their peptide carrier system to treat rheumatoid arthritis. Use of antiapoB siRNAs to treat hypercholesterolemia was published by Alnylam Pharmaceuticals as preclinical work and Protiva Biotherapeutics, Sirna Therapeutics have published preclinical work relating to the treatment of hepatitis B infection (Morrissey et al., Citation2005; Soutschek et al., Citation2004). Various preclinical data have been promulgated by Calando Pharmaceuticals, Galenea, and Novartis relating to siRNA treatment of Ewing sarcoma, influenza in mice, and CNS independently (Ge et al., Citation2004; Hu-Lieskovan, Heidel, Bartlett, Davis, & Triche, Citation2005; Thakker et al., Citation2004). Intradigm has published a number of collaborative studies relating to siRNA treatment of cancer, arthritis, ocular diseases, and viral diseases while use of siRNAs in a mouse cancer model system was described by Abbott (Li et al., Citation2005). Exploitation of sound delivery by liposome-based system and hydrodynamic delivery system were reported by NeoPharm and Mirus, respectively. Novel chitosan delivery system was employed by TransGenex Nanobiotech’s to treat RSV using ddRNAi (Zhang et al., Citation2005).
Alnylam Pharmaceuticals (Cambridge, Massachusetts), a well-established siRNA-therapeutics company whose leading candidate siRNA ALN-RSV01 is presently under a phase II clinical trial is also in the pipeline to launch siRNAs directed against genes implicated in hypercholesterolaemia, Huntington’s disease (in a joint venture with Medtronic of Minneapolis, Minnesota), hepatitis C (in a joint venture with Isis Pharmaceuticals in Carlsbad, California), progressive multifocal leukoencephalopathy (in a joint venture with Biogen Idec of Cambridge, Massachusetts), and pandemic flu (in a joint venture with the Swiss company Novartis).
International Pachyonychia Congenita Consortium (IPCC) has formulated a siRNA targeting to pachyonychia congenita which allows for correct production of keratin as a treatment for a rare skin disorder in collaboration with TransDerm. With four HIV-positive patients in phase I clinical trial by lentiviral vector-mediated direct administration of Pol III promoter expressed shRNA targeting the HIV tat and rev shared exons is in progress by Benitec biopharma in collaboration with City of Hope National Medical Center. They have started a trial for the treatment of AIDS lymphoma where the gene-modified stem cells have been infused into blood stem cells to treat AIDS-related lymphomas.
Some companies, such as Regulus Therapeutics have chosen to focus on miRNAs as therapeutic targets. Santaris Pharma has recently conducted for the first time the phase I clinical trial to target a human miRNA (miR-122) with better chemical modification of a locked nucleic acid (LNA) anti miRNA to treat hepatitis C virus infection. The approach is intended because miR-122 facilitates replication of this virus in the liver and its down regulation is potentially useful in the treatment of hypercholesterolaemia.
Due to increasing capital availability and shortening of time frame involved in commercializing siRNA-based drugs as indicated above, partnerships and joint ventures have become quite accepted in the field of siRNA biotechnology. This short glimpse of active siRNA drug development programs and role of prosecuted pharmaceutical and biotechnology companies are summarized in Table (Behlke, Citation2006; Burnett, Rossiand, & Tiemann, Citation2011; Haussecker, Citation2012; Rettig & Behlke, Citation2012).
Table 1. Current status of siRNA drug development programs.
10. Challenges of siRNA as therapeutics
The promise of a better feature with siRNA is still vague until several key puzzles of associated safety, efficacy, and off-target effect are solved. Effects of RNAi observed in vivo are not due solely to target-specific silencing. But in addition, off-target effects, the in vivo use of RNAi has to deal, in particular, with the non-specific effects caused by activation of two components of the innate immune system: the IFN response and signaling mediated by TLRs (Toll-like receptors). Questions pertaining to the delivery of siRNA yet to be answered include delivery related toxicity, resistant and better biodistribution. The following section narrates a few of these challenges in the field of siRNA as therapeutics.
10.1. Interferon induction and the innate immune system
Perhaps the most significant unexpected ‘side effect’ encountered in the use of antisense DNA oligonucleotides is a sequence-specific ability to trigger the innate immune system. This relies upon a series of receptor molecules present on pathogen to induce a variety of cellular responses when these motifs encountered the Toll-like receptor family. Toll-like receptor 3 was reported to recognize dsRNA and certain siRNAs have been shown to trigger release of IFN-α, IFN-β, and interleukin 8 (IL-8) in HEK293 cells (Kariko, Bhuyan, Capodici, & Weissman, Citation2004).
siRNAs stimulate the innate immune system by triggering IFN secretion from human peripheral blood mononuclear cells (PBMCs). This must be considered during studies of systemic administration (Judge et al., Citation2005). For instance intravenous administration of unmodified siRNAs complexed with lipid particles to mice is more likely to cause IFN pathway activation. While, cationic DOTAP liposomes are significantly enhancing the ability of siRNA to trigger IFN responses in PBMCs of mice (Ma et al., Citation2005; Marques & Williams, Citation2005).
10.2. Off-target effects
Watson–Crick base pairing is highly specific and even a single mismatch at only one position is suffice to prevent proper hybridization. Systematic analysis of the functional impact caused by base changes at all positions in a target site has been reported. Many of the off-target effects attributed to siRNAs are derived from miRNA-like target interactions. These can be triggered by surprisingly limited sequence homology. Recently, it was revealed that the capability of siRNA to induce this seed dependent off-target effect is highly correlated with the thermodynamic stability of the duplex formed between the seed region of the siRNA guide strand and its target mRNA. The melting temperature (Tm) for the formation of the seed duplex shows strong correlation with seed dependent off-target effect (Naito et al., Citation2009). With the use of microarrays, it has become increasingly apparent that introducing foreign siRNAs into the cell alters the expression of non-target genes, as well as target genes. Because microarrays only reflect mRNA levels, they do not take into account any genes affected at the translational level, and so at present it is not clear how extensive the problem of off-target effects is. Given that the application of synthetic siRNAs results in transient inhibition of gene expression, specific off-target effect may not be a major concern for many clinical applications (Castanotto & Rossi, Citation2009).
10.3. Delivery related toxicity
The development of carriers, e.g. polymers, lipids, and conjugates, will be critical for the systemic delivery of siRNA therapeutics. The incorporation of surface pegylation and cell-specific targeting ligands in the carriers may improve the pharmacokinetics, biodistribution, and selectivity of siRNA therapeutics. The safety, effectiveness, ease of production, and manufacturing are important considerations for selecting the appropriate siRNA carriers (Wang, Ze Lu, Guillaume, & Jessie, Citation2010). Although a large number of studies have reported successful in vivo delivery of nucleic acids using liposome or polyplex reagents, issues of toxicity remained puzzling and must be considered. Evaluation of delivery associated toxicity of intravenous injection of cationic DOTAP liposomes directly with the cardiolipin analog CCLA: DOPE liposomes in mice were reported. A single dose of 100 mg/kg DOTAP liposomes to mice resulted in the death of two out of three. While CCLA liposomes showed no death out of three mice (Chien et al., Citation2005). Polyplex delivery systems have similar toxicity problems. The LD50 of linear PEI is around 4 mg/kg in mice, significantly limiting in vivo utility (Chollet, Favrot, Hurbin, & Coll, Citation2002). Recent reviews by Dass, Audouy, and Simberg provided in-depth information on cationic liposome delivery methods and in vivo toxicity (Audouy, de Leij, Hoekstra, & Molema, Citation2002; Dass, Citation2002; Simberg, Weisman, Talmon, & Barenholz, Citation2004).
10.4. Resistance
siRNA drug resistance is likely to develop by selection of escape mutations of the target sequence during viral replication or cancer cell division and plausibly it mediates in three ways. First, viral-mediated resistance is due to antigenic drift and shift. Virus has escape target sequence of guide strand and turn to resistant form. Two recent reports have indicated that siRNA-resistant HIV strains can emerge in as little as 25 days (Boden, Pusch, Lee, Tucker, & Ramratnam, Citation2003; Das et al., Citation2004). Second form of resistance is the fact that not all sequences can be targeted by siRNAs and that is likely due to a lack of accessibility of the RNA sequence, either hidden by RNA binding proteins or by complex secondary structures. Third, cells may also develop resistance to RNAi through loss of genes essential for RISC complex formation or selection of suppressors that inhibit degradation. For example cymbidium ring spot virus is resistant to RNAi via production of p19, a protein that inhibits RNAi by sequestering dsRNAs (Ryther, Flynt, Phillips, & Patton, Citation2005).
11. Delivering siRNA as a functional genomic tool
11.1. Drug target identification and validation
The identification of key gene as drug target needs to determine which genes are involved or correlated with the process, i.e. which genes are either up- or down-regulated in the cells and tissues of the biological system of interest. The traditional approach to identifying genes involved starts with genome-wide screening of targeted gene expression and when applied at pathological level has generated a list of candidates as drug target but it lacks validation. A more fascinating way is to use reverse genetic approaches such as ODNs or ribozymes to reduce the expression of specific genes. These have proven successful for use in drug target validation, but have never passed the hurdle of genome-wide target identification. Consequently, a tool that can selectively down regulate individual genes within the cells or tissues is widely recognized as a valuable means for understanding gene function and is highly welcome in the field of biomedical research for not only drug target identification but for its validation.
siRNA has been rapidly adopted as a gene function tool due to its high gene silencing efficiency at low concentration, ease of finding accessible target sites, high specificity, good stability, and custom siRNA synthesis at moderately low cost. Functional genomic approaches delineating a complete signal transduction pathway using multiple siRNAs, channelizes to effectuation of a plethora of studies for the pertinent candidate genes in vitro in diverse medical specialties such as silencing of HIV cell line to primary blood lymphocyte, selective suppression of the gene mutation for ALS (amyotrophic lateral sclerosis) and Huntington’s disease. The emergence of siRNA selective inhibition of gene expression has changed the landscape of cancer research on tumorigenic genes. Since the RNAi-mediated gene silencing machinery is also intact in cancer cells, multiple in vitro siRNA studies have put in to action to exploit out small molecule. Antibody directed anticancer drugs are used to identify and validate tumorigenic targets. For this purpose one unique target identification approach named as Efficacy-FirstTM discovery reported, where siRNAs are directly administered in to the xenograft tumor model to induce phenotypic effects on tumor growth rate and allow identification of up- or down-regulated genes by correlating with induced tumor growth behaviors. It not only allows the selection of pool of gene targets remarkably over expressed in tumors but also differentiates gene target playing role in disease control. This combines to Disease-ControlTM validation. Importantly, this approach offers an ability to gain insight into the genes and proteins associated with the later stages of pathology since it can be applied to established disease tissues (Lu, Xie, & Woodle, Citation2003).
Use of siRNA to reveal tumorigenic properties of new targets and its relationship with p53 relevant pathways represents one of the most active research areas. siRNA is used as a tool to characterize tumorigenic target and study tumor gene function. Many of these studies provided not only better understanding of the mechanism of action of the validated gene targets, but also potential means for siRNA-based cancer therapeutics. Use of synthetic siRNAs to suppress gene expression in mammalian cells is able to discriminate a single base difference between mutant and WT p53 in cells expressing both forms, resulting in the restoration of wild type p53 protein function. This result indicates that siRNAs may be used to suppress expression of point-mutated genes and revealed that it is potential for selective and personalized antitumor therapy. Besides p53, activation and over expression of various growth factors, such as EGF, FGF, PDGF, TGF, IGF, and VEGF are often reported to display higher oncogenic response. Among the well-known cancer cell growth factors VEGF, VEGF, R2, and EGFR represent three of the most widely recognized and highly validated targets. siRNA is used to down regulate epidermal growth factor receptor (EGFR or erbB1), a tyrosine kinase specific for the epidermal growth factor (EGF). One study showed that endogenous erbB1 can be specifically and extensively suppressed in A431 human epidermoid carcinoma cells. As a consequence EGF-induced tyrosine phosphorylation was inhibited and cell proliferation was reduced due to induction of apoptosis. Selective inhibition of the expression of fusion protein was achieved with a siRNA specific for the EGFP mRNA, whereas the erbB1 specific siRNA inhibited the expression of both molecules. This result indicated that siRNA-mediated inhibition of erbB1 and other erbB tyrosine kinases could constitute a useful therapeutic approach in the treatment of human cancer (Nagy, Arndt-Jovin, & Jovin, Citation2003).
11.2. In vivo investigation of gene function
siRNAs have broadened the horizons of the types of experiments that can be done in mammalian model systems. For example, to determine the relative amount of gene product needed for certain processes at particular developmental stages, it is now possible to modulate gene dosage in a spatial and temporal manner by simply varying the amount of siRNA expressed in the cell. The early in vitro experiments rapidly established the role of siRNA-mediated knockdown as a valuable tool for studying the genetic impact on human diseases. However, bearing in mind the complexities of the RNAi machinery, designing siRNAs for experimental gene silencing has to be conducted carefully in order to achieve maximum mRNA knockdown while minimizing undesired effects.
The role of genes involved in obesity has been identified using this approach. Mice with a targeted disruption of the stearoyl-CoA desaturase-1 (SCD-1) isoform were found to have reduced body adiposity and increased insulin sensitivity (Ntambi et al., Citation2002).
11.3. siRNAs as tools for genome-wide screening
Genetic screening for identifying new gene and investigating its function in various model organism has been practiced by saturate genetic screening or reverse genetics. However, most of them are limited to non-mammalian model. Only a few non saturating genetic screens had been conducted in mice. While current reverse genetic approaches for studying embryonic development in zebra fish use anti-sense molecules such as morpholino phosphorodiamidates oligonucelotides. But with the advent of siRNAs, it is now feasible to carry out reverse genetic screens in mammalian tissue culture cells, Xenopus oocytes, chicken, mouse, and rat embryos.
Several near genome-wide RNAi screens have been conducted using long dsRNA in C. elegans and Drosophila melanogaster. These have identified genes involved in fundamental processes such as cell division, apoptosis, cell morphology, and physiological processes such as fat metabolism. Several large-scale RNAi screens have been conducted in mammalian tissue culture cells using synthetic siRNAs screens to identify genes involved in apoptosis, signaling, regulation of protein stability, and the ultraviolet radiation damage response.
Screening by RNAi is relatively fast and easy, but looking to downfall of screening compared to classical genetic screens, siRNAs can identify mutations that are not in coding regions and also produce dominant loss or gain of function by mutations which are often useful, and sometimes essential for understanding gene function. Another pitfall of screening with RNAi is that siRNAs almost never fully deplete the target mRNA and usually several different siRNAs must be screened before an effective siRNA is identified. Transient RNAi in rapidly dividing tissue culture cells usually lasts three to five days. However, even if applied to slowly dividing cells, it is possible that an effective siRNA will have difficulty in depleting a stable protein (Dorsett & Tuschl, Citation2004).
12. Exploring horizon of siRNA
12.1. Novel siRNA-based molecular beacons for dual imaging and therapy
Novel bioengneering modification to siRNAs made it possible to combine therapeutic along with imaging function for its targeted delivery. In vivo monitoring of gene expression is achieved successfully by utilizing molecular beacons (MBs). Novel siRNA-based probe utilizing two short 21nt RNA complementary strands as the stem and a highly flexible two different PEG lengths (Mr 3400 and 5000) are covalently linked to the RNA strands as the loop of a siRNA-based MBs as shown in Figure .
Detection and knockdown of telomerase expression in human breast cancer cells using probe is reported sucessfully. This has provided a novel model of siRNA target validation that is not currently possible in live cells. This holds promising potential in biological applications for disease detection and therapy based on mRNA expression such as a telomerase-targeted siRNA probe in cancer (Chang, Zhu, & Drezek, Citation2007).
12.2. Novel therapeutics
Endogenous small RNAs have been found in various organisms such as humans, mice, the fruitfly D. melanogaster, and C. elegans. This may have originated from transposons, viruses, and repetitive sequences. Some of them are characterized by their interactions with the PIWI subfamily (or PIWI clade) of Argonaute proteins and thus named PIWI interacting RNAs (piRNAs), notably identification restricted to only germline cell. Recently a new class of endogenous siRNAs (endo-siRNAs or esiRNAs) has been identified in the gonads and somatic tissues of D. melanogaster and in mouse oocytes. Several families of small RNAs including repeat-associated siRNAs (ra-siRNAs), transacting siRNAs (ta-siRNAs), and natural antisense transcript siRNAs (natRNAs) are found in fungi, plants and animals (Table. ), but so far none of these has been observed in mammals (Castanotto & Rossi, Citation2009).
Table 2. Different cellular small RNAs involved in gene silencing.
Various evidence suggests that piRNAs act through different cellular pathways than siRNAs, miRNAs, and endo-siRNAs. In mice, regulation by retrotransposon movement has been proposed, which offers alternative targeting strategies for therapeutic targets (Dorsett & Tuschl, Citation2004).
13. Conclusion
Considering the relative youth of the RNAi field, a remarkable number of in vivo studies have already been published that include preclinical development of siRNA drugs or the use of siRNA to validate targets for small molecule in drug development projects. The list of diseases for which RNAi is being tested as a therapeutic agent is extensive. These include Parkinson’s disease, Lou Gehrig’s disease, HIV infection, wet age-related macular degeneration, type 2 diabetes, obesity, hypercholesterolaemia, rheumatoid arthritis, respiratory diseases, and cancers. With siRNA, virtually every gene in the human genome would become amenable to regulation, thus opening unprecedented opportunities in the field of drug discovery. Whether siRNA will explore as a novel chemical entity that lead ‘bench to bedside’ is probably dependent largely on resolving various pharmacokinetic obstacles such as plasma stability and especially cellular uptake. Data show that local administration of siRNA seems to be more advisable than systemic application. Although various obstacles are in the way, new emerging high-throughput approaches like the combination of automation and rational design for in silico designing of siRNA will lead to better efficacy, potency, specificity, stability, and most desired targeted bioavailability. There is also the development of new, noninvasive imaging methods to monitor the in vivo delivery of siRNAs, such as siRNA with conventional molecular beacon aid studies of tissue uptake and biodistribution.
With assertive, reliable, safer, and productive monstrance of first siRNA in vivo clinical trials led to proclaimed and involution by over 30 pharmaceutical and biotechnology industries. More than 30 companies prosecute in business in active drug development program with siRNA-based therapeutics despite present obstacles. The business has already enriched to US $1 billion by 2010, and is still expected to grow to $10.5 billion by the year 2015. The share of various therapeutic areas in 2015 is projected to be $4.6 billion for infections, $1.3 billion for cancer, $1.5 billion for neurological disorders, $700 million for degenerative disorders of macula and retina, and $2.4 billion for all the other disorders. This is quite lucrative and shows positive signs of further growth to current opportunities in the field of bio-therapeutics.
The functional genomics driven by RNAi methods holds great promise for unveiling new dimensions of siRNA-mediated gene silencing and its profound implications for understanding gene regulation, investigation of genes function rapidly identification, and validation of drug. Complementing siRNA-based screens along proteomic methods will yield a relatively descriptive outlook on particular cellular processes that can be further studied. Given the way that siRNA has transformed basic research with the unprecedented speed to real practice at clinic, in coming years siRNA research promise to be exciting for the development of personalized medicine which will fasten the drug development and answer many ‘undrugable’ diseases of present.
References
- Allerson, C. R., Sioufi, N., Jarres, R., Prakash, T., Naik, N., Berdeja, A., … Bhat, B. (2005). Fully 2‘-modified oligonucleotide duplexes with improved in vitro potency and stability compared to unmodified small interfering RNA. Journal of Medicinal Chemistry, 48, 901–904.10.1021/jm049167j
- Audouy, S. A., de Leij, L., Hoekstra, D., & Molema, G. (2002). In vivo characteristics of cationic liposomes as delivery vectors for gene therapy. Pharmaceutical Research, 19, 1599–1605.10.1023/A:1020989709019
- Aza-Blanc, P., Cooper, C., Wagner, K., Batalov, S., Deveraux, Q., & Cooke, M. (2003). Identification of modulators of TRAIL-induced apoptosis via RNAi-based phenotypic screening. Molecular Cell, 12, 627–637.10.1016/S1097-2765(03)00348-4
- Behlke, M. A. (2006). Progress towards in vivo use of siRNAs. Molecular Therapy, 13, 644–670.10.1016/j.ymthe.2006.01.001
- Behlke, M. A. (2008). Chemical modification of siRNAs for in vivo use. Oligonucleotides, 18, 305–319.
- Bernstein, E., Caudy, A., Hammond, S., & Hannon, G. (2001). Role for a bidentate ribonuclease in the initiation step of RNA interference. Nature, 409, 363–366.10.1038/35053110
- Bitko, V., Musiyenko, A., Shulyayeva, O., & Barik, S. (2005). Inhibition of respiratory viruses by nasally administered siRNA. Nature Medicine, 11, 50–55.10.1038/nm1164
- Boden, D., Pusch, O., Lee, F., Tucker, L., & Ramratnam, B. (2003). Human immunodeficiency virus type 1 escape from RNA interference. Journal of Virology, 77, 11531–11535.10.1128/JVI.77.21.11531-11535.2003
- Braasch, D. A., Paroo, Z., Constantinescu, A., Ren, G., Oz, O., Mason, R., & Corey, D. (2004). Biodistribution of phosphodiester and phosphorothioate siRNA. Bioorganic & Medicinal Chemistry Letters, 14, 1139–1143.10.1016/j.bmcl.2003.12.074
- Bramsen, J. B., & Kjems, J. (2011). Chemical modification of small interfering RNA. Methods Mol. Biol., 721, 77–103.
- Burnett, J. C., & Rossi, J. (2012). RNA-based therapeutics: Current progress and future prospects. Chemistry & Biology, 19, 60–71.10.1016/j.chembiol.2011.12.008
- Burnett, J. C., Rossiand, J. J., & Tiemann, K. (2011). Current progress of siRNA/shRNA therapeutics in clinical trials. Biotechnology Journal, 6, 1130–1146.10.1002/biot.201100054
- Castanotto, D., & Rossi, J. (2009). The promises and pitfalls of RNA-interference-based therapeutics. Nature, 457, 426–433.10.1038/nature07758
- Celotto, A. M., & Graveley, B. (2002). Exon-specific RNAi: A tool for dissecting the functional relevance of alternative splicing. RNA, 8, 718–724.10.1017/S1355838202021064
- Chang, E., Zhu, M., & Drezek, R. (2007). Novel siRNA-based molecular beacons for dual imaging and therapy. Biotechnology Journal, 2, 1–5.
- Chien, P. Y., Wang, J., Carbonaro, D., Lei, S., Miller, B., Sheikh, S., … Ahmad, I. (2005). Novel cationic cardiolipin analogue-based liposome for efficient DNA and small interfering RNA delivery in vitro and in vivo. Cancer Gene Therapy, 12, 321–328.10.1038/sj.cgt.7700793
- Chollet, P., Favrot, M., Hurbin, A., & Coll, J. (2002). Side-effects of a systemic injection of linear polyethylenimine-DNA complexes. The Journal of Gene Medicine, 4, 84–91.10.1002/(ISSN)1521-2254
- Choung, S., Kim, Y., Kim, S., Park, H., & Choi, Y. (2006). Chemical modification of siRNAs to improve serum stability without loss of efficacy. Biochemical and Biophysical Research Communications, 342, 919–927.10.1016/j.bbrc.2006.02.049
- Coburn, G., & Cullen, B. (2003). siRNAs: A new wave of RNA-based therapeutics. Journal of Antimicrobial Chemotherapy, 51, 753–756.10.1093/jac/dkg166
- Couzin, J. (2002). Breakthrough of the year: Small RNAs make big splash. Science, 298, 2296–2297.10.1126/science.298.5602.2296
- Das, A. T., Brummelkamp, T., Westerhout, E., Vink, M., Madiredjo, M., Bernards, R., & Berkhout, B. (2004). Human immunodeficiency virus type 1 escapes from RNA interference-mediated inhibition. Journal of Virology, 78, 2601–2605.10.1128/JVI.78.5.2601-2605.2004
- DasGupta, R., & Perrimon, N. (2004). Using RNAi to catch Drosophila genes in a web of interactions: Insights into cancer research. Oncogene, 23, 8359–8365.10.1038/sj.onc.1208028
- Dass, C. R. (2002). Cytotoxicity issues pertinent to lipoplex-mediated gene therapy in vivo. Journal of Pharmacy and Pharmacology, 54, 593–601.10.1211/0022357021778817
- Davidson, B. L., & McCray, P. (2011). Current prospects for RNA interference-based therapies. Nature Reviews Genetics, 12, 329–340.10.1038/nrg2968
- de Fougerolles, A., Manoharan, M., Meyers, R., & Vornlocher, H. (2005). RNA interference in vivo: toward synthetic small inhibitory RNA-based therapeutics. Methods Enzymology, 392, 278–296.10.1016/S0076-6879(04)92016-2
- de Fougerolles, A., Vornlocher, H., Maraganore, J., & Lieberman, J. (2007). Interfering with disease: A progress report on siRNA-based therapeutics. Nature Reviews Drug Discovery, 6, 443–453.10.1038/nrd2310
- Dorsett, Y., & Tuschl, T. (2004). siRNAs: Applications in functional genomics and potential as therapeutics. Nature Reviews Drug Discovery, 3, 318–329.10.1038/nrd1345
- Dykxhoorn, D. M., & Lieberman, J. (2006). Knocking down disease with siRNAs. Cell, 126, 231–235.10.1016/j.cell.2006.07.007
- Eckstein, F. (2002). Developments in RNA chemistry, a personal view. Biochimie, 84, 841–848.10.1016/S0300-9084(02)01459-1
- Elbashir, S. M., Lendeckel, W., & Tuschl, T. (2001). RNA interference is mediated by 21- and 22-nucleotide RNAs. Genes & Development, 15, 188–200.10.1101/gad.862301
- Elbashir, S. M., Martinez, J., Patkaniowska, A., Lendeckel, W., & Tuschl, T. (2001). Functional anatomy of siRNAs for mediating efficient RNAi in Drosophila melanogaster embryo lysate. The EMBO Journal, 20, 6877–6888.10.1093/emboj/20.23.6877
- Faustino, N., & Cooper, T. (2003). Pre-mRNA splicing and human disease. Genes & Development, 17, 419–437.10.1101/gad.1048803
- Fire, A., Xu, S., Montgomery, M., Kostas, S., Driver, S., & Mello, C. (1998). Potent and specific genetic interference by double-stranded RNA in Caenorhabditis elegans. Nature, 391, 806–811.10.1038/35888
- Gaglione, M., & Messere, A. (2010). Recent progress in chemically modified siRNAs. Mini Reviews in Medical Chemistry, 10, 578–595.
- Ge, Q., Filip, L., Bai, A., Nguyen, T., Eisen, H., & Chen, J. (2004). Inhibition of influenza virus production in virus-infected mice by RNA interference. Proceedings of the National Academy of Sciences, 101, 8676–8681.10.1073/pnas.0402486101
- Grimm, D., Streetz, K., Jopling, C., Storm, T., Pandey, K., Davis, C., Marion, P., Salazar, F., & Kay, M. (2006). Fatality in mice due to oversaturation of cellular microRNA/short hairpin RNA pathways. Nature, 441, 537–541.10.1038/nature04791
- Hacein-Bey-Abina, S., von Kalle, C., Schmidt, M., Le Deist, F., Wulffraat, N., McIntyre, E., … Fischer, A. (2003). A serious adverse event after successful gene therapy for X-linked severe combined immunodeficiency. New England Journal of Medicine, 348, 255–256.10.1056/NEJM200301163480314
- Haussecker, D. (2012). The business of RNAi therapeutics. Molecular Therapeutics Nuclei Acids, 2, 1–12.
- He, L., & Hannon, G. (2004). MicroRNAs: small RNAs with a big role in gene regulation. Nature Reviews Genetics, 5, 522–531.10.1038/nrg1379
- Holen, T., Amarzguioui, M., Babaie, E., & Prydz, H. (2003). Similar behaviour of single-strand and double-strand siRNAs suggests they act through a common RNAi pathway. Nucleic Acids Research, 31, 2401–2407.10.1093/nar/gkg338
- Hornung, V., Guenthner-Biller, M., Bourquin, C., Ablasser, A., Schlee, M., Uematsu, S., … Hartmann, G. (2005). Sequence-specific potent induction of IFN-α by short interfering RNA in plasmacytoid dendritic cells through TLR7. Nature Medicine, 11, 263–270.10.1038/nm1191
- Huesken, D., Lange, J., Mickanin, C., Weiler, J., Asselbergs, F., Warner, J., … Hall, J. (2005). Design of a genome-wide siRNA library using an artificial neural network. Nature Biotechnology, 23, 995–1001.10.1038/nbt1118
- Hu-Lieskovan, S., Heidel, J., Bartlett, D., Davis, M., & Triche, T. (2005). Sequence-specific knockdown of EWS-FLI1 by targeted, nonviral delivery of small interfering RNA inhibits tumor growth in a murine model of metastatic Ewing’;s sarcoma. Cancer Research, 65, 8984–8992.10.1158/0008-5472.CAN-05-0565
- Judge, A. D., Sood, V., Shaw, J., Fang, D., McClintock, K., & Maclachlan, I. (2005). Sequence-dependent stimulation of the mammalian innate immune response by synthetic siRNA. Nature Biotechnology, 23, 457–462.10.1038/nbt1081
- Kariko, K., Bhuyan, P., Capodici, J., & Weissman, D. (2004). Small interfering RNAs mediate sequence-independent gene suppression and induce immune activation by signaling through toll-like receptor 3. The Journal of Immunology, 172, 6545–6549.10.4049/jimmunol.172.11.6545
- Kenski, D. M., Butora, G., Willingham, A. T., Cooper, A. J., Fu, W., Qi, N., … Flanagan, W. M. (2012). siRNA-optimized modifications for enhanced in vivo activity. Molecular Therapy – Nucleic Acids, 1, e5, doi:10.1038/mtna.2011.410.1038/mtna.2011.4
- Ketting, R. F., Fischer, S., Bernstein, E., Sijen, T., Hannon, G., & Plasterk, R. (2001). Dicer functions in RNA interference and in synthesis of small RNA involved in developmental timing in C. elegans. Genes & Development, 15, 2654–2659.10.1101/gad.927801
- Khvorova, A., Reynolds, A., & Jayasena, S. (2003). Functional siRNAs and miRNAs exhibit strand bias. Cell, 115, 209–216.10.1016/S0092-8674(03)00801-8
- Kim, D. H., Behlke, M., Rose, S., Chang, M., Choi, S., & Rossi, J. (2005). Synthetic dsRNA Dicer substrates enhance RNAi potency and efficacy. Nature Biotechnology, 23, 222–226.10.1038/nbt1051
- Kim, D. H., & Rossi, J. (2007). Strategies for silencing human disease using RNA interference. Nature Reviews Genetics, 8, 173–184.10.1038/nrg2006
- Layzer, J. M., McCaffrey, A., Tanner, A., Huang, Z., Kay, M., & Sullenger, B. (2004). In vivo activity of nuclease-resistant siRNAs. RNA, 10, 766–771.10.1261/rna.5239604
- Li, L., Lin, X., Staver, M., Shoemaker, A., Semizarov, D., Fesik, S., & Shen, Y. (2005). Evaluating hypoxia-inducible factor-1 as a cancer therapeutic target via inducible RNA interference in vivo. Cancer Research, 65, 7249–7258.10.1158/0008-5472.CAN-04-4426
- Lin, X., Ruan, X., Anderson, M., McDowell, J., Kroeger, P., Fesik, S., & Shen, Y. (2005). siRNA-mediated off-target gene silencing triggered by a 7 nt complementation. Nucleic Acids Research, 33, 4527–4535.10.1093/nar/gki762
- Liu, J., Carmell, M., Rivas, F., Marsden, C., Thomson, J., Song, J., … Hannon, G. (2004). Argonaute2 is the catalytic engine of mammalian RNAi. Science, 305, 1437–1441.10.1126/science.1102513
- Liu, Q., Rand, T., Kalidas, S., Du, F., Kim, H., Smith, D., & Wang, X. (2003). R2D2, a bridge between the initiation and effector steps of the Drosophila RNAi pathway. Science, 301, 1921–1925.10.1126/science.1088710
- Lu, P. Y., Xie, F., & Woodle, M. (2003). siRNA-mediated antitumorgenesis for drug target validation and therapeutics. Current Opinion in Molecular Therapeutics, 5, 225–234.
- Ma, Z., Li, J., He, F., Wilson, A., Pitt, B., & Li, S. (2005). Cationic lipids enhance siRNA-mediated interferon response in mice. Biochemical and Biophysical Research Communications, 330, 755–759.10.1016/j.bbrc.2005.03.041
- Manoharan, M. (1999). 2′-Carbohydrate modifications in antisense oligonucleotide therapy: importance of conformation, configuration and conjugation. Biochimica et Biophysica Acta (BBA) - Gene Structure and Expression, 1489, 117–130.10.1016/S0167-4781(99)00138-4
- Manoharan, M. (2002). Oligonucleotide conjugates as potential antisense drugs with improved uptake, biodistribution, targeted delivery, and mechanism of action. Antisense and Nucleic Acid Drug Development, 12, 103–128.10.1089/108729002760070849
- Marques, J. T., & Williams, B. (2005). Activation of the mammalian immune system by siRNAs. Nature Biotechnology, 23, 1399–1405.10.1038/nbt1161
- Martinez, J., Patkaniowska, A., Urlaub, H., Luhrmann, R., & Tuschl, T. (2002). Single-stranded antisense siRNAs guide target RNA cleavage in RNAi. Cell, 110, 563–574.10.1016/S0092-8674(02)00908-X
- Matranga, C., Tomari, Y., Shin, C., Bartel, D., & Zamore, P. (2005). Passenger-strand cleavage facilitates assembly of siRNA into Ago2-containing RNAi enzyme complexes. Cell, 123, 607–620.10.1016/j.cell.2005.08.044
- McCaffrey, A. P., Meuse, L., Pham, T., Conklin, D., Hannon, G., & Kay, M. (2002). Gene expression: RNA interference in adult mice. Nature, 418, 38–39.10.1038/418038a
- McManus, M. T., Haines, B., Dillon, C., Whitehurst, C., van Parijs, L., Chen, J., & Sharp, P. A. (2002). Small interfering RNA-mediated gene silencing in T lymphocytes. The Journal of Immunology, 169, 5754–5760.10.4049/jimmunol.169.10.5754
- Meister, G., & Tuschl, T. (2004). Mechanisms of gene silencing by double-stranded RNA. Nature, 431, 343–349.10.1038/nature02873
- Morrissey, D. V., Lockridge, J., Shaw, L., Blanchard, K., Jensen, K., Breen, W., … Polisky, B. (2005). Potent and persistent in vivo anti-HBV activity of chemically modified siRNAs. Nature Biotechnology, 23, 1002–1007.10.1038/nbt1122
- Nagy, P., Arndt-Jovin, D., & Jovin, T. (2003). Small interfering RNAs suppress the expression of endogenous and GFP-fused epidermal growth factor receptor (erbB1) and induce apoptosis in erbB1-overexpressing cells. Experimental Cell Research, 285, 39–49.10.1016/S0014-4827(02)00050-2
- Naito, Y., Yoshimura, J., Morishita, S., & Ui-Tei, K. (2009). siDirect 2.0: updated software for designing functional siRNA with reduced seed-dependent off-target effect. BMC Bioinformatics, 10, 392–399.10.1186/1471-2105-10-392
- Nattanan, T., & Thienprasert, P. (2012). Overview of RNA interference therapeutics. Songklanakarin Journal of Science and Technology, 34, 293–301.
- Ntambi, J. M., Miyazaki, M., Stoehr, J., Lan, H., Kendziorski, C., Yandell, B., … Attie, A. (2002). Loss of stearoyl-CoA desaturase-1 function protects mice against adiposity. Proceedings of the National Academy of Sciences, 99, 11482–11486.10.1073/pnas.132384699
- Opalinska, J. B., & Gewirtz, A. (2002). Nucleic-acid therapeutics: Basic principles and recent applications. Nature Reviews Drug Discovery, 1, 503–514.10.1038/nrd837
- Pei, Y., & Tuschl, T. (2006). On the art of identifying effective and specific siRNAs. Nature Methods, 3, 670–676.10.1038/nmeth911
- Poulin, G., Nandakumar, R., & Ahringer, J. (2004). Genome-wide RNAi screens in Caenorhabditis elegans: Impact on cancer research. Oncogene, 23, 8340–8345.10.1038/sj.onc.1208010
- Raoul, C., Abbas-Terki, T., Bensadoun, J., Guillot, S., Haase, G., Szulc, J., … Aebischer, P. (2005). Lentiviral-mediated silencing of SOD1 through RNA interference retards disease onset and progression in a mouse model of ALS. Nature Medicine, 11, 423–428.10.1038/nm1207
- Rettig, G. R., & Behlke, M. A. (2012). Progress toward in vivo use of siRNAs-II. Molecular Therapy, 20, 483–512.10.1038/mt.2011.263
- Reynolds, A., Leake, D., Boese, Q., Scaringe, S., Marshall, W., & Khvorova, A. (2004). Rational siRNA design for RNA interference. Nature Biotechnology, 22, 326–330.10.1038/nbt936
- Reynolds, A., Anderson, E., Vermeulen, A., Fedorov, Y., Robinson, K., Leake, D., … Khvorova, A. (2006). Induction of the interferon response by siRNA is cell type- and duplex length-dependent. RNA, 12, 988–993.10.1261/rna.2340906
- Ryther, R. C., Flynt, A., Harris, B., Phillips, J., III, & Patton, J. (2004). GH1 splicing is regulated by multiple enhancers whose mutation produces a dominant-negative GH isoform that can be degraded by allele-specific small interfering RNA (siRNA). Journal of Endocrinology, 145, 2988–2996.10.1210/en.2003-1724
- Ryther, R. C., Flynt, A., Phillips, J., III, & Patton, J. (2005). siRNA therapeutics: Big potential from small RNAs. Gene Therapy, 12, 5–11.10.1038/sj.gt.3302356
- Scacheri, P. C., Rozenblatt-Rosen, O., Caplen, N., Wolfsberg, T., Umayam, L., Lee, J., … Collins, F. (2004). Short interfering RNAs can induce unexpected and divergent changes in the levels of untargeted proteins in mammalian cells. Proceedings of the National Academy of Sciences, 101, 1892–1897.10.1073/pnas.0308698100
- Schwarz, D. S., Hutvágner, G., Du, T., Xu, Z., Aronin, N., & Zamore, P. (2003). Asymmetry in the assembly of the RNAi enzyme complex. Cell, 115, 199–208.10.1016/S0092-8674(03)00759-1
- Semizarov, D., Frost, L., Sarthy, A., Kroeger, P., Halbert, D., & Fesik, S. (2003). Specificity of short interfering RNA determined through gene expression signatures. Proceedings of the National Academy of Sciences, 100, 6347–6352.10.1073/pnas.1131959100
- Shirane, D., Sugao, K., Namiki, S., Tanabe, M., Iino, M., & Hirose, K. (2004). Enzymatic production of RNAi libraries from cDNAs. Nature Genetics, 36, 190–196.10.1038/ng1290
- Simberg, D., Weisman, S., Talmon, Y., & Barenholz, Y. (2004). DOTAP (and other cationic lipids): chemistry, biophysics, and transfection. Critical Reviews in Therapeutic Drug Carrier Systems, 21, 257–317.10.1615/CritRevTherDrugCarrierSyst.v21.i4
- Sorensen, D. R., Leirdal, M., & Sioud, M. (2003). Gene silencing by systemic delivery of synthetic siRNAs in adult mice. Journal of Molecular Biology, 327, 761–766.10.1016/S0022-2836(03)00181-5
- Soutschek, J., Akinc, A., Bramlage, B., Charisse, K., Constien, R., Donoghue, M., … Vornlocher, H. (2004). Therapeutic silencing of an endogenous gene by systemic administration of modified siRNAs. Nature, 432, 173–178.10.1038/nature03121
- Thakker, D. R., Natt, F., Hüsken, D., Maier, R., Müller, M., van der Putten, H., Hoyer, D., & Cryan, J. (2004). Neurochemical and behavioral consequences of widespread gene knockdown in the adult mouse brain by using nonviral RNA interference. Proceedings of the National Academy of Sciences, 101, 17270–17275.10.1073/pnas.0406214101
- Tiemann, K., & Rossi, J. J. (2009). RNAi-based therapeutics-current status, challenges and prospects. EMBO Molecular Medicine, 1, 142–151.10.1002/emmm.200900023
- Tousignant, J. D., Gates, A., Ingram, L., Johnson, C., Nietupski, J., Cheng, S., … Scheule, R. (2000). Comprehensive analysis of the acute toxicities induced by systemic administration of cationic lipid: plasmid DNA complexes in mice. Hum. Gene Ther., 11, 2493–2513.
- Urban-Klein, B., Werth, S., Abuharbeid, S., Czubayko, F., & Aigner, A. (2005). RNAi-mediated gene-targeting through systemic application of polyethylenimine (PEI)-complexed siRNA in vivo. Gene Therapy, 12, 461–466.10.1038/sj.gt.3302425
- Vaishnaw, A. K., Gollob, J., Gamba-Vitalo, C., Hutabarat, R., Sah, D., Meyers, R., … Maraganore, J. (2010). A status report on RNAi therapeutics. Silence, 1, 14.10.1186/1758-907X-1-14
- Victor, M. M., Xia, H., Marrs, G., Gouvion, C., Lee, G., Davidson, B., & Paulson, H. (2003). Allele-specific silencing of dominant disease genes. Proceedings of National Academy of Science USA, 100, 7195–7200.
- Wang, J., Lu, Z., & Guillaume, W. (2010). Delivery of siRNA therapeutics: Barriers and carriers. The AAPS Journal, 12, 492–503.10.1208/s12248-010-9210-4
- Weinstein, S., & Peer, D. (2010). RNAi nanomedicines: Challanges and opportunities within the immune system. Nanotechnology, 21, 1–13.
- Yi, R., Qin, Y., Macara, I., & Cullen, B. (2003). Exportin-5 mediates the nuclear export of pre-microRNAs and short hairpin RNAs. Genes & Development, 17, 3011–3016.10.1101/gad.1158803
- Zhang, W., Yang, H., Kong, X., Mohapatra, S., San Juan-Vergara, H., Hellermann, G., … Mohapatra, S. (2005). Inhibition of respiratory syncytial virus infection with intranasal siRNA nanoparticles targeting the viral NS1 gene. Nature Medicine, 11, 56–62.10.1038/nm1174
- Zhao, L. N., Li, T., Pan, Y., Ning, H., Liu, Z., & Fan, D. (2005). Application of siRNA Library in High-Throughput Genetic Screens of Mammalian Cells. Journal of Cancer Molecules, 1, 19–24.
- Zhou, J., Shum, K., Burnett, J. C., & Rossi, J. (2013). Nanoparticle-based delivery of RNAi therapeutics: Progress and challenges. Pharmaceuticals, 6, 85–107.10.3390/ph6010085