Abstract
This paper examined heat-induced radiosensitization in two Chinese hamster heat-resistant cell lines, HR-1 and OC-14, that were isolated from the same wild-type HA-1 cell line. It found a reduction of the magnitude of heat-induced radiosensitization after exposure to 43°C in both HR-1 and OC-14 cells and a similar reduction after exposure to 45°C in HR-1 cells, but not in OC-14 cells. The effect of heat exposure on a class of ionizing radiation-induced DNA damage that inhibits the ability of nuclear DNA to undergo super-coiling changes was also studied using the fluorescent halo assay in these three cell lines. Wild type cells exposed to either 43 or 45°C before irradiation had a DNA rewinding ability that was intermediate between control and unheated cells, a phenomenon previously described as a masking effect. This masking effect was significantly reduced in HR-1 cells exposed to either 43 or 45°C or in OC-14 cells exposed to 43°C under conditions that heat-induced radiosensitization was reduced. In contrast, the masking effect was not altered in OC-14 cells exposed to 45°C, conditions under which heat-induced radiosensitization was similar to that observed in wild-type HA-1 cells. These results suggest that a reduction in the masking effect is associated with a reduction of the magnitude of heat-induced radiosensitization in the HR-1 and OC-14 heat-resistant cell lines. The reduction of the masking effect in the cell lines resistant to heat-induced radiosensitization was associated with neither a reduction in the magnitude of the heat-induced increase in total nuclear protein content nor major differences in the protein profiles of the nucleoids isolated from heated cells.
Introduction
The biological rationale for the use of hyperthermia in the clinic in the treatment of tumours rests mainly on the fact that hyperthermia is one of the most potent sensitizers for the action of ionizing radiation (reviewed in Citation[1]). Recently, randomized phase III trials have indicated that the combination of conventional radiotherapy with hyperthermia leads to significantly better tumour control of malignant melanomas Citation[2], Citation[3], recurrent breast carcinomas Citation[4], deep pelvic tumours Citation[5], Citation[6] and glioblastoma Citation[7]. Thus, both cellular and clinical studies show the potential usefulness of hyperthermia as a radiosensitizer.
Although the phenomenon of heat-induced radiosensitization has been well established and studied in cells in culture, in animal tumour models and within the clinic in the treatment of human tumours, the details of the underlying mechanism(s) remain to be elucidated. A number of studies have shown that exposure to hyperthermia interferes with the repair of radiation-induced DNA lesions and, thus, it is currently accepted that inhibition of DNA repair is a major mechanism underlying heat-induced radiosensitization. However, the details of the mechanisms of such inhibition by heat are currently unknown Citation[8].
Any effort to increase the efficacy of the clinical applications of this combined modality must consider aspects of heat-induced radiosensitization other than the hyperthermia-induced inhibition of radiation-induced DNA damage. Repeated heating of tumours can have two other consequences. One is the development of transient thermotolerance, while the other is the selection and/or induction of cell populations that are permanently heat-resistant. The later process has not yet been verified in vivo. However, heat-resistant sub-populations have been selected after repeated cycles of heat shock and regrowth of the surviving tissue culture cells Citation[9–14]. Both transient and permanent heat resistance have the potential to affect the efficacy of the combined modality of heat and radiation. The extent of heat-induced radiosensitization correlates well, but not completely, with the cytotoxicity of the heat treatment Citation[8], Citation[15]. Thus, the reduction of the toxicity of hyperthermia in thermotolerant cells has been associated with a reduction of heat-induced radiosensitization in some but not all cases Citation[16], Citation[17]. The impact of permanent heat resistance on heat-induced radiosensitization has not been studied extensively. Heat-induced radiosensitization was not altered in the permanently heat-resistant cells isolated from RIF-1 transplantable mouse tumour cells Citation[13], Citation[18].
The goal of the present study was to examine further the relationship between permanent heat resistance and heat-induced radiosensitization. Two heat-resistant cell lines that were derived from the same wild-type cell line, HA-1, whose heat-resistance arises by two different mechanisms were chosen for study. One permanently heat-resistant cell line, HR-1, was isolated after repeated heatings at 43°C followed by selection after a single acute heat treatment at 45°C Citation[12]. Permanent heat resistance of these cells is associated with the increased constitutive expression of hsc70, a member of the hsp70 family expressed under normal growth conditions in HA-1 cells, as a consequence of the amplification of the structural gene that encodes hsc70 Citation[12], Citation[19], Citation[20]. Another cell line, OC-14, was selected from HA-1 cells for resistance to H2O2 Citation[21]. Resistance to H2O2 has been shown to be associated with the amplification of the structural gene encoding catalase, as well as two-to-four fold increases in the activity of several cellular anti-oxidants, including glutathione, glutathione transferase, superoxide dismutase and glutathione peroxidase Citation[22], Citation[23]. Interestingly, the OC-14 cell line is resistant to exposures to 43°C but not 45°C Citation[24]. The mechanism of heat resistance in the OC-14 cells line is not known at the present time; it has been established that the heat-resistance of OC-14 cells is not associated with increased constitutive expression of any of the major heat shock proteins Citation[25].
This report examined heat-induced radiosensitization, the effect of heat on the ionizing radiation induced alterations in the ability of nuclear DNA to undergo super-coiling changes and heat-induced alterations in nuclear protein binding in these cell lines.
Materials and methods
Cell culture
Parental wild-type HA-1 and heat-resistant HR-1 cells and OC-14 cells were grown in Eagle's MEM supplemented with Earle's basic salt solution, 10% heat inactivated (56°C, 30 min) foetal calf serum (Hyclone), 100 units ml−1 of penicillin and 100 µg ml−1 streptomycin, in a humidified 5% CO2 atmosphere at 37°C. Exponentially growing cells were used throughout all experiments. In some experiments a known number of cells were plated on monolayers of lethally irradiated HeLa cells.
Heating, radiation exposure and clonogenic survival assays
Monolayers of exponentially growing cells in tissue culture dishes at 80–85% confluence had their medium changed with pre-warmed (37°C), pH equilibrated medium right before experiments, the dishes were sealed with parafilm and then incubated for 10 min in a 37°C waterbath. Immediately after such pre-treatment, the dishes were immersed into a circulating water bath with precise temperature control (±0.1°C) for various lengths of time at 43 or 45°C.
Control cells or pre-heated cells were exposed to ionizing radiation from a Pantak (Branford, CT) model PMC1000 X-ray generator operated at 220 kV and 10 mA. Exposure to radiation was performed in a chamber containing 5% CO2 at 37°C. For heated cells, the time interval between the end of the exposure to hyperthermia and the irradiation was less than 2 min. Some of the experiments were performed with pre-plated cells.
Following various experimental treatments, cells were removed from the dishes by trypsinization and counted using a Coulter Counter (Beckman Coulter, Fullerton, CA) that had been calibrated with a haemocytometer. After appropriate dilutions, a number of cells that would yield 100–200 surviving colonies were plated in triplicate. Colonies were allowed to grow 9–11 days, fixed in fresh Carnoy's solution for 10 min, stained with crystal violet and counted under a dissecting microscope.
Fluorescent halo assay
The fluorescent halo assay was performed with modifications to assay for radiation-induced changes in the super-coiling status of DNA loops associated with the nuclear matrix, by determining their response to titration with increased levels of propidium iodide (PI) Citation[26–28]. Single cell suspensions of control or heated cells obtained by trypsinization were washed three times in Spinner's salt solution (0.4 g KCl, 0.1 g MgSO4, 6.8 g NaCl, 2.2 g NaHCO3, 1.4 g NaH2PO4, 1 g glucose per litre at pH 7.4) at 4°C, collected by centrifugation at 500× G at 4°C and resuspended at a concentration of 1–2 × 105 ml−1 in Spinner's in 15 ml centrifuge tubes and kept on ice. One tube served as control and the others were subjected to graded doses of γ irradiation, on ice from a 137Cs irradiator (J.L. Shepard Associates, Glendale, CA, model Mark I) at an irradiation rate of 3.57 Gy min−1.
Nucleoids were obtained by mixing 250 µl each of the cell suspensions with a lysis solution containing 3 M NaCl, 10 mM EDTA, 20 mM Tris Base, pH 8.0, 0.025% Triton X-100 and various concentrations of PI, in a four well Lab Tek Slide at room temperature (NuncMiles Scientific, Naperville, IL). At this concentration of Triton X-100 lysis was complete in 15 min and provided stable nucleoids whose halos could be measured up to 1 h. After 15 min of incubation in the dark at room temperature (23–25°C), the DNA halos were visualized with an inverted fluorescent microscope, using an excitation wavelength of 520–570 nm and a 20× achromatic objective. The images were collected with a CCD camera and captured with a frame grabber in a PC. Fields of uniform focus were selected for image capture. The captured images were analysed using an AutoHalo macro program (Computer Imaging Associates, Madison, WI), running on an Optimas image analysis platform (Media Cybernetics). Size calibration of the system was performed using a stage micrometer. The software measures four diameters for each particle (one vertical, one horizontal and two diagonal) and then calculates the average maximum halo diameter. Previous work has shown that for measuring relative changes in nucleoid diameter comparing maximum diameter is equivalent to comparing average diameters Citation[29]. A minimum of 50 halos was analysed for each PI concentration. PI response curves were generated three or more times for each cell line and/or experimental condition examined and the data were averaged.
Heat-induced excess nuclear proteins
Nuclei were prepared from control and heated cells as described before Citation[30]. Briefly, nuclei isolated by standard procedures Citation[31] were treated for 5 min at 37°C with RNAse (1 mg ml−1, boiled for 3 min before use), stained with 0.1 mg ml−1 of fluorescein isothiocyanate (FITC) for 1 h at 4°C and then stained further with 35 µg ml−1 of propidium iodide (PI) overnight at 4°C. The ratio of green FITC fluorescence (protein content) to red fluorescence (DNA content) was monitored with a Becton-Dickinson FACS IV equipped with a dual detection system. At least 20 000 nuclei were analysed from each sample.
For electrophoretic analysis, cells were pre-labelled with 15 µCi of 35S-methionine overnight in medium containing 1% the normal concentration of methionine. Nucleoids were then prepared from control cells and cells exposed to 43°C for various lengths of time as described Citation[32]. Briefly, control and heated cells were harvested as described above for the fluorescent halo assay. The final cell pellet was resuspended in 2 ml of Spinner's salt solution and diluted with an equal volume of the FHA lysis solution and lysis was allowed to proceed for 20 min at room temperature. Nucleoids were then collected by sedimentation at 45 000 rpm for 30 min at 4°C. The supernatants were discarded; the pellets were resuspended in 50 µl of spinner's salt solution and then digested with 2 µg of electrophoretically purified DNAase (Worthington, DPFF) for 10 min, at 4°C. The solution was diluted with equal volume of 2× Laemmli sample buffer and then boiled for 5 min. One dimensional gel electrophoresis in the presence of SDS was performed exactly as described Citation[32]. The samples contained material from an equal number of cells.
Results
Heat survival
The HR-1 cell line is resistant to all elevated temperatures tested, 42–45°C Citation[12]. In contrast, the OC-14 cell line is resistant to 43°C hyperthermia, but not resistant to 45°C hyperthermia Citation[24]. A comparison of the complete heat response of HA-1, HR-1 and OC-14 cells at 43 and 45°C was performed (). The HR-1 cells display a significant amount of heat resistance at both 43 and 45°C, while the OC-14 cells did not display any significant resistance to 45°C. These results confirm earlier initial characterization of these cell lines Citation[12], Citation[24].
Figure 1. The effect of exposure to 43°C (a, b) and 45°C (c, d) for various lengths of time on the clonogenic survival of wild-type HA-1 cells and the heat-resistant variants HR-1 (a, c) and OC-14 (b, d). Heating and clonogenic survival assays were performed as described in Materials and methods. The figure represents the average of three independent experiments; the error bars represent ±1 SEM.
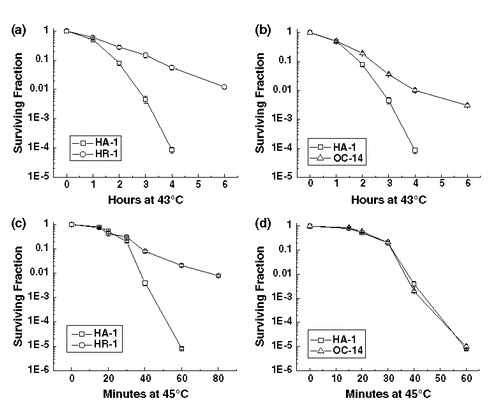
Effect of hyperthermia combined with radiation
The response to heat combined with ionizing radiation examined after exposure to 43°C for 60 min is illustrated in . First, the response to ionizing radiation alone is very similar, if not identical, in all of the three cell lines examined. Thus, the mechanisms involved in conferring heat resistance to the HR-1 and OC-14 cells do not alter their sensitivity to ionizing radiation. Secondly, the interaction of heat and ionizing radiation leads to increased killing in all three cell lines. However, both the HR-1 and OC-14 cells lines display reduced heat-induced radiosensitization. Similar effects were observed after an initial heat dose of 30, 45 and 75 min at 43°C (Spitz and Laszlo, unpublished results). The effect of prior exposure to 45°C for 15 min on the radiation response of these three cell lines was also determined. The results () indicate that, while there is a reduction of heat-induced radiosensitization in the HR-1 cells when compared to their wild-type counterparts, the response of the OC-14 cells is similar to that of the wild-type HA-1 cells. Similar results were obtained with 10 and 20 min exposures to 45°C (Laszlo and Davidson, unpublished results). The data illustrated in and have been normalized to the cell killing induced by the initial sensitizing heat treatment. Thus, the reduction of heat-induced radiosensitization in the heat-resistant cell lines appears not to be simply due to the effect of resistance to heat-induced cell killing alone. Experiments performed with pre-plated cells indicated that the trypsin effect Citation[1] was not involved in the differences in heat-induced radiosensitization (Laszlo and Harvey, unpublished results).
Figure 2. Heat induced radiosensitization at 43°C. Control (open symbols) and heated (43°C, 1 h) (closed symbols) HA-1 (circles), HR-1 (squares) and OC-14 (triangles) cells were exposed to increasing doses of ionizing radiation and clonogenic survival determined. Heating, irradiation and clonogenic survival assays were performed as described in Materials and methods. The figure represents the average of three independent experiments; the error bars represent ±1 SEM.
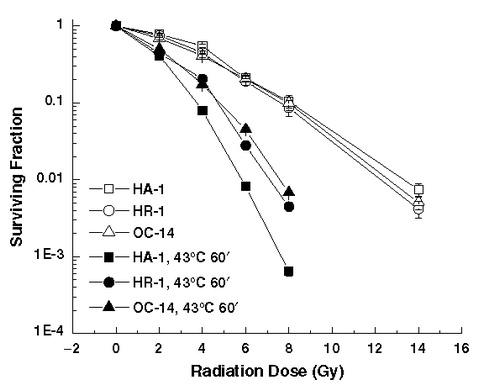
Figure 3. Heat induced radiosensitization at 45°C. Control (open symbols) and heated (45°C, 15 min) (solid symbols), HA-1 (squares), HR-1 (circles) and OC-14 (triangles) cells were exposed to increasing doses of ionizing radiation and clonogenic survival was determined. Heating, irradiation and clonogenic survival assays were performed as described in Materials and methods. The figure represents the average of four independent experiments ±1 SEM.
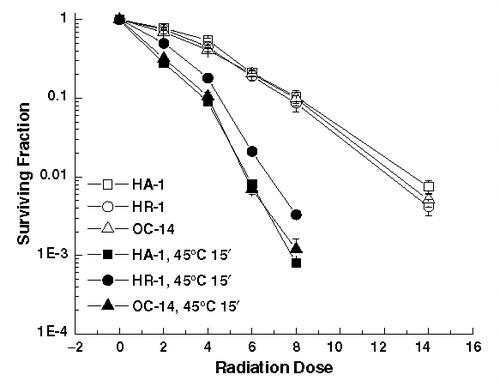
A quantitative evaluation of these effects is presented in , where the thermal enhancement ratios (TER) at a survival of 10% and 1% for the three different cell lines are presented. The TERs for the wild-type HA-1 cells are similar to that reported with other rodent cells in culture Citation[1]. The TER was reduced for both OC-14 and HR-1 cells heated at 43°C, but only in the HR-1 cells heated at 45°C.
Table I. Alterations in heat induced radiosensitization in HA-1, HR-1 and OC-14 cells.
Recovery from heat-induced radiosensitization in the three cell lines was examined next. Two sets of results support the possibility that faster recovery from heat-induced radiosensitization could account for the differences seen above. Faster recovery from heat-induced perturbations in cellular physiology has been found to be associated with the heat resistance of HR-1 and OC-14 cells Citation[25], Citation[30], Citation[33] and faster recovery from heat-induced radiosensitization has been observed in transiently thermotolerant cells Citation[29], Citation[34]. The recovery from heat-induced radiosensitization was determined from treatments involving isodoses of ionizing radiation () and from doses of radiation with similar initial survival (), all in combination with an initial exposure of 1 h to 43°C. Under both of these experimental conditions, recovery from heat-induced radiosensitization in the heat-resistant cells did not appear to be significantly faster in the heat-resistant cell lines than in their wild-type counterparts. Similar results were obtained with pre-plated cells, eliminating any trypsin effects (Laszlo and Harvey, unpublished results). Thus, faster recovery from heat-induced radiosensitization does not account for the decreased levels of heat-induced radiosensitization observed in HR-1 and OC-14 cells.
Figure 4. Recovery from heat-induced radiosensitization. (a) Control (open symbols) and heated (43°C, 1 h) (solid symbols) HA-1 (squares), HR-1 (circles) and OC-14 (triangles) cells were exposed 6, 8 to 8 Gy of ionizing radiation respectively immediately after heating or allowed to recover at 37°C for up to 12 h before irradiation and then plated for clonogenic survival. Heating, irradiation and clonogenic survival experiments were performed as described in Materials and methods. A representative experiment of two independent repeats is illustrated in (b) Control (open symbols) and heated (43°C for 1 h) (solid symbols) HA-1 (squares), HR-1 (circles) and OC-14 (triangles) cells were exposed to Gy of ionizing radiation after various times of recovery from heating and then treated as described above. A representative experiment of two independent repeats is illustrated in the figure.
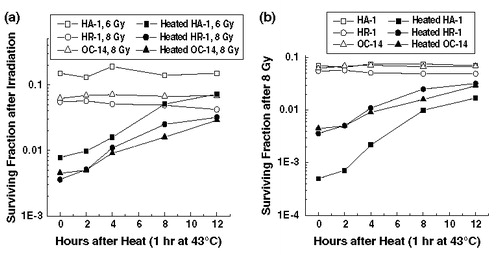
Heat induced alterations in ionizing radiation induced DNA damage as detected by the fluorescent halo assay
The ionizing radiation-induced DNA damage was examined as monitored by the fluorescent halo assay (FHA) in order to gain insight into the mechanisms involved in the alterations in heat-induced radiosensitization in OC-14 and HR-1 cells. The FHA measures the ability of DNA loops attached to the nuclear matrix to undergo supercoiling changes due to the intercalation of the fluorescent dye, propidium iodide (PI), as assayed by fluorescent microscopy and image analysis Citation[26], Citation[28], Citation[35], Citation[36]. With increasing concentrations of PI, the endogenous left-handed negative supercoils unwind maximally and then rewind into right-handed positive supercoils when the PI concentration is increased further. The effect of the different concentrations of PI is visualized as a ‘halo’ emanating from a ‘core’ using a fluorescent microscope. This rewinding process is incomplete in cells exposed to ionizing radiation, due to the single and double strand DNA breaks induced by such treatment. The inhibition of the rewinding process is manifested as increased halo diameter at concentrations above 10 µg ml−1 of PI and is dose-dependent up to 20 Gy of IR Citation[26], Citation[36]. Exposure to heat alone leads to a slight decrease in halo diameter Citation[29], presumably due to heat-induced changes in protein associations with the nucleoid Citation[32]. Exposure to a combination of radiation and heat leads to a ‘masking’ of the radiation-induced reductions in positive super-coiling capacity: the inhibition of the rewinding by increased concentrations of PI after a combination of heat and radiation is less than that observed with radiation alone Citation[37].
The effect of prior exposure to 43°C for 1 h on super-coiling ability after exposure to 8 Gy was determined in all three cell lines (). The effect of heat alone, a slight reduction in the halo diameter, is similar in the three cell lines (triangles, ). The inhibition of rewinding due to ionizing radiation exposure is also similar in the three cell lines (squares, ). This result is consistent with the finding that the response of these three cell lines to ionizing radiation is similar ( and ). In HA-1 cells, the exposure to a combination of heat and IR (diamonds, ) is associated with a smaller inhibition of rewinding than exposure to IR alone (squares vs. diamonds, ). This is the heat-induced ‘masking’ of the ionizing radiation-induced DNA damage detected by the FHA Citation[37]. Surprisingly, this masking effect is significantly diminished in the HR-1 and OC-14 cells (squares vs. diamond, and ). Similar results were obtained when cells were exposed to other heat doses (43°C for 30, 45 min and 90 min) or other doses of ionizing radiation (4, 12 and 16 Gy) (Laszlo, Davidson and Harvey, unpublished observations).
Figure 5. The effect of heat on ionizing radiation induced alterations in nucleoid halo diameters in cells exposed to 43°C for 1 h. Nucleoids were prepared from control (○), irradiated control (□), heated (Δ) and irradiated heated (⋄) HA-1 cells (a), HR-1 cells (b) and OC-14 cells (c) and were then exposed to various concentrations of propidium iodide to perform the FHA assay as described in materials and methods. In HA-1 cells, the DNA in the nucleoids from control and heated cells rewinds the most, radiation alone the least, with the radiation and heat combination intermediate (the masking effect). In both HR-1 and OC-14 cells, the inhibition of rewinding in irradiated control and heated cells is similar to that of radiation alone (lack of the masking effect). The figure represents the average of three independent experiments ±1 SEM.
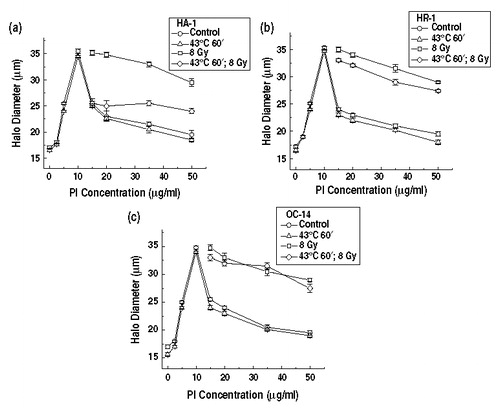
In order to establish whether or not there is an association of the reduction of the masking effect with resistance to heat-induced radiosensitization, the effect of exposure to 45°C on the response of the FHA to ionizing radiation was examined in the three different cells lines, with the results illustrated in . While the heat-induced masking effect is observed in all three cell lines under these experimental conditions, the magnitude of the masking effect is only reduced in HR-1 cells (compare diamonds vs. squares in and vs. ). Since OC-14 cells do not display diminished heat-induced radiosensitization under these conditions (), there appears to be a relationship between the reduction of heat-induced radiosensitization and a reduction of the masking effect measured by the FHA in HR-1 and OC-14 cells.
Figure 6. The effect of heat on ionizing radiation induced alterations in nucleoid halo diameters in cells exposed to 45°C for 15 min. Nucleoids were prepared from control (○), irradiated control (□), heated (Δ) and irradiated heated (⋄) HA-1 cells (a), HR-1 cells (b) and OC-14 cells (c) and were then exposed to various concentrations of propidium iodide to perform the FHA assay as described in materials and methods. In HA-1 cells, the DNA in the nucleoids from control and heated cells rewinds the most, radiation alone the least, with the radiation and heat combination intermediate (the masking effect). In HR-1 cell only the inhibition of reminding in irradiated heated cells is attenuated (reduced masking effect). The figure represents the average of three independent experiments ±1 SEM.
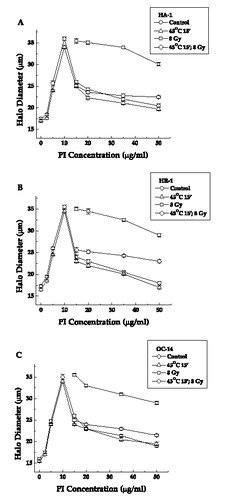
The differences in halo rewinding can be quantitated by using the excess halo diameter (EHD), the total sum of the halo diameters observed at concentrations >10 µg ml−1 of PI Citation[27], Citation[37]. The results from this type of analysis are summarized in and and . The EHD for control cells of the three different cell lines that have been irradiated with 8 Gy is quite similar, although it is somewhat smaller for the OC-14 cells ( and ). The masking effect is detected in HA-1 cells treated at both 43 and 45°C prior to irradiation. In HR-1 and OC-14 cells, the masking effect is almost completely absent at 43°C, while at 45°C it is reduced only in HR-1 cells. The comparison of these results with those obtained for clonogenic survival after exposure to heat and radiation ( and ) indicates the following pattern: resistance to heat-induced radiosensitization is correlated with a modification of the masking effect in the IR-induced DNA damage detected by FHA. On the one hand, when resistance to heat-induced radiosensitization is observed, when OC-14 cells were exposed to 43°C or HR-1 cells are exposed to 43°C and 45°C, the masking effect is reduced. On the other hand, if there is no resistance to heat-induced radiosensitization, as in HA-1 cells exposed to 43 or 45°C or OC-14 cells exposed to 45°C, the magnitude of the observed masking effect is not affected.
Figure 7. The effect of heating at 43°C (a) and at 45° (b) prior to irradiation on the inhibition of DNA super-coil rewinding in nucleoids from HA-1, HR-1 and OC-14 cells. The excess halo diameter (EHD) after 8 Gy of irradiation of non-heated and heated cells from each cell line was computed by adding the halo diameters measured at 15, 20, 35 and 50 µg of PI from control cells and subtracting this value from the equivalent sum from the irradiated cells. The data represents the average of three independent experiments ±1 SEM.
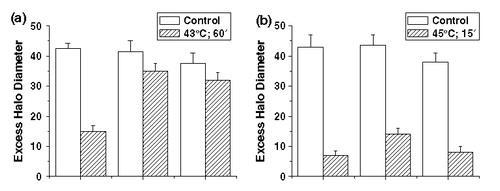
Table II. Summary of the quantitation of heat on the FHA in three cell lines.
Heat-induced excess nuclear proteins
It is well established that one of the immediate effects of exposure to elevated temperature is an increase in the protein content of the nucleus due to protein aggregation (reviewed in Citation[38–40]). These observations suggest a model in which the masking effect is due to increased binding of nuclear proteins to DNA-nuclear matrix anchoring regions, which in turn plays a role in interfering with template availability for the DNA repair machinery. Thus, the reduction of the masking effect in the heat-resistant cell lines could be a consequence of alterations in the heat-induced binding of proteins and/or alterations in the composition of the proteins involved. This notion was tested by comparing the heat-induced increase in nuclear protein content in HA-1 and OC-14 cells to 43°C (). No significant differences in the levels of heat-induced excess nuclear proteins in these cell lines were observed under conditions that resistance to heat-induced radiosensitization () and a reduction of the masking effect were observed (). Therefore, it was concluded that the difference in the masking effect between HA-1 and OC-14 cells is probably not due to differences in the magnitude of heat-induced nuclear protein aggregation, as determined by these techniques. Similar results have already been obtained for HR-1 cells Citation[30].
Figure 8. The effect of exposure to 43°C on nuclear protein content. Nuclei were prepared from HA-1 (□) and OC-14 (Δ) cells exposed to 43°C for 0, 45 and 90 min and the relative nuclear protein content was determined as described in materials and methods. A representative experiment of two independent repeats is illustrated in the figure.
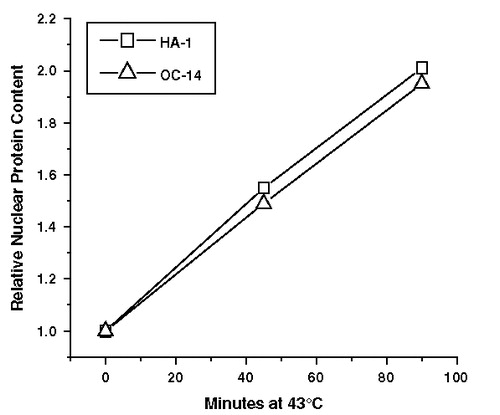
Since alterations in the heat-induced change in total nuclear protein content did not reflect the measured changes in heat-induced radiosensitization or the masking effect, it was examined if there are any alterations in the levels of individual polypeptide bands associated with nucleoids isolated from heated cells as resolved by gel electrophoresis. The profiles of the labelled proteins of nucleoids prepared from control and heated (60 min at 43°C), HA-1 and OC-14 cells that were pre-labelled with 35S-methionine are shown in . The increase in nuclear proteins in control vs. heated cells is detected; however, there are no significant differences between the profiles of the proteins found in nucleoids isolated from heated HA-1 and OC-14 cells. This impression was confirmed by densitometric tracings of the autoradiograms illustrated in (data not shown). Similar results were obtained when nucleoids isolated from control and heated HA-1 and HR-1 cells were compared (Citation[30], and data not shown).
Figure 9. The effect of exposure to 43°C on the profile of nucleoid proteins. Nucleoids were prepared from control and heated HA-1 and OC-14 cells that were pre-labelled with 35S-methionine and analysed by 1-dimensional PAGE in the presence of SDS, as described in Materials and methods. Equal number of particles was loaded in each lane. The slight difference in the intensity of the samples from HA-1 and OC-14 cells is due to the fact that OC-14 cells are somewhat smaller and contain less protein per cell than HA-1 cells. The figure represents an autoradiograph obtained after 24 h exposure. The arrows mark the migration of the corresponding molecular weight markers.
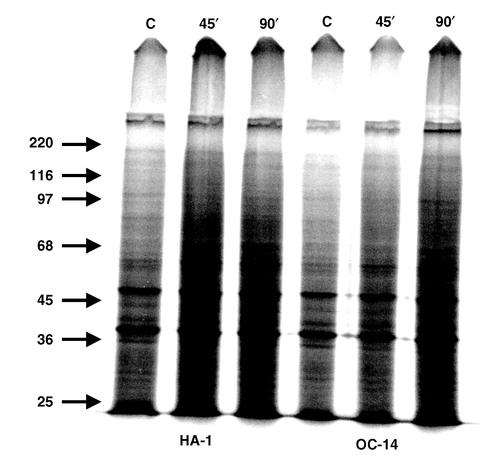
Discussion
This study addresses the nature of the relationship between mechanisms that protect cells from heat-induced cell killing and mechanisms that protect cells from heat-induced radiosensitization. There are at least three possibilities: (1) Resistance to heat-induced radiosensitization and heat involve the same pathways; (2) Resistance to radiosensitization and heat share some pathways; and (3) The resistance to heat and to radiosensitization is mediated by distinct pathways. The data presented in this report considered in the context of previously published work provide strong evidence for the second possibility.
If the mechanisms of resistance to heat-induced radiosensitization and heat alone were the same, then there would be a close correlation between heat-induced cell killing and heat-induced radiosensitization. The permanently heat-resistant phenotype was not accompanied by alterations in heat-induced radiosensitization in case of heat-resistant variants isolated from RIF cells Citation[13], Citation[18] or from V-79 cells Citation[41]. In the authors’ hands, a series of heat-resistant cell lines that over-express hsp 27 (a gift of Dr J. Landry, University of Laval, Quebec) also did not display differences in heat-induced radiosensitization (Laszlo and Davidson, unpublished observations). Glycerol, a chemical heat protector, did not affect heat-induced radiosensitization Citation[42]. The results obtained with transiently thermotolerant cells are equivocal: in some cases there is protection, while in others there is not, even in studies with the same cell line (reviewed in Citation[17]). Continuous exposure of HeLa cells to 41–41.5°C induced cell killing that increases continuously up to 32 h of incubation, while the maximum heat-induced radiosensitization is achieved after 4 h of incubation and does not increase upon further incubation Citation[43]. NSY human colon cancer cells that can grow at 41.1°C are sensitized to radiation within 2 h of exposure to 41.1°C, while the HCT-15 cells that are sensitive to 41.1°C are only slightly sensitized after a 24-h incubation at this temperature Citation[44]. Membrane fluidization, which sensitizes cells to heat-induced cell killing, does not sensitize cells to heat-induced radiosensitization Citation[45], Citation[46]. In contrast to the reported more rapid recovery from heat-induced radiosensitization in transiently thermotolerant cells Citation[29], recovery was similar in wild type and heat-resistant cells ( and ). This observation also supports the notion that the permanently heat-resistant state and the transiently thermotolerant state are different Citation[30], Citation[33].
Since, in the above described examples, resistance to heat-induced cell killing does not lead to resistance to heat-induced radiosensitization and, conversely, sensitization to heat-induced cell killing does not increase heat-induced radiosensitization, the pathways that lead to resistance to heat-induced radiosensitization do not appear to be identical to those that lead to resistance to heat-induced cell killing.
The possibility that the mechanisms leading to resistance to heat-induced cell killing and radiosensitization are completely independent also appears to be unlikely. First, there are several examples of resistance to heat-induced cell killing being accompanied by resistance to heat-induced radiosensitization. This study found that heat-induced radiosensitization is reduced in the HR-1 cell line at both 43 and 45°C. There are several instances in which resistance to heat-induced radiosensitization has been reported in transiently thermotolerant cells Citation[17]. Secondly, sensitization to heat-induced cell killing by procaine has been reported to sensitize cells to heat-induced radiosensitization Citation[47]. Thirdly, the results with OC-14 cells represent a combination of both of the above possibilities. These cells are resistant to heat-induced radiosensitization after exposure to 43°C () when they are resistant to heat-induced cell killing (); resistance to heat-induced radiosensitization in OC-14 cells under these experimental conditions has also been confirmed by others Citation[48]. On the other hand, OC-14 cells are not resistant to heat-induced radiosensitization when they do not display heat-resistance, i.e. exposure to 45°C ( and ). Given that there are several cases in which resistance to heat-induced radiosensitization is accompanied by parallel changes in resistance to heat-induced cell killing, it appears that resistance to these two effects of hyperthermia cannot be completely independent and, thus, must share some common pathways.
Current notions concerning heat-induced radiosensitization implicate heat-induced alterations in the repair of the DNA damage induced by ionizing radiation, rather than alterations in the levels of initial radiation-induced DNA damage Citation[8], Citation[15]. One possibility is that heat-induced alterations in the accessibility of the DNA damage to the repair machinery are involved, rather than heat-induced alterations in the DNA repair machinery itself, as first suggested by Warters and Roti Roti Citation[49]. Such alterations in the accessibility of DNA-damage are thought to involve heat-induced protein aggregation in the nucleus Citation[38–40]. Heat-induced protein aggregation in the nucleus impairs the accessibility and/or the processivity of the DNA repair machinery at the site of the DNA lesion induced by ionizing radiation, perhaps similar to the way in which radiation induced DNA damage is masked in nucleoids isolated from heated cells ( and ). The observation that a reduction in the masking effect is associated with a reduction of heat-induced radiosensitization is consistent with this model, since reduced masking would result in the abatement of the inhibition of the initial steps in DNA repair as a consequence of a reduction of the heat-induced alterations in template accessibility.
Alterations in the repair of ionizing radiation-induced DNA damage in heated cells could also arise because of the alteration of chromatin structure in heated cells. It has been reported that alterations in the structure of the chromatin regions involved in the anchoring of super-helical loop domains of DNA to the nuclear matrix affect the intrinsic radiosensitivity of a variety of cell lines Citation[28], Citation[50–52]. The effects of heat on the structure of such chromatin domains correlate with the perturbation and/or inhibition of nuclear processes involving DNA that are thought to involve the nuclear matrix, including replication and transcription Citation[40], Citation[53], Citation[54]. In this report, the effect of hyperthermia on the repair of ionizing radiation induced DNA damage that is detected by the FHA was compared in wild type and heat-resistant cell lines. The masking by heat of the radiation effect, which is manifested by a reduction in the EHD observed in cells exposed to radiation alone, was measured by methods described in Citation[28], Citation[37].
It was found that the heat-induced masking effect was significantly reduced in HR-1 and OC-14 cells after an exposure to 43°C prior to irradiation when compared with the HA-1 parental cells ( and , ). OC-14 cells are resistant to exposures at 43°C but not 45°C Citation[24] (). Correspondingly, there is no resistance to heat-induced radiosensitization in the OC-14 cells exposed to 45°C (). On the other hand, HR-1 cells are resistant to exposures at 45°C Citation[12] () and display resistance to heat-induced radiosensitization at this temperature (). FHA analysis of HR-1 and OC-14 cells exposed to 45°C indicated that a reduction of the masking effects only occurred in HR-1 cells ( and ). Therefore, there appears to be a relationship between the inhibition of the masking effect and resistance to heat-induced radiosensitization in these two cell lines.
One of the nuclear effects of hyperthermia is the aggregation of proteins that become associated with the chromatin and nuclear matrix, as described above. Such increased association of nuclear proteins with chromatin in heated cells has been shown to inhibit the repair of ionizing radiation-induced base damage and repair of UV induced DNA damage Citation[49], Citation[55]. This is due to interference with the accessibility of the DNA damage to the corresponding repair machineries, rather than a direct heat effect on them. These results have been interpreted to indicate that heat-induced protein aggregation in the nucleus results in a steric hindrance of template accessibility to the repair systems. The results indicating that the masking effect is reduced in heat-resistant cells displaying resistance to heat-induced radiosensitization are consistent with this notion. However, no differences were detected in the total excess nuclear proteins levels induced by heat between the wild-type and heat-resistant cells () Citation[30] or any major compositional differences in protein content of the nucleoids isolated from heated wild-type and heat-resistant cells () Citation[30]. Thus, the alterations in the masking effect associated with resistance to heat-induced radiosensitization may be the consequence of alterations in the binding of the excess nuclear proteins that are not detected by the analysis of total nuclear proteins () or by the one-dimensional electrophoretic analysis illustrated in or alterations in post-transcriptional modifications. Alternatively, the binding of some of the individual heat-induced excess nuclear proteins at the sites of DNA loop attachment to the nuclear matrix may be altered in the heat-resistant cell lines. This possibility is supported by the report that, while the heat-induced increase in nuclear matrix associated proteins was 1.7-fold in heated cells, the increase in the levels of specific proteins involved in DNA replication and repair ranged from 40–1400-fold Citation[54].
The work presented here is the first report of a cell line-specific alteration in the magnitude of the heat-induced masking of ionizing radiation-induced damage that is detected by the FHA. It is of significant interest to determine whether or not such alterations in the masking effect that correlate with the reduction of the initial magnitude of heat-induced radiosensitization in two different heat-resistant cell lines are also associated with alterations of the repair of ionizing radiation-induced DNA damage. Furthermore, identification of the specific nuclear proteins that may be involved in the alterations in the masking effect associated with resistance to heat-induced radiosensitization should aid in further delineation of the mechanisms involved in heat-induced radiosensitization.
Acknowledgements
This work was supported by P01-CA- 75556. DRS was also supported by HL-51469.
References
- Raaphorst GP. Thermal radiosensitization in vitro. Biology of thermal potentiation of radiotherapy Hyperthermia and oncology, M Urano, E Douple. VSP, UtrechtThe Netherlands 1989; 2: 17–53
- Overgaard J, Gonzalez DG, Hulshof MCCM, Arcangeli G, Dahl O, Mella O, Bentzen SM. Randomised trial of hyperthermia as adjuvant to radiotherapy for recurrent or metastatic malignant melanoma. Lancet 1995; 345: 540–543
- Overgaard J, Gonzalez DG, Hulshof MCCH, Arcangeli G, Dahl O, Mella O, Bentzen SM. Hyperthermia as an adjuvant to radiation therapy of recurrent or metastatic malignant melanoma. A multicentre randomized trial by the European Society for Hyperthermic Oncology. International Journal of Hyperthermia 1996; 12: 3–20
- Vernon CC, Hand JW, Field SB, Machin D, Whaley JB, Van Der Zee J, Van Putten WLJ, Van Rhoon GC, Van Dijk JDP, Gonzalez DG, Liu FF, Goodman P, Sherar M. Radiotherapy with or without hyperthermia in the treatment of superficial localized breast cancer: Results from five randomized controlled trials. International Journal of Radiation Oncology, Biology & Physics 1996; 35: 731–744
- Van Der Zee J. Heating the patient: a promising approach?. Annals of Oncology 2002; 13: 1173–1184
- Van Der Zee J, Gonzalez GD, Van Rhoon GC, Van Dijk JD, Van Putten WL, Hart AA. Comparison of radiotherapy alone with radiotherapy plus hyperthermia in locally advanced pelvic tumours: A prospective, randomised, multicentre trial. Dutch Deep Hyperthermia Group. Lancet 2000; 355: 1119–1125
- Sneed PK, Stauffer PR, McDermott MW, Diedrich CJ, Lamborn KR, Prados MD, Chang S, Weaver KA, Spry L, Malec MK, Lamb SA, Voss B, Davis RL, Wara WM, Larson DA, Phillips TL, Gutin PH. Survival benefit of hyperthermia in a prospective randomized trial of brachytherapy boost +/− hyperthermia for glioblastoma multiforme. International Journal of Radiation Oncology, Biology & Physics 1998; 40: 287–295
- Kampinga HH, Dikomey E. Hyperthermic radiosensitization: Mode of action and clinical relevance. International Journal of Radiation Biology 2001; 77: 399–408
- Harris M. Temperature-resistant variants in clonal populations of pig kidney cells. Experimental Cell Research 1967; 46: 301–314
- Harris M. Stable heat-resistant variants in populations of Chinese hamster cells. Journal of the National Cancer Institute 1980; 64: 1495–1501
- Tao TW, Calderwood SK, Hahn GM. Stable heat-resistant clones selected from wild-type and surface variants of B-16 melanoma. International Journal of Cancer 1983; 32: 533–535
- Laszlo A, Li GC. Heat-resistant variants of Chinese hamster fibroblasts altered in expression of heat shock protein. Proceedings of the National Academy of Sciences (USA). 1985; 82: 8029–8033
- Hahn GM, Van Kersern I. Isolation and initial characterization of thermoresistant RIF tumour cell strains. Cancer Research 1988; 48: 1803–1807
- Laszlo A, Venetianer A. Heat resistance in mammalian cells: Lessons and challenges in ‘Stress of Life: Adaptation from Molecules to Man’, P Csermely. Annals of the New York Acad of Sciences. 1998; 851: 169–179
- Biology of thermal potentiation of radiotherapy. Hyperthermia and oncology, M Urano, E Douple. VSP, The Netherlands 1989; 2
- Konings AWT. Effects of heat and radiation on mammalian cells. Radiation, Physics, and Chemistry 1987; 30: 339–349
- Henle KJ, Nagle WA, Moss AJ. Effect of thermotolerance on the cellular heat-radiation response. Biology of thermal potentiation of radiotherapy. Hyperthermia and oncology, M Urano, E Douple. VSP, UtrechtThe Netherlands 1989; 2: 53–81
- Kampinga HH, Konings AWT, Evers AJ, Brunsting JF, Misfud N, Anderson RL. Resistance to heat radiosensitization and protein damage in thermotolerant and thermoresistant cells. International Journal of Radiation Biology 1997; 71: 315–326
- Laszlo A. Regulation of the synthesis of heat-shock proteins in heat-resistant variants of Chinese hamster fibroblasts. Radiation Research 1988; 116: 427–443
- Chen MS, Featherstone T, Laszlo A. Amplification and altered expression of the hsc70/U14 snoRNA gene in a heat-resistant Chinese hamster cell line. Cell Stress and Chaperones 1996; 1: 47–61
- Spitz DR, Li GC, McCormick ML, Sun Y, Oberley LW. Stable H2O2-resistant variants of Chinese hamster fibroblasts demonstrate increases in catalase activity. Radiation Research 1988; 114: 114–124
- Spitz DR, Adams DT, Sherman CM, Roberts RJ. Mechanisms of cellular resistance to hydrogen peroxide, hyperoxia, and 4-hydroxy-2-nonenal toxicity: The significance of increased catalase activity in H2O2 resistant fibroblasts. Archives of Biochemistry & Biophysics 1992; 279: 249–260
- Hunt CR, Sim JE, Sullivan SJ, Featherstone T, Golden WC, Von Kapp-Herc, Hock RA, Gomez RA, Parsian AJ, Spitz DR. Genomic instability and catalase gene amplification induced by chronic exposure to oxidative stress. Cancer Research 1998; 58: 3986–3992
- Spitz DR, Li GC. Heat-induced cytotoxicity in H2O2-resistant Chinese hamster fibroblasts. Journal of Cell Physiology 1990; 142: 255–260
- Laszlo A, Davidson T, McDonald B, Hsu V, Sim JE, Spitz DR. On the mechanism of heat resistance in oxidative stress resistant Chinese hamster cells, Manuscript submitted
- Roti Roti JL, Wright WD. Visualization of DNA loops in nucleoids from HeLa cells: Assays for DNA damage and repair. Cytometry 1987; 8: 461–467
- Kampinga HH, Wright WD, Konings AWT, Roti Roti JL. The interaction of heat and radiation affecting the ability of nuclear DNA to undergo supercoiling changes. Radiation Research 1988; 116: 114–123
- Malyapa RS, Wright WD, Roti Roti JL. Radiation sensitivity correlates with changes in DNA supercoiling and nucleoid protein content in cells of three Chinese hamster cell lines. Radiation Research 1994; 140: 312–320
- Kampinga HH, Turkel-Uygur N, Roti Roti JL, Konings AWT. The relationship of increased nuclear protein content induced by hyperthermia to killing of HeLa S3 cells. Radiation Research 1989; 117: 511–522
- Laszlo A. The thermoresistant state: Protection from initial damage or better repair?. Experimental Cell Research 1992; 202: 519–531
- Higashikubo R, Wright WD, Roti Roti JL. Flow cytometric methods for studying isolated nuclei: DNA accessibility to Dnase I and protein-DNA content. Methods in Cell Biology 1990; 33: 325–336
- Laszlo A, Wright W, Roti Roti JL. Initial characterization of heat-induced nuclear proteins in HeLa cells. Journal of Cell Physiology 1992; 151: 519–532
- Laszlo A, Davidson T, Hu A, Landry J, Bedford J. Putative determinants of the cellular response to hyperthermia. International Journal of Radiation Biology 1993; 63: 569–581
- Stege GJJ, Kampinga HH, Konings AWT. Heat-induced intranuclear protein aggregation and thermal radiosensitization. International Journal of Radiation Biology 1995; 67: 203–209
- Wright WD, Higashikubo R, Roti Roti JL. Fluorescent methods for studying subnuclear particles. Flow Cytometry. Methods in cell biology, Z Darzynkiewicz, HA Crissman. Academic Press, New York 1990; 33: 353–362
- Roti Roti JL, Wright WD, Taylor YC. DNA loop structure and radiation response. Advances in Radiation Biology 1993; 17: 227–259
- Wynstra JH, Wright WD, Roti Roti JL. Repair of radiation-induced DNA damage in thermotolerant and nonthermotolerant HeLa cells. Radiation Research 1990; 124: 85–89
- Roti Roti JL, Laszlo A. The effect of hyperthermia on cellular macromolecules. Hyperthermia and oncology, M Urano. VNU Scientific Publishers, The Netherlands 1998; 1: 13–56
- Kampinga HH. Thermotolerance in mammalian cells: Protein denaturation and aggregation, and stress proteins. Journal of Cell Science 1993; 104: 11–18
- Roti Roti JL, Kampinga HH, Malyapa RS, Wright WD, VanderWaal RP, Xu M. Nuclear matrix as a target for hyperthermic killing of cancer cells. Cell Stress Chaperones 1998; 3: 245–255
- Campbell SD, Kruuv J, Lepock JR. Characterization and radiation response of a heat-resistant variation of V79 cells. Radiation Research 1983; 93: 51–63
- Mivechi NF, Hofer KG. Evidence for separate modes of action in thermal radiosensitization and direct thermal cell death. Cancer 1983; 51: 38–43
- Mackey MA, Anolik SL, Roti Roti JL. Changes in heat and radiation sensitivity during long duration, moderate hyperthermia in HeLa S3 cells. International Journal of Radiation Oncology, Biology & Physics 1992; 24: 543–550
- Xu M, Wright WD, Higashikubo R, Wang L-L, Roti Roti JL. Thermal radiosensitization of human tumour cell lines with different sensitivities to 41.1°C. International Journal of Hyperthermia 1999; 15: 279–290
- Konings AWT, Ruifrok ACC. Role of membrane lipids and membrane fluidity in thermosensitivity and thermotolerance of mammalian cells. Radiation Research 1985; 102: 86–98
- Wolters H, Kelholt D, Konings AWT. Effect of hyperthermia on the repair of sublethal radiation damage in normal and membrane fatty acid substituted fibroblasts. Radiation Research 1987; 109: 294–302
- Djordjevic B. Variable interaction of heat and procaine in potentiation of radiation lethality in mammalian cells of neoplastic origin. International Journal of Radiation Biology 1983; 43: 399–409
- Bradbury CM, Locke JE, Wei SJ, Rene LM, Karimpour S, Hunt C, Spitz DR, Gius D. Increased activator protein 1 activity as well as resistance to heat-induced radiosensitization, hydrogen peroxide, and cisplatin are inhibited by indomethacin in oxidative stress-resistant cells. Cancer Research 2001; 61: 3486–3492
- Warters RL, Roti Roti JL. Excision of x-ray-induced thymine damage in chromatin from heated cells. Radiation Research 1979; 79: 113–121
- Taylor YC, Duncan PG, Zhang X, Wright WD. Differences in the DNA supercoiling response of irradiated cell lines from ataxia-telangiectasia versus unaffected individuals. International Journal of Radiation Biology 1991; 59: 359–371
- Yasui LS, Fink TJ, Enrique AM. Nuclear scaffold organization in the x-ray sensitive Chinese hamster mutant cell line, xrs5. International Journal of Radiation Biology 1994; 65: 185–192
- Woudstra EC, Roesink JM, Rosemann M, Brunsting JF, Driessen C, Orta T, Konings AWT, Peacock JH, Kampinga HH. Chromatin structure and cellular radiosensitivity: A comparison of two human tumour cell lines. International Journal of Radiation Biology 1996; 70: 693–703
- VanderWaal RP, Thampy G, Wright WD, Roti Roti JL. Heat-induced modifications in the association of specific proteins with the nuclear matrix. Radiation Research 1996; 145: 746–753
- VanderWaal RP, Griffith CL, Wright WD, Borelli MJ, Roti Roti JL. Delaying S-phase progression rescues cells from heat-induced S-phase hypertoxicity. Journal of Cell Physiology 2001; 187: 236–243
- Sakkers RJ, Brunsting JF, Filon AR, Kampinga HH, Konings AW, Mullenders LH. Altered association of transciptionally active DNA with the nuclear-matrix after heat shock. International Journal of Radiation Biology 1999; 5: 875–883