Abstract
Purpose: The aim of this study was to verify hyperthermia treatment planning calculations by means of measurements performed during hyperthermia treatments. The calculated specific absorption rate (SARcalc) was compared with clinically measured SAR values, during 11 treatments in seven cervical carcinoma patients.
Methods: Hyperthermia treatments were performed using the 70 MHz AMC-4 waveguide system. Temperatures were measured using multisensor thermocouple probes. One invasive thermometry catheter in the cervical tumour and two non-invasive catheters in the vagina were used. For optimal tissue contact and fixation of the catheters, a gynaecological tampon was inserted, moisturized with distilled water (4 treatments), or saline (6 treatments) for better thermal contact. During one treatment no tampon was used. At the start of treatment the temperature rise (ΔTmeas) after a short power pulse was measured, which is proportional to SARmeas. The SARcalc along the catheter tracks was extracted from the calculated SAR distribution and compared with the ΔTmeas-profiles.
Results: The correlation between ΔTmeas and SARcalc was on average R = 0.56 ± 0.28, but appeared highly dependent on the wetness of the tampon (preferably with saline) and the tissue contact of the catheters. Correlations were strong (R ∼ 0.85–0.93) when thermal contact was good, but much weaker (R ∼ 0.14–0.48) for cases with poor thermal contact.
Conclusion: Good correlations between measurements and calculations were found when tissue contact of the catheters was good. The main difficulties for accurate verification were of clinical nature, arising from improper use of the gynaecological tampon. Poor thermal contact between thermocouples and tissue caused measurement artefacts that were difficult to correlate with calculations.
Introduction
Hyperthermia (HT) is the application of elevated temperatures to tumours (41–45°C) and is an effective anti-cancer treatment in combination with radiotherapy and chemotherapy. Hyperthermia treatments aim at uniform tumour temperatures of usually 43°C for 1 h Citation[1]. This thermal dose can be difficult to achieve, owing to the occurrence of treatment-limiting hot spots in normal tissue and systemic stress at high power Citation[2–4]. The occurrence of hot spots is determined by the patients’ local anatomy. The occurrence of systemic stress is mainly dependent on the amount of power applied by the heating device. Such limitations are difficult to avoid intuitively on account of the large number of degrees of freedom of the heating device, i.e. the powers and phases of the antennas Citation[5–7]. Therefore, hyperthermia treatment planning (HTP) is a useful tool for improvement of clinical hyperthermia treatments.
For clinical application of HTP, it is important to obtain a reliable and accurate model of the patients’ anatomy in terms of dielectric and thermal properties of the different tissue types and/or organs Citation[8], Citation[9]. For this purpose a CT or MRI scan of the patient, preferably in treatment position, is segmented into tissue types and/or organs. Unfortunately, dielectric properties of different tissue types and organs are usually rather difficult to measure accurately Citation[10–13], and consequently a large spread in values is reported in the literature Citation[9], Citation[14–16]. To obtain insight in the reliability of the current HTP systems, clinical verification studies are essential.
Verification of various HTP systems has been performed using analytical solutions, phantom measurements Citation[17–19], and isolated (perfused) tissues or organs Citation[20], generally showing a satisfactory agreement between calculations and measurements. Recently, Van den Berg et al. described a sophisticated method using MRI for validation of electromagnetic simulations in phantoms and human cadavers Citation[19], Citation[21]. There have been only few reports in the literature on verification of HTP in patients Citation[22], Citation[23]. Sreenivasa et al. reported a good correlation between model calculations and clinical data regarding hot spots and prediction of heating capability in patients, using the HyperPlan HTP system for the BSD-2000 HT system Citation[23]. Gellermann et al. showed that measured E-field or SAR profiles correlated well with calculated values using a contour-based FDTD method, but the deviations were larger for the voxel-based FDTD method Citation[22].
The aim of this study was to determine the correlation between HTP calculations and measurements during hyperthermia treatments. It was attempted to verify the dielectric input of the HTP system in terms of SAR distributions, as a prerequisite for verification of the subsequent thermal computations in the future. SAR calculations from Finite Difference Time Domain (FDTD)-based HTP were compared with measured SAR profiles along minimally invasive thermometry tracks during HT treatments of cervical carcinoma patients. The results of these verifications as well as the encountered difficulties will be discussed.
Methods
Hyperthermia treatments and measurements
Within an ongoing phase III study, patients with locally advanced cervical carcinoma are treated with chemoradiotherapy +/− weekly hyperthermia (HT) for 5 weeks. The HT treatment is given using the 70 MHz AMC-4 phased array system Citation[24]. During 11 treatment sessions in seven patients extensive (minimally) invasive thermometry was applied as well as pre-treatment CT scans for verification of HTP. (left panel) shows a cervical carcinoma patient during an HT treatment. Catheters for insertion of thermometry probes were placed in the target region. Self-anchoring catheters Citation[25] (Ella-CS, Czech Republic) were placed in the left and right fornix of the vagina and (minimally) invasively in the cervical tumour. The intratumoral depth was aimed to be ∼4–5 cm. (right panel) shows a schematic representation of the location of the vaginal and intratumoral catheters. Regular HT thermometry catheters (Ella-CS, Czech Republic) were placed in the bladder and the rectum, as well as an intravaginal E-field probe near the tumour.
Figure 1. Left: Locoregional hyperthermia treatment of a cervical carcinoma patient with the 70 MHz AMC-4 phased array system; the location of the four antennas is indicated. Right: Schematic representation of the intratumoral and left and right vaginal thermocouple probes in the target region; the anatomical drawing was reprinted from The Northern Cancer Network website (www.cancernorth.nhs.uk).
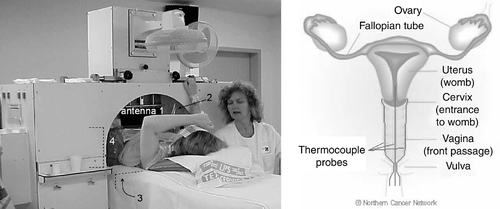
A gynaecological tampon was inserted in the vagina for optimal tissue contact and fixation of the catheters. For better thermal contact between catheters and tampon, the tampon was moisturized with sterile distilled water (4 treatments), or with sterile saline (0.3% NaCl solution, 6 treatments). A saline solution was used that mimics the average dielectric and thermal properties of human tissue at 70 MHz Citation[26]. During one treatment no tampon was used. To prevent catheter displacement further during transport of the patient, the catheters were taped to the inside of the patient's thighs. The patient was transported to the CT scanner and the HT treatment room using a mobilizer (Medical Laboratory Automation Inc.).
A CT scan spanning 60 cm body length with a slice-thickness of 5 mm was made of the patient in treatment position, i.e. in supine position on a waterbolus and mattresses similar to those used during the HT treatment, with the catheters in situ. To visualize and distinguish the thermometry catheters, X-ray cable dummies (Nucletron BV, The Netherlands) were inserted prior to the scan.
The patient was positioned on the HT treatment table such that the target region was located in front of the aperture of the bottom antenna of the AMC-4 system. The position of the catheters was verified by dorsoventral and lateral X-ray images, with the X-ray cable dummies in the catheters. The cable dummies were removed and multisensor (14 points) copper-constantan thermocouple probes (TCs) with a spacing of 5 or 10 mm were inserted in the catheters. To minimize disturbances of the TCs by the EM-field, a minimum of 12 ferrite beads per thermocouple probe was used. The constantan (Ø 70 µm) wire and the 14 copper (Ø 40 µm) wires of the thermocouple probes are sufficiently thin to ignore any perturbation of the EM-field. For better thermal contact between TCs and surroundings, the catheters were filled with distilled water. The patient was positioned between the four waveguides plus waterboli, and the HT treatment was started. Clinical experience has led to standard power settings of the hyperthermia system for different tumour sites. For treatment of cervical tumours the standard power ratios for the top, bottom, left and right antennas are 3:3:2:2. Phase settings are adjusted such that a maximal E-field is measured by the E-field probe at the target location, which corresponds to a local SAR maximum in the target region. The top antenna serves as reference (phase 0°).
At the start of every treatment, a measurement of the temperature rise (ΔT) after a 30-s power pulse of 800 W was performed, measured standardly after a 5-s power-off period of the AMC-4 system. To minimize the influence of self-heating of the thermocouple probes, the 30-s power pulse was preceded by a 5-s pre-pulse of 800 W, followed by a 5-s power-off period. The ΔT is the difference between the temperatures measured after the two power-off periods Citation[7]. This method was adapted from the method described by de Leeuw et al. Citation[27], which made use of a 25- or 50-s pre-pulse, in order to minimize any possible contribution of thermal conduction. Self-heating of thermocouples is assumed to be very fast, thus its contribution will be equal for a short (5 s) pre-pulse and for a longer (e.g. 25, 30 or 50 s) pre-pulse. Conduction can be neglected during a short pre-pulse, but may have some effect during a longer pre-pulse. To estimate what the effect of a longer pre-pulse would have been, the relation between the measured ΔT and the temperature rise during the first or second duty cycle power-on pulse (DC1, DC2) was investigated. The clinically applied duty cycle consisted of 25 s power-on, followed by 5 s power-off, after which the temperatures are measured (see below). The correlation between ΔT and DC1 or DC2 was on average 0.90 ± 0.02 and 0.87 ± 0.04, respectively. The ratio between DC1 or DC2 and ΔT was on average 0.85 ± 0.10 and 0.82 ± 0.10, respectively, which is almost equal to the ratio of both power-on periods (25 s/30 s = 0.83). It was concluded that a 5-s pre-pulse was appropriate for ΔT measurements. Since ΔT is proportional to the specific absorption rate (SAR (W kg−1)) Citation[27], these measurements provide measured SAR profiles along the thermocouple tracks:with c the specific heat capacity of the tissue (J kg−1°C−1). These measurements will be indicated by ΔTmeas.
During the rest of the treatment, temperatures were measured every 30 s, standardly after a 5-s power-off period of the AMC-4 system. According to protocol, the steady state period of 60 min started when at least one measurement point in the target region reached 41°C, or alternatively after an induction period of 30 min. During the HT treatment the cooling water in the waterbolus was kept at a temperature of 12°C.
Hyperthermia treatment planning
For HTP the aforementioned CT scan was used, made with the patient in treatment position and with the catheters in situ. Tumour, tampon (if used) and catheters were outlined manually. The catheters were removed from the data set prior to the calculations. The tampon was assigned the properties of its condition (dry/air, water or saline) during the concerned treatment. It was taken into account that the volume of a saturated tampon consists of ∼85% water or saline and ∼15% tampon, as determined empirically. The rest of the data set was automatically segmented into muscle, fat, bone and inner air (e.g. in bowels), using thresholding based on a histogram of Hounsfield Units of the CT data set Citation[28].
The data set was downscaled to 2 × 2 × 5 mm3 (high resolution, HR), and to 1 × 1 × 1 cm3 (low resolution, LR), using the ‘winner takes all’ principl Citation[29], Citation[30], which means that an LR voxel is assigned the properties of the most frequently occurring tissue type of the corresponding HR voxels. The E-field (E (V m−1)) distributions were calculated for each antenna of the AMC-4 system separately with unit amplitude and zero phase, using our FDTD-based HTP system Citation[6], Citation[31]. Owing to computer limitations, the resolution for direct E-field calculations was limited to 1 × 1 × 1 cm3 (LR), which is too low for reliable treatment planniCitation[6], Citation[32], Citation[33]. Therefore, a quasistatic zooming technique was applied to obtain the HR power density and SAR (W kg−1) distributions Citation[6], Citation[32–34], according to the clinically applied power/phase-settings of the AMC-4 system. The SAR was calculated according towith σ being the conductivity (S m−1), ρ the density (kg m−3), and V the HR potential distribution (V) determined using the quasistatic approximation of Maxwell's equations. The potential is estimated by integrating the LR E-field distribution over a small zoom volume, for which quasistatic computation is allowed. Several zoom volumes are necessary to cover the volume of interest (patient volume) Citation[32], Citation[33]. The calculated SAR will be indicated by SARcalc. To include the actual efficiency of the AMC-4 system, the calculated SAR distributions were scaled with a factor that was determined from ΔT measurements in homogeneous phantoms with well-known properties Citation[35], such that Equation (1) holds for the concerned specific heat capacity (data not shown). The dielectric and thermal properties used in the calculations were obtained from the literatu Citation[15], Citation[16], and are summarized in .
Table I. Dielectric and thermal properties at 70 MHz for the different tissue types used in the calculations; conductivity (σ (S m−1)), relative permittivity (εr (−)), density (ρ (kg m−3)), and specific heat capacity (c (J kg−1°C−1)).
Verification of hyperthermia treatment planning
For the verification of the calculated SAR distributions the intratumoral, left and right vaginal catheter tracks were reconstructed using the CT data set and the X-ray images. Thanks to the X-ray cable dummies the catheter tracks could be reconstructed accurately and well distinguished from each other. Furthermore, it was confirmed that the catheters were not displaced during transport from the CT table to the HT treatment table, by matching the CT scan and the X-ray images. The SAR along the intratumoral and vaginal catheter tracks was extracted from the calculated SAR distribution (SARcalc) and compared with the clinically measured ΔT profiles (ΔTmeas) along these catheter tracks.
Statistics
Data are presented as mean ± SD. The correspondence between ΔTmeas and SARcalc was expressed in terms of the correlation coefficient R, as determined using SPSS 12. A value of p < 0.05 was considered statistically significant.
Results
Extensive (minimally) invasive thermometry and pre-treatment CT scans were applied for 11 treatment sessions in seven patients, for verification of HTP. For practical reasons, the intratumoral depth of the (minimally) invasive catheter () was generally 1–2 cm, although it was originally aimed to be deeper (∼4–5 cm). The depth was limited to minimize pain complaints of the patient during implantation of the catheters, and to prevent possible spill of tumour cells beyond the cervical tumour into healthy tissue. Characteristics of the 11 treatment sessions are summarized in . During the first two treatments (patients 1, 2), the quality of the pre-treatment CT scan was not sufficient for treatment planning purposes (see also Discussion). During the subsequent four treatments (patients 3, 4), the gynaecological tampon, used to pursue good tissue contact of the catheters, was moisturized with distilled water. Unfortunately, the tissue contact of the catheters was not always optimal. During the following four treatments (patients 5, 6), the tampon was moisturized with saline. However, during two treatments the tampon appeared too dry. In the last treatment (patient 7) no tampon was used under the assumption that the vagina would re-close after insertion of the catheters. However, the tissue contact of the catheters was still suboptimal. For six treatments (4 patients) the correlation between measurements and calculations could be determined.
Table II. Overview of all 11 treatment sessions in seven cervical carcinoma patients, in which verification of HTP has been applied. The summarized properties concern the session number, the conditions of the gynaecological tampon, the case as presented below (n.p., not presented), whether a correlation between ΔTmeas and SARcalc was calculated (N, not calculated; Y, calculated), and comments on the encountered difficulties in the verification process.
From the aforementioned 11 treatment sessions, the results of four specific cases will be presented: a treatment with a tampon moisturized with distilled water (case 1); a tampon moisturized with saline with good thermal contact of the thermometry catheters (case 2); a tampon badly moisturized with saline and therefore remaining too dry (case 3); and a treatment using no tampon (case 4). The characteristics of these four cases are summarized in , concerning the conditions of the gynaecological tampon and the tissue contact of the catheters, power dissipation in the tampon and reliability of the SAR calculations, thermal contact of the catheters and reliability of the ΔT measurements, indicating whether all conditions for accurate verification had been fulfilled. Each case will be discussed in detail below.
Table III. Overview of the four presented cases (see ). The summarized properties concern the conditions of the gynaecological tampon, the tissue contact of the catheters (✗ poor contact, ✓ good contact), the presence of power dissipation in the tampon (✗ no dissipation, ✓tissue equivalent dissipation) and its consequence for reliable SAR calculations (SARcalc, ✗ unreliable, ✓ reliable), the quality of the thermal contact of the catheters with tissue (✗ poor thermal contact, ✓ good thermal contact) and its consequence for reliable ΔT measurements (ΔTmeas, ✗ unreliable, ✓ reliable). The last column provides an indication whether all conditions for accurate verification have been fulfilled (✗ not all conditions fulfilled, ✓ conditions fulfilled).
Coronal and sagittal cross sections of the CT scans of the patients from the four cases are shown in . The CT scans were recorded with the patients in treatment position, i.e. in supine position, on a waterbolus and mattresses, with the catheters in situ. Only by making these CT scans did it become clear that in some cases the gynaecological tampon was too dry and some air was present in the (top of the) vagina, and that in some cases the tissue contact of the catheters was suboptimal.
Figure 2. Coronal (panels 1a–4a) and sagittal (panels 1b–4b) cross sections of the CT scans of the patients from cases 1–4. The CT scans were recorded with the patients in treatment position, i.e. supine position, on a waterbolus (w) and mattresses, with the catheters (c) in situ. In some cases (e.g. 3) the gynaecological tampon was too dry, thus some air (a) was present in the (top of the) vagina.
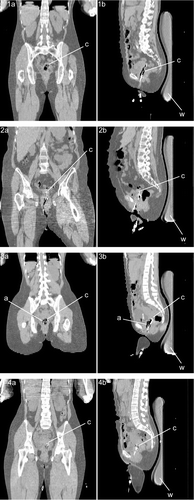
Dielectric and thermal characteristics
In case 1, a tampon moisturized with distilled water was used. This should result in good thermal contact of the thermometry catheters with the surroundings, tampon and tissue, providing reliable ΔT measurements. Unfortunately, the tissue contact of the catheters was suboptimal. Judging by the CT scan, only the deepest ∼2.5–3 cm of the vaginal catheter tracks was in good contact with the tissue, the remaining ∼3.5–4 cm of the thermocouple tracks being surrounded by the wet tampon. Since the tampon was assigned the properties of distilled water in this case, its modelled conductivity was very low (∼5.5 · 10−6 S m−1), which resulted in very low calculated SAR values in the vicinity of the caudal part of the vaginal thermocouple tracks (∼3.5 · 10−4 W kg−1, ). However, the measurements appeared uninfluenced by the distilled water surrounding the caudal part of the thermocouple probes, resulting in ΔT values ∼0.4°C over the entire thermocouple length (see Discussion). This hampered accurate verification of the calculated SAR distribution along the entire thermocouple tracks for this case.
Figure 3. Sagittal cross sections of the segmented anatomy (red: muscle; yellow: fat; orange: tumour; green: bone; blue: (inner) air, water or saline) for the four cases (panels 1a–4a). The calculated SAR distributions (SARcalc) are shown in panels 1b–4b, ranging from 0 W kg−1 (blue) to 50 W kg−1 (red), from 0 to 50 W kg−1, from 0 to 75 W kg−1, and from 0 to 60 W kg−1, respectively. The corresponding calculated temperature rise distributions after 30 s are shown in panels 1c–4c, ranging from 0°C (blue) to 0.75°C (red) for all panels. Note the superficial low temperature rises (∼0°C) due to bolus cooling.
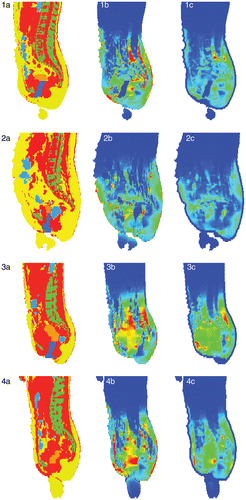
Figure 4. Measured ΔT profiles (ΔTmeas) along the intratumoral, left and right vaginal thermocouple tracks for the four cases (panels 1a–4a). The corresponding calculated SAR profiles (SARcalc) along the intratumoral, left and right vaginal thermocouple tracks are shown in panels 1b–4b.
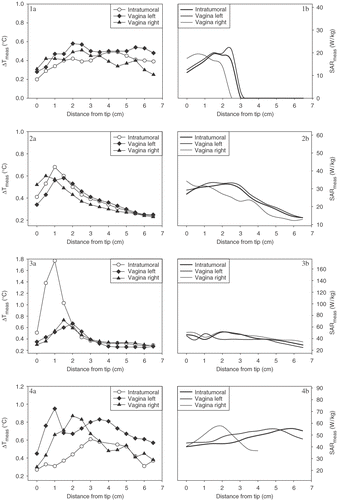
In case 2, a tampon moisturized with saline was used. In this case the tissue contact of the catheters was generally good, implying good thermal contact of the thermometry catheters. Therefore, both the SAR calculations and the ΔT measurements were reliable, providing the conditions required for accurate verification.
In case 3, the tampon was moisturized with saline, but appeared not fully saturated. This could only be determined by the CT scan, which showed that the tampon was (too) dry and thus some air was present in the top of the vagina. This resulted in unreliable SAR calculations in that region, since the entire tampon was assigned the properties of saline in this case. Furthermore, the tissue contact of the catheters was not good, resulting in poor thermal contact of the thermometry with the surrounding tissue, and thus unreliable ΔT measurements. These issues hampered accurate verification for this case.
In case 4, no tampon was used. It was assumed that the vaginal cavity re-closes after insertion of the thermometry catheters and the E-field probe, ensuring good tissue contact of the catheters even without using a gynaecological tampon. A further advantage of this option is that there is no additional foreign object in the region of interest, i.e. the vaginal cavity. This facilitated reliable SAR calculations in the close vicinity of the thermometry catheters. Nevertheless, the tissue contact of the catheters was still not optimal, resulting in suboptimal thermal contact with the tissue and thus resulting in unreliable ΔT measurements, which hampered accurate verification for this case.
Correlation between calculations and measurements
Sagittal cross sections of the SAR distributions for the four cases, calculated for the clinically applied power/phase-settings of the AMC-4 system, are shown in panels 1b–4b of , together with the corresponding segmented anatomy in panels 1a–4a. The depicted SAR values were typically in the range 0–50 W kg−1, but were strongly determined by the patient-specific local anatomy, and varied for individual patients. All SAR distributions were scaled to a total absorbed power in the patient of 800 W corrected for the actual efficiency of the AMC-4 system, which consequently resulted in slightly different SAR levels (W kg−1) for patients of different body size and weight. Furthermore, several SAR hot spots can be observed, also at superficial locations in the patient. The corresponding calculated ΔT distributions after a 30-s power pulse of 800 W are shown in panels 1c–4c of . The depicted temperature rises were typically in the range 0–0.75°C, and showed less anatomy-determined heterogeneity than the calculated SAR distributions. Fewer hot spots are observed, primarily owing to the influence of bolus cooling on superficial high SAR values.
The measured ΔT profiles (ΔTmeas) along the intratumoral, left and right vaginal thermocouple tracks for the four cases are shown in the left panels of . For some cases, where the thermal contact between thermometry and tissue was not good, extremely high ΔTmeas values of ∼1–1.8°C were observed (e.g. case 3). These high ΔT values are artefacts (see Discussion) and therefore difficult to correlate with the calculations. The corresponding calculated SAR profiles (SARcalc) along the intratumoral, left and right vaginal thermocouple tracks were extracted from the calculated SAR distributions (), and are shown in the right panels of .
Averaged over all six treatments in four patients, for which a correlation could be determined, the correlation between ΔTmeas and SARcalc was R = 0.56 ± 0.28 (mean ± SD), which is rather weak for reliable verification. For the four presented cases, the correlations (R) between ΔTmeas and SARcalc for the intratumoral, left and right vaginal thermocouple tracks are summarized in . Only for case 2 were the correlations strong (0.90 ± 0.04) and highly significant (p < 0.01) for all three thermocouple tracks. For the other three cases the correlations were generally weak and only marginally significant (p < 0.05).
Table IV. Correlations (R) between ΔTmeas and SARcalc for the intratumoral, left and right vaginal thermocouple probes, and the mean ± SD of the three, for the four cases. *p < 0.05, **p < 0.01. For case 1 the correlations were calculated using all thermosensors over the entire length of the thermocouple tracks, or using the thermosensors of the deepest ∼2.5–3 cm only, for which the tissue contact was good (between parentheses).
Discussion
Hyperthermia treatment planning (HTP) is a useful tool for improvement of clinical hyperthermia treatments. Accurate verification of HTP calculations, preferably by using in vivo measurements, is a prerequisite for clinical application of HTP. In this study, the correlation between SAR calculations from HTP and ΔT measurements from clinical hyperthermia was determined for 11 treatment sessions of seven cervical carcinoma patients.
Difficulties during the verification procedure
Several difficulties in different steps of the procedure were encountered, which hampered the verification for five treatments (the first 4 patients) out of the total of 11 treatments (7 patients, ). In most cases the intratumoral depth of the (minimally) invasive catheter was smaller than intended, resulting in only a few measurement points in tumour tissue. More invasive measurement points would have strengthened the clinical verification of HTP in the region of primary interest, i.e. the tumour.
During the first two treatments (2 patients) the quality of the pre-treatment CT scan was not sufficient for treatment planning purposes. Suboptimal patient positioning in combination with a body size which, for some patients, was too large for the limited field-of-view of the CT scanner (Ø 48 cm) resulted in scanning artefacts, which impaired the automatic segmentation of the different tissue types.
During the following four treatments (2 patients) the gynaecological tampon, which was inserted in the vagina to persue good tissue contact of the catheters, was moisturized with distilled water. In principle this would be adequate, as long as the thermometry catheters are indeed in optimal contact with the tissue. In that case the thermocouples would accurately register the tissue temperatures, and the properties of the tampon, either dry or moisturized, would not significantly affect these measurements. Unfortunately, the tissue contact of the catheters was not always optimal, and some catheters were surrounded by wet tampon along a part of their length. The thermal contact between thermocouples, wet tampon and tissue is still good for such a situation, but the dielectric properties of the surroundings of the thermocouples are no longer resemblant of the tissue properties. Calculations of the SAR in a tampon moisturized with distilled water results in SARcalc ≈ 0 W kg−1, owing to the extremely low conductivity of water (). Obviously, this is not resemblant of tissue SAR. In case 1, a sharp transition was visible in the SARcalc profiles between the thermosensors that were in contact with the tissue and the sensors that were surrounded by the wet tampon (, panel 1b). By contrast, the ΔTmeas profiles showed no such transition, and more or less normal values for all thermosensors along the three catheter tracks (, panel 1a). In the CT scan of this patient it appeared as if the tampon were too dry, and some air remained in the vagina (, panels 1a, 1b). However, based on the measurements, it was hypothesized that the distilled water in the (badly) moisturized tampon may have mixed with body fluids present in the vagina, during the period between the CT scan and the start of the hyperthermia treatment. Since the conductivity of (distilled) water is extremely low, small changes in its composition can result in relatively large changes in its dielectric properties (e.g. a mixture of 90% distilled water (σ = 5.5 · 10−6 S m−1) and 10% physiological body fluid (∼0.9% NaCl) has a significantly increased conductivity of σ ≈ 0.17 S m−1 Citation[36]), which will be reflected in the measured SAR levels. This hypothesis is, however, very difficult to confirm from the CT scan or by other measurements. It is, therefore, virtually impossible to account for possible changes in the composition of the fluid that moisturizes the tampon in the HTP calculations. This discrepancy hampered accurate verification for the treatments with a tampon moisturized with distilled water.
During the subsequent four treatments (2 patients) the tampon was moisturized with saline to circumvent the aforementioned difficulties. A saline solution was used that mimics the average dielectric and thermal properties of human tissue at 70 MHz (σ = 0.55 S m−1, εr = 75, ρ = 1000 kg m−3, c = 4180 J kg−1°C−1) Citation[26]. To estimate the dielectric properties of the tampon moisturized with saline, it was determined empirically that the volume of a saturated tampon consists of 85% saline and 15% tampon, resulting in σ = 0.47 S m−1, εr = 64, ρ = 850 kg m−3 and c = 3550 J kg−1°C−1. This can be accurately modelled in the HTP system and provides good thermal contact between thermometry, tampon and tissue, and should therefore fulfil all conditions for accurate verification.
Unfortunately, during at last two treatments (1 patient) the tampon was not fully saturated with saline and therefore remained too dry at some locations inside the vagina. Moreover, during these treatments the tampon was not inserted such that all catheters were pressed into contact with the tissue over the entire length. Hence, in these cases some air was present in the (top of the) vagina and the tissue contact of the catheters was not optimal, resulting in poor thermal contact of the catheters with the surrounding tissue. This resulted in extremely high ΔTmeas (∼1–1.8°C) in these regions, which appeared erroneous and not resemblant of tissue ΔT Citation[37]. In the HTP calculations for the four treatments with a tampon moisturized with saline, the entire tampon was modelled as if it were saturated with saline. This resulted in more or less normal SARcalc values in the entire vaginal region, which are likely to be resemblant of tissue SAR, without the aforementioned artefacts. Again, this discrepancy hampered accurate verification for the treatments with a badly moisturized tampon.
In the last treatment no gynaecological tampon was used, under the assumption that the vagina would re-close after insertion of the thermometry catheters, ensuring good tissue contact of the catheters even without using a gynaecological tampon. This option did provide reliable SAR calculations in the close vicinity of the thermometry catheters. Nevertheless, the tissue contact of the catheters was still not optimal, resulting in suboptimal thermal contact with the tissue and thus resulting in sub-reliable ΔT measurements. Again, this hampered accurate verification for this case.
Correlation between ΔTmeas and SARcalc
The correlation between ΔTmeas and SARcalc was largely dependent on the aforementioned conditions and circumstances. Only when all conditions for accurate verification were fulfilled, the correlation was good for all three vaginal thermocouple tracks. This was the case for only two treatments (). For these two treatments the correlations were 0.70 ± 0.29 and 0.90 ± 0.04 respectively, averaged over the intratumoral and left and right vaginal thermocouple tracks. For the rest of the treatments the correlations were much weaker (0.37–0.57). Several practical difficulties, as described above, hampered accurate verification for these treatments, resulting in suboptimal correlations between ΔTmeas and SARcalc. From this perspective, optimal tissue contact of the thermometry catheters proved to be the most important prerequisite for accurate verification.
The measured ΔT is dependent on the thermal contact between thermometry and tissue. In this study, thermocouple thermometry was used, which has been proven to provide reliable temperature information when good thermal contact with the tissue is ensured Citation[27], Citation[38–40]. For some cases the thermal contact between thermocouples and tissue was suboptimal, causing artefacts in the ΔT measurements, resulting in very high ΔTmeas due to self-heating of the thermocouple probes Citation[37].
The calculated local SAR depends on the calculated local E-field distribution, the conductivity and density of the tissue (Equation (2)). The E-field distribution is dependent on the source definition of the 70 MHz waveguides of the AMC-4 hyperthermia system in the HTP system and on the dielectric properties of the heterogeneous model of the patient's anatomy. The implementation of the AMC-4 system and the calculation method of E-field distributions within the present HTP system have been accurately verified in homogeneous and inhomogeneous phantom models Citation[41], Citation[42]. Segmentation of the patient's anatomy and assignment of dielectric properties was based on the Hounsfield Units of the CT scan made for the planning. However, CT grey values do not necessarily correspond to a certain penetration depth for E-fields, inasmuch as they are related to radiodensity. Dielectric properties of the tissue types used in the calculations were obtained from literature Citation[15], Citation[16], but unfortunately there is a large spread in the reported values, which may be of influence on the calculations.
In the anatomical data set of the patient the tumour was outlined manually, and the rest of the data set was automatically segmented into muscle, fat, bone and inner air, using Hounsfield Unit based thresholding. As a result of this, the cervix uteri, vagina and part of its surroundings were assigned the properties of muscle tissue while others parts of the surroundings were assigned the properties of fat or bone. The cervical tumour was assigned the properties of tumour tissue as obtained from literature. The question remains, however, whether the properties of muscle, fat or bone were sufficiently accurate for the quantitative verification of SAR calculations using ΔT measurements in cervical and vaginal tissue, and whether literature values of the properties of tumour tissue in general were representative for cervical tumours.
Implications for steady state temperature measurements
The question remains to what extent uncertainties or artefacts in ΔT measurements are reflected in steady state temperature (SST) measurements. Suboptimal thermal contact between thermocouples and tissue results in artefacts in ΔT measurements Citation[37], as observed, for example, in case 3 (, panel 3a). The observed patterns in the ΔT profiles along the thermocouple tracks were generally well reflected in the SST profiles (data not shown), only distinct phenomena in ΔT were less pronounced in SST. This implies that artefacts in the ΔT measurements are also partly present in the SST measurements, thus possibly influencing the reported temperatures of the concerned treatment. However, the influence of possible artefacts in the measured SST largely disappears when expressing the achieved (tumour/target) temperatures in terms of T90, the temperature at least achieved in 90% of the target volume, which is rather insensitive to such extremes.
Recommendations and future research
Based on the encountered difficulties in the verification process, it is recommended for optimal intravaginal thermometry within the current setting (1) to use catheters that are sufficiently flexible to be pressed into contact with the tissue, even in an irregularly shaped cavity, (2) to insert the thermometry catheters manually followed by manual placement of the tampon, preferably without using a speculum, thereby pursuing optimal tissue contact of the catheters, and (3) to use a tampon moisturized with saline, to prevent unreliable measurements in case of suboptimal tissue contact of the catheters.
Several methodological adaptations are possible to improve the quality of the thermal contact between thermometry and tissue, thereby improving the quality of the intracavitary temperature rise measurements, so that these measurements can be correlated more reliably with calculations. For intraluminal temperature measurements during hyperthermia treatment of patients with oesophageal carcinoma, a balloon catheter is applied Citation[40]. Thermocouple probes are mounted on the outside of the balloon, which is inflated in the oesophagus, thereby pressing the thermocouples into contact with the tissue, resulting in optimal thermal contact. The question remains whether this may be an effective solution for intracavitary temperature (rise) measurements in case of cervical carcinoma, based on the irregular shape of the vaginal cavity, notably the fornices, in comparison to the oesophageal lumen. Another possibility is the use of additional subcutaneous thermometry probes, placed at relevant locations that can be determined using HTP and pre-treatment CT scans. For such thermometry probes the thermal contact with tissue will obviously be optimal, rendering the temperature (rise) measurements reliable for accurate verification of HTP. A new verification study using additional subcutaneous thermometry is planned at the Academic Medical Center.
Conclusion
A good correlation between measurements and calculations was found when tissue contact of the catheters was good. The main difficulties for accurate verification were of a clinical nature: difficulties arising from improper use of the gynaecological tampon and the limited number of measurements in tissue. Although it was originally aimed to be deeper, the intratumoral depth of the (minimally) invasive catheter was small. The specific location of this self-anchoring intratumoral catheter in the top of the vagina (see ) in combination with the (concave) shape of the local anatomy, notably the vaginal fornices, makes it difficult to ensure optimal tissue contact over the entire catheter length by pressing it into contact with tissue using a gynaecological tampon. Remaining air in the vagina and suboptimal tissue contact of the catheters resulted in poor thermal contact between thermocouples and tissue, causing measurement artefacts that were difficult to correlate with calculations.
Acknowledgements
This study is supported by the Dutch Cancer Society (grants 2002-2622, 2001-2462).
References
- Sapareto SA, Dewey WC. Thermal dose determination in cancer therapy. Int J Radiat Oncol Biol Phys 1984; 10: 787–800
- Oleson JR, Samulski TV, Leopold KA, Clegg ST, Dewhirst MW, Dodge RK, George SL. Sensitivity of hyperthermia trial outcomes to temperature and time: Implications for thermal goals of treatment. Int J Radiat Oncol Biol Phys 1993; 25: 289–297
- Wust P, Stahl H, Loffel J, Seebass M, Riess H, Felix R. Clinical, physiological and anatomical determinants for radiofrequency hyperthermia. Int J Hyperthermia 1995; 11: 151–167
- Hand JW, Machin D, Vernon CC, Whaley JB. Analysis of thermal parameters obtained during phase III trials of hyperthermia as an adjunct to radiotherapy in the treatment of breast carcinoma. Int J Hyperthermia 1997; 13: 343–364
- Wiersma J, van Maarseveen RA, van Dijk JD. A flexible optimization tool for hyperthermia treatments with RF phased array systems. Int J Hyperthermia 2002; 18: 73–85
- Kok HP, van Haaren PM, Van de Kamer JB, Wiersma J, van Dijk JD, Crezee J. High-resolution temperature-based optimization for hyperthermia treatment planning. Phys Med Biol 2005; 50: 3127–3141
- Kok HP, van Haaren PM, Van de Kamer JB, Zum Vorde Sive Vording PJ, Wiersma J, Hulshof MC, Geijsen ED, van Lanschot JJ, Crezee J. Prospective treatment planning to improve locoregional hyperthermia for oesophageal cancer. Int J Hyperthermia 2006; 22: 375–389
- Wust P, Nadobny J, Seebass M, Stalling D, Gellermann J, Hege HC, Deuflhard P, Felix R. Influence of patient models and numerical methods on predicted power deposition patterns. Int J Hyperthermia 1999; 15: 519–540
- Van de Kamer JB, Van Wieringen N, de Leeuw AA, Lagendijk JJ. The significance of accurate dielectric tissue data for hyperthermia treatment planning. Int J Hyperthermia 2001; 17: 123–142
- Crezee J, Van Wieringen N, Kroeze H, Lagendijk JJW. A prototype impedance probe for estimation of dielectric tissue properties for hyperthermia treatment planning. ESHO-99 Abstracts, RotterdamThe Netherlands 1999; 116
- Eyuboglu BM, Pilkington TC, Wolf PD. Estimation of tissue resistivities from multiple-electrode impedance measurements. Phys Med Biol 1994; 39: 1–17
- Meaney PM, Paulsen KD, Hartov A, Crane RK. An active microwave imaging system for reconstruction of 2-D electrical property distributions. IEEE Trans Biomed Eng 1995; 42: 1017–1026
- Farace P, Pontalti R, Cristoforetti L, Antolini R, Scarpa M. An automated method for mapping human tissue permittivities by MRI in hyperthermia treatment planning. Phys Med Biol 1997; 42: 2159–2174
- Joines WT. Frequency-dependent absorption of electromagnetic energy in biological tissue. IEEE Trans Biomed Eng 1984; 31: 17–20
- ESHO Taskgroup Committee. Treatment Planning and Modelling in Hyperthermia, a Task Group Report of the European Society for Hyperthermic Oncology. Tor Vergata, Rome 1992
- Gabriel C, Gabriel S, Corthout E. The dielectric properties of biological tissues: I. Literature survey. Phys Med Biol 1996; 41: 2231–2249
- Wiersma J, van Dijk JD. RF hyperthermia array modelling; Validation by means of measured EM-field distributions. Int J Hyperthermia 2001; 17: 63–81
- Clegg ST, Das SK, Zhang Y, Macfall J, Fullar E, Samulski TV. Verification of a hyperthermia model method using MR thermometry. Int J Hyperthermia 1995; 11: 409–424
- Van den Berg CA, Bartels LW, de Leeuw AA, Lagendijk JJ, Van de Kamer JB. Experimental validation of hyperthermia SAR treatment planning using MR B1+ imaging. Phys Med Biol 2004; 49: 5029–5042
- Crezee J, Lagendijk JJ. Experimental verification of bioheat transfer theories: Measurement of temperature profiles around large artificial vessels in perfused tissue. Phys Med Biol 1990; 35: 905–923
- Van den Berg CA, Bartels LW, van den BB, Kroeze H, de Leeuw AA, Van de Kamer JB, Lagendijk JJ. The use of MR B+1 imaging for validation of FDTD electromagnetic simulations of human anatomies. Phys Med Biol 2006; 51: 4735–4746
- Gellermann J, Wust P, Stalling D, Seebass M, Nadobny J, Beck R, Hege H, Deuflhard P, Felix R. Clinical evaluation and verification of the hyperthermia treatment planning system hyperplan. Int J Radiat Oncol Biol Phys 2000; 47: 1145–1156
- Sreenivasa G, Gellermann J, Rau B, Nadobny J, Schlag P, Deuflhard P, Felix R, Wust P. Clinical use of the hyperthermia treatment planning system HyperPlan to predict effectiveness and toxicity. Int J Radiat Oncol Biol Phys 2003; 55: 407–419
- van Dijk JD, Schneider C, van Os R, Blank LE, Gonzalez DG. Results of deep body hyperthermia with large waveguide radiators. Adv Exp Med Biol 1990; 267: 315–319
- Pieters BR, van der Grient JN, Blank LE, Koedooder K, Hulshof MC, de Reijke TM. Minimal displacement of novel self-anchoring catheters suitable for temporary prostate implants. Radiother Oncol 2006; 80: 69–72
- Schneider CJ, Olmi R, van Dijk JD. Phantom design: Applicability and physical properties. Principles and practice of thermoradiotherapy and thermochemotherapy, MH Seegenschmiedt, P Fessenden, CC Vernon. Springer Verlag, Berlin 1994; 381–397
- de Leeuw AA, Crezee J, Lagendijk JJ. Temperature and SAR measurements in deep-body hyperthermia with thermocouple thermometry. Int J Hyperthermia 1993; 9: 685–697
- Hornsleth SN, Mella O, Dahl O. A new segmentation algorithm for finite difference based treatment planning systems. Hyperthermic oncology, C Franconi, G Arcangeli, R Cavaliere. Tor Vergata, Rome 1996; 2: 521–523
- James BJ, Sullivan DM. Direct use of CT scans for hyperthermia treatment planning. IEEE Trans Biomed Eng 1992; 39: 845–851
- James BJ, Sullivan DM. Creation of three-dimensional patient models for hyperthermia treatment planning. IEEE Trans Biomed Eng 1992; 39: 238–242
- Van de Kamer JB, de Leeuw AA, Hornsleth SN, Kroeze H, Kotte AN, Lagendijk JJ. Development of a regional hyperthermia treatment planning system. Int J Hyperthermia 2001; 17: 207–220
- Van de Kamer JB, de Leeuw AA, Kroeze H, Lagendijk JJ. Quasistatic zooming for regional hyperthermia treatment planning. Phys Med Biol 2001; 46: 1017–1030
- Van de Kamer JB, Lagendijk JJ, de Leeuw AA, Kroeze H. High-resolution SAR modelling for regional hyperthermia: Testing quasistatic zooming at 10 MHz. Phys Med Biol 2001; 46: 183–196
- Van de Kamer JB, Kroeze H, de Leeuw AA, Lagendijk JJ. Quasistatic zooming of FDTD E-field computations: The impact of down-scaling techniques. Phys Med Biol 2001; 46: 1539–1551
- van Stam G, Wiersma J, Schneider CJ, Olmi R, Sijbrands J, van Dijk JD. Verification of calculated SAR distributions in an inhomogeneous phantom. In Franconi C, Arcangeli G, Cavaliere R, eds. Proceedings of the 7th International Congress on Hyperthermic Oncology. Papers presented at the conference (P 2b), Rome:Tor Vergata 1996; vol. 2: 501–503
- Stogryn A. Equations for calculating the dielectric constant of saline water. IEEE Trans Microw Theory Tech 1971; 19: 733–736
- Kok HP, Van den Berg CAT, Van Haaren PMA, Crezee J. Artefacts in intracavitary temperature measurements during regional hyperthermia. Phys Med Biol 2007
- Lagendijk JJ, van Rhoon GC, Hornsleth SN, Wust P, De Leeuw AC, Schneider CJ, van Dijk JD, van der Zee J, Heek-Romanowski R, Rahman SA, Gromoll C. ESHO quality assurance guidelines for regional hyperthermia. Int J Hyperthermia 1998; 14: 125–133
- van Vulpen M, de Leeuw AA, Van de Kamer JB, Kroeze H, Boon TA, Warlam-Rodenhuis CC, Lagendijk JJ, Battermann JJ. Comparison of intra-luminal versus intra-tumoural temperature measurements in patients with locally advanced prostate cancer treated with the coaxial TEM system: Report of a feasibility study. Int J Hyperthermia 2003; 19: 481–497
- van Haaren PM, Kok HP, Zum Vorde Sive Vording PJ, van Dijk JD, Hulshof MC, Fockens P, van Lanschot JJ, Crezee J. Reliability of temperature and SAR measurements at oesophageal tumour locations. Int J Hyperthermia 2006; 22: 545–561
- Van Haaren PMA, Kok HP, Wiersma J, Van de Kamer JB, van Dijk JDP, Crezee J. Faster EM-field calculations for locoregional hyperthermia treatment planning using the FDTD method. 21st Annual Meeting of the European Society for Hyperthermic Oncology, Munich. Germany 2003; 137–138, Abstract book
- Haaren PMA, Kok HP, van Stam G, Wiersma J, Van de Kamer JB, van Dijk JDP, Crezee J. SAR measurements and FDTD calculations in inhomogeneous phantom models. 9th International Congress on Hyperthermic Oncology. St. Louis, USA 2004; 167, Abstracts