Abstract
Introduction: For treatment of oesophageal cancer, neo-adjuvant locoregional hyperthermia (HT) has been applied in combination with chemotherapy (ChT) ± radiotherapy (RT) at the institute. Until now, 26 patients were treated within a completed phase I study combining HT with ChT and 29 patients within an ongoing phase II study combining HT with ChT + RT.
Methods: HT was given with the 70 MHz AMC-4 waveguide system. Initially, oesophageal temperatures were measured using multi-sensor thermocouple probes (TCs) inside a nasogastric tube (NT), but the question arose whether these measurements were reliable enough to quantify the achieved tumour temperatures accurately. Presently, TCs are mounted on the outside of an inflatable balloon catheter (BC) for better intra-luminal fixation and better contact with the tumour. During 14 treatment sessions in four patients TCs inside a NT and mounted on a BC were used simultaneously. Data from these 14 treatment sessions were used to compare temperature and Specific Absorption Rate (SAR) measurements (‘ΔT-measurements’) using NTs or BCs. To determine the predictive value of the local SAR for the tumour temperatures achieved during treatment, the relation between the initial ΔT and steady state temperature (SST) was evaluated.
Results: There was a strong correlation between the temperature measured in the NT (Ttube) and the temperature measured with a BC (Tballoon): R = 0.88 ± 0.13. However, Ttube was on average ∼1°C higher than Tballoon and there was a large variation between the different treatments in the relation between both measurements, rendering Ttube a probably unreliable measure for tumour temperatures. The correlation between the ΔT measured in the NT (ΔTtube) and with a BC (ΔTballoon) was rather weak: R = 0.46 ± 0.25. The correlation between the initial ΔT and the SST was much stronger for the BC measurements, R = 0.78 ± 0.19, than for the NT measurements, R = 0.61 ± 0.23. Thus, ΔTballoon has a higher predictive value for the achieved tumour temperatures than ΔTtube. Both ΔT and SST were generally higher for the NT measurements than for the BC measurements, suggesting an over-estimation of tumour temperatures. Averaged over all treatments in the phase I trial using a NT (20 treatments) or a BC (45 treatments), T90 was significantly higher when measured with a NT.
Conclusion: Oesophageal temperature and SAR (ΔT) measurements inside a NT are less reliable than BC measurements. These artefacts are due to bad thermal contact with the tumour tissue and are, therefore, not specific for thermocouple thermometry. For reliable temperature or SAR measurements inside lumina or cavities good thermal contact must be assured, e.g. by using a balloon catheter.
Introduction
Hyperthermia treatments aim at effective tumour heating (41–45°C), without over-heating normal tissue. Accurate temperature measurements during treatment are essential for optimal treatment control and quantification of the achieved tumour temperatures. In addition, measurements of the absorbed power distribution in the patient, i.e. the Specific Absorption Rate (SAR), are also useful to obtain insight in the power steering capabilities of the hyperthermia system used. At this institute locoregional hyperthermia is given using the 70 MHz AMC-4 phased array system Citation[1]. Clinical experience has led to standard power settings of the hyperthermia system for different tumour sites. Phase settings are adjusted such that a maximal E-field is measured at the tumour location, which corresponds to a local SAR maximum in the tumour.
Clinical thermometry can be performed (minimally) invasively and non-invasively. Invasive thermometry methods require implantation of catheters in tissue. This ensures good thermal contact with tissue, but also involves implant risks for the patient Citation[2]. Minimally invasive thermometry is generally performed by intra-luminal or intra-cavitary measurements, e.g. in oesophagus, vagina, bladder or rectum. Several methods for intra-luminal or intra-cavitary thermometry are available such as measurements inside catheters Citation[3], (naso)gastric tubes or using inflatable balloon catheters Citation[4]. Although sophisticated non-invasive thermometry methods such as radiometry Citation[5], ultrasound Citation[6] and MRI Citation[7–9] are advancing, it is still necessary to obtain accurate and reliable temperature information from traditional (minimally) invasive methods Citation[10].
Temperature measurements can be performed by thermocouples Citation[3], Citation[11], thermistor probes Citation[12–14] or fibre-optic probes Citation[9], Citation[14], Citation[15]. An advantage of thermocouples is the possibility of combining multiple sensors in one probe. A disadvantage of metallic probes, such as thermocouples and thermistors, is their susceptibility to EM disturbances Citation[11], Citation[16]. In turn, metallic probes can cause perturbations in the EM-field itself Citation[16], thus possibly affecting the heating pattern in the patient. However, also catheters, in which thermometry probes are inserted, can cause perturbations in the EM-field Citation[17]. The influence of EM perturbations on temperature measurements can be minimized by proper alignment of the probes with respect to the EM-field, preferably perpendicular to the principal EM-field component Citation[16], by using ferrite beads around the probe leads Citation[11], and by measuring during power-off of the hyperthermia system. Fibre-optic probes are not disturbed by the EM-field, but may be subject to stability problems Citation[18]. Fibre-optic probes and thermistor probes perform single point measurements, but scanning the probe along the catheter track also enables thermal mapping.
At this institute, multi-sensor copper-constantan thermocouple probes are used for both temperature and SAR measurements Citation[11]. To eliminate the influence of self-heating of the thermocouples, the power of the AMC-4 system is switched off for a few seconds before temperature or SAR measurements are performed. At the start of treatment the local SAR is estimated by applying a short power pulse of the AMC-4 system and measuring the temperature rise (ΔT) after the pulse. During the rest of the treatment, temperatures are measured every 30 s. During locoregional hyperthermia treatments, intra-luminal thermometry is applied in pre-existing body cavities, such as bladder, vagina and rectum for pelvic malignancies and the oesophagus for oesophageal cancer.
During hyperthermia treatments of oesophageal cancer patients, thermocouples were initially inserted in a nasogastric tube, which is relatively easy to apply and not very uncomfortable for the patient. Presently the thermocouples are mounted on an inflatable balloon catheter for better intra-luminal fixation and better contact with the tumour. These measurements are assumed to be more accurate due to optimal thermal contact with the tumour tissue. During several treatment sessions both intra-luminal measurement techniques have been combined. In the present study it was investigated whether the measurements inside the tube were reliable enough to quantify tumour SAR and temperature accurately, in comparison with the measurements with the balloon catheter. It is obvious that the thermal contact with the oesophageal tumour tissue is optimal for thermocouples mounted on an inflated balloon catheter, thus providing reliable measurements of tumour temperatures and SAR. At best, the thermal contact and consequently the accuracy of temperature and SAR measurements of thermocouples inside a nasogastric tube are as good as with a balloon catheter.
Methods
Hyperthermia treatments and thermometry
Between January 1999–February 2002, a phase I study applying neo-adjuvant locoregional hyperthermia combined with concurrent chemotherapy (cisplatin and etoposide) for operable oesophageal cancer was performed at the institute Citation[4]. A total of 26 patients were treated with a median tumour length of 6 cm (range 2–12 cm). Hyperthermia was given with the 70 MHz AMC-4 phased array system Citation[1]. Temperatures were monitored rectally, intra-oesophageally at the tumour level and intra-muscularly near the spine, using multi-sensor copper-constantan thermocouple probes (TCs), with a measurement accuracy of 0.005°C and surrounded by a Teflon coating (Ø 0.9 mm, Ella-CS, Czech Republic). To eliminate disturbances of the TCs by the EM-field a minimum of 12 ferrite beads per thermocouple probe was used. The upper and lower boundaries of the oesophageal tumour position were determined during endoscopic examination prior to each hyperthermia treatment session, with an accuracy of <0.5 cm. Oesophageal thermometry was placed accordingly. The position of the nostril or the front teeth was marked on the thermometry device, in order to monitor this position during the hyperthermia treatment session with an accuracy of <0.5 cm. Initially, oesophageal temperatures were measured using two 21-point TCs (spacing 1 cm) inside two opposing lumina of a three-lumen silastic nasogastric tube (Sherwood Medical, St. Louis, MO; , configuration C1), but the question arose whether these measurements were reliable enough to quantify the achieved tumour temperatures accurately Citation[19], Citation[20]. After 20 hyperthermia treatments the two TCs were mounted on opposite sides of an inflatable balloon catheter (length 8 cm, diameter 1 cm, Angiomed AG Germany) for better intra-luminal fixation and better contact with the tumour (, configuration C2). The balloon was inflated with air at a pressure of ∼1 atm, which was sufficient for accurate intra-luminal fixation and still far below the burst rate of 10 atm. Measurements with balloon catheters were assumed to be more accurate due to optimal thermal contact with the tumour tissue. During a transitional period of 10 treatment sessions in three patients, one or two TCs inside a nasogastric tube and one or two TCs mounted on a balloon catheter were used simultaneously (, configuration C3).
Figure 1. Schematic representation of the three types of intra-luminal thermometry used for temperature and SAR measurements at oesophageal tumour locations. Left: multi-sensor thermocouple probes (TCs) inserted in two opposing lumina of a three-lumen nasogastric tube (configuration C1). Middle: TCs mounted on an inflatable balloon catheter (configuration C2; for clarity only one TC is drawn in the upper picture). Right: TCs inside a three-lumen nasogastric tube or a regular single lumen nasogastric tube and TCs mounted on a balloon catheter used simultaneously (configurations C3 and C4).
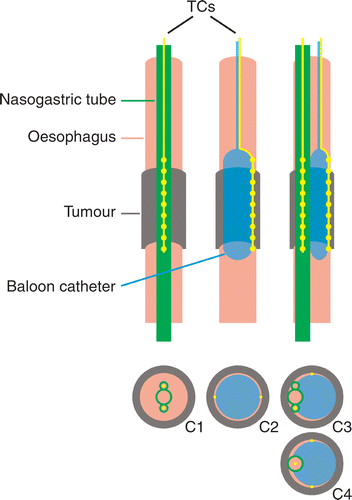
In August 2003 a phase II study applying neo-adjuvant radiotherapy (23 × 1.8 Gy) combined with weekly locoregional hyperthermia (for 5 weeks) with concurrent chemotherapy (carboplatin and paclitaxel) for operable oesophageal cancer was started at the institute Citation[21]. Until now, 29 patients have been treated with a median tumour length of 6 cm (range 2–12 cm) at the start of the hyperthermia treatment. In this study, oesophageal temperatures are standardly measured using two 21-point TCs (spacing 1 cm) mounted on the outside of a balloon catheter (length 8 cm, diameter 1 cm, Angiomed AG Germany, or length 6 cm, diameter 1 cm, Ella-CS Czech Republic; , configuration C2). One patient needed a nasogastric nutrition tube throughout the pre-operative treatment period due to oesophageal stenosis. This was a regular single lumen nasogastric tube and an additional 14-point TC probe (spacing 1 cm) was applied inside this tube during four treatment sessions (, configuration C4). All materials used (nasogastric tubes, balloon catheters, thermocouple probes) were fully biocompatible.
Thus, in a total of 14 treatment sessions in four patients, TCs inside a nasogastric tube and TCs mounted on a balloon catheter were used simultaneously. In this study, data from the 14 treatment sessions combining a tube and a balloon catheter were used to compare the measurements inside the nasogastric tube and the measurements using the balloon catheter. This provides the most reliable comparison, since both measurements were performed during the same treatment session in the same patient. Temperature measurements as well as measurements of the Specific Absorption Rate (SAR) were evaluated. An overview of the different thermocouple (TC) configurations, the number of TCs, patients and treatment sessions, the type of analysis in which the concerned data were used (see above) and the figures in which the results were presented is shown in .
Table I. Overview of the different thermocouple (TC) configurations used in the phase I and phase II study (configuration C1–C4, see ), the number of thermocouple probes used (# TCs), the number of patients (# patients), the number of treatment sessions (# treatment sessions), the type of analysis in which the concerned data were used, and the figure number in which the results were presented.
Measurements
At the start of every treatment session, power and phase settings of the AMC-4 system were adjusted in order to create a local SAR maximum in the tumour. A measurement of the local SAR (W kg−1) was performed, usingwith ct the specific heat capacity of the tissue (J kg−1°C−1) and ΔT the temperature rise (°C) after a 30 s power pulse of 800 W (‘ΔT-measurement’) Citation[11], measured during power-off. From here on the term ‘ΔT ’ will be used to indicate this type of measurement. During the rest of the treatment, temperatures were measured every 30 s, during a 5 s power-off period of the AMC-4 system. According to protocol, the steady state period of 60 min started as soon as one of the tumour measurement points reached 41°C or alternatively after an induction period of 30 min. To determine the predictive value of SAR (ΔT) for the tumour temperatures achieved during the treatment, the relation between the initial ΔT and the steady state temperature (SST) was evaluated.
Data analysis
The relation between the temperatures measured in the nasogastric tube (Ttube) and the temperatures measured with the balloon catheter (Tballoon) was assumed to be linear and was therefore described byresulting in a slope and an offset for each of the 14 treatment sessions. Furthermore, the relation between slope and Toffset for all 14 treatment sessions was examined. The ideal situation would be if slope = 1 and Toffset = 0, implying Tballoon = Ttube, or at least if a fixed relation between Ttube and Tballoon was found.
The relation between the initial ΔT measured at the start of treatment and the mean SST during the treatment per thermosensor can be determined by considering Pennes’ bio heat transfer equation Citation[22] at two different moments of the treatment. For homogeneous tissue this energy balance iswith ρt the tissue density (kg m−3) and ct the specific heat capacity (J kg−1°C−1). The first term on the right hand side represents the conduction in tissue, with kt the thermal conductivity of tissue (W m−1°C−1). The second term on the right hand side represents the perfusion, with cb the specific heat of blood, Wb the volumetric perfusion rate of tissue (kg m−3 s−1) and Tart the arterial temperature, which is usually taken equal to the body core temperature (37°C). The absorbed power density is represented by P (W m−3). In the absence of sharp temperature gradients, which is a valid assumption in the close vicinity of the oesophagus, conduction can be neglected. At the start of treatment the perfusion term can also be neglected and equation (3) becomes
with ΔT the initial temperature rise and Δt the duration of the ΔT-measurement (30 s). Since SAR ≡ P/ρt, equation (3a) is equivalent to equation (1). At steady state equation (3) becomes
with SST the steady state temperature. Assuming a constant power density P during the treatment and substituting for tumour tissue ρt = 1050 kg m−3, ct = 3639 J kg−1°C−1, cb = 3840 J kg−1°C−1 Citation[23], the resulting relation between ΔT and SST becomes
It should be noted that the perfusion Wb is dependent on the (steady state) temperature in a non-linear fashion Citation[24], Citation[25], resulting in a non-linear relation between ΔT and SST. Based on the curves presented by Song Citation[24], the perfusion Wb was assumed to be exponentially increasing with SST in the range of interest:
with Wb,0 the basal volumetric perfusion of resting tissue (∼1 kg m−3 s−1 Citation[24]). The exponential term represents the increase in perfusion upon heating, quantified by the parameters Wb,1 (kg m−3 s−1) and A (°C−1). Equations (4) and (5) were used to reconstruct the relation between ΔT and SST obtained from the measurements.
To investigate to what extent the type of thermometry might have influenced the reported temperatures, the achieved tumour temperatures for all treatments of the phase I study using a nasogastric tube (20 treatments) and all treatments using a balloon catheter (45 treatments) were analysed.
Statistics
Data are presented as mean ± SD. The correspondence between two parameters (e.g. x1 and x2) was expressed as the correlation coefficient R, as determined using SPSS 12. The correlation coefficient squared (R2) quantifies the amount of explained variance (R2 = 1 corresponds to 100% explained) and is therefore a measure for the predictive value of x1 for x2, or vice versa. Similarly, the measure is used to quantify the quality of model fits. Tumour temperatures were quantified by T90, T50 and T10, the temperature at least achieved in 90%, 50% and 10% of the tumour, respectively. For this purpose the tumour temperatures were taken into account, measured every 30 s during the 60 min steady state period, by all thermosensors at tumour level. For the first 20 hyperthermia treatments of the phase I study the reported tumour temperatures were calculated using nasogastric tube measurements, for the remaining 45 treatments of the phase I study balloon catheter measurements were used Citation[4]. In the phase II study only balloon catheter measurements were used for calculation of the reported tumour temperatures. Differences in tumour temperatures between nasogastric tubes and balloon catheters were examined by an unpaired, two-tailed Student T-test. A p-value (probability of obtaining the results by chance) of <0.05 was considered significant.
Results
Measured ΔT and temperature profiles
Typical ΔT profiles along the thermocouple tracks measured simultaneously in a nasogastric tube and with a balloon catheter in an individual patient are shown in . Tumour length was 6 cm in this patient. There is a reasonable correspondence between the two measurements in a number of points along the tracks. However, the deviation between tube and balloon measurement was on average 0.17 ± 0.15°C (mean ± SD), which corresponded to a deviation of 40 ± 31% relative to the balloon catheter measurements (0.43 ± 0.10°C).
Figure 2. Typical ΔT profiles along the thermocouple tracks measured simultaneously in a nasogastric tube and with a balloon catheter, in an individual patient with a tumour length of 6 cm.
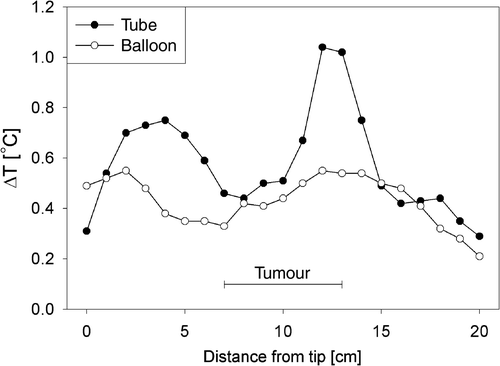
Typical developments of the temperature profiles along the thermocouple tracks during the same treatment session are shown in . Temperature profiles measured at the start of the treatment, at the start of the steady state period and at 15, 30 and 60 min of the steady state period are shown. There is a fair correspondence between the tube () and the balloon measurements () for most of the profiles. However, it can be seen that the temperatures measured inside the tube were generally slightly higher than those measured with the balloon. In this case the deviations were gradually increasing from 0.06 ± 0.05°C at the start of the treatment to 0.97 ± 0.77°C (mean ± SD) at 60 min of steady state. This corresponded to deviations in the order of 35 ± 27%, relative to the balloon catheter measurements (39.3 ± 0.8°C) with respect to the body core temperature (37°C).
Figure 3. Typical development of temperature profiles along the thermocouple tracks during treatment, measured simultaneously in a nasogastric tube (a) and with a balloon catheter (b), at the start of the treatment, at the start of the steady state period (SST) and at 15, 30, and 60 min of the steady state period. Tumour length was 6 cm.
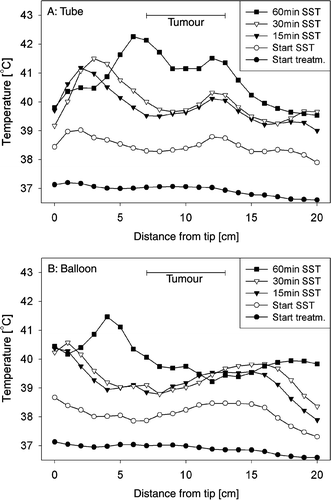
Histograms of the differences in ΔT and temperature measurements between corresponding thermosensors in nasogastric tubes and on balloon catheters are shown in . The difference in ΔT was on average 0.14 ± 0.22°C and the difference in temperature was on average 1.1 ± 1.0°C, averaged over the 14 treatment sessions.
Relation between Ttube and Tballoon
There was a strong and significant correlation between the temperatures measured in the nasogastric tube (Ttube) and the temperatures measured with the balloon catheter (Tballoon): the correlation coefficient was R = 0.88 ± 0.13 (mean ± SD, range 0.63–1.00), averaged over the 14 treatment sessions. Nevertheless, Ttube was generally higher than Tballoon, on average ∼1°C in the observed temperature range (37–42°C). Expressing the relation between Ttube and Tballoon as described in equation (2) resulted in slope = 0.66 ± 0.26 (range 0.26–1.08) and Toffset = 11.7 ± 9.7°C (range −4.0–26.7°C), averaged over the 14 treatment sessions. In other words, there is a large variation between the different treatment sessions. shows two extreme cases together with the average relation: Tballoon = 0.66·Ttube + 11.7°C.
Figure 5. (a) Relation between the temperatures measured in a nasogastric tube (Ttube) and with a balloon catheter (Tballoon) for two different treatment sessions (symbols). The relation between both measurements was described by a linear fit (equation (2)) per treatment session (thick solid lines). The line of unity (upper solid line) and the average relation between Tballoon and Ttube for all 14 treatment sessions (dashed line) are also shown. (b) Relation between Toffset and slope from equation (2) for the 14 treatment sessions.
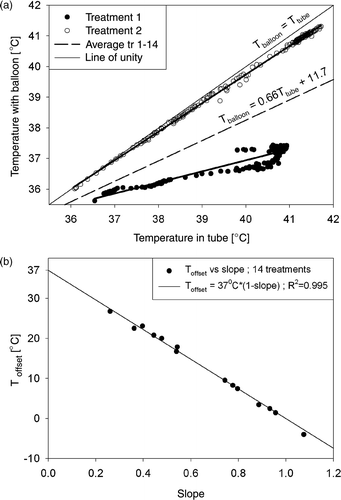
There was, however, a very strong relation (R = −0.998) between Toffset and slope for the 14 treatment sessions (): Toffset = 37°C·(1 − slope). Substitution of this relation into equation (2) results in (Tballoon − 37°C) = slope·(Ttube − 37°C), indicating a proportional relation between the temperature rises with respect to the average basal body temperature (37°C), measured with a nasogastric tube and a balloon catheter. Note the large range of slope and Toffset in .
Relation between ΔTtube and ΔTballoon
The correlation between the ΔT measured in the nasogastric tube (ΔTtube) and the ΔT measured with a balloon catheter (ΔTballoon) was rather weak: the correlation coefficient was R = 0.46 ± 0.25 (mean ± SD, range 0.07–0.88), averaged over the 14 treatment sessions.
Relation between ΔT and SST
The correlation between the initial ΔT (as a measure for SAR, see equation (1)) measured at the start of a treatment and the mean steady state temperature (SST) during the treatment was not very strong for nasogastric tube measurements (ΔTtube ↔ SSTtube): R = 0.61 ± 0.23 (mean ± SD, range 0.20–0.88). This correlation was much stronger for balloon catheter measurements (ΔTballoon ↔ SSTballoon): R = 0.78 ± 0.19 (mean ± SD, range 0.47–0.97). Thus, ΔTballoon has a higher predictive value (R2 = 0.64 ± 0.28) for the tumour temperatures achieved during the treatment than ΔTtube (R2 = 0.41 ± 0.25). Both correlations were significant (p < 0.05). shows the relation between ΔT and SST for all 14 treatment sessions fitted with the model described in equations (4) and (5). The quality of the model fit was stronger for the balloon catheter measurements ( = 0.49) than for the nasogastric tube measurements (
= 0.19).
Figure 6. (a) Relation between ΔT and SST for nasogastric tube and balloon catheter measurements. The depicted lines are model fits using equations (4) and (5). (b) Reconstructed perfusion as a function of temperature for tube and balloon catheter measurements, together with the perfusion of tumour and muscle tissue. All curves have been normalized to the values at 37°C. The curves for tumour and muscle perfusion have been reproduced from Song Citation[24].
![Figure 6. (a) Relation between ΔT and SST for nasogastric tube and balloon catheter measurements. The depicted lines are model fits using equations (4) and (5). (b) Reconstructed perfusion as a function of temperature for tube and balloon catheter measurements, together with the perfusion of tumour and muscle tissue. All curves have been normalized to the values at 37°C. The curves for tumour and muscle perfusion have been reproduced from Song Citation[24].](/cms/asset/63f87c63-4eb1-4458-b93b-5ea16de47d89/ihyt_a_193093_f0006_b.gif)
The reconstructed relations between perfusion (Wb) and SST, as determined from the model fits, were characterized by = 4.17 kg m−3 s−1,
= 3.1·10−7 kg m−3 s−1 and Atube = 2.79°C−1, and
= 5.85 kg m−3 s−1,
= 7.2·10−6 kg m−3 s−1 and Aballoon = 2.75°C−1, for the nasogastric tube and the balloon catheter measurements, respectively. Both relations between Wb, normalized to the perfusion at 37°C
, and SST are shown in , together with the relative perfusions for muscle and tumour tissue as reproduced from Song Citation[24]. The curve for balloon catheters appeared in better correspondence with the data presented by Song Citation[24]. Again, a ∼1°C difference was observed in the ‘threshold’-temperature above which the perfusion becomes significantly higher than the basal volumetric perfusion at 37°C (∼40.5°C and ∼41.5°C for the balloons and the tubes, respectively).
Tumour temperatures T90, T50, T10
Both ΔT and SST were generally higher for nasogastric tube measurements than for balloon catheter measurements (). This probably suggests an over-estimation of tumour temperatures achieved during the treatment. Averaged over all treatments in the phase I trial using a nasogastric tube (20 treatments) and all treatments using a balloon catheter (45 treatments), the tumour temperatures were indeed somewhat higher when measured with a nasogastric tube, but with a smaller spread, as indicated by the T90, T50 and T10 in (mean ± SD). The difference between tube and balloon measurements was significant for T90 ( = 39.44 ± 0.52°C vs
= 38.96 ± 0.76°C, p = 0.01, Student T-test).
Figure 7. Tumour temperatures measured with nasogastric tubes (20 treatments) or balloon catheters (45 treatments), quantified by T90, T50 and T10 (minimal, mean and maximal temperature, respectively), averaged over all treatments of the phase I study (mean ± SD). The difference between tube and balloon measurements was significant for T90.
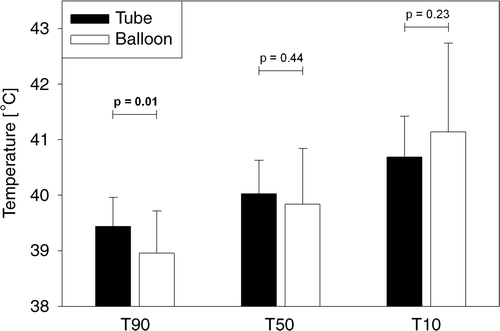
Discussion
During hyperthermia treatments, reliable thermometry is essential for optimal treatment control and effect quantification. Temperature and SAR measurements at oesophageal tumour locations obtained with different intra-luminal thermometry techniques have been compared in this study. The reliability of thermometry inside a nasogastric tube in the oesophagus was investigated and compared with thermometry mounted on an inflatable balloon catheter, fixed in the oesophagus in contact with the tumour.
Thermal contact
Good thermal contact with tissue is of vital importance for reliable temperature and SAR measurements Citation[26]. When thermal contact is not optimal, i.e. when some air is present between thermometry and tissue, artefacts occur especially in SAR measurements, which will also be reflected in measurements of the steady state temperature (SST). When using thermocouple thermometry in poor thermal contact with tissue, the measured SAR and likewise the measured steady state temperatures will be higher than the values in tissue, due to self-heating of the thermocouples and the poor thermal exchange with the surroundings Citation[26]. When using thermometry not disturbed by EM-fields, such as fibre-optic thermometry, but still in poor thermal contact with tissue, the measured SAR and steady state temperatures will be lower than the values in the tissue, due to the absence of power absorption in the probe itself and, again, the poor thermal exchange with the surroundings. Hence, the observed problems are not specific for thermocouple thermometry.
In the case of a thermocouple probe inside a nasogastric tube in the oesophagus, it is hardly avoidable that some air is present in the tube as well as around the tube in the oesophagus. In the case of a thermocouple probe mounted on a balloon catheter that is inflated in the oesophagus, the TC is pressed against the oesophageal wall, ensuring optimal tissue contact. Therefore, the balloon catheter was assumed to provide reliable measurements and the reliability of the tube measurements was determined in relation to the balloon measurements.
Effects on reported tumour temperatures
The measurements inside the nasogastric tube in combination with a balloon catheter, as described in the present study, are probably better than those obtained using a tube only. Due to the presence of the balloon catheter, the nasogastric tube is moved towards the oesophageal wall and in closer contact with the tumour (, configuration C3 or C4). Although the tube is rather rigid, it might be slightly squeezed by inflation of the balloon. This improves thermal contact between the thermocouple inside the tube and the tumour tissue, in comparison with a thermocouple inside a tube with no balloon catheter present. Therefore, obtaining temperature and SAR information from nasogastric tube measurements alone will probably result in even larger deviations or artefacts than observed with the data presented here. This explains the significant difference in T90 between all treatments from the phase I study performed with a nasogastric tube and those performed with a balloon catheter. It is highly unlikely that there have been any other differences in the treatments between these two groups that could have influenced the achieved tumour temperatures. Furthermore, a smaller spread was observed in measured tumour temperatures when using a nasogastric tube than using a balloon catheter. This is due to the fact that a thermocouple inside a tube is to some extent thermally isolated from its surroundings, which filters spatial inhomogeneities and temporal variations in the temperature distribution of the surroundings over the length of the tube and over the duration of the hyperthermia treatment. This resulted in smaller differences between T90, T50 and T10 per treatment for tubes than for balloons, therefore the difference was statistically significant for T90 (∼0.5°C) but not so for T50 and T10 (see ).
Relation between SAR and steady state temperatures
The rationale to explore the relation between the initial temperature rise (ΔT) and the achieved steady state tumour temperatures (SST) for the different thermometry configurations used was to obtain insight (1) in the predictive value of the arrangement of a local SAR maximum in the tumour for adequate hyperthermia treatment in terms of resulting tumour temperatures, (2) in the extent to which artefacts or measurement uncertainties in ΔT-measurements are reflected in SST-measurements and (3) in the temperature-dependent perfusion levels during hyperthermia treatment and the resulting reconstructed perfusion profiles in comparison to literature data. For this purpose, in the first place the measured ΔT and SST data for the different thermometry configurations were studied. The correlation between ΔT and SST was much stronger for balloon catheter measurements than for nasogastric tube measurements, implying a higher intrinsic reliability of the balloon catheter measurements. This means that the predictive value of ΔTballoon for the achieved tumour temperatures of roughly 64% was much higher than the predictive value of ΔTtube of roughly 41%. Nevertheless, the predictive value of ΔTballoon is still only 64%, which is far below 100%, implying that other factors than just SAR play a role in the development of the steady state temperature distribution, such as conduction, perfusion, large blood vessels (e.g. aorta) and bolus cooling. A high SAR value does not always result in a high SST and likewise for low SAR values, which is mainly due to the heterogeneous perfusion values of different tissue types Citation[27]. Furthermore, some of the above mentioned properties, especially perfusion, may vary significantly for individual patients.
According to the data presented here, the relation between ΔT and SST was not linear, but could be approached by a model that encompassed an exponentially increasing perfusion as a function of the temperature, indicating that the temperatures do not persistently rise above certain SAR levels. This is due to a strong increase in perfusion under hyperthermia conditions Citation[24], which partly compensates for the power absorbed in the tumour at high SAR levels, thus preventing a further temperature increase. The reconstructed relation between perfusion and temperature appeared in better correspondence with literature data Citation[24] for balloon catheter measurements than for nasogastric tube measurements, since the perfusion curve for nasogastric tube measurements still increases beyond the temperature (∼42.5°C) where the perfusion should decay according to Song's Citation[24] data. This was not the case for balloon catheter measurements in the observed temperature range, which, however, remained ∼1°C lower than for the nasogastric tube measurements. More importantly, a difference of ∼1°C was observed in the ‘threshold’-temperature above which the perfusion becomes significantly higher than the basal perfusion, causing an equivalent ∼1°C difference in the observed steady state temperatures at comparable SAR levels, between nasogastric tubes and balloon catheters. This again emphasized that the temperatures measured in nasogastric tubes were ∼1°C too high in comparison with balloon catheter measurements.
Limitations of the simplified analytical model
In the present study a simple model was introduced for the relation between SAR (ΔT) and steady state temperatures. Application of this model substantiated the observation that balloon catheter measurements represented true tumour temperatures better than nasogastric tube measurements. Because of its simplicity and the assumptions made in the theoretical description, the model can be susceptible to uncertainties in measurements of ΔT and steady state temperatures, which will be discussed below.
The measured ΔT depended on the local tissue density (ρt), specific heat capacity (ct) and the power density (P), under the assumption that conduction and perfusion can be neglected during ΔT-measurements (equation 3a). The differences in measured ΔT-values between nasogastric tubes and balloon catheters ( and ) may therefore be caused by (1) differences in the thermal (and dielectric) environment of thermocouples inside nasogastric tubes or mounted on balloon catheters, reflected in the values of ρt and ct which are no longer those of pure tumour tissue, but also comprise the properties of the different thermal environment, (2) the fact that in reality the conduction and perfusion may have some influence on ΔT-measurements and (3) small differences in the local power density, e.g. by differences in power absorption of the thermocouples themselves (self-heating).
At steady state it was assumed that ΔT/Δt = 0, rendering the steady state temperatures (SST) dependent on the local power distribution and perfusion (equation 3b). According to protocol, the steady state period of 60 min started as soon as one of the tumour measurement points registered 41°C or alternatively after an induction period of 30 min. However, during this ‘steady state period’ the measured temperatures may not yet be fully steady (), thus ΔT/Δt may be different from zero. Nevertheless, the values of ΔT/Δt during this ‘steady state period’ are generally much smaller (maximally 2°C/15 min ≈ 0.002°C s−1, typically 0.5°C/15 min ≈ 0.0006°C s−1) than those during the initial ΔT-measurements (∼0.5°C/30 s ≈ 0.02°C s−1). Therefore, all temperatures measured every 30 s during this 60 min ‘steady state period’ were taken into account for calculation of the mean steady state tumour temperatures.
Accuracy and stability
Temperature and ΔT-measurements were performed during a 5 s power-off period of the AMC-4 system. Switching off the power may have resulted in slightly lower measured values because of some temperature drop, which was neglected in this study. However, due to EM disturbances of the thermocouples it was not possible to perform reliable temperature measurements during power-on of the AMC-4 system.
For the relation between ΔT and SST a constant power density during the hyperthermia treatment session was assumed (equation 4). The power stability of the AMC-4 hyperthermia system is ± 5 W per channel, i.e. ∼3% of the applied power. The phase stability of the AMC-4 system is ± 3°. Assuming constant properties of the local anatomical structures and tissues over the duration of the hyperthermia treatment session, the local SAR-levels can be expected to be stable within ±5%.
Conclusion
Oesophageal temperatures and SAR (ΔT) measured inside a nasogastric tube are probably less reliable in comparison with measurements using a balloon catheter. There is a relation between tube and balloon measurements, however this relation is unknown and subject to a large spread between different treatments or patients. The tube measurements are on average ∼1°C higher than the balloon measurements. Conclusions regarding differences between nasogastric tube and balloon catheter measurements were mainly based on the results presented in , which showed data from individual measurements from all treatment sessions of all patients, to some extent on the results presented in , which showed data from individual treatment sessions, and to a lesser degree on the results presented in , where the data of all treatment sessions of all patients were pooled. The extent of artefacts in temperature and SAR measurements may be determined by the thermometry technique, but the occurrence of such artefacts is due to the poor thermal contact with the tumour tissue. The present study provides a mainly qualitative indication that temperatures and SARs measured with thermocouples mounted on a balloon catheter correlate better with true values of tumour tissue than those measured with thermocouples inside a nasogastric tube. More exact theoretical models and experimental investigations are necessary for a quantitative estimation of factors influencing the temperature measurement behaviour in specific thermal configurations. This complicated behaviour has only been tentatively taken into account by the present simplified model. In conclusion, for temperature or SAR measurements inside lumina or cavities good thermal contact must be assured, e.g. by using a balloon catheter.
Acknowledgement
This research was supported by the Dutch Cancer Society (grant 2002-2622).
References
- van Dijk JD, Schneider C, van Os R, Blank LE, Gonzalez DG. Results of deep body hyperthermia with large waveguide radiators. Adv Exp Med Biol 1990; 267: 315–319
- van der Zee J, Peer-Valstar JN, Rietveld PJ, de Graaf-Strukowska L, van Rhoon GC. Practical limitations of interstitial thermometry during deep hyperthermia. Int J Radiat Oncol Biol Phys 1998; 40: 1205–1212
- van der Zee J, van Rhoon GC, Broekmeijer-Reurink MP, Reinhold HS. The use of implanted closed-tip catheters for the introduction of thermometry probes during local hyperthermia treatment series. Int J Hyperthermia 1987; 3: 337–345
- Albregts M, Hulshof MC, Zum Vorde Sive Vording PJ, van Lanschot JJ, Richel DJ, Crezee H, Fockens P, van Dijk JD, Gonzalez GD. A feasibility study in oesophageal carcinoma using deep loco-regional hyperthermia combined with concurrent chemotherapy followed by surgery. Int J Hyperthermia 2004; 20: 647–659
- Jacobsen S, Stauffer P. Non-invasive temperature profile estimation in a lossy medium based on multi-band radiometric signals sensed by a microwave dual-purpose body-contacting antenna. Int J Hyperthermia 2002; 18: 86–103
- Straube WL, Arthur RM. Theoretical estimation of the temperature dependence of backscattered ultrasonic power for noninvasive thermometry. Ultrasound Med Biol 1994; 20: 915–922
- Hekmatyar SK, Poptani H, Babsky A, Leeper DB, Bansal N. Non-invasive magnetic resonance thermometry using thulium-1,4,7,10-tetraazacyclododecane-1,4,7,10-tetraacetate (TmDOTA(-)). Int J Hyperthermia 2002; 18: 165–179
- Hoffmann W, Rhein KH, Wojcik F, Noeske R, Seifert F, Wlodarczyk W, Fahling H, Wust P, Rinneberg H. 2 Performance and use of current sheet antennae for RF-hyperthermia of a phantom monitored by 3 tesla MR-thermography. Int J Hyperthermia 2002; 18: 454–471
- Clegg ST, Das SK, Zhang Y, Macfall J, Fullar E, Samulski TV. Verification of a hyperthermia model method using MR thermometry. Int J Hyperthermia 1995; 11: 409–424
- Lagendijk JJ, van Rhoon GC, Hornsleth SN, Wust P, De Leeuw AC, Schneider CJ, van Dijk JD, van der Zee J, Heek-Romanowski R, Rahman SA, Gromoll C. ESHO quality assurance guidelines for regional hyperthermia. Int J Hyperthermia 1998; 14: 125–133
- de Leeuw AA, Crezee J, Lagendijk JJ. Temperature and SAR measurements in deep-body hyperthermia with thermocouple thermometry. Int J Hyperthermia 1993; 9: 685–697
- Bowman RR. A probe for measuring temperature in radio-frequency heated material. IEEE Trans Microwave Theory Tech 1976; 24: 43–45
- Lyng H, Monge OR, Bohler PJ, Rofstad EK. Temperature distribution in locally advanced breast carcinoma during hyperthermic treatment: Relationship to perfusion, vascular density, and histology. Int J Radiat Oncol Biol Phys 1991; 21: 423–430
- Shibasaki M, Kondo N, Tominaga H, Aoki K, Hasegawa E, Idota Y, Moriwaki T. Continuous measurement of tympanic temperature with a new infrared method using an optical fiber. J Appl Physiol 1998; 85: 921–926
- Katzir A, Bowman HF, Asfour Y, Zur A, Valeri CR. Infrared fibers for radiometer thermometry in hypothermia and hyperthermia treatment. IEEE Trans Biomed Eng 1989; 36: 634–637
- Chan KW, Chou CK, McDougall JA, Luk KH. Changes in heating patterns due to perturbations by thermometer probes at 915 and 434 MHz. Int J Hyperthermia 1988; 4: 447–456
- Chan KW, Chou CK, McDougall JA, Luk KH. Perturbations due to the use of catheters with non-perturbing thermometry probes. Int J Hyperthermia 1988; 4: 699–702
- Hand JW. Guidelines for thermometry in clinical hyperthermia. Front Med Biol Eng 1992; 4: 99–104
- van Vulpen M, de Leeuw AA, Van de Kamer JB, Kroeze H, Boon TA, Warlam-Rodenhuis CC, Lagendijk JJ, Battermann JJ. Comparison of intra-luminal versus intra-tumoural temperature measurements in patients with locally advanced prostate cancer treated with the coaxial TEM system: Report of a feasibility study. Int J Hyperthermia 2003; 19: 481–497
- Wust P, Gellermann J, Harder C, Tilly W, Rau B, Dinges S, Schlag P, Budach V, Felix R. Rationale for using invasive thermometry for regional hyperthermia of pelvic tumors. Int J Radiat Oncol Biol Phys 1998; 41: 1129–1137
- Hulshof MC, Crezee J, Zum Vorde Sive Vording PJ, Geijsen D, van Lanschott JJ, Richel DJ. Toxicity and first treatment results after of neo-adjuvant thermal radiochemotherapy in operable oesophageal cancer. 22nd Annual Meeting of the European Society for Hyperthermic Oncology 2005; 26
- Pennes HH. Analysis of tissue and arterial blood temperatures in the resting human forearm 1948. J Appl Physiol 1998; 85: 5–34
- ESHO Taskgroup Committee. Treatment planning and modelling in hyperthermia. a task group report of the European Society for Hyperthermic Oncology. Rome, 1992. Tor Vergata
- Song CW. Effect of local hyperthermia on blood flow and microenvironment: A review. Cancer Res 1984; 44: 4721s–4730s
- Muller-Klieser W, Vaupel P. Effect of hyperthermia on tumor blood flow. Biorheology 1984; 21: 529–538
- Kok HP, Van den Berg CAT, Van Haaren PMA, Crezee J. Artefacts in temperature measurements in cavities during regional hyperthermia. 22nd Annual Meeting of the European Society for Hyperthermic Oncology. 2005; 60–61
- Kok HP, van Haaren PM, Van de Kamer JB, Wiersma J, van Dijk JD, Crezee J. High-resolution temperature-based optimization for hyperthermia treatment planning. Phys Med Biol 2005; 50: 3127–3141