Abstract
Purpose: Heat shock protein 70 (HSP70) is released from tumour cells and stimulates a potent anti-tumour immune response.
Methods: This study examined the role of hyperthermia, including heating conditions from the fever range, the hyperthermia range and the thermal ablation range, in HSP70 release from prostate carcinoma cells. It has observed HSP70 release from human prostate carcinoma cell lines (PC-3 and LNCaP) treated with hyperthermia.
Results: The effects of hyperthermia were complex and appeared to involve at least two mechanisms for HSP70 release. Hyperthermia at 40°C strongly stimulated HSP70 release by an active secretion pathway. However, as temperatures were increased, this rapid secretion pathway became progressively inhibited and by a temperature of 55°C, active secretion was abolished. However, when cells exposed to these heating conditions were allowed to recover at 37°C for 24 h after heating, HSP70 release was observed at the high ablation temperature range and this appeared to be related to a concomitant damage to the plasma membrane.
Conclusions: Thus, at least two mechanisms contribute to HSP70 release during hyperthermia and the relative contribution from each pathway depends on the temperature conditions.
Introduction
Heat shock proteins (HSP) are essential intra-cellular molecular chaperones Citation[1]. However, recent studies show that a fraction of these proteins, normally localized to cytoplasm or nucleus can be released from cells and function as inter-cellular signalling ligands Citation[2]. Even though necrosis is one of the mechanisms of HSP release from cells Citation[3], many cells reportedly undergo active release of HSP70 such as peripheral blood mononuclear cells (PBMC) Citation[4], Citation[5], human colonic adenocarcinoma cells Citation[6], glial cells Citation[7], splanchnic tissues Citation[8] and psychological stressor induced extra-cellular HSP70 release Citation[9]. Several studies have shown that its release is unaffected by inhibitors of common secretory pathways, e.g. monensin or colchicine Citation[10]; monensin, brefeldin A, tunicamycin and thapsigargin Citation[6].
Such extra-cellular HSP70 interacts with immune effector cells though high affinity receptors and can thus orchestrate immune responses Citation[11–14]. Such interactions include binding of free extra-cellular HSP70 to LOX-1 receptors on dendritic cells or association of cell surface HSP70 with CD94 on natural killer cells Citation[12], Citation[15]. This property of HSP70 is of significance in tumour immunology as HSP70 complexed to tumour antigens is an effective component of anti-tumour vaccines Citation[3], Citation[16–20]. Intriguingly, HSP70-peptide complexes activate both the innate and adaptive immune responsesCitation[14], Citation[17], Citation[18]. It has been suggested that HSP70 may be sequentially induced in tumour cells in vivo by therapy and then released from cells undergoing subsequent necrosis in association with tumour antigens and thus mediate anti-tumour immunity Citation[3], Citation[16]. Recent experiments show that HSP70 is also released from prostate carcinoma cells through an active mechanism independently of either the rate of HSP70 synthesis or cell death Citation[19]. Active HSP70 secretion involves translocation though a lysosomal compartment and can be inhibited by lysomotropic agents. The factors required for HSP70 release resemble those involved in IL-1beta secretion and a mechanism involving trafficking through a pathway including transport by ABC family transporters and entry into a secretory lysosome compartment is involved Citation[19].
The present study has examined the relative contributions of HSP70 secretion and passive release after necrosis in accumulation of extra-cellular HSP70. It has examined a range of conditions, including fever range heating (40°C), hyperthermia range heating (43°C, 45°C) and thermal ablation range heating (50°C, 55°C). The studies indicate that fever range, 40°C hyperthermia stimulates active secretion of HSP70 but that increasing temperature inhibits secretion, with complete inhibition observed at 55°C. However, when HSP70 accumulation is examined in cells incubated at 37°C after heating, HSP70 accumulation is observed at the higher temperatures and HSP70 release occurs simultaneously with permeabilization of the plasma membrane as assayed by trypan blue exclusion.
Materials and methods
Cell culture
The prostate carcinoma cell lines PC-3 and LNCaP were cultured respectively in complete Ham's F-12 and RPMI 1640 media supplemented with 10% foetal calf serum (Mediatech, Herndon, VA, USA), glutamine (Invitrogen (Gibco), Carlsbad, CA, USA), penicillin and streptomycin (Invitrogen).
Hyperthermia treatments and HSP70 release
Five million cells at a concentration of 1 × 106 cells per ml (PC-3 or LNCaP) were cultured at 37°C overnight and fed with fresh medium before treatment at 37°C, 43°C, 45°C, 50°C and 55°C for 30 min, 40°C for 6 h and 37°C representing the untreated control group and the heat shock group, respectively. Heat shock was carried out in a circulating water bath and temperature monitored to a resolution of 0.1°C using a high-resolution mercury-in-glass thermometer. Aliquots of medium were harvested at the indicated times in experiments and cleared by spinning at 900 g for 15 min.
Detection of extra-cellular HSP70 by ELISA
Sandwich enzyme-linked immunosorbant assay ELISA was used for detection of HSP70 in supernatants described previously Citation[20]. Briefly, ultra high binding 96-well micro-titer ELISA plates (ThermoLabsystems, Waltham, MA, USA) were coated overnight with HSP70 monoclonal antibody (Stressgen, Ann Arbor, MI, USA) in carbonate buffer followed by three washes in phosphate buffered saline plus 0.1% Tween 20 (PBST; Sigma). The plates were blocked with 1% bovine serum albumin (BSA; Sigma, St. Louis, MO, USA) in PBST followed by three washes in PBST. Samples and recombinant human HSP70 protein (Stressgen) were added to the wells and incubated for 1 h followed by three washes in PBST. Rabbit polyclonal anti-HSP70 (Stressgen) was added to each well and incubated for 1 h followed by three washes with PBST. Anti- rabbit IgG alkaline phosphatase conjugated monoclonal antibody (Sigma) was added to the 96-well plates followed by three washes with PBST. Finally, the substrate p-nitro phenyl phosphate substrate (Sigma) was added and absorbance was read by spectrophotometer (Bio-Rad, Hercules, CA, USA) at absorbances of 405 nm and 650 nm (reference wavelength).
Statistical analysis
Data are shown as mean ± SD. Statistical significance of differences between experimental groups was analysed by using a Student's t-test. Statistical significance was defined as follows: ☆p < 0.05; ☆☆p < 0.01; ☆☆☆p < 0.001.
Results
Rate of HSP70 release from prostate carcinoma cells after heat shock
This study first investigated the rate of HSP70 release in PC-3 and LNCaP cells exposed to hyperthermia. These cell lines were chosen for their contrasting levels of intra-cellular HSP70. It first investigated whether the rate of HSP70 release is related to the overall intra-cellular levels of the protein using PC-3 and LNCaP cells. These cell lines were chosen for their contrasting levels of intra-cellular HSP70. It has been shown previously that while LNCaP express minimal levels of intra-cellular HSP70, PC-3 express HSP70 abundantly even at non-stress temperatures Citation[21]. As a stimulus for HSP70 release, heat shock was used initially, which has previously been shown to cause release of a number of leaderless proteins including FGF-1 and IL-1alpha Citation[22]. Two heat shock conditions were used for the experiments, including 40°C which is in the fever range, physiological, and minimally toxic in tissue culture and stimulates tumour immunity in mic Citation[23], Citation[24] and 43°C which strongly induces transcriptional activation of hsp70 genes Citation[25].
The rate of extracellular HSP70 release in both cell lines after heat shock at 40°C and 43°C was examined next. HSP70 release was assayed in samples of medium by ELISA. As some reports in the literature indicate that HSP70 family members may be released from cells in membrane-bounded vesicles, this study investigated whether treating samples with detergents such as NP-40 or Lubrol affects recovery of extra-cellular HSP70 by causing release of HSP70 from vesicles. However, it did not observe significant changes when medium from PC-3 or LNCaP cells was treated with detergents suggesting that most HSP70 released from these cells is in free solution Citation[19]. The rate of HSP70 release was therefore assayed as free extra-cellular HSP70 released into cell medium. Relative accumulation of extra-cellular HSP70 in PC-3 and LNCaP cells is shown in . For standard heating conditions, this study used 30 min at 43°C as this condition gives maximum hsp gene induction or 6 h at 40°C as these are the conditions which lead to most of the immune effects associated with fever range heating Citation[24]. In each cell line, basal HSP70 release was observed that was higher in PC-3 cells and which was significantly increased in both cell lines after heat shock (). HSP70 accumulation was significantly elevated at both 40°C and at the higher temperature (43°C). In addition, HSP70 release occurred only during the exposure to heat shock and was not markedly increased in the recovery period at 37°C (data not shown).
Figure 1. HSP70 release from prostate carcinoma cells at 40°C and 43°C. HSP70 release following heat shock treatment. 1 × 106 cells per ml PC-3 (a and c) and LNCaP (b and d) cells were incubated at 37°C control group represented by 37 or non-heat shocked cells, 40°C for 6 h represented by 40, and 43°C for 30 min represented by 43. (a) (PC-3) and (b) (LNCaP) represent HSP70 release immediately after heat shock while (c) (PC-3) and (d) (LNCaP) represent HSP70 released 24 h after heat shock. Supernatants were taken and analysed for the presence of HSP70 by ELISA. Statistical analyses compared conditions 37 vs. 43 (*p < 0.05) (a) and 37 vs. 40 (a) and 37 vs. 43 (b) (**p < 0.01) and 37 vs. 40 (***p < 0.001) (b). Data represents the mean ± SD of three independent experiments.
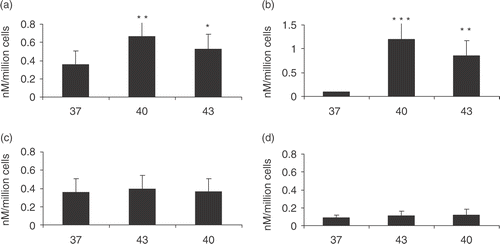
Heat shock induced HSP70 release is inhibited by proteasomal inhibitor MG132
Molecular chaperones mediate two quality control pathways in response to thermal stress. These include either (1) the refolding of denatured proteins by the HSP or (2) the targeting of damaged proteins to the proteasomal pathway of protein degradation Citation[26]. The effect of inhibiting pathway (2) was examined by the proteasomal inhibitor MG132, on HSP70 release (). The rationale behind the experiment was that if HSP70 release is related to the degree of protein damage, inhibition of proteasome function using MG132 would be predicted to increase HSP70 release. However, the contrary finding was observed and MG132 inhibited temperature dependent HSP70 release in a dose dependent manner (). HSP70 release may thus not be linked to the accumulation of unfolded proteins in the cell and may be an independent effect of elevated temperature not simply related to the degree of protein damage.
Figure 2. Effect of exposure to MG-132 on HSP70 release. 1 × 106 cells per ml PC-3 (a) and LNCaP (b) cells were incubated with 0.5 and 5 µM of MG132 (0.5 and 5, respectively) or no treatment with MG132 (43) followed by heat treatment at 43°C for 30 min. The control group was not treated with heat nor MG132. Supernatants were taken and analysed for the presence of HSP70 by ELISA. Statistical analyses compared conditions MG132 0.05 μM vs. 43 30 (*p < 0.05) in (a) and (b); while MG132 5 µM vs. 43 30 (**p < 0.01) in (a) and (b). Data represents the mean ± SD of three independent experiments.
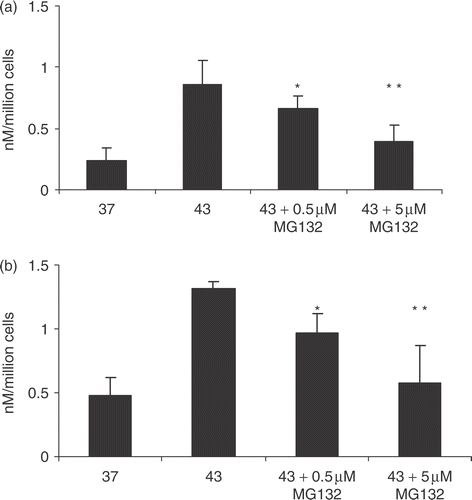
HSP70 release from thermotolerant cells
As many of the targets of heat can be protected in thermotolerant cells, HSP70 release was examined. The rationale for carrying out the experiments was that if HSP70 release is a response to heat damage to proteins, one might expect to observe protection of cells and decreased HSP70 release in thermotolerant cells. This was confirmed in the studies where the thermotolerant cells in exhibited a reduction in HSP70 release comparing Figures and , with the exception of PC3 cells pre-heat shocked at 43°C where there was a slight increase.
Figure 3. HSP70 release in thermotolerant cells. PC3 (a) and LNCaP (b) cells were heat shocked at indicated temperatures and 24 h later the supernatants were discarded and fresh media was added followed by a second heat shock at the indicated temperatures. The supernatants were harvested immediately after the second heat shock and analysed for HSP70 release. 43/43 represents 1st heat shock at 43°C for 30 min followed by a second heat shock 24 h later at 43°C for 30 min; 40/40 represents 1st heat shock at 40°C for 6 h followed by a second heat shock 24 h later at 40°C for 6 h and 43/40 represents 1st heat shock at 4°C for 30 min followed by a second heat shock 24 h later at 40°C for 6 h. Supernatants were taken and analysed for the presence of HSP70 by ELISA. Statistical analyses compared conditions 37 vs. 40/40(*p < 0.05) and 37 vs. 43/43 and 43/40(**p < 0.01) in (a); while 37 vs. 43/43 and 43/40 (*p < 0.05) and 37 vs. 40/40 (**p < 0.01) in (b). There were statistical significance observed when comparing 40 (Figure 1) vs. 40/40 (Figure 3) (**p < 0.01) for the LNCaP cells; 40 (Figure 1) vs. 40/40 (Figure 3) in the PC3 cell group and 43 (Figure 1) vs. 43/43 (Figure 3) in the LNCap cell group (*p < 0.05). Data represents the mean ± SD of three independent experiments.
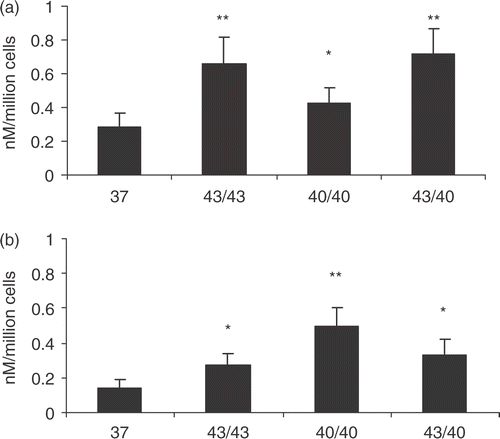
Temperature dependent response to hyperthermia
This study next examined the effects of a range of temperatures on hyperthermia-induced HSP70 release from the prostate carcinoma cell lines ( and ). It examined heat shock conditions including: physiologic fever range heating (40°C), hyperthermia range heating (43°C, 45°C) and thermal ablation range heating (50°C, 55°C). In PC-3 cells, HSP70 release measure immediately after heating was maximal at 40°C and was also elevated at 43°C (). (However, this is somewhat misleading as the heating at 40°C is for 6 h rather than 30 min as with the other temperatures; the 6 h condition was included as this is the condition which leads to maximum HSP70 release. HSP70 release after 30 min at 40°C is slightly less than release at 43°C.) However, as temperatures were increased above 43°C, HSP70 release decreased progressively and was below the control level by 55°C (). These data are consistent with HSP70 release by active secretion and suggest that increasing temperature above 43°C leads to progressive thermal inactivation of the secretion pathway (). In addition, there would be much more opportunity for a variety of cellular processes to occur at 40°C due to the length of time in heating of 6 h compared to all the other times of 30 min. However, when extra-cellular HSP70 was assayed at 24 h after recovery from heat a different pattern was seen (). Significant levels of HSP70 release were observed at 45°C, 50°C and 55°C (). At each of these temperatures significant numbers of cells took up trypan blue, indicating a compromised plasma membrane and indeed, 100% of cells were trypan blue positive at 50°C and 55°C (). When these experiments were repeated in LNCaP cells, an almost identical pattern of response was observed indicating rapid HSP70 secretion at 40°C and 43°C and delayed release at higher temperatures that occurs in cells in which trypan blue uptake is high (). Minimal differences were observed in trypan blue uptake immediately after the heat shock (data not shown).
Figure 4. HSP70 release and trypan blue uptake in PC-3 cells exposed to temperatures from 37–55°C. PC3 cells were heat treatments at 37°C, 43°C, 45°C, 50°C and 55°C for 30 min and 40°C for 6 h. Supernatants were harvested immediately after the heat treatment (a) and 24 h after the heat treatment (b) and tested for the presence of HSP70 by ELISA. Trypan blue uptake, 4C, was analysed 24 h after the heat treatments. Statistical analyses compared 37 vs. 45 and 37 vs. 43 (a) 37 vs. 43, 50, 55 (b) (*p < 0.05); 37 vs. 43 (a) and 37 vs. 45 (b) (**p < 0.01); 37 vs. 40 (a) (***p < 0.001). Data represents the mean ± SD of three experiments done in triplicate.
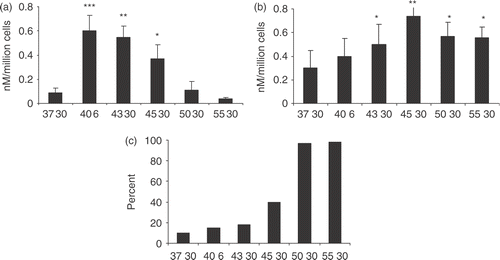
Figure 5. HSP70 release and trypan blue uptake in LNCaP cells exposed to temperatures from 37–55°C. LNCaP cells were heat treated as described in Figure 4 and supernatants were harvested immediately after the heat treatment (a) and 24 h after the heat treatment (b) and tested for the presence of HSP70 by ELISA. Trypan blue uptake, 5C, was analysed 24 h after the heat treatments. Statistical analyses compared 37 vs. 45 (a) and 37 vs. 40, 43, 50, 55 (b) (*p < 0.05); 37 vs. 43 (a) and 37 vs. 45 (b) (**p < 0.01); 37 vs. 40 (a) (***p < 0.001). Data represents the mean ± SD of three experiments done in triplicate.
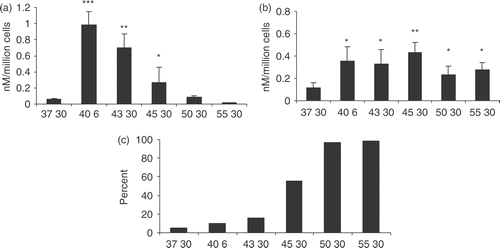
Discussion
Previous reports show that the release of members of the HSP70 family of proteins from cells involves physiological mechanisms such as co-secretion in exosomes of HSC70 with transferrin (contains a secretion leader) and the active HSP70 secretion by the non-canonical pathway employed by cytokines such as IL1b Citation[19], Citation[27]. These experiments indicate that at temperatures of 43°C and under, the latter pathway is employed for HSP70 secretion ( and ). The mechanisms of secretion of leaderless proteins such as HSP70 are complex and incompletely understood, although two main mechanisms have been suggested. The first (a) utilized by proteins such as FGF-1 and IL-1alpha, appears to involve translocation across the plasma membrane after partial denaturation to a ‘molten globule’ form Citation[28]. It has been conjectured that heat shock stimulates this pathway in part by permitting the proteins to partially unfold, a requirement for membrane translocation of many proteins Citation[29], Citation[30]. A similar mechanism is plausible for HSP70 secretion as these experiments suggest that proteasomal inhibition decreases HSP70 release by hyperthermia, a finding which suggests that enhanced accumulation of unfolded proteins may not be a trigger for HSP70 trigger (2">). However, it is also possible that proteasomal inhibitors, by increasing HSP70 substrates in the cell, may thus inhibit HSP70 release due to a decrease in free levels. More common however, (b) appears to be the pathway utilized by IL-1beta which involves entry of the secreted protein into endolysosomes and release from the cell by a vesiculation mechanism Citation[31]. Previous studies strongly support such a mechanism for HSP70 secretion under moderate heating conditions Citation[19]. Other secreted proteins that are largely of cytoplasmic/nuclear distribution in addition to HSP70, such as high mobility protein b1 (HMGB1) and engrailed-2, are released through this pathway under specialized conditions that promote secretio Citation[32], Citation[33]. Several other schemes for HSP70 release into the extra-cellular medium have been proposed and indeed may be operable under some circumstances. It has been proposed that HSP70 may be released from cells in lipid-bounded ‘endosomes’ Citation[31]. Such a scheme is not supported by the experiments in a previous study of prostate carcinoma cells in which one could only detect HSP70 in the extra-cellular medium in free solution Citation[19]. However, it is possible that HSP70 may be released from other cell types by such a mechanismCitation[5], Citation[34], Citation[35]. Secretion mechanisms may vary between cell types and, for instance, IL-1 beta secretion involves quite distinct mechanisms in different cell types Citation[26], Citation[35].
Previous studies have shown that anti-tumour immunity can be generated when intra-cellular HSP70 levels are increased by forced over expression and then cells are exposed to a treatment that induces necrosis, especially if the necrosis occurs gradually Citation[16], Citation[36]. It was examined here whether HSP70 is released under ablation heating conditions in which cell necrosis occurs. Slowly developing HSP70 release occurred in PC-3 and LNCaP cells at temperatures of 50°C and 55°C, at which the plasma membrane is compromised ( and ). However, this increase in HSP70 leakage from necrotic cells exposed to temperatures above 50°C occurred at the cost of inactivating the physiological secretion mechanism, as these extreme temperatures inhibited the rapid HSP70 secretion pathway ( and ). These experiments show, therefore, that HSP70 release from cells under cancer treatment involves at least two mechanisms including active secretion and passive release and these individual mechanisms may be more or less activated by a given treatment protocol. Hyperthermia has been shown to kill cells through a complex range of death pathways, which include programmed cell death mechanisms, mitotic catastrophe and necrosis Citation[25–39]. Programmed cell death through the apoptosis pathway does not seem to be a major pathway for thermal killing of prostate carcinoma cells, at least up to 45°C Citation[38] (E. Jones and S. K. Calderwood, unpublished). However, at 45°C and above, an increasing fraction of cells with compromised plasma membranes was observed, suggesting that necrosis increases at these temperatures ( and ). Previous studies suggest that necrotic death favours the generation of immunogenic forms of HSP70 Citation[16], Citation[36], Citation[40], Citation[41]. The current experiments suggest that enhanced HSP70 secretion may contribute to increase immunogenicity under conditions that favour necrosis.
These experiments, therefore, suggest that HSP70 in cells treated with hyperthermia acquires a novel, extra-cellular role. As HSP70 has immunological properties, this pathway may play a significant role in tumour immune response to hyperthermia.
Acknowledgements
We acknowledge the fine technical support of Rong Zhong and thank Jimmy Theriault, Kishiko Ogawa and Abdul Khaleque for helpful discussions. This work was supported by National Institutes of Health Grants CA047407, CA094397, CA094397-05S1 support for S.S.M. We thank Dr Mary Ann Stevenson and the Department of Radiation Oncology at BIDMC/Harvard Medical School for support.
References
- Lindquist S, Craig EA. The heat-shock proteins. Annu Rev Genet 1988; 22: 631–677
- Asea A, Kraeft SK, Kurt-Jones EA, Stevenson MA, Chen LB, Finberg RW, Koo GC, Calderwood SK. HSP70 stimulates cytokine production through a CD14-dependant pathway, demonstrating its dual role as a chaperone and cytokine. Nat Med 2000; 6: 435–442
- Basu S, Binder RJ, Suto R, Anderson KM, Srivastava PK. Necrotic but not apoptotic cell death releases heat shock proteins, which deliver a partial maturation signal to dendritic cells and activate the NF-kappa B pathway. Int Immunol 2000; 12: 1539–1546
- Hunter-Lavin C, Davies EL, Bacelar MM, Marshall MJ, Andrew SM, Williams JH. Hsp70 release from peripheral blood mononuclear cells. Biochem Biophys Res Commun 2004; 324: 511–517
- Lancaster GI, Febbraio MA. Exosome-dependent trafficking of HSP70: A novel secretory pathway for cellular stress proteins. J Biol Chem 2005; 280(24)23349–23355
- Broquet AH, Thomas G, Masliah J, Trugnan G, Bachelet M. Expression of the molecular chaperone Hsp70 in detergent-resistant microdomains correlates with its membrane delivery and release. J Biol Chem 2003; 278: 21601–21606
- Guzhova I, Kislyakova K, Moskaliova O, Fridlanskaya I, Tytell M, Cheetham M, Margulis B. In vitro studies show that Hsp70 can be released by glia and that exogenous Hsp70 can enhance neuronal stress tolerance. Brain Res 2001; 914: 66–73
- Febbraio MA, Ott P, Nielsen HB, Steensberg A, Keller C, Krustrup P, Secher NH, Pedersen BK. Exercise induces hepatosplanchnic release of heat shock protein 72 in humans. J Physiol 2002; 544: 957–962
- Fleshner M, Campisi J, Amiri L, Diamond DM. Cat exposure induces both intra- and extracellular Hsp72: The role of adrenal hormones. Psychoneuroendocrinology 2004; 29: 1142–1152
- Hightower LE, Guidon PT, Jr. Selective release from cultured mammalian cells of heat-shock (stress) proteins that resemble glia-axon transfer proteins. J Cell Physiol 1989; 138: 257–266
- Srivastava P. Interaction of heat shock proteins with peptides and antigen presenting cells: Chaperoning of the innate and adaptive immune responses. Annu Rev Immunol 2002; 20: 395–425
- Theriault JR, Mambula SS, Sawamura T, Stevenson MA, Calderwood SK. Extracellular HSP70 binding to surface receptors present on antigen presenting cells and endothelial/epithelial cells. FEBS Lett 2005; 579: 1951–1960
- Calderwood SK, Theriault JR, Gong J. Message in a bottle: Role of the 70-kDa heat shock protein family in anti-tumor immunity. Eur J Immunol 2005; 35: 2518–2527
- Calderwood SK, Theriault JR, Gong J. How is the immune response affected by hyperthermia and heat shock proteins?. Int J Hyperthermia 2005; 21: 713–716
- Chen X, Tao Q, Yu H, Zhang L, Cao X. Tumor cell membrane-bound heat shock protein 70 elicits antitumor immunity. Immunol Lett 2002; 84: 81–87
- Daniels GA, Sanchez-Perez L, Diaz RM, Kottke T, Thompson J, Lai M, Gough M, Karim M, Bushell A, Chong H, Melcher A, Harrington K, Vile RG. A simple method to cure established tumors by inflammatory killing of normal cells. Nat Biotechnol 2004; 22: 1125–1132
- Noessner E, Gastpar R, Milani V, Brandl A, Hutzler PJ, Kuppner MC, Roos M, Kremmer E, Asea A, Calderwood SK, Issels RD. Tumor-derived heat shock protein 70 peptide complexes are cross-presented by human dendritic cells. J Immunol 2002; 169: 5424–5432
- Baker-LePain JC, Reed RC, Nicchitta CV. ISO: A critical evaluation of the role of peptides in heat shock/chaperone protein-mediated tumor rejection. Curr Opin Immunol 2003; 15: 89–94
- Mambula SS, Calderwood SK. HSP70 is secreted from tumor cells by a non-classical pathway involving lysosomal endosomes. Journal of Immunology 2006, in press
- Mambula SS, Sau K, Henneke P, Golenbock DT, Levitz SM. Toll-like receptor (TLR) signaling in response to Aspergillus fumigatus. J Biol Chem 2002; 277: 39320–39326
- Tang D, Khaleque MA, Jones EL, Theriault JR, Li C, Wong WH, Stevenson MA, Calderwood SK. Expression of heat shock proteins and heat shock protein messenger ribonucleic acid in human prostate carcinoma in vitro and in tumors in vivo. Cell Stress Chaperones 2005; 10: 46–58
- Jackson A, Friedman S, Zhan X, Engleka KA, Forough R, Maciag T. Heat shock induces the release of fibroblast growth factor 1 from NIH 3T3 cells. Proc Natl Acad Sci USA 1992; 89: 10691–10695
- Burd R, Dziedzic TS, Xu Y, Caligiuri MA, Subjeck JR, Repasky EA. Tumor cell apoptosis, lymphocyte recruitment and tumor vascular changes are induced by low temperature, long duration (fever-like) whole body hyperthermia. J Cell Physiol 1998; 177: 137–147
- Ostberg JR, Patel R, Repasky EA. Regulation of immune activity by mild (fever-range) whole body hyperthermia: Effects on epidermal Langerhans cells. Cell Stress Chaperones 2000; 5: 458–461
- Hahn GM, Li GC. Thermotolerance and heat shock proteins in mammalian cells. Radiat Res 1982; 92: 452–457
- Marty V, Medina C, Combe C, Parnet P, Amedee T. ATP binding cassette transporter ABC1 is required for the release of interleukin-1beta by P2X7-stimulated and lipopolysaccharide-primed mouse Schwann cells. Glia 2005; 49: 511–519
- Asea AAaSKC. Regulation of signal transduction by intracellular and extracellular HSP70. Cambridge University Press, CambridgeUK 2005
- Prudovsky I, Mandinova A, Soldi R, Bagala C, Graziani I, Landriscina M, Tarantini F, Duarte M, Bellum S, Doherty H, Maciag T. The non-classical export routes: FGF1 and IL-1alpha point the way. J Cell Sci 2003; 116: 4871–4881
- Arai M, Kuwajima K. Role of the molten globule state in protein folding. Adv Protein Chem 2000; 53: 209–282
- Ptitsyn OB. Molten globule and protein folding. Adv Protein Chem 1995; 47: 83–229
- Tytell M. Release of heat shock proteins (Hsps) and the effects of extracellular Hsps on neural cells and tissues. Int J Hyperthermia 2005; 21: 445–455
- Gardella S, Andrei C, Ferrera D, Lotti LV, Torrisi MR, Bianchi ME, Rubartelli A. The nuclear protein HMGB1 is secreted by monocytes via a non-classical, vesicle-mediated secretory pathway. EMBO Rep 2002; 3: 995–1001
- Maizel A, Bensaude O, Prochiantz A, Joliot A. A short region of its homeodomain is necessary for engrailed nuclear export and secretion. Development 1999; 126: 3183–3190
- Gastpar R, Gehrmann M, Bausero MA, Asea A, Gross C, Schroeder JA, Multhoff G. Heat shock protein 70 surface-positive tumor exosomes stimulate migratory and cytolytic activity of natural killer cells. Cancer Res 2005; 65: 5238–5247
- Andrei C, Dazzi C, Lotti L, Torrisi MR, Chimini G, Rubartelli A. The secretory route of the leaderless protein interleukin 1beta involves exocytosis of endolysosome-related vesicles. Mol Biol Cell 1999; 10: 1463–1475
- Calderwood SK. Chaperones and slow death—a recipe for tumor immunotherapy. Trends Biotechnol 2005; 23: 57–59
- Andrei C, Margiocco P, Poggi A, Lotti LV, Torrisi MR, Rubartelli A. Phospholipases C and A2 control lysosome-mediated IL-1 beta secretion: Implications for inflammatory processes. Proc Natl Acad Sci USA 2004; 101: 9745–9750
- Jones EL, Zhao MJ, Stevenson MA, Calderwood SK. The 70 kilodalton heat shock protein is an inhibitor of apoptosis in prostate cancer. Int J Hyperthermia 2004; 20: 835–849
- Gabai VL, Meriin AB, Yaglom JA, Volloch VZ, Sherman MY. Role of Hsp70 in regulation of stress-kinase JNK: Implications in apoptosis and aging. FEBS Lett 1998; 438: 1–4
- Melcher A, Todryk S, Hardwick N, Ford M, Jacobson M, Vile RG. Tumor immunogenicity is determined by the mechanism of cell death via induction of heat shock protein expression. Nat Med 1998; 4: 581–587
- Srivastava PK. Hypothesis: Controlled necrosis as a tool for immunotherapy of human cancer. Cancer Immun 2003; 3: 4